Marine coastal ecosystem: Difference between revisions
Epipelagic (talk | contribs) →Overview: mission statement |
Epipelagic (talk | contribs) coastal predators |
||
Line 70: | Line 70: | ||
[[File:Global distribution of coral, mangrove, and seagrass diversity.png|thumb|upright=1|{{Center|Global distribution of coral, mangrove, and seagrass diversity}}]] |
[[File:Global distribution of coral, mangrove, and seagrass diversity.png|thumb|upright=1|{{Center|Global distribution of coral, mangrove, and seagrass diversity}}]] |
||
{{clear}} |
|||
==Coastal predators== |
|||
[[File:Effects of predators on coastal plant communities.jpg|thumb|upright=1.6| {{center|'''Effects of predators on coastal plant communities'''{{hsp}}<ref name=Atwood2018>{{cite journal |doi = 10.3389/fpls.2018.01289|doi-access = free|title = The Importance of Marine Predators in the Provisioning of Ecosystem Services by Coastal Plant Communities|year = 2018|last1 = Atwood|first1 = Trisha B.|last2 = Hammill|first2 = Edd|journal = Frontiers in Plant Science|volume = 9|page = 1289|pmid = 30233626|pmc = 6129962}} [[File:CC-BY icon.svg|50px]] Material was copied from this source, which is available under a [https://creativecommons.org/licenses/by/4.0/ Creative Commons Attribution 4.0 International License].</ref>}} Predicted effects of predators, or lack of predators, on ecosystem services (carbon sequestration, coastal protection, and ecosystem stability) in coastal plant communities. It is predicted that predators, through direct and indirect interactions with lower [[trophic level]]s, support increased carbon uptake in plants and soils, protect coasts from [[storm surge]]s and flooding, and support stability and resistance.<ref name=Atwood2018 />]] |
|||
[[Food web]] theory predicts that current global declines in marine predators could generate unwanted consequences for many marine ecosystems. In coastal plant communities, such as kelp, seagrass meadows, mangrove forests and salt marshes, several studies have documented the far-reaching effects of changing predator populations. Across coastal ecosystems, the loss of marine predators appears to negatively affect coastal plant communities and the ecosystem services they provide.<ref name=Atwood2018 /> |
|||
The green world hypothesis predicts loss of predator control on [[herbivore]]s could result in runaway consumption that would eventually denude a landscape or seascape of vegetation.<ref>{{cite journal | last=Hairston | first=Nelson G. | last2=Smith | first2=Frederick E. | last3=Slobodkin | first3=Lawrence B. | title=Community Structure, Population Control, and Competition | journal=The American Naturalist | publisher=University of Chicago Press | volume=94 | issue=879 | year=1960 | issn=0003-0147 | doi=10.1086/282146 | pages=421–425}}</ref> Since the inception of the green world hypothesis, ecologists have tried to understand the prevalence of indirect and alternating effects of predators on lower trophic levels ([[trophic cascade]]s), and their overall impact on ecosystems.<ref name=Estes2011>{{cite journal |doi = 10.1126/science.1205106|title = Trophic Downgrading of Planet Earth|year = 2011|last1 = Estes|first1 = James A.|last2 = Terborgh|first2 = John|last3 = Brashares|first3 = Justin S.|last4 = Power|first4 = Mary E.|last5 = Berger|first5 = Joel|last6 = Bond|first6 = William J.|last7 = Carpenter|first7 = Stephen R.|last8 = Essington|first8 = Timothy E.|last9 = Holt|first9 = Robert D.|last10 = Jackson|first10 = Jeremy B. C.|last11 = Marquis|first11 = Robert J.|last12 = Oksanen|first12 = Lauri|last13 = Oksanen|first13 = Tarja|last14 = Paine|first14 = Robert T.|last15 = Pikitch|first15 = Ellen K.|last16 = Ripple|first16 = William J.|last17 = Sandin|first17 = Stuart A.|last18 = Scheffer|first18 = Marten|last19 = Schoener|first19 = Thomas W.|last20 = Shurin|first20 = Jonathan B.|last21 = Sinclair|first21 = Anthony R. E.|last22 = Soulé|first22 = Michael E.|last23 = Virtanen|first23 = Risto|last24 = Wardle|first24 = David A.|journal = Science|volume = 333|issue = 6040|pages = 301–306|pmid = 21764740|bibcode = 2011Sci...333..301E|s2cid = 7752940}}</ref> Multiple lines of evidence now suggest that top predators are key to the persistence of some ecosystems.<ref name=Estes2011 /><ref name=Atwood2018 /> |
|||
With an estimated habitat loss greater than 50 percent, coastal plant communities are among the world’s most endangered ecosystems.<ref>{{cite journal |doi = 10.1146/annurev.energy.30.050504.144248|title = WETLAND RESOURCES: Status, Trends, Ecosystem Services, and Restorability|year = 2005|last1 = Zedler|first1 = Joy B.|last2 = Kercher|first2 = Suzanne|journal = Annual Review of Environment and Resources|volume = 30|pages = 39–74}}</ref><ref>{{cite journal |doi = 10.1073/pnas.0905620106|title = Accelerating loss of seagrasses across the globe threatens coastal ecosystems|year = 2009|last1 = Waycott|first1 = M.|last2 = Duarte|first2 = C. M.|last3 = Carruthers|first3 = T. J. B.|last4 = Orth|first4 = R. J.|last5 = Dennison|first5 = W. C.|last6 = Olyarnik|first6 = S.|last7 = Calladine|first7 = A.|last8 = Fourqurean|first8 = J. W.|last9 = Heck|first9 = K. L.|last10 = Hughes|first10 = A. R.|last11 = Kendrick|first11 = G. A.|last12 = Kenworthy|first12 = W. J.|last13 = Short|first13 = F. T.|last14 = Williams|first14 = S. L.|journal = Proceedings of the National Academy of Sciences|volume = 106|issue = 30|pages = 12377–12381|pmid = 19587236|pmc = 2707273|bibcode = 2009PNAS..10612377W|doi-access = free}}</ref><ref>{{cite journal |doi = 10.1038/nclimate1970|title = The role of coastal plant communities for climate change mitigation and adaptation|year = 2013|last1 = Duarte|first1 = Carlos M.|last2 = Losada|first2 = Iñigo J.|last3 = Hendriks|first3 = Iris E.|last4 = Mazarrasa|first4 = Inés|last5 = Marbà|first5 = Núria|journal = Nature Climate Change|volume = 3|issue = 11|pages = 961–968|bibcode = 2013NatCC...3..961D}}</ref> As bleak as this number is, the predators that patrol coastal systems have fared far worse. Several predatory taxa including species of [[marine mammal]]s, [[elasmobranch]]s, and [[seabird]]s have declined by 90 to 100 percent compared to historical populations.<ref name=Lotze2006>{{cite journal |doi = 10.1126/science.1128035|title = Depletion, Degradation, and Recovery Potential of Estuaries and Coastal Seas|year = 2006|last1 = Lotze|first1 = Heike K.|last2 = Lenihan|first2 = Hunter S.|last3 = Bourque|first3 = Bruce J.|last4 = Bradbury|first4 = Roger H.|last5 = Cooke|first5 = Richard G.|last6 = Kay|first6 = Matthew C.|last7 = Kidwell|first7 = Susan M.|last8 = Kirby|first8 = Michael X.|last9 = Peterson|first9 = Charles H.|last10 = Jackson|first10 = Jeremy B. C.|journal = Science|volume = 312|issue = 5781|pages = 1806–1809|pmid = 16794081|bibcode = 2006Sci...312.1806L|s2cid = 12703389}}</ref><ref>{{cite journal |doi = 10.1371/journal.pone.0129342|doi-access = free|title = Population Trend of the World's Monitored Seabirds, 1950-2010|year = 2015|last1 = Paleczny|first1 = Michelle|last2 = Hammill|first2 = Edd|last3 = Karpouzi|first3 = Vasiliki|last4 = Pauly|first4 = Daniel|journal = PLOS ONE|volume = 10|issue = 6|pages = e0129342|pmid = 26058068|pmc = 4461279|bibcode = 2015PLoSO..1029342P}}</ref> Predator declines pre-date habitat declines,<ref name=Lotze2006 /> suggesting alterations to predator populations may be a major driver of change for coastal systems.<ref>{{cite journal | last=Jackson | first=J. B. C. | title=What was natural in the coastal oceans? | journal=Proceedings of the National Academy of Sciences | publisher=Proceedings of the National Academy of Sciences | volume=98 | issue=10 | date=8 May 2001 | issn=0027-8424 | doi=10.1073/pnas.091092898 | pages=5411–5418}}</ref><ref>{{cite journal | last=Jackson | first=Jeremy B. C. | last2=Kirby | first2=Michael X. | last3=Berger | first3=Wolfgang H. | last4=Bjorndal | first4=Karen A. | last5=Botsford | first5=Louis W. | last6=Bourque | first6=Bruce J. | last7=Bradbury | first7=Roger H. | last8=Cooke | first8=Richard | last9=Erlandson | first9=Jon | last10=Estes | first10=James A. | last11=Hughes | first11=Terence P. | last12=Kidwell | first12=Susan | last13=Lange | first13=Carina B. | last14=Lenihan | first14=Hunter S. | last15=Pandolfi | first15=John M. | last16=Peterson | first16=Charles H. | last17=Steneck | first17=Robert S. | last18=Tegner | first18=Mia J. | last19=Warner | first19=Robert R. | title=Historical Overfishing and the Recent Collapse of Coastal Ecosystems | journal=Science | publisher=American Association for the Advancement of Science (AAAS) | volume=293 | issue=5530 | date=27 July 2001 | issn=0036-8075 | doi=10.1126/science.1059199 | pages=629–637}}</ref><ref name=Atwood2018 /> |
|||
There is little doubt that collapsing marine predator populations results from [[overharvesting]] by humans. Localized declines and extinctions of coastal predators by humans began over 40,000 years ago with subsistence harvesting.<ref>{{cite journal |doi = 10.1126/science.1255641|title = Marine defaunation: Animal loss in the global ocean|year = 2015|last1 = McCauley|first1 = Douglas J.|last2 = Pinsky|first2 = Malin L.|last3 = Palumbi|first3 = Stephen R.|last4 = Estes|first4 = James A.|last5 = Joyce|first5 = Francis H.|last6 = Warner|first6 = Robert R.|journal = Science|volume = 347|issue = 6219|pmid = 25593191|s2cid = 2500224|url = https://escholarship.org/uc/item/1gj9w82r}}</ref> However, for most large bodied, marine predators ([[toothed whale]]s, large [[pelagic fish]], sea birds, [[pinniped]]s, and [[otter]]s) the beginning of their sharp global declines occurred over the last century, coinciding with the expansion of coastal human populations and advances in [[industrial fishing]].<ref name=Lotze2006 /><ref>{{cite journal |doi = 10.1016/j.tree.2008.12.004|title = Historical baselines for large marine animals|year = 2009|last1 = Lotze|first1 = Heike K.|last2 = Worm|first2 = Boris|journal = Trends in Ecology & Evolution|volume = 24|issue = 5|pages = 254–262|pmid = 19251340}}</ref> Following global declines in marine predators, evidence of trophic cascades in coastal ecosystems started to emerge,<ref>{{cite journal |doi = 10.1126/science.185.4156.1058|title = Sea Otters: Their Role in Structuring Nearshore Communities|year = 1974|last1 = Estes|first1 = James A.|last2 = Palmisano|first2 = John F.|journal = Science|volume = 185|issue = 4156|pages = 1058–1060|pmid = 17738247|bibcode = 1974Sci...185.1058E|s2cid = 35892592}}</ref><ref>{{cite journal |doi = 10.3354/meps140071|title = Fish predation and the structure of the sea urchin Paracentrotus lividus populations in the NW Mediterranean|year = 1996|last1 = Sala|first1 = E.|last2 = Zabala|first2 = M.|journal = Marine Ecology Progress Series|volume = 140|pages = 71–81|bibcode = 1996MEPS..140...71S}}</ref><ref>{{cite journal |doi = 10.1126/science.1138657|title = Cascading Effects of the Loss of Apex Predatory Sharks from a Coastal Ocean|year = 2007|last1 = Myers|first1 = Ransom A.|last2 = Baum|first2 = Julia K.|last3 = Shepherd|first3 = Travis D.|last4 = Powers|first4 = Sean P.|last5 = Peterson|first5 = Charles H.|journal = Science|volume = 315|issue = 5820|pages = 1846–1850|pmid = 17395829|bibcode = 2007Sci...315.1846M|s2cid = 22332630}}</ref><ref>{{cite journal |doi = 10.3389/fmars.2014.00028|doi-access = free|title = Seagrasses in the age of sea turtle conservation and shark overfishing|year = 2014|last1 = Heithaus|first1 = Michael R.|last2 = Alcoverro|first2 = Teresa|last3 = Arthur|first3 = Rohan|last4 = Burkholder|first4 = Derek A.|last5 = Coates|first5 = Kathryn A.|last6 = Christianen|first6 = Marjolijn J. A.|last7 = Kelkar|first7 = Nachiket|last8 = Manuel|first8 = Sarah A.|last9 = Wirsing|first9 = Aaron J.|last10 = Kenworthy|first10 = W. Judson|last11 = Fourqurean|first11 = James W.|journal = Frontiers in Marine Science|volume = 1}}</ref> with the disturbing realisation that they affected more than just populations of lower trophic levels.<ref name=Estes2011 /><ref name=Atwood2018 /> |
|||
Understanding the importance of predators in coastal plant communities has been bolstered by their documented ability to influence ecosystem services. Multiple examples have shown that changes to the strength or direction of predator effects on lower trophic levels can influence [[coastal erosion]],<ref>{{cite journal |doi = 10.1371/journal.pone.0093296|doi-access = free|title = Indirect Human Impacts Reverse Centuries of Carbon Sequestration and Salt Marsh Accretion|year = 2014|last1 = Coverdale|first1 = Tyler C.|last2 = Brisson|first2 = Caitlin P.|last3 = Young|first3 = Eric W.|last4 = Yin|first4 = Stephanie F.|last5 = Donnelly|first5 = Jeffrey P.|last6 = Bertness|first6 = Mark D.|journal = PLOS ONE|volume = 9|issue = 3|pages = e93296|pmid = 24675669|pmc = 3968132|bibcode = 2014PLoSO...993296C}}</ref> [[carbon sequestration]],<ref>{{cite journal |doi = 10.1890/110176|title = Do trophic cascades affect the storage and flux of atmospheric carbon? An analysis of sea otters and kelp forests|year = 2012|last1 = Wilmers|first1 = Christopher C.|last2 = Estes|first2 = James A.|last3 = Edwards|first3 = Matthew|last4 = Laidre|first4 = Kristin L.|last5 = Konar|first5 = Brenda|journal = Frontiers in Ecology and the Environment|volume = 10|issue = 8|pages = 409–415}}</ref><ref name=Atwood2015>{{cite journal |doi = 10.1038/NCLIMATE2763|title = Predators help protect carbon stocks in blue carbon ecosystems|year = 2015|last1 = Atwood|first1 = Trisha B.|last2 = Connolly|first2 = Rod M.|last3 = Ritchie|first3 = Euan G.|last4 = Lovelock|first4 = Catherine E.|last5 = Heithaus|first5 = Michael R.|last6 = Hays|first6 = Graeme C.|last7 = Fourqurean|first7 = James W.|last8 = MacReadie|first8 = Peter I.|journal = Nature Climate Change|volume = 5|issue = 12|pages = 1038–1045|bibcode = 2015NatCC...5.1038A}}</ref> and [[Resilience (ecology)|ecosystem resilience]].<ref>{{cite journal | last=Hughes | first=Brent B. | last2=Hammerstrom | first2=Kamille K. | last3=Grant | first3=Nora E. | last4=Hoshijima | first4=Umi | last5=Eby | first5=Ron | last6=Wasson | first6=Kerstin | title=Trophic cascades on the edge: fostering seagrass resilience via a novel pathway | journal=Oecologia | publisher=Springer Science and Business Media LLC | volume=182 | issue=1 | date=11 May 2016 | issn=0029-8549 | doi=10.1007/s00442-016-3652-z | pages=231–241}}</ref> The idea that the [[extirpation]] of predators can have far-reaching effects on the persistence of coastal plants and their ecosystem services has become a major motivation for their conservation in coastal systems.<ref name=Estes2011 /><ref name=Atwood2015 /><ref name=Atwood2018 /> |
|||
{{clear}} |
{{clear}} |
Revision as of 07:11, 1 November 2021
Marine habitats |
---|
![]() |
Coastal habitats |
Ocean surface |
Open ocean |
Sea floor |
A marine coastal ecosystem is a marine ecosystem which occurs when the land meets the ocean. Marine coastal ecosystems include many different types of marine habitats, such as estuaries and lagoons, salt marshes and mangrove forests, seagrass meadows and coral reefs, kelp forests and backwaters. Directly and indirectly these provide a vast range of ecosystem services for humans, such as sequestering carbon, cycling nutrients and elements, providing nurseries and fishing grounds for commercial fisheries, preventing coastal erosion and moderating extreme events, as well as providing recreational services and supporting tourism.
Overview
Coastal seas are highly productive systems, providing an array of ecosystem services to humankind, such as processing of nutrient effluents from land and climate regulation.[1] However, coastal ecosystems are threatened by human-induced pressures such as climate change and eutrophication. In the coastal zone, the fluxes and transformations of nutrients and carbon sustaining coastal ecosystem functions and services are strongly regulated by benthic (that is, occurring at the seafloor) biological and chemical processes.[1]
Coastal systems also contribute to the regulation of climate and nutrient cycles, by efficiently processing anthropogenic emissions from land before they reach the ocean.[2][3][4][5] The high value of these ecosystem services is obvious considering that a large proportion of the world population lives close to the coast.[6][7][1]
Currently, coastal seas around the world are undergoing major ecological changes driven by human-induced pressures, such as climate change, anthropogenic nutrient inputs, overfishing and the spread of invasive species.[8][9] In many cases, the changes alter underlying ecological functions to such an extent that new states are achieved and baselines are shifted.[10][11][1]
In 2015, the United Nations established 17 Sustainable Development Goals with the aim of achieving certain targets by 2030. Their mission statement for their 14th goal, Life below water, is to "conserve and sustainably use the oceans, seas and marine resources for sustainable development".[12] The United Nations has also declared 2021–2030 the UN Decade on Ecosystem Restoration, but restoration of coastal ecosystems is not receiving appropriate attention.[13]
Intertidal zone

Intertidal zones are the areas that are visible and exposed to air during low tide and covered up by saltwater during high tide.[14] There are four physical divisions of the intertidal zone with each one having its distinct characteristics and wildlife. These divisions are the Spray zone, High intertidal zone, Middle Intertidal zone, and Low intertidal zone. The Spray zone is a damp area that is usually only reached by the ocean and submerged only under high tides or storms. The high intertidal zone is submerged at high tide but remains dry for long periods between high tides.[14] Due to the large variance of conditions possible in this region, it is inhabited by resilient wildlife that can withstand these changes such as barnacles, marine snails, mussels and hermit crabs.[14] Tides flow over the middle intertidal zone two times a day and this zone has a larger variety of wildlife.[14] The low intertidal zone is submerged nearly all the time except during the lowest tides and life is more abundant here due to the protection that the water gives.[14]
Coastal wetlands
Coastal wetlands are among the most productive ecosystems on Earth and generate vital services that benefit human societies around the world. Sediment-stabilization by wetlands such as salt marshes and mangroves serves to protect coastal communities from storm-waves, flooding, and land erosion.[15] Coastal wetlands also reduce pollution from human waste,[16][17] remove excess nutrients from the water column,[18] trap pollutants,[19] and sequester carbon.[20] Further, near-shore wetlands act as both essential nursery habitats and feeding grounds for game fish, supporting a diverse group of economically important species.[21][22][23][24][25]
Salt marshes

Salt marshes are a transition from the ocean to the land, where fresh and saltwater mix.[26] The soil in these marshes is often made up of mud and a layer of organic material called peat. Peat is characterized as waterlogged and root-filled decomposing plant matter that often causes low oxygen levels (hypoxia). These hypoxic conditions causes growth of the bacteria that also gives salt marshes the sulfurous smell they are often known for.[27] Salt marshes exist around the world and are needed for healthy ecosystems and a healthy economy. They are extremely productive ecosystems and they provide essential services for more than 75 percent of fishery species and protect shorelines from erosion and flooding.[27] Salt marshes can be generally divided into the high marsh, low marsh, and the upland border. The low marsh is closer to the ocean, with it being flooded at nearly every tide except low tide.[26] The high marsh is located between the low marsh and the upland border and it usually only flooded when higher than usual tides are present.[26] The upland border is the freshwater edge of the marsh and is usually located at elevations slightly higher than the high marsh. This region is usually only flooded under extreme weather conditions and experiences much less waterlogged conditions and salt stress than other areas of the marsh.[26]
Mangrove forests

Mangroves are trees or shrubs that grow in low-oxygen soil near coastlines in tropical or subtropical latitudes.[28] They are an extremely productive and complex ecosystem that connects the land and sea. Mangroves consist of species that are not necessarily related to each other and are often grouped for the characteristics they share rather than genetic similarity.[29] Because of their proximity to the coast, they have all developed adaptions such as salt excretion and root aeration to live in salty, oxygen-depleted water.[29] Mangroves can often be recognized by their dense tangle of roots that act to protect the coast by reducing erosion from storm surges, currents, wave, and tides.[28] The mangrove ecosystem is also an important source of food for many species as well as excellent at sequestering carbon dioxide from the atmosphere with global mangrove carbon storage is estimated at 34 million metric tons per year.[29]
Estuaries
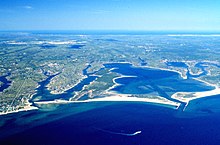
Estuaries occur where there is a noticeable change in salinity between saltwater and freshwater sources. This is typically found where rivers meet the ocean or sea. The wildlife found within estuaries is unique as the water in these areas is brackish - a mix of freshwater flowing to the ocean and salty seawater.[30] Other types of estuaries also exist and have similar characteristics as traditional brackish estuaries. The Great Lakes are a prime example. There, river water mixes with lake water and creates freshwater estuaries.[30] Estuaries are extremely productive ecosystems that many humans and animal species rely on for various activities.[31] This can be seen as, of the 32 largest cities in the world, 22 are located on estuaries as they provide many environmental and economic benefits such as crucial habitat for many species, and being economic hubs for many coastal communities.[31] Estuaries also provide essential ecosystem services such as water filtration, habitat protection, erosion control, gas regulation nutrient cycling, and it even gives education, recreation and tourism opportunities to people.[32]

Lagoons
Lagoons are areas that are separated from larger water by natural barriers such as coral reefs or sandbars. There are two types of lagoons, coastal and oceanic/atoll lagoons.[33] A coastal lagoon is, as the definition above, simply a body of water that is separated from the ocean by a barrier. An atoll lagoon is a circular coral reef or several coral islands that surround a lagoon. Atoll lagoons are often much deeper than coastal lagoons.[34] Most lagoons are very shallow meaning that they are greatly affected by changed in precipitation, evaporation and wind. This means that salinity and temperature are widely varied in lagoons and that they can have water that ranges from fresh to hypersaline.[34] Lagoons can be found in on coasts all over the world, on every continent except Antarctica and is an extremely diverse habitat being home to a wide array of species including birds, fish, crabs, plankton and more.[34] Lagoons are also important to the economy as they provide a wide array of ecosystem services in addition to being the home of so many different species. Some of these services include fisheries, nutrient cycling, flood protection, water filtration, and even human tradition.[34]
Seagrass meadows

Seagrasses form dense underwater meadows which are among the most productive ecosystems in the world. They provide habitats and food for a diversity of marine life comparable to coral reefs. This includes invertebrates like shrimp and crabs, cod and flatfish, marine mammals and birds. They provide refuges for endangered species such as seahorses, turtles, and dugongs. They function as nursery habitats for shrimps, scallops and many commercial fish species. Seagrass meadows provide coastal storm protection by the way their leaves absorb energy from waves as they hit the coast. They keep coastal waters healthy by absorbing bacteria and nutrients, and slow the speed of climate change by sequestering carbon dioxide into the sediment of the ocean floor.
Seagrasses evolved from marine algae which colonized land and became land plants, and then returned to the ocean about 100 million years ago. However, today seagrass meadows are being damaged by human activities such as pollution from land runoff, fishing boats that drag dredges or trawls across the meadows uprooting the grass, and overfishing which unbalances the ecosystem. Seagrass meadows are currently being destroyed at a rate of about two football fields every hour.
Coral reefs
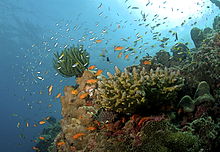
Coral reefs are one of the most well-known marine ecosystems in the world, with the largest being the Great Barrier Reef. These reefs are composed of large coral colonies of a variety of species living together. The corals from multiple symbiotic relationships with the organisms around them.[35]
Kelp forests

Kelp forests occur worldwide throughout temperate and polar coastal oceans.[36] In 2007, kelp forests were also discovered in tropical waters near Ecuador.[37]
Physically formed by brown macroalgae, kelp forests provide a unique habitat for marine organisms[38] and are a source for understanding many ecological processes. Over the last century, they have been the focus of extensive research, particularly in trophic ecology, and continue to provoke important ideas that are relevant beyond this unique ecosystem. For example, kelp forests can influence coastal oceanographic patterns[39] and provide many ecosystem services.[40]
However, the influence of humans has often contributed to kelp forest degradation. Of particular concern are the effects of overfishing nearshore ecosystems, which can release herbivores from their normal population regulation and result in the overgrazing of kelp and other algae.[41] This can rapidly result in transitions to barren landscapes where relatively few species persist.[42][43] Already due to the combined effects of overfishing and climate change, kelp forests have all but disappeared in many especially vulnerable places, such as Tasmania's east coast and the coast of Northern California.[44][45] The implementation of marine protected areas is one management strategy useful for addressing such issues, since it may limit the impacts of fishing and buffer the ecosystem from additive effects of other environmental stressors.

Coastal predators

Food web theory predicts that current global declines in marine predators could generate unwanted consequences for many marine ecosystems. In coastal plant communities, such as kelp, seagrass meadows, mangrove forests and salt marshes, several studies have documented the far-reaching effects of changing predator populations. Across coastal ecosystems, the loss of marine predators appears to negatively affect coastal plant communities and the ecosystem services they provide.[46]
The green world hypothesis predicts loss of predator control on herbivores could result in runaway consumption that would eventually denude a landscape or seascape of vegetation.[47] Since the inception of the green world hypothesis, ecologists have tried to understand the prevalence of indirect and alternating effects of predators on lower trophic levels (trophic cascades), and their overall impact on ecosystems.[48] Multiple lines of evidence now suggest that top predators are key to the persistence of some ecosystems.[48][46]
With an estimated habitat loss greater than 50 percent, coastal plant communities are among the world’s most endangered ecosystems.[49][50][51] As bleak as this number is, the predators that patrol coastal systems have fared far worse. Several predatory taxa including species of marine mammals, elasmobranchs, and seabirds have declined by 90 to 100 percent compared to historical populations.[52][53] Predator declines pre-date habitat declines,[52] suggesting alterations to predator populations may be a major driver of change for coastal systems.[54][55][46]
There is little doubt that collapsing marine predator populations results from overharvesting by humans. Localized declines and extinctions of coastal predators by humans began over 40,000 years ago with subsistence harvesting.[56] However, for most large bodied, marine predators (toothed whales, large pelagic fish, sea birds, pinnipeds, and otters) the beginning of their sharp global declines occurred over the last century, coinciding with the expansion of coastal human populations and advances in industrial fishing.[52][57] Following global declines in marine predators, evidence of trophic cascades in coastal ecosystems started to emerge,[58][59][60][61] with the disturbing realisation that they affected more than just populations of lower trophic levels.[48][46]
Understanding the importance of predators in coastal plant communities has been bolstered by their documented ability to influence ecosystem services. Multiple examples have shown that changes to the strength or direction of predator effects on lower trophic levels can influence coastal erosion,[62] carbon sequestration,[63][64] and ecosystem resilience.[65] The idea that the extirpation of predators can have far-reaching effects on the persistence of coastal plants and their ecosystem services has become a major motivation for their conservation in coastal systems.[48][64][46]
Biogeochemistry
Globally, eutrophication is one of the major environmental problems in coastal ecosystems. Over the last century the annual riverine inputs of nitrogen and phosphorus to the oceans have increased from 19 to 37 megatonnes of nitrogen and from 2 to 4 megatonnes of phosphorus.[66] Regionally, these increases were even more substantial as observed in the United States, Europe and China. In the Baltic Sea nitrogen and phosphorus loads increased by roughly a factor of three and six, respectively.[67] The riverine nitrogen flux has increased by an order of magnitude to coastal waters of China within thirty years, while phosphorus export has tripled between 1970 and 2000.[68][69][1]
Efforts to mitigate eutrophication through nutrient load reductions are hampered by the effects of climate change.[9] Changes in precipitation increase the runoff of N, P and carbon (C) from land, which together with warming and increased CO2 dissolution alter the coupled marine nutrient and carbon cycles.[70][71][1]
In contrast to the open ocean where biogeochemical cycling is largely dominated by pelagic processes driven primarily by ocean circulation, in the coastal zone, pelagic and benthic processes interact strongly and are driven by a complex and dynamic physical environment.[72] Eutrophication in coastal areas leads to shifts toward rapidly growing opportunistic algae, and generally to a decline in benthic macrovegetation because of decreased light penetration, substrate change and more reducing sediments.[73][74] Increased production and warming waters have caused expanding hypoxia at the seafloor with a consequent loss of benthic fauna.[75][76] Hypoxic systems tend to lose many long-lived higher organisms and biogeochemical cycles typically become dominated by benthic bacterial processes and rapid pelagic turnover.[77] However, if hypoxia does not occur, benthic fauna tends to increase in biomass with eutrophication.[78][79][80][1]

controlling benthic biogeochemical fluxes [1]

of benthic animals [1]
with links to carbon, nitrogen, phosphorus and oxygen cycles. POM = particulate organic matter. DIN, DIP = dissolved inorganic nitrogen and phosphorus respectively
Changes in benthic biota have far-reaching impacts on biogeochemical cycles in the coastal zone and beyond. In the illuminated zone, benthic microphytes and macrophytes mediate biogeochemical fluxes through primary production, nutrient storage and sediment stabilization and act as a habitat and food source for a variety of animals, as shown in the diagram on the left above. Benthic animals contribute to biogeochemical transformations and fluxes between water and sediments both directly through their metabolism and indirectly by physically reworking the sediments and their porewaters and stimulating bacterial processes. Grazing on pelagic organic matter and biodeposition of feces and pseudofeces by suspension-feeding fauna increases organic matter sedimentation rates.[81][82] In addition, nutrients and carbon are retained in biomass and transformed from organic to inorganic forms through metabolic processes.[83][80][84] Bioturbation, including sediment reworking and burrow ventilation activities (bioirrigation), redistributes particles and solutes within the sediment and enhances sediment-water fluxes of solutes.[85][86] Bioturbation can also enhance resuspension of particles, a phenomenon termed "bioresuspension".[87] Together, all these processes affect physical and chemical conditions at the sediment-water interface,[88] and strongly influence organic matter degradation.[89] When up-scaled to the ecosystem level, such modified conditions can significantly alter the functioning of coastal ecosystems and ultimately, the role of the coastal zone in filtering and transforming nutrients and carbon.[1]
References
- ^ a b c d e f g h i j Ehrnsten, Eva; Sun, Xiaole; Humborg, Christoph; Norkko, Alf; Savchuk, Oleg P.; Slomp, Caroline P.; Timmermann, Karen; Gustafsson, Bo G. (2020). "Understanding Environmental Changes in Temperate Coastal Seas: Linking Models of Benthic Fauna to Carbon and Nutrient Fluxes". Frontiers in Marine Science. 7. doi:10.3389/fmars.2020.00450.
Material was copied from this source, which is available under a Creative Commons Attribution 4.0 International License.
- ^ Tappin, A.D. (2002). "An Examination of the Fluxes of Nitrogen and Phosphorus in Temperate and Tropical Estuaries: Current Estimates and Uncertainties". Estuarine, Coastal and Shelf Science. 55 (6): 885–901. Bibcode:2002ECSS...55..885T. doi:10.1006/ecss.2002.1034.
- ^ Laruelle, G. G.; Roubeix, V.; Sferratore, A.; Brodherr, B.; Ciuffa, D.; Conley, D. J.; Dürr, H. H.; Garnier, J.; Lancelot, C.; Le Thi Phuong, Q.; Meunier, J.-D.; Meybeck, M.; Michalopoulos, P.; Moriceau, B.; Ní Longphuirt, S.; Loucaides, S.; Papush, L.; Presti, M.; Ragueneau, O.; Regnier, P.; Saccone, L.; Slomp, C. P.; Spiteri, C.; Van Cappellen, P. (2009). "Anthropogenic perturbations of the silicon cycle at the global scale: Key role of the land-ocean transition". Global Biogeochemical Cycles. 23 (4): n/a. Bibcode:2009GBioC..23.4031L. doi:10.1029/2008GB003267.
- ^ Regnier, Pierre; Friedlingstein, Pierre; Ciais, Philippe; MacKenzie, Fred T.; Gruber, Nicolas; Janssens, Ivan A.; Laruelle, Goulven G.; Lauerwald, Ronny; Luyssaert, Sebastiaan; Andersson, Andreas J.; Arndt, Sandra; Arnosti, Carol; Borges, Alberto V.; Dale, Andrew W.; Gallego-Sala, Angela; Goddéris, Yves; Goossens, Nicolas; Hartmann, Jens; Heinze, Christoph; Ilyina, Tatiana; Joos, Fortunat; Larowe, Douglas E.; Leifeld, Jens; Meysman, Filip J. R.; Munhoven, Guy; Raymond, Peter A.; Spahni, Renato; Suntharalingam, Parvadha; Thullner, Martin (2013). "Anthropogenic perturbation of the carbon fluxes from land to ocean". Nature Geoscience. 6 (8): 597–607. Bibcode:2013NatGe...6..597R. doi:10.1038/ngeo1830.
- ^ Ramesh, R.; Chen, Z.; Cummins, V.; Day, J.; d'Elia, C.; Dennison, B.; Forbes, D.L.; Glaeser, B.; Glaser, M.; Glavovic, B.; Kremer, H.; Lange, M.; Larsen, J.N.; Le Tissier, M.; Newton, A.; Pelling, M.; Purvaja, R.; Wolanski, E. (2015). "Land–Ocean Interactions in the Coastal Zone: Past, present & future". Anthropocene. 12: 85–98. doi:10.1016/j.ancene.2016.01.005.
- ^ Costanza, Robert; d'Arge, Ralph; De Groot, Rudolf; Farber, Stephen; Grasso, Monica; Hannon, Bruce; Limburg, Karin; Naeem, Shahid; O'Neill, Robert V.; Paruelo, Jose; Raskin, Robert G.; Sutton, Paul; Van Den Belt, Marjan (1997). "The value of the world's ecosystem services and natural capital". Nature. 387 (6630): 253–260. Bibcode:1997Natur.387..253C. doi:10.1038/387253a0. S2CID 672256.
- ^ Costanza, Robert; De Groot, Rudolf; Sutton, Paul; Van Der Ploeg, Sander; Anderson, Sharolyn J.; Kubiszewski, Ida; Farber, Stephen; Turner, R. Kerry (2014). "Changes in the global value of ecosystem services". Global Environmental Change. 26: 152–158. doi:10.1016/j.gloenvcha.2014.04.002.
- ^ Halpern, Benjamin S.; Walbridge, Shaun; Selkoe, Kimberly A.; Kappel, Carrie V.; Micheli, Fiorenza; d'Agrosa, Caterina; Bruno, John F.; Casey, Kenneth S.; Ebert, Colin; Fox, Helen E.; Fujita, Rod; Heinemann, Dennis; Lenihan, Hunter S.; Madin, Elizabeth M. P.; Perry, Matthew T.; Selig, Elizabeth R.; Spalding, Mark; Steneck, Robert; Watson, Reg (2008). "A Global Map of Human Impact on Marine Ecosystems". Science. 319 (5865): 948–952. Bibcode:2008Sci...319..948H. doi:10.1126/science.1149345. PMID 18276889. S2CID 26206024.
- ^ a b Cloern, James E.; Abreu, Paulo C.; Carstensen, Jacob; Chauvaud, Laurent; Elmgren, Ragnar; Grall, Jacques; Greening, Holly; Johansson, John Olov Roger; Kahru, Mati; Sherwood, Edward T.; Xu, Jie; Yin, Kedong (2016). "Human activities and climate variability drive fast-paced change across the world's estuarine-coastal ecosystems". Global Change Biology. 22 (2): 513–529. Bibcode:2016GCBio..22..513C. doi:10.1111/gcb.13059. PMID 26242490.
- ^ Lotze, Heike K.; Lenihan, Hunter S.; Bourque, Bruce J.; Bradbury, Roger H.; Cooke, Richard G.; Kay, Matthew C.; Kidwell, Susan M.; Kirby, Michael X.; Peterson, Charles H.; Jackson, Jeremy B. C. (2006). "Depletion, Degradation, and Recovery Potential of Estuaries and Coastal Seas". Science. 312 (5781): 1806–1809. Bibcode:2006Sci...312.1806L. doi:10.1126/science.1128035. PMID 16794081. S2CID 12703389.
- ^ Kemp, W. M.; Testa, J. M.; Conley, D. J.; Gilbert, D.; Hagy, J. D. (2009). "Temporal responses of coastal hypoxia to nutrient loading and physical controls". Biogeosciences. 6 (12): 2985–3008. Bibcode:2009BGeo....6.2985K. doi:10.5194/bg-6-2985-2009.
{{cite journal}}
: CS1 maint: unflagged free DOI (link) - ^ United Nations (2017) Resolution adopted by the General Assembly on 6 July 2017, Work of the Statistical Commission pertaining to the 2030 Agenda for Sustainable Development (A/RES/71/313)
- ^ Waltham, Nathan J.; Elliott, Michael; Lee, Shing Yip; Lovelock, Catherine; Duarte, Carlos M.; Buelow, Christina; Simenstad, Charles; Nagelkerken, Ivan; Claassens, Louw; Wen, Colin K-C; Barletta, Mario (2020). "UN Decade on Ecosystem Restoration 2021–2030—What Chance for Success in Restoring Coastal Ecosystems?". Frontiers in Marine Science. 7: 71. doi:10.3389/fmars.2020.00071. hdl:2440/123896. ISSN 2296-7745.
- ^ a b c d e US Department of Commerce, National Oceanic and Atmospheric Administration. "What is the intertidal zone?". oceanservice.noaa.gov. Retrieved 2019-03-21.
- ^ Gedan, Keryn B.; Kirwan, Matthew L.; Wolanski, Eric; Barbier, Edward B.; Silliman, Brian R. (2011). "The present and future role of coastal wetland vegetation in protecting shorelines: Answering recent challenges to the paradigm". Climatic Change. 106: 7–29. doi:10.1007/s10584-010-0003-7. S2CID 17867808.
- ^ Kadlec, Robert (2009). Treatment wetlands. Boca Raton, FL. ISBN 978-1-4200-1251-4. OCLC 311307374.
{{cite book}}
: CS1 maint: location missing publisher (link) - ^ Yang, Q.; Tam, N.F.Y.; Wong, Y.S.; Luan, T.G.; Su, W.S.; Lan, C.Y.; Shin, P.K.S.; Cheung, S.G. (2008). "Potential use of mangroves as constructed wetland for municipal sewage treatment in Futian, Shenzhen, China". Marine Pollution Bulletin. 57 (6–12): 735–743. doi:10.1016/j.marpolbul.2008.01.037. PMID 18342338.
- ^ Ouyang, Xiaoguang; Guo, Fen (2016). "Paradigms of mangroves in treatment of anthropogenic wastewater pollution". Science of the Total Environment. 544: 971–979. doi:10.1016/j.scitotenv.2015.12.013. PMID 26706768.
- ^ Gambrell, R. P. (1994). "Trace and Toxic Metals in Wetlands-A Review". Journal of Environmental Quality. 23 (5): 883–891. doi:10.2134/jeq1994.00472425002300050005x.
- ^ McLeod, Elizabeth; Chmura, Gail L.; Bouillon, Steven; Salm, Rodney; Björk, Mats; Duarte, Carlos M.; Lovelock, Catherine E.; Schlesinger, William H.; Silliman, Brian R. (2011). "A blueprint for blue carbon: Toward an improved understanding of the role of vegetated coastal habitats in sequestering CO 2". Frontiers in Ecology and the Environment. 9 (10): 552–560. doi:10.1890/110004.
- ^ Lipcius, Romuald N.; Seitz, Rochelle D.; Seebo, Michael S.; Colón-Carrión, Duamed (2005). "Density, abundance and survival of the blue crab in seagrass and unstructured salt marsh nurseries of Chesapeake Bay". Journal of Experimental Marine Biology and Ecology. 319 (1–2): 69–80. doi:10.1016/j.jembe.2004.12.034.
- ^ Mumby, Peter J. (2006). "Connectivity of reef fish between mangroves and coral reefs: Algorithms for the design of marine reserves at seascape scales". Biological Conservation. 128 (2): 215–222. doi:10.1016/j.biocon.2005.09.042.
- ^ Aburto-Oropeza, O.; Ezcurra, E.; Danemann, G.; Valdez, V.; Murray, J.; Sala, E. (2008). "Mangroves in the Gulf of California increase fishery yields". Proceedings of the National Academy of Sciences. 105 (30): 10456–10459. doi:10.1073/pnas.0804601105. PMC 2492483. PMID 18645185.
- ^ Nagelkerken, I.; Blaber, S.J.M.; Bouillon, S.; Green, P.; Haywood, M.; Kirton, L.G.; Meynecke, J.-O.; Pawlik, J.; Penrose, H.M.; Sasekumar, A.; Somerfield, P.J. (2008). "The habitat function of mangroves for terrestrial and marine fauna: A review". Aquatic Botany. 89 (2): 155–185. doi:10.1016/j.aquabot.2007.12.007.
- ^ Renzi, Julianna J.; He, Qiang; Silliman, Brian R. (2019). "Harnessing Positive Species Interactions to Enhance Coastal Wetland Restoration". Frontiers in Ecology and Evolution. 7. doi:10.3389/fevo.2019.00131.
Material was copied from this source, which is available under a Creative Commons Attribution 4.0 International License.
- ^ a b c d "What is a Salt Marsh?" (PDF). New Hampshire Department of Environmental Services. 2004.
- ^ a b US Department of Commerce, National Oceanic and Atmospheric Administration. "What is a salt marsh?". oceanservice.noaa.gov. Retrieved 2019-03-20.
- ^ a b US Department of Commerce, National Oceanic and Atmospheric Administration. "What is a mangrove forest?". oceanservice.noaa.gov. Retrieved 2019-03-21.
- ^ a b c "Mangroves". Smithsonian Ocean. Retrieved 2019-03-21.
- ^ a b US Department of Commerce, National Oceanic and Atmospheric Administration. "What is an estuary?". oceanservice.noaa.gov. Retrieved 2019-03-22.
- ^ a b US Department of Commerce, National Oceanic and Atmospheric Administration. "Estuaries, NOS Education Offering". oceanservice.noaa.gov. Retrieved 2019-03-22.
- ^ "Estuaries". www.crd.bc.ca. 2013-11-14. Retrieved 2019-03-24.
- ^ US Department of Commerce, National Oceanic and Atmospheric Administration. "What is a lagoon?". oceanservice.noaa.gov. Retrieved 2019-03-24.
- ^ a b c d Miththapala, Sriyanie (2013). "Lagoons and Estuaries" (PDF). IUCN, International Union for Conservation of Nature.
- ^ "Corals and Coral Reefs". Ocean Portal | Smithsonian. 2012-09-12. Retrieved 2018-03-27.
- ^ Mann, K.H. 1973. Seaweeds: their productivity and strategy for growth. Science 182: 975-981.
- ^ Graham, M.H., B.P. Kinlan, L.D. Druehl, L.E. Garske, and S. Banks. 2007. Deep-water kelp refugia as potential hotspots of tropical marine diversity and productivity. Proceedings of the National Academy of Sciences 104: 16576-16580.
- ^ Christie, H., Jørgensen, N.M., Norderhaug, K.M., Waage-Nielsen, E., 2003. Species distribution and habitat exploitation of fauna associated with kelp (Laminaria hyperborea) along the Norwegian coast. Journal of the Marine Biological Association of the UK 83, 687-699.
- ^ Jackson, G.A. and C.D. Winant. 1983. Effect of a kelp forest on coastal currents. Continental Shelf Report 2: 75-80.
- ^ Steneck, R.S., M.H. Graham, B.J. Bourque, D. Corbett, J.M. Erlandson, J.A. Estes and M.J. Tegner. 2002. Kelp forest ecosystems: biodiversity, stability, resilience and future. Environmental Conservation 29: 436-459.
- ^ Sala, E., C.F. Bourdouresque and M. Harmelin-Vivien. 1998. Fishing, trophic cascades, and the structure of algal assemblages: evaluation of an old but untested paradigm. Oikos 82: 425-439.
- ^ Dayton, P.K. 1985a. Ecology of kelp communities. Annual Review of Ecology and Systematics 16: 215-245.
- ^ Norderhaug, K.M., Christie, H., 2009. Sea urchin grazing and kelp re-vegetation in the NE Atlantic. Marine Biology Research 5, 515-528
- ^ Morton, Adam; Cordell, Marni; Fanner, David; Ball, Andy; Evershed, Nick. "The dead sea: Tasmania's underwater forests disappearing in our lifetime". the Guardian. Retrieved 2020-10-22.
- ^ Steinbauer, James. "What Will It Take to Bring Back the Kelp Forest? - Bay Nature Magazine". Bay Nature. Retrieved 2020-10-22.
- ^ a b c d e f g Atwood, Trisha B.; Hammill, Edd (2018). "The Importance of Marine Predators in the Provisioning of Ecosystem Services by Coastal Plant Communities". Frontiers in Plant Science. 9: 1289. doi:10.3389/fpls.2018.01289. PMC 6129962. PMID 30233626.
Material was copied from this source, which is available under a Creative Commons Attribution 4.0 International License.
- ^ Hairston, Nelson G.; Smith, Frederick E.; Slobodkin, Lawrence B. (1960). "Community Structure, Population Control, and Competition". The American Naturalist. 94 (879). University of Chicago Press: 421–425. doi:10.1086/282146. ISSN 0003-0147.
- ^ a b c d Estes, James A.; Terborgh, John; Brashares, Justin S.; Power, Mary E.; Berger, Joel; Bond, William J.; Carpenter, Stephen R.; Essington, Timothy E.; Holt, Robert D.; Jackson, Jeremy B. C.; Marquis, Robert J.; Oksanen, Lauri; Oksanen, Tarja; Paine, Robert T.; Pikitch, Ellen K.; Ripple, William J.; Sandin, Stuart A.; Scheffer, Marten; Schoener, Thomas W.; Shurin, Jonathan B.; Sinclair, Anthony R. E.; Soulé, Michael E.; Virtanen, Risto; Wardle, David A. (2011). "Trophic Downgrading of Planet Earth". Science. 333 (6040): 301–306. Bibcode:2011Sci...333..301E. doi:10.1126/science.1205106. PMID 21764740. S2CID 7752940.
- ^ Zedler, Joy B.; Kercher, Suzanne (2005). "WETLAND RESOURCES: Status, Trends, Ecosystem Services, and Restorability". Annual Review of Environment and Resources. 30: 39–74. doi:10.1146/annurev.energy.30.050504.144248.
- ^ Waycott, M.; Duarte, C. M.; Carruthers, T. J. B.; Orth, R. J.; Dennison, W. C.; Olyarnik, S.; Calladine, A.; Fourqurean, J. W.; Heck, K. L.; Hughes, A. R.; Kendrick, G. A.; Kenworthy, W. J.; Short, F. T.; Williams, S. L. (2009). "Accelerating loss of seagrasses across the globe threatens coastal ecosystems". Proceedings of the National Academy of Sciences. 106 (30): 12377–12381. Bibcode:2009PNAS..10612377W. doi:10.1073/pnas.0905620106. PMC 2707273. PMID 19587236.
- ^ Duarte, Carlos M.; Losada, Iñigo J.; Hendriks, Iris E.; Mazarrasa, Inés; Marbà, Núria (2013). "The role of coastal plant communities for climate change mitigation and adaptation". Nature Climate Change. 3 (11): 961–968. Bibcode:2013NatCC...3..961D. doi:10.1038/nclimate1970.
- ^ a b c Lotze, Heike K.; Lenihan, Hunter S.; Bourque, Bruce J.; Bradbury, Roger H.; Cooke, Richard G.; Kay, Matthew C.; Kidwell, Susan M.; Kirby, Michael X.; Peterson, Charles H.; Jackson, Jeremy B. C. (2006). "Depletion, Degradation, and Recovery Potential of Estuaries and Coastal Seas". Science. 312 (5781): 1806–1809. Bibcode:2006Sci...312.1806L. doi:10.1126/science.1128035. PMID 16794081. S2CID 12703389.
- ^ Paleczny, Michelle; Hammill, Edd; Karpouzi, Vasiliki; Pauly, Daniel (2015). "Population Trend of the World's Monitored Seabirds, 1950-2010". PLOS ONE. 10 (6): e0129342. Bibcode:2015PLoSO..1029342P. doi:10.1371/journal.pone.0129342. PMC 4461279. PMID 26058068.
- ^ Jackson, J. B. C. (8 May 2001). "What was natural in the coastal oceans?". Proceedings of the National Academy of Sciences. 98 (10). Proceedings of the National Academy of Sciences: 5411–5418. doi:10.1073/pnas.091092898. ISSN 0027-8424.
- ^ Jackson, Jeremy B. C.; Kirby, Michael X.; Berger, Wolfgang H.; Bjorndal, Karen A.; Botsford, Louis W.; Bourque, Bruce J.; Bradbury, Roger H.; Cooke, Richard; Erlandson, Jon; Estes, James A.; Hughes, Terence P.; Kidwell, Susan; Lange, Carina B.; Lenihan, Hunter S.; Pandolfi, John M.; Peterson, Charles H.; Steneck, Robert S.; Tegner, Mia J.; Warner, Robert R. (27 July 2001). "Historical Overfishing and the Recent Collapse of Coastal Ecosystems". Science. 293 (5530). American Association for the Advancement of Science (AAAS): 629–637. doi:10.1126/science.1059199. ISSN 0036-8075.
- ^ McCauley, Douglas J.; Pinsky, Malin L.; Palumbi, Stephen R.; Estes, James A.; Joyce, Francis H.; Warner, Robert R. (2015). "Marine defaunation: Animal loss in the global ocean". Science. 347 (6219). doi:10.1126/science.1255641. PMID 25593191. S2CID 2500224.
- ^ Lotze, Heike K.; Worm, Boris (2009). "Historical baselines for large marine animals". Trends in Ecology & Evolution. 24 (5): 254–262. doi:10.1016/j.tree.2008.12.004. PMID 19251340.
- ^ Estes, James A.; Palmisano, John F. (1974). "Sea Otters: Their Role in Structuring Nearshore Communities". Science. 185 (4156): 1058–1060. Bibcode:1974Sci...185.1058E. doi:10.1126/science.185.4156.1058. PMID 17738247. S2CID 35892592.
- ^ Sala, E.; Zabala, M. (1996). "Fish predation and the structure of the sea urchin Paracentrotus lividus populations in the NW Mediterranean". Marine Ecology Progress Series. 140: 71–81. Bibcode:1996MEPS..140...71S. doi:10.3354/meps140071.
- ^ Myers, Ransom A.; Baum, Julia K.; Shepherd, Travis D.; Powers, Sean P.; Peterson, Charles H. (2007). "Cascading Effects of the Loss of Apex Predatory Sharks from a Coastal Ocean". Science. 315 (5820): 1846–1850. Bibcode:2007Sci...315.1846M. doi:10.1126/science.1138657. PMID 17395829. S2CID 22332630.
- ^ Heithaus, Michael R.; Alcoverro, Teresa; Arthur, Rohan; Burkholder, Derek A.; Coates, Kathryn A.; Christianen, Marjolijn J. A.; Kelkar, Nachiket; Manuel, Sarah A.; Wirsing, Aaron J.; Kenworthy, W. Judson; Fourqurean, James W. (2014). "Seagrasses in the age of sea turtle conservation and shark overfishing". Frontiers in Marine Science. 1. doi:10.3389/fmars.2014.00028.
- ^ Coverdale, Tyler C.; Brisson, Caitlin P.; Young, Eric W.; Yin, Stephanie F.; Donnelly, Jeffrey P.; Bertness, Mark D. (2014). "Indirect Human Impacts Reverse Centuries of Carbon Sequestration and Salt Marsh Accretion". PLOS ONE. 9 (3): e93296. Bibcode:2014PLoSO...993296C. doi:10.1371/journal.pone.0093296. PMC 3968132. PMID 24675669.
- ^ Wilmers, Christopher C.; Estes, James A.; Edwards, Matthew; Laidre, Kristin L.; Konar, Brenda (2012). "Do trophic cascades affect the storage and flux of atmospheric carbon? An analysis of sea otters and kelp forests". Frontiers in Ecology and the Environment. 10 (8): 409–415. doi:10.1890/110176.
- ^ a b Atwood, Trisha B.; Connolly, Rod M.; Ritchie, Euan G.; Lovelock, Catherine E.; Heithaus, Michael R.; Hays, Graeme C.; Fourqurean, James W.; MacReadie, Peter I. (2015). "Predators help protect carbon stocks in blue carbon ecosystems". Nature Climate Change. 5 (12): 1038–1045. Bibcode:2015NatCC...5.1038A. doi:10.1038/NCLIMATE2763.
- ^ Hughes, Brent B.; Hammerstrom, Kamille K.; Grant, Nora E.; Hoshijima, Umi; Eby, Ron; Wasson, Kerstin (11 May 2016). "Trophic cascades on the edge: fostering seagrass resilience via a novel pathway". Oecologia. 182 (1). Springer Science and Business Media LLC: 231–241. doi:10.1007/s00442-016-3652-z. ISSN 0029-8549.
- ^ Beusen, Arthur H. W.; Bouwman, Alexander F.; Van Beek, Ludovicus P. H.; Mogollón, José M.; Middelburg, Jack J. (2016). "Global riverine N and P transport to ocean increased during the 20th century despite increased retention along the aquatic continuum". Biogeosciences. 13 (8): 2441–2451. Bibcode:2016BGeo...13.2441B. doi:10.5194/bg-13-2441-2016.
{{cite journal}}
: CS1 maint: unflagged free DOI (link) - ^ Gustafsson, Bo G.; Schenk, Frederik; Blenckner, Thorsten; Eilola, Kari; Meier, H. E. Markus; Müller-Karulis, Bärbel; Neumann, Thomas; Ruoho-Airola, Tuija; Savchuk, Oleg P.; Zorita, Eduardo (2012). "Reconstructing the Development of Baltic Sea Eutrophication 1850–2006". Ambio. 41 (6): 534–548. doi:10.1007/s13280-012-0318-x. PMC 3428479. PMID 22926877.
- ^ Qu, Hong Juan; Kroeze, Carolien (4 August 2011). "Nutrient export by rivers to the coastal waters of China: management strategies and future trends". Regional Environmental Change. 12 (1). Springer Science and Business Media LLC: 153–167. doi:10.1007/s10113-011-0248-3. ISSN 1436-3798.
- ^ Cui, S.; Shi, Y.; Groffman, P. M.; Schlesinger, W. H.; Zhu, Y.-G. (2013). "Centennial-scale analysis of the creation and fate of reactive nitrogen in China (1910-2010)". Proceedings of the National Academy of Sciences. 110 (6): 2052–2057. Bibcode:2013PNAS..110.2052C. doi:10.1073/pnas.1221638110. PMC 3568337. PMID 23341613.
- ^ Falkowski, P.; Scholes, R. J.; Boyle, E.; Canadell, J.; Canfield, D.; Elser, J.; Gruber, N.; Hibbard, K.; Högberg, P.; Linder, S.; MacKenzie, F. T.; Moore Iii, B.; Pedersen, T.; Rosenthal, Y.; Seitzinger, S.; Smetacek, V.; Steffen, W. (2000). "The Global Carbon Cycle: A Test of Our Knowledge of Earth as a System". Science. 290 (5490): 291–296. Bibcode:2000Sci...290..291F. doi:10.1126/science.290.5490.291. PMID 11030643.
- ^ Gruber, Nicolas; Galloway, James N. (2008). "An Earth-system perspective of the global nitrogen cycle". Nature. 451 (7176): 293–296. Bibcode:2008Natur.451..293G. doi:10.1038/nature06592. PMID 18202647. S2CID 4318396.
- ^ Statham, Peter J. (2012). "Nutrients in estuaries — an overview and the potential impacts of climate change". Science of the Total Environment. 434: 213–227. Bibcode:2012ScTEn.434..213S. doi:10.1016/j.scitotenv.2011.09.088. PMID 22119025.
- ^ Cloern, JE (2001). "Our evolving conceptual model of the coastal eutrophication problem". Marine Ecology Progress Series. 210: 223–253. Bibcode:2001MEPS..210..223C. doi:10.3354/meps210223.
- ^ Grall, Jacques; Chauvaud, Laurent (2002). "Marine eutrophication and benthos: The need for new approaches and concepts". Global Change Biology. 8 (9): 813–830. Bibcode:2002GCBio...8..813G. doi:10.1046/j.1365-2486.2002.00519.x.
- ^ Diaz, Robert J.; Rosenberg, Rutger (2008). "Spreading Dead Zones and Consequences for Marine Ecosystems". Science. 321 (5891): 926–929. Bibcode:2008Sci...321..926D. doi:10.1126/science.1156401. PMID 18703733. S2CID 32818786.
- ^ Breitburg, Denise; Levin, Lisa A.; Oschlies, Andreas; Grégoire, Marilaure; Chavez, Francisco P.; Conley, Daniel J.; Garçon, Véronique; Gilbert, Denis; Gutiérrez, Dimitri; Isensee, Kirsten; Jacinto, Gil S.; Limburg, Karin E.; Montes, Ivonne; Naqvi, S. W. A.; Pitcher, Grant C.; Rabalais, Nancy N.; Roman, Michael R.; Rose, Kenneth A.; Seibel, Brad A.; Telszewski, Maciej; Yasuhara, Moriaki; Zhang, Jing (2018). "Declining oxygen in the global ocean and coastal waters". Science. 359 (6371). Bibcode:2018Sci...359M7240B. doi:10.1126/science.aam7240. PMID 29301986. S2CID 206657115.
- ^ Griffiths, Jennifer R.; Kadin, Martina; Nascimento, Francisco J. A.; Tamelander, Tobias; Törnroos, Anna; Bonaglia, Stefano; Bonsdorff, Erik; Brüchert, Volker; Gårdmark, Anna; Järnström, Marie; Kotta, Jonne; Lindegren, Martin; Nordström, Marie C.; Norkko, Alf; Olsson, Jens; Weigel, Benjamin; Žydelis, Ramunas; Blenckner, Thorsten; Niiranen, Susa; Winder, Monika (2017). "The importance of benthic-pelagic coupling for marine ecosystem functioning in a changing world". Global Change Biology. 23 (6): 2179–2196. Bibcode:2017GCBio..23.2179G. doi:10.1111/gcb.13642. PMID 28132408. S2CID 13874905.
- ^ Cederwall, H. and Elmgren, R. (1980) "Biomass increase of benthic macrofauna demonstrates eutrophication of the Baltic Sea". In: Symposium of the Baltic Marine Biologists: relationship and exchange between the pelagic and benthic biota.
- ^ Pearson, T. H., and Rosenberg, R. (1987). “Feast and famine: Stucturing factors in marine benthic communities,” in Organization Of Communities:The 27th Symposium of the British Ecological Society, Aberystwyth 1986, Eds: P. S. Giller and J. H. R. Gee (Oxford: Blackwell), 373–395.
- ^ a b Josefson, A.B.; Rasmussen, B. (2000). "Nutrient Retention by Benthic Macrofaunal Biomass of Danish Estuaries: Importance of Nutrient Load and Residence Time". Estuarine, Coastal and Shelf Science. 50 (2): 205–216. Bibcode:2000ECSS...50..205J. doi:10.1006/ecss.1999.0562.
- ^ Kautsky, N.; Evans, S. (1987). "Role of biodeposition by Mytilus edulis in the circulation of matter and nutrients in a Baltic coastal ecosystem". Marine Ecology Progress Series. 38: 201–212. Bibcode:1987MEPS...38..201K. doi:10.3354/meps038201.
- ^ Newell R.I. (2004) "Ecosystem influences of natural and cultivated populations of suspension-feeding bivalve molluscs: a review". Journal of Shellfish Research, 23(1): 51–62.
- ^ Herman, P.M.J.; Middelburg, J.J.; Van De Koppel, J.; Heip, C.H.R. (1999). "Ecology of Estuarine Macrobenthos". Estuaries. Advances in Ecological Research. Vol. 29. pp. 195–240. doi:10.1016/S0065-2504(08)60194-4. ISBN 9780120139293.
- ^ Ehrnsten, Eva; Norkko, Alf; Timmermann, Karen; Gustafsson, Bo G. (2019). "Benthic-pelagic coupling in coastal seas – Modelling macrofaunal biomass and carbon processing in response to organic matter supply". Journal of Marine Systems. 196: 36–47. Bibcode:2019JMS...196...36E. doi:10.1016/j.jmarsys.2019.04.003. S2CID 155821874.
- ^ Kristensen, E.; Penha-Lopes, G.; Delefosse, M.; Valdemarsen, T.; Quintana, CO; Banta, GT (2012). "What is bioturbation? The need for a precise definition for fauna in aquatic sciences". Marine Ecology Progress Series. 446: 285–302. Bibcode:2012MEPS..446..285K. doi:10.3354/meps09506.
- ^ Villnäs, Anna; Norkko, Joanna; Hietanen, Susanna; Josefson, Alf B.; Lukkari, Kaarina; Norkko, Alf (2013). "The role of recurrent disturbances for ecosystem multifunctionality". Ecology. 94 (10): 2275–2287. doi:10.1890/12-1716.1. hdl:10138/233616. PMID 24358713.
- ^ Le Hir, P.; Monbet, Y.; Orvain, F. (2007). "Sediment erodability in sediment transport modelling: Can we account for biota effects?". Continental Shelf Research. 27 (8): 1116–1142. Bibcode:2007CSR....27.1116L. doi:10.1016/j.csr.2005.11.016.
- ^ Stief, P. (2013). "Stimulation of microbial nitrogen cycling in aquatic ecosystems by benthic macrofauna: Mechanisms and environmental implications". Biogeosciences. 10 (12): 7829–7846. Bibcode:2013BGeo...10.7829S. doi:10.5194/bg-10-7829-2013.
{{cite journal}}
: CS1 maint: unflagged free DOI (link) - ^ Aller, Robert C. (1982). "Carbonate Dissolution in Nearshore Terrigenous Muds: The Role of Physical and Biological Reworking". The Journal of Geology. 90 (1). University of Chicago Press: 79–95. doi:10.1086/628652. ISSN 0022-1376.