Electron paramagnetic resonance
Electron Paramagnetic Resonance (EPR) or Electron Spin Resonance (ESR) is a spectroscopic technique which detects species that have unpaired electrons, generally meaning that it must be a free radical, if it is an organic molecule, or that it has transition metal ions if it is an inorganic complex. Because most stable molecules have a closed-shell configuration without a suitable unpaired spin, the technique is less widely used than nuclear magnetic resonance (NMR).
The basic physical concepts of the technique are analogous to those of NMR, but instead of the spins of the atom's nuclei, electron spins are excited. Because of the difference in mass between nuclei and electrons, weaker magnetic fields and higher frequencies are used, compared to NMR. For electrons in a magnetic field of 0.3 tesla, spin resonance occurs at around 10 GHz.
EPR is used in solid-state physics, for the identification and quantification of radicals (i.e., molecules with unpaired electrons), in chemistry, to identify reaction pathways, as well as in biology and medicine for tagging biological spin probes.
Since radicals are very reactive, they do not normally occur in high concentrations in biological environments. With the help of specially designed nonreactive radical molecules that attach to specific sites in a biological cell, it is possible to obtain information on the environment of these so-called spin-label or spin-probe molecules.
To detect some subtle details of some systems, high-field-high-frequency electron spin resonance spectroscopy is required. While ESR is affordable for a medium-sized academic laboratory, there are few scientific centers in the world offering high-field-high-frequency electron spin resonance spectroscopy, among them ILL in Grenoble, France and one in Tallahassee, USA.
EPR theory
Units and constants
A magnetic Field is described by some constants and units:
- Magnetic induction in teslas (T)
- Magnetic flux density in amperes per metre (A/m)
- and relationship:
- The CGS unit for magnetic induction is the gauss (G) which is equivalent to 10-4 T
Furthermore, in describing EPR, following units are very important:
- Planck's constant h = 6.63 x 10-34 J s
- Boltzmann constant k = 1.38 x 10-23 J K-1
- Bohr magneton B = 9.27 x 10-24 J T-1
Basics
Quantum mechanically, an electron has a magnetic moment of B (the Bohr magneton). When placed in an external magnetic field of strength B0, this magnetic moment can align itself parallel or antiparallel to the external field. The former is a lower energy state than the latter (this is the Zeeman effect), and the energy separation between the two is = , where ge is the gyromagnetic ratio of the electron, the ratio of its magnetic dipole moment to its angular momentum. To move between the two energy levels, the electron can absorb electromagnetic radiation of the correct energy:
= = geBB0
and this is the fundamental equation of EPR spectroscopy. The paramagnetic centre is placed in a magnetic field and the electron caused to resonate between the two states; the energy absorbed as it does so is monitored, and converted into the EPR spectrum.
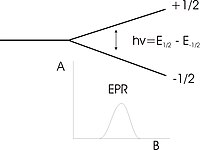
A free electron (on its own) has a g value of 2.002319304386 (which is ge, the electronic g factor). This means that for radiation at the commonly used frequency of 9.5 GHz (known as X-band microwave radiation, and thus giving rise to X-band spectra), resonance occurs at a magnetic field of about 0.34 tesla (3400 gauss).
EPR signals can be generated by resonant energy absorption measurements made at different electromagnetic radiation frequencies in a constant external magnetic field (i.e. you scan with a range of different frequency radiation whilst holding the field constant, like in an NMR experiment). Conversely, measurements can be provided by changing the magnetic field B and using a constant frequency radiation; due to technical considerations, this second way is more common. This means that an EPR spectrum is normally plotted with the magnetic field along the x-axis, with peaks at the field that cause resonance (whereas an NMR spectrum has peaks at the frequencies that cause resonance).
In real life, electrons are normally associated with atoms. There are three important consequences of this. Firstly, the electron may gain or lose angular momentum (through spin-orbit coupling) which will affect the value of the g-factor — this often varies from 2.0023, especially in transition metal compounds. Secondly, this change in angular momentum is not the same for all orientations of the atom or molecule in an external magnetic field. In other words, the g-factor changes according to the orientation of the paramagnetic atom in the magnetic field—it is anisotropic. This anisotropy depends upon the electronic structure of the atom in question, and so can yield information about the atomic (or molecular) orbitals containing the unpaired electron. Thirdly, if the atom(s) which the electron is associated with has (or have) a non-zero nuclear spin, the magnetic field associated with this atom will affect the electron too. This leads to the phenomenon of hyperfine coupling, which is analogous to coupling in NMR in splitting the resonance signal into doublets, triplets and so forth.
In practice a single, isolated, paramagnetic centre never occurs, but only a population of a large number of centres. If this population of centres is in thermodynamic equilibrium, its statistical distribution is described by the Boltzmann distribution.
Boltzmann distribution
= exp=exp
where is the number of paramagnetic centres occupying the level,
k is the Boltzmann constant, and T is the temperature in kelvins.
For X-band radiation ( = 10 GHz) at room temperature, = 0.998. Because the lower level has more electrons than the higher one, transitions from the lower to the higher level are more probable, which is why there is a net absorption of radiation.
EPR spectral parameters
The g factor
Knowledge of the g factor gives us information about the paramagnetic center's electronic structure. When an unpaired electron is in an atom, it feels not only the external magnetic field B0 applied by the spectrometer, but also the effects of any local magnetic fields. Therefore, the effective field Beff felt by the electron is
Beff = B0(1 - σ)
where σ allows for the effects of the local fields (it can be positive or negative), and therefore the resonance condition is
= = geBBeff = geBB0(1 - )
The quantity ge(1 - ) is called the g factor, given the symbol g, so
E = h = gBB0
Given this last equation, you can measure g from the ESR experiment by measuring the field B0 and the frequency at which resonance occurs. If g differs from ge (2.0023), this implies that the ratio of the electron's magnetic moment to its angular momentum has changed from the free electron value. Since the electron's magnetic moment is constant (it's the Bohr magneton), then the electron must have gained or lost angular momentum. It does this though spin-orbit coupling, and because the mechanisms of spin-orbit coupling are well understood, the magnitude of the change can be used to give information about the nature of the atomic or molecular orbital containing the electron.
For free radicals and solid or liquid state ions, orbital electronic momentum is hard-linked with environment and cannot occur at any orientation to a magnetic field. This is due to orbital magnetic moment freezing phenomena in crystal network. Due to this phenomenon, the g factor of an EPR signal for many paramagnetic atoms in the solid state has a different value from the free-electron value (g′ = 2.002319304386). In the case of a free ion, the g parameter has isotropic properties. In crystal g value is depended on external magnetic field vector.
see also Landé_g-factor
Resonance linewidth definition
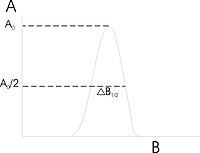
Resonance linewidths are defined in magnetic induction units B and are measured along the x axis, from line center to y value crossing chosen point of energy spectrum. These defined widths are called halfwidths and possess some advantages: for asymmetric lines values of left and right halfwidth can be given. Halfwidth is distance measured from center of line to the point in which absorption value has half of maximal absorption value in the center of resonance line. First inclination width is a distance from center of the line to the point of maximal absorption curve inclination. In a practical approach, full definition of linewidth is used. In the case of symmetric lines, halfwidth , and full inclination width
External links
- [1] Professor Phil Rieger: Electron Spin Spectroscopy graduate tutorial
- EPR Laboratory - list of EPR laboratories all over the world class