Desert Fireball Network: Difference between revisions
No edit summary |
added camera hardware refs |
||
Line 93: | Line 93: | ||
== Camera Hardware == |
== Camera Hardware == |
||
[[File:Lambina DFN Station.jpg|alt=camera on stand with solar panels|thumb|430x430px|Lambina DFN Station: a typical outback fireball observatory]] |
[[File:Lambina DFN Station.jpg|alt=camera on stand with solar panels|thumb|430x430px|Lambina DFN Station: a typical outback fireball observatory]] |
||
The DFN observatories use consumer still photographic cameras (specifically [[Digital single-lens reflex camera|DSLRs]]) with 8mm [[Stereographic projection|stereographic]] [[Fisheye lens|fish-eye]] lenses covering nearly the entire sky from each station. The cameras are controlled via an embedded [[Linux]] PC using [[GPhoto|gPhoto2]] and images are archived to multiple hard disk drives for storage until the observatories are visited for maintenance (every 8–18 months depending on the storage capacity). |
The DFN observatories use consumer still photographic cameras (specifically [[Digital single-lens reflex camera|DSLRs]]) with 8mm [[Stereographic projection|stereographic]] [[Fisheye lens|fish-eye]] lenses covering nearly the entire sky from each station. The cameras are controlled via an embedded [[Linux]] PC using [[GPhoto|gPhoto2]] and images are archived to multiple hard disk drives for storage until the observatories are visited for maintenance (every 8–18 months depending on the storage capacity)<ref>{{Cite journal|last=Howie|first=Robert M.|last2=Paxman|first2=Jonathan|last3=Bland|first3=Philip A.|last4=Towner|first4=Martin C.|last5=Cupak|first5=Martin|last6=Sansom|first6=Eleanor K.|last7=Devillepoix|first7=Hadrien A. R.|date=2017-06-01|title=How to build a continental scale fireball camera network|url=https://link.springer.com/article/10.1007/s10686-017-9532-7|journal=Experimental Astronomy|language=en|volume=43|issue=3|pages=237–266|doi=10.1007/s10686-017-9532-7|issn=0922-6435}}</ref>. |
||
The observatories take one [[Long-exposure photography|long exposure]] image every 30 seconds for the entire night. After capture, automated event detection searches the images for fireballs, and events are corroborated on the central server using images from multiple stations. |
The observatories take one [[Long-exposure photography|long exposure]] image every 30 seconds for the entire night. After capture, automated event detection searches the images for fireballs, and events are corroborated on the central server using images from multiple stations. |
||
A [[Satellite navigation|GNSS]] syncronised time code is embedded in the long exposure images by the operation of a [[Twisted nematic field effect|liquid crystal]] (LC) shutter to provide absolute timing data for fireball trajectories after triangulation with temporal precision better than one millisecond. Absolute timing is used for the calculation of meteoroid orbits and the relative timing also embedded by the timecode is required for trajectory analysis (specifically to calculate the mass from the deceleration of the meteoroid). |
A [[Satellite navigation|GNSS]] syncronised time code is embedded in the long exposure images by the operation of a [[Twisted nematic field effect|liquid crystal]] (LC) shutter to provide absolute timing data for fireball trajectories after triangulation with temporal precision better than one millisecond<ref>{{Cite journal|last=Howie|first=Robert M.|last2=Paxman|first2=Jonathan|last3=Bland|first3=Philip A.|last4=Towner|first4=Martin C.|last5=Sansom|first5=Eleanor K.|last6=Devillepoix|first6=Hadrien A. R.|date=2017-08-01|title=Submillisecond fireball timing using de Bruijn timecodes|url=http://onlinelibrary.wiley.com/doi/10.1111/maps.12878/abstract|journal=Meteoritics & Planetary Science|language=en|volume=52|issue=8|pages=1669–1682|doi=10.1111/maps.12878|issn=1945-5100}}</ref>. Absolute timing is used for the calculation of meteoroid orbits and the relative timing also embedded by the timecode is required for trajectory analysis (specifically to calculate the mass from the deceleration of the meteoroid). |
||
[[File:DFNext Internals.jpg|alt=inside of observatory showing components|thumb|422x422px|Internals of the latest iteration of the DFN observatory design (as of August 2017) displaying cameras, storage, power management circuit board and embedded PC.]] |
[[File:DFNext Internals.jpg|alt=inside of observatory showing components|thumb|422x422px|Internals of the latest iteration of the DFN observatory design (as of August 2017) displaying cameras, storage, power management circuit board and embedded PC.]] |
||
Revision as of 16:05, 29 August 2017
The Desert Fireball Network (DFN) is a network of cameras in Australia. It is designed to track meteoroids entering the atmosphere, and recover meteorites. It currently operates 50 [1] autonomous cameras, spread across Western Australia and South Australia: Nullarbor plain, WA wheatbelt, and South Australian desert. The locations of the stations were chosen to facilitate meteorite searching.
Abbreviation | DFN |
---|---|
Type | Camera network |
Purpose | Record meteorite falls |
Headquarters | Perth |
Region served | Australia |
Affiliations | Curtin University |
Website | http://fireballsinthesky.com.au |
DFN Mission
![]() | This section is empty. You can help by adding to it. (August 2017) |
History
![]() | This section is empty. You can help by adding to it. (August 2017) |
Science of Fireball Tracking
Trajectory
Orbit
What can we learn from meteorites?
Meteorites found
Meteorite name | Fall observation date | Country | State, province, or region | Classification | Instrumentally observed - orbital data | Meteoritical Bulletin(s), other references |
---|---|---|---|---|---|---|
Bunburra Rockhole | July 21, 2007 | Australia | South Australia | Brecciated achondrite | Yes | [2][3][4] |
Mason Gully | April 13, 2010 | Australia | Western Australia | H5 | Yes | [5][6][7] |
Murrili | November 27, 2015 | Australia | South Australia | H5 | Yes | [8][9] |
Dingle Dell | October 31, 2016 | Australia | Western Australia | L/LL5 | Yes | [10][11] |
Camera Hardware

The DFN observatories use consumer still photographic cameras (specifically DSLRs) with 8mm stereographic fish-eye lenses covering nearly the entire sky from each station. The cameras are controlled via an embedded Linux PC using gPhoto2 and images are archived to multiple hard disk drives for storage until the observatories are visited for maintenance (every 8–18 months depending on the storage capacity)[12].
The observatories take one long exposure image every 30 seconds for the entire night. After capture, automated event detection searches the images for fireballs, and events are corroborated on the central server using images from multiple stations.
A GNSS syncronised time code is embedded in the long exposure images by the operation of a liquid crystal (LC) shutter to provide absolute timing data for fireball trajectories after triangulation with temporal precision better than one millisecond[13]. Absolute timing is used for the calculation of meteoroid orbits and the relative timing also embedded by the timecode is required for trajectory analysis (specifically to calculate the mass from the deceleration of the meteoroid).
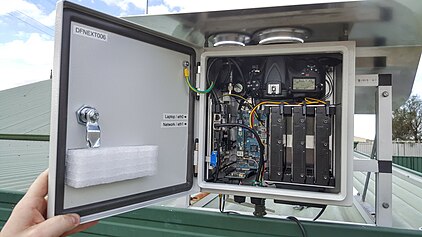
Data processing pipeline
The rate of data acquisition requires an automated digital pipeline for data reduction. Neural network algorithms are used to detect fireball events. A wireless link to each Automated Fireball Observatory allows a cross-check for multi station confirmation and enables images to be remotely downloaded. Software has been created to facilitate the location of fireball trajectories in pixel coordinates. These are converted to celestial coordinates, to a minute of arc precision, by using a powerful astrometric calibration tool created to automatically identify surrounding stars, and use them as a referencing system. The different observation angles are triangulated using a modified least squares minimisation approach, which now includes weightings based on image quality to produce the full observed trajectory . A shutter system within the lens of each observatory encodes a unique non-repeating DeBrujin sequence into each fireball. This provides accurate, absolute timing information for the duration of the trajectory to 0.4 ms. Purpose written software uses entry parameters to determine orbits for each meteoroid. In order to determine if there will be a potential meteorite, the estimation of the changing meteoroid mass is modeled. Once ablation stops, the atmospheric winds strongly affect a meteoroid’s path to the ground. Data from the Global Forecasting System is used in an atmospheric wind model with a 0.008 degree resolution mesh uniquely created around the area of the fireball. A Monte Carlo dark flight simulation is performed to determine a likely search area for main mass and fragments.
Weather modeling
The dark flight trajectory of a meteoroid is significantly affected by the atmospheric winds, especially by the jet stream. As a result, the meteorite fall position can be shifted by up to several kilometers compared to a scenario with no winds.
The weather situation in the area around the end of the luminous flight is numerically modeled using the third generation of Weather Research and Forecasting (WRF) model with dynamic solver ARW (Advanced Research WRF). The weather model is typically initialized using global one-degree-resolution National Centers for Environmental Prediction (NCEP) Final analysis (FNL) Operational Model Global Tropospheric Analysis data. The model produces 3D matrix for given area and time, with horizontal resolution down to 1 km. From this 3D data, weather profiles are extracted; the components include wind speed, wind direction, pressure, temperature and relative humidity at heights ranging up to about 30 km altitude, in most cases fully covering the dark flight.
High volume data handling and archiving
The DFN produces 100s of terabytes of data per year, which mostly consists of high resolution all-sky images. With the proposed network expansion, this volume is going to increase. For the primary purpose of this network, meteorite recovery, only a small fraction of this data (images containing fireballs) is needed, and it is handled by the data processing pipeline (above). However, there are many other potential uses for the data is areas of Astronomy or Space Situational Awareness.
The full data volumes recorded by the cameras are too large to be transferred remotely. Removable hard drives are therefore collected during regular servicing of the DFN observatory sites, replaced with blank hard drives, and then transported to Perth to be archived in a data store at the Pawsey Supercomputing centre. The multi-petabyte data store allows searching of the dataset, using generic and project-custom metadata, and data sharing with other research groups.
Meteorite Searching
Meteorite fall predictions from a camera network typically produce a "fall line"—a straight or curved line on the ground typically a few km long—where it is believed the meteorite has fallen somewhere along the line, but its precise location is unknown. This is a result of the triangulation process, the effect of atmospheric winds during the fall, and knowledge of the apparent visible deceleration of the meteorite, but a lack of knowledge of its density, shape and precise mass.
Meteorite searching theory owes much to search and rescue theory, albeit somewhat simplified as the meteorite is not a moving target. Most of the falls observed by the DFN are in the remote outback, and so searching teams usually consist of 4-6 people, who camp on site for up to two weeks. This means that the searching strategy is focused on efficiency, rather than speed: meteorite recovery on the final day of the expedition is just as scientifically valuable as the first day, which contrasts to, for example, missing person search and rescue, where speed is of the essence. The practical searching techniques used by the DFN team are adapted to the predicted fall size and error ellipse:
- Searching on foot, gridding the area using GPS units to guide walkers, or using survey flags to mark areas, useful for smaller predicted masses, or a smaller error ellipse. This allows detailed coverage of the area with a higher confidence, but less area is searched per unit time.
- For larger areas, searching using quadbikes or ATVs. This is most applicable for larger predicted falls, or good clear area with good long distance visibility.
- Current research is focusing on the use of drones as a technique to improve efficiency.
Outreach
![]() | This section is empty. You can help by adding to it. (August 2017) |
Partners
![]() | This section is empty. You can help by adding to it. (August 2017) |
See also
References
- ^ Wan, Ben (2014-02-03). "Desert Fireball Network - Camera locations on map". Fireballs in the sky. Retrieved 2015-12-31.
- ^ "Meteoritical Bulletin Database".
- ^ "An Anomalous Basaltic Meteorite from the Innermost Main Belt". Science.
- ^ Benedix, G. K.; Bland, P. A.; Friedrich, J. M.; Mittlefehldt, D. W.; Sanborn, M. E.; Yin, Q.-Z.; Greenwood, R. C.; Franchi, I. A.; Bevan, A. W. R. (2017). "Bunburra Rockhole: Exploring the geology of a new differentiated asteroid". Geochimica et Cosmochimica Acta. 208: 145–159.
- ^ "Meteoritical Bulletin Database".
- ^ "Characterisation of Mason Gully (H5): The second recovered fall from the Desert Fireball Network". Meteoritics and Planetary Science. 51: 596–613. doi:10.1111/maps.12605.
- ^ Dyl, Kathryn A.; Benedix, Gretchen K.; Bland, Phil A.; Friedrich, Jon M.; Spurný, Pavel; Towner, Martin C.; O'Keefe, Mary Claire; Howard, Kieren; Greenwood, Richard (2016-03-01). "Characterization of Mason Gully (H5): The second recovered fall from the Desert Fireball Network". Meteoritics & Planetary Science. 51 (3): 596–613. doi:10.1111/maps.12605. ISSN 1945-5100.
- ^ "Meteoritical Bulletin Database".
- ^ Benedix, Gretchen K.; Forman, Lucy V.; Daly, Luke; Greenwood, Richard C.; Franchi, Ian A.; Friedrich, Jon M.; Meier, M. M.; Maden, Colin; Busemann, Henner (2016). "Mineralogy and petrology of the Murrili meteorite".
{{cite journal}}
: Cite journal requires|journal=
(help) - ^ "Meteoritical Bulletin Database".
- ^ Benedix, G. K.; Forman, L. V.; Daly, L.; Godel, B.; Esteban, L.; Meier, M. M. M.; Maden, C.; Busemann, H.; Yin, Q.-Z. (2017). "Mineralogy, Petrology and Chronology of the Dingle Dell Meteorite".
{{cite journal}}
: Cite journal requires|journal=
(help) - ^ Howie, Robert M.; Paxman, Jonathan; Bland, Philip A.; Towner, Martin C.; Cupak, Martin; Sansom, Eleanor K.; Devillepoix, Hadrien A. R. (2017-06-01). "How to build a continental scale fireball camera network". Experimental Astronomy. 43 (3): 237–266. doi:10.1007/s10686-017-9532-7. ISSN 0922-6435.
- ^ Howie, Robert M.; Paxman, Jonathan; Bland, Philip A.; Towner, Martin C.; Sansom, Eleanor K.; Devillepoix, Hadrien A. R. (2017-08-01). "Submillisecond fireball timing using de Bruijn timecodes". Meteoritics & Planetary Science. 52 (8): 1669–1682. doi:10.1111/maps.12878. ISSN 1945-5100.