Peptide vaccine
Peptide-based synthetic vaccines, also called epitope vaccines, are subunit vaccines made from peptides. The peptides mimic the epitopes of the antigen that triggers direct or potent immune responses.[1] Peptide vaccines can not only induce protection against infectious pathogens and non-infectious diseases but also be utilized as therapeutic cancer vaccines, where peptides from tumor-associated antigens are used to induce an effective anti-tumor T-cell response.[2]
History
The traditional vaccines are the whole live or fixed pathogens. The second generation of vaccines is mainly the protein purified from the pathogen. The third generation of vaccines is the DNA or plasmid that can express the proteins of the pathogen. Peptide vaccines are the latest step in the evolution of vaccines.[3]
Advantages and disadvantages
Compared with the traditional vaccines such as the whole fixed pathogens or protein molecules, the peptide vaccines have several advantages and disadvantages.[4]
Advantages:
- The vaccines are fully synthesized by chemical synthesis and can be treated as chemical entity.
- With more advanced solid-phase peptide synthesis (SPPS) using automation and microwave techniques, the production of peptides becomes more efficient.
- The vaccines do not have any biological contamination since they are chemically synthesized.
- The vaccines are water-soluble and can be kept stable under simple conditions.
- The peptides can be specially designed for specificity. A single peptide vaccine can be designed to have multiple epitopes to generate immune responses for several diseases.
- The vaccines only contain a short peptide chain, so they are less like to lead to allergic or auto-immune responses.
Disadvantages:
- Poor immunogenicity.
- Unstable in cells.
- Lack of native conformation.
- Only effective for a limited population.
Epitope design
The whole peptide vaccine is to mimic the epitope of an antigen, so epitope design is the most important stage of vaccine development and requires an accurate understanding of the amino acid sequence of the immunogenic protein interested. The designed epitope is expected to generate strong and long-period immuno-response against the pathogen. The followings are the points to consider when designing the epitope:
- The non-dominant epitope could generate a stronger immune response than the dominant epitope. Ex. The antibodies from people infected by hookworm can recognize the dominant epitope of the antigen called Necator americanus APR-1 protein, but the antibodies can't induce protection against hookworm. However, other non-dominant epitopes on APR-1 protein show the ability to induce the production of neutralizing antibodies against hookworm. Therefore, the non-dominant epitopes are the better candidate for peptide vaccines against hookworm infection.[5]
- Take hypersensitivity into consideration. Ex. Some IgE-inducing epitopes cause hypersensitivity reactions after vaccination in humans due to the overlap with IgG epitopes in the Na-ASP-2 protein which is an antigen from hookworm.[6]
- Some short peptide epitopes need elongating to maintain the native conformation. The elongated sequences can include proper secondary structure. Also, some short peptides can be stabled or cyclized together to maintain the proper conformation. Ex. B-cell epitopes could only have 5 amino acids. To induce an immune response, a sequence from yeast GCN4 protein is used to improve the conformation of the peptide vaccines by forming alpha-helix..[7]
- Use adjuvants associated with the epitope to induce the immune response.[8]
Applications
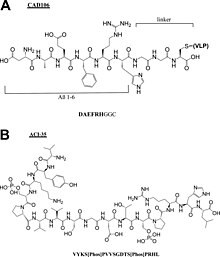
Cancer
- Gp100 peptide vaccine is studied to treat melanoma. To generate a greater in vitro CTL response, the peptide, gp100:209-217(210M), is modified and binds to HLA-A2*0201. After vaccination, more circulating T cells can recognize and kill melanoma cancer cells in vitro.[10]
- Rindopepimut is the epidermal growth factor receptor (EGFR)-derived peptide vaccine to treat glioblastoma multiforme (GBM). The 14-mer peptide is coupled with keyhole limpet hemocyanin (KLH), which can reduce the risk of cancer.[11]
- E75, GP2, and AE37 are three different HER2/neu-derived single-peptide vaccines to treat breast cancer. HER2/neu usually has low expression in healthy tissues. E75 consisting of 9 amino acids is the immunodominant epitope of the HER2 protein. GP2 consisting of 9 amino acids is the subdominant epitope. Both E75 and GP2 stimulate the CD8+ lymphocytes but GP2 has a lower affinity than E75. AE37 stimulates CD4+ lymphocytes.[12]
Other common diseases
- EpiVacCorona, a peptide-based vaccine against COVID-19.
- IC41 is a peptide vaccine candidate against the Hepatitis C virus. It consists of five synthetic peptides along with the synthetic adjuvant called poly-l-arginine.[13]
- Multimeric-001 is the most efficient peptide vaccine candidate against influenza. It contains B- and T-cell epitopes from Hemagglutinin. Matrix I and nucleoprotein are combined into a single recombinantly-expressed polypeptide.[14][15]
- Alzheimer peptide vaccines: CAD106,[16] UB311,[17] Lu, AF20513,[18] ABvac40,[19] ACI-35,[20] AADvac-1.[21]
References
- ^ Skwarczynski M, Toth I (February 2016). "Peptide-based synthetic vaccines". Chemical Science. 7 (2): 842–854. doi:10.1039/C5SC03892H. PMC 5529997. PMID 28791117.
- ^ Melief CJ, van der Burg SH (May 2008). "Immunotherapy of established (pre)malignant disease by synthetic long peptide vaccines". Nature Reviews. Cancer. 8 (5): 351–360. doi:10.1038/nrc2373. PMID 18418403. S2CID 205468352.
- ^ Schneble E, Clifton GT, Hale DF, Peoples GE (2016). "Peptide-Based Cancer Vaccine Strategies and Clinical Results". In Thomas S (ed.). Vaccine Design. Methods in Molecular Biology, Vaccine Design: Methods and Protocols: Volume 1: Vaccines for Human Diseases. Vol. 1403. New York, NY: Springer. pp. 797–817. doi:10.1007/978-1-4939-3387-7_46. ISBN 978-1-4939-3387-7. PMID 27076168.
{{cite book}}
:|journal=
ignored (help) - ^ Skwarczynski M, Toth I (February 2016). "Peptide-based synthetic vaccines". Chemical Science. 7 (2): 842–854. doi:10.1039/C5SC03892H. PMC 5529997. PMID 28791117.
- ^ Pearson MS, Pickering DA, Tribolet L, Cooper L, Mulvenna J, Oliveira LM, et al. (May 2010). "Neutralizing antibodies to the hookworm hemoglobinase Na-APR-1: implications for a multivalent vaccine against hookworm infection and schistosomiasis". The Journal of Infectious Diseases. 201 (10): 1561–1569. doi:10.1086/651953. PMID 20367477.
- ^ Diemert DJ, Pinto AG, Freire J, Jariwala A, Santiago H, Hamilton RG, et al. (July 2012). "Generalized urticaria induced by the Na-ASP-2 hookworm vaccine: implications for the development of vaccines against helminths". The Journal of Allergy and Clinical Immunology. 130 (1): 169–76.e6. doi:10.1016/j.jaci.2012.04.027. PMID 22633322.
- ^ Cooper JA, Hayman W, Reed C, Kagawa H, Good MF, Saul A (April 1997). "Mapping of conformational B cell epitopes within alpha-helical coiled coil proteins". Molecular Immunology. 34 (6): 433–440. doi:10.1016/S0161-5890(97)00056-4. PMID 9307059.
- ^ Azmi F, Ahmad Fuaad AA, Skwarczynski M, Toth I (March 2014). "Recent progress in adjuvant discovery for peptide-based subunit vaccines". Human Vaccines & Immunotherapeutics. 10 (3): 778–796. doi:10.4161/hv.27332. PMC 4130256. PMID 24300669.
- ^ Malonis RJ, Lai JR, Vergnolle O (March 2020). "Peptide-Based Vaccines: Current Progress and Future Challenges". Chemical Reviews. 120 (6): 3210–3229. doi:10.1021/acs.chemrev.9b00472. PMC 7094793. PMID 31804810.
- ^ Marincola FM, Rivoltini L, Salgaller ML, Player M, Rosenberg SA (July 1996). "Differential anti-MART-1/MelanA CTL activity in peripheral blood of HLA-A2 melanoma patients in comparison to healthy donors: evidence of in vivo priming by tumor cells". Journal of Immunotherapy with Emphasis on Tumor Immunology. 19 (4): 266–277. doi:10.1097/00002371-199607000-00003. PMID 8877721.
- ^ Neal DE, Sharples L, Smith K, Fennelly J, Hall RR, Harris AL (April 1990). "The epidermal growth factor receptor and the prognosis of bladder cancer". Cancer. 65 (7): 1619–1625. doi:10.1002/1097-0142(19900401)65:7<1619::aid-cncr2820650728>3.0.co;2-q. PMID 2311071.
- ^ Palatnik-de-Sousa CB, Soares IS, Rosa DS (2018-04-18). "Editorial: Epitope Discovery and Synthetic Vaccine Design". Frontiers in Immunology. 9: 826. doi:10.3389/fimmu.2018.00826. PMC 5915546. PMID 29720983.
- ^ Firbas C, Jilma B, Tauber E, Buerger V, Jelovcan S, Lingnau K, et al. (May 2006). "Immunogenicity and safety of a novel therapeutic hepatitis C virus (HCV) peptide vaccine: a randomized, placebo controlled trial for dose optimization in 128 healthy subjects". Vaccine. 24 (20): 4343–4353. doi:10.1016/j.vaccine.2006.03.009. PMID 16581161.
- ^ Atsmon J, Caraco Y, Ziv-Sefer S, Shaikevich D, Abramov E, Volokhov I, et al. (October 2014). "Priming by a novel universal influenza vaccine (Multimeric-001)-a gateway for improving immune response in the elderly population". Vaccine. 32 (44): 5816–5823. doi:10.1016/j.vaccine.2014.08.031. PMID 25173483.
- ^ van Doorn E, Liu H, Ben-Yedidia T, Hassin S, Visontai I, Norley S, et al. (March 2017). "Evaluating the immunogenicity and safety of a BiondVax-developed universal influenza vaccine (Multimeric-001) either as a standalone vaccine or as a primer to H5N1 influenza vaccine: Phase IIb study protocol". Medicine. 96 (11): e6339. doi:10.1097/md.0000000000006339. PMC 5369918. PMID 28296763.
- ^ Wiessner C, Wiederhold KH, Tissot AC, Frey P, Danner S, Jacobson LH, et al. (June 2011). "The second-generation active Aβ immunotherapy CAD106 reduces amyloid accumulation in APP transgenic mice while minimizing potential side effects". The Journal of Neuroscience. 31 (25): 9323–9331. doi:10.1523/jneurosci.0293-11.2011. PMC 6623465. PMID 21697382.
- ^ Wang CY, Finstad CL, Walfield AM, Sia C, Sokoll KK, Chang TY, et al. (April 2007). "Site-specific UBITh amyloid-beta vaccine for immunotherapy of Alzheimer's disease". Vaccine. 25 (16): 3041–3052. doi:10.1016/j.vaccine.2007.01.031. PMID 17287052.
- ^ Davtyan H, Ghochikyan A, Petrushina I, Hovakimyan A, Davtyan A, Poghosyan A, et al. (March 2013). "Immunogenicity, efficacy, safety, and mechanism of action of epitope vaccine (Lu AF20513) for Alzheimer's disease: prelude to a clinical trial". The Journal of Neuroscience. 33 (11): 4923–4934. doi:10.1523/jneurosci.4672-12.2013. PMC 3634356. PMID 23486963.
- ^ Lacosta AM, Pascual-Lucas M, Pesini P, Casabona D, Pérez-Grijalba V, Marcos-Campos I, et al. (January 2018). "Safety, tolerability and immunogenicity of an active anti-Aβ40 vaccine (ABvac40) in patients with Alzheimer's disease: a randomised, double-blind, placebo-controlled, phase I trial". Alzheimer's Research & Therapy. 10 (1): 12. doi:10.1002/alz.045720. PMC 5789644. PMID 29378651.
- ^ Hickman DT, López-Deber MP, Ndao DM, Silva AB, Nand D, Pihlgren M, et al. (April 2011). "Sequence-independent control of peptide conformation in liposomal vaccines for targeting protein misfolding diseases". The Journal of Biological Chemistry. 286 (16): 13966–13976. doi:10.1074/jbc.m110.186338. PMC 3077597. PMID 21343310.
- ^ Kontsekova E, Zilka N, Kovacech B, Novak P, Novak M (2014). "First-in-man tau vaccine targeting structural determinants essential for pathological tau-tau interaction reduces tau oligomerisation and neurofibrillary degeneration in an Alzheimer's disease model". Alzheimer's Research & Therapy. 6 (4): 44. doi:10.1186/alzrt278. PMC 4255368. PMID 25478017.
{{cite journal}}
: CS1 maint: unflagged free DOI (link)