Astrophysical jet
An astrophysical jet (hereafter 'jet') is a phenomenon often seen in astronomy, where streams of matter are emitted along the axis of rotation of a compact object. While it is still the subject of ongoing research to understand how jets are formed and powered, the two most often proposed origins are dynamic interactions within the accretion disk or a process from the central object (such as a black hole). When matter is emitted at speeds approaching the speed of light, these jets are called relativistic jets, because the effects of special relativity become important. The largest jets are those from black holes in active galaxies such as quasars and radio galaxies. Other systems which often contain jets include cataclysmic variable stars, X-ray binaries and T Tauri stars. Herbig–Haro objects are caused by the interaction of jets with the interstellar medium. Bipolar outflows or jets may also be associated with protostars (young, forming stars),[1] or with evolved post-AGB stars (often in the form of bipolar nebulae).
Accretion disks around many stellar objects are able to produce jets, although those from super massive black holes are generally the fastest and most active. It has been theorized the speed of the jet is about the same as the escape velocity of the central object, making the speed of a jet from an accreting black hole near the speed of light, while protostellar jets are much slower. While it is not known exactly how accretion disks manage to produce jets, they are thought to generate tangled magnetic fields that cause the jets to collimate. The hydrodynamics of a de Laval nozzle may also give a hint to the mechanisms involved.
One of the best ways of exploring how jets are produced is to determine the composition of the jets at a radius where they can be directly observed. For example, it has been suggested if a jet originates from the accretion disk, its plasma is likely to have ion-electron composition, whereas if it originates from the black hole or neutron star it will likely be positron-electron in nature. Also, the plasma emits various forms of radiation such as X-rays and radio waves, which aid diagnosis.
Relativistic jet
Relativistic jets are extremely powerful jets[2] of plasma, with speeds close to the speed of light, that are emitted near the central massive objects of some active galaxies, notably radio galaxies and quasars. Their lengths can reach several thousand[3] or even hundreds of thousands of light years.[4] Because the jet speed is close to the speed of light, the effects of the Special Theory of Relativity are important; in particular, relativistic beaming will change the apparent brightness. The mechanics behind both the creation of the jets[5][6] and the composition of the jets[7] are still a matter of much debate in the scientific community. Jet composition might vary; some studies favour a model in which the jets are composed of an electrically neutral mixture of nuclei, electrons, and positrons, while others are consistent with a jet primarily of positron-electron plasma.[8][9]
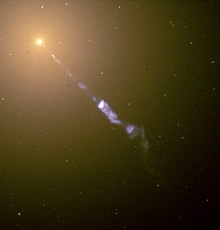
Similar jets, though on a much smaller scale, can develop from neutron stars and stellar black holes. These systems are often called microquasars. An example is SS433, whose well-observed jet has a velocity of 0.23c, although other microquasars appear to have much higher (but less well measured) jet velocities. Even weaker and less relativistic jets may be associated with many binary systems; the acceleration mechanism for these jets may be similar to the magnetic reconnection processes observed in the Earth's magnetosphere and the solar wind.
The general hypothesis among astrophysicists is that the formation of relativistic jets is the key to explaining the production of gamma-ray bursts. These jets have Lorentz factors of ~100 (that is, speeds of roughly 0.99995c), making them some of the swiftest celestial objects currently known. Stellar black holes and neutron stars[10] are also known to have relativistic jets.[11]

(Credits: NASA / ISDC / CXC / Pavan, L.; Bordas, P.; Puehlhofer, G.; Filipovic, M. D.; De Horta, A.; O'Brien, A.; Balbo, M.; Walter, R.; Bozzo, E.; Ferrigno, C.; Crawford, E.; Stella, L. (2013). "The long helical jet of the Lighthouse nebula, IGR J11014-6103". Astronomy & Astrophysics. 562 (562): A122. arXiv:1309.6792. doi:10.1051/0004-6361/201322588. )
IGR J11014-6103 has the largest jet observed in the Milky Way Galaxy. Fortunately this relativistic helical jet is not obscured by explosion debris or accretion material. Numbers in this paragraph are tentative. In the composite image shown (click on image to enlarge) the spinning neutron star (labeled PULSAR in the image) is the point-like object with a WIND NEBULA tail trailing behind it for about 3 light years. The JET, aligned with the pulsar’s rotation axis, is perpendicular to the pulsar's trajectory and extends out over 37 light years (about 10X the distance from our solar system to the nearest star). The estimated velocity of this long term jet is 0.8c. In this image the high speed runaway pulsar was created and ejected about 10 – 30 thousand years prior from a supernova explosion which created the SUPERNOVA REMNANT; the pulsar is shown about 60 light years from the original supernova. This pulsar is not listed in the latest revision of Accreting Millisecond X-Ray Pulsars (AMXPs)[12] and no accretion material has been detected. The jet is observed in X-rays and has no detected radio signature.[13] The pulsar's speed is reported between 0.003 – 0.008c; faster than most runaway neutron stars.[14][15][16][17] The spin rate of the neutron star in IGR J11014-6103 is only 15.9 Hz, suggesting the jet might not be rotation powered.[18][19] Mass estimates for this star are not yet available.
Theories for the IGR J11014-6103 Jet: Recent observations indicate some neutron stars make jets about as efficiently as black holes. Theories for neutron star jets with velocities near c fall into two general categories: (a) Jets originating outside the star. (b) Jets originating within the star. The following theories are a partial list and should not be considered authoritative as there is not a consensus on the subject and sources do not go into sufficient detail:
Jets originating outside the star: Most theories predict the jets are driven by the enormous rotation energy of the compact objects and accretion disks that surround it. Through magneto-hydrodynamic mechanisms, the rotation energy is evacuated through the poles by means of jets, as the rest can fall towards the gravitational attraction center.[20] It has been theorized in the case of a black hole, this energy is stored in a giant vortex of space-time that is constantly dragged around the black hole. However neutron stars have powerful jets similar to black holes, but there is no vortex effect, so something else must be powering the jet.[21] Some theories suggest the neutron star magnetic field powers the jets and the rotation of the intense magnetic field generates intense electric fields that literally tear electrons from the star surface to form the jets.[22]
Jets originating within the star: Observations of some jets with velocities near c suggest the jets are composed primarily of electron–positron plasma.[23] Electron-positron clouds surrounding some old neutron stars and stellar black holes also suggest this composition.[24][25] A possible explanation for neutron star jets with velocity near c is collapsing core nuclei produce a 450-MeV electron-positron beam. Trace nuclei swept up in such a beam could achieve an astounding energy equal to almost (nucleus mass/electron mass) X 450-MeV.
Rotating black hole as energy source
Because of the enormous amount of energy needed to launch a relativistic jet, some jets are thought to be powered by spinning black holes. There are two well known theories for how the energy is transferred from the black hole to the jet.
- Blandford–Znajek process.[26] This is the most popular theory for the extraction of energy from the central black hole. The magnetic fields around the accretion disk are dragged by the spin of the black hole. The relativistic material is possibly launched by the tightening of the field lines.
- Penrose mechanism.[27] This extracts energy from a rotating black hole by frame dragging. This theory was later proven to be able to extract relativistic particle energy and momentum,[28] and subsequently shown to be a possible mechanism for the formation of jets.[29]
A Hubble Space Telescope survey indicated that relativistic jets may be more likely to form from supermassive black holes resulting from the merger of two galaxies and their galaxy centre's black holes. Not all galaxy mergers create relativistic jets.[30][31] NASA/ESA Video
Other images
-
Centaurus A in x-rays showing the relativistic jet
-
The M87 jet seen by the Very Large Array in radio frequency (the viewing field is larger and rotated with respect to the above image.)
-
Galaxy NGC 3862, an extragalactic jet of material moving at nearly the speed of light can be seen at the three o'clock position.[32]
See also
References
- ^ "Star sheds via reverse whirlpool". Astronomy.com. 27 December 2007. Retrieved 26 May 2015.
- ^ Wehrle, A.E.; Zacharias, N.; Johnston, K.; et al. (11 Feb 2009). "What is the structure of Relativistic Jets in AGN on Scales of Light Days?" (PDF). Astro2010: the Astronomy and Astrophysics Decadal Survey. 2010: 310. Bibcode:2009astro2010S.310W.
{{cite journal}}
: CS1 maint: multiple names: authors list (link) - ^ Biretta, J. (6 Jan 1999). "Hubble Detects Faster-Than-Light Motion in Galaxy M87".
- ^ "Evidence for Ultra-Energetic Particles in Jet from Black Hole". Yale University – Office of Public Affairs. 20 June 2006. Archived from the original on 2008.
{{cite web}}
: Check date values in:|archive-date=
(help) - ^ Meier, David L (2003). "The theory and simulation of relativistic jet formation: Towards a unified model for micro- and macroquasars". New Astronomy Reviews. 47 (6–7): 667. arXiv:astro-ph/0312048. Bibcode:2003NewAR..47..667M. doi:10.1016/S1387-6473(03)00120-9.
- ^ Semenov, V.; Dyadechkin, Sergey; Punsly, Brian (2004). "Simulations of Jets Driven by Black Hole Rotation". Science. 305 (5686): 978–980. arXiv:astro-ph/0408371. Bibcode:2004Sci...305..978S. doi:10.1126/science.1100638. PMID 15310894.
- ^ Georganopoulos, Markos; Kazanas, Demosthenes; Perlman, Eric; Stecker, Floyd W. (2005). "Bulk Comptonization of the Cosmic Microwave Background by Extragalactic Jets as a Probe of Their Matter Content". The Astrophysical Journal. 625 (2): 656. arXiv:astro-ph/0502201. Bibcode:2005ApJ...625..656G. doi:10.1086/429558.
- ^ "NASA - Vast Cloud of Antimatter Traced to Binary Stars".
- ^ Wardle, J.F.C (1998). "Electron–positron jets associated with the quasar 3C279". Nature. 395 (1 October 1998): 457–461. Bibcode:1998Natur.395..457W. doi:10.1038/26675.
- ^ "Neutron Stars and Pulsars".
- ^ "Neutron Star Jets Near Speed of Light --". The Daily Galaxy --Great Discoveries Channel: Sci, Space, Tech.
- ^ Patruno, A.; Watts, A. L. (2012). "Accreting Millisecond X-Ray Pulsars". arXiv:1206.2727 [astro-ph.HE].
- ^ "Runaway pulsar has astronomers scratching their heads".
- ^ "Fastest Pulsar Moving With Tremendous Speed Of 6 Million Miles Per Hour - Found - MessageToEagle.com".
- ^ "Chandra :: Photo Album :: IGR J11014-6103 :: June 28, 2012".
- ^ Pavan, L.; Pühlhofer, G.; Bordas, P.; Audard, M.; Balbo, M.; Bozzo, E.; Eckert, D.; Ferrigno, C.; Filipović, M. D.; Verdugo, M.; Walter, R. (2015). "A closer view of the IGR J11014-6103 outflows". arXiv:1511.01944 [astro-ph.HE].
- ^ "Neutron star jet: An exploded star creates a truly bizzare scene". Slate Magazine.
- ^ Pavan, L.; Bordas, P.; Pühlhofer, G.; Filipović, M. D.; De Horta, A.; o' Brien, A.; Balbo, M.; Walter, R.; Bozzo, E.; Ferrigno, C.; Crawford, E.; Stella, L. (2014). "The long helical jet of the Lighthouse nebula, IGR J11014-6103" (PDF). Astronomy & Astrophysics. 562: A122. doi:10.1051/0004-6361/201322588. Long helical jet of Lighthouse nebula page 7
- ^ Halpern, J. P.; Tomsick, J. A.; Gotthelf, E. V.; Camilo, F.; Ng, C. -Y.; Bodaghee, A.; Rodriguez, J.; Chaty, S.; Rahoui, F. (2014). "Discovery of X-ray Pulsations from the INTEGRAL Source IGR J11014-6103". The Astrophysical Journal. 795 (2): L27. arXiv:1410.2332. doi:10.1088/2041-8205/795/2/L27.
- ^ Mirabel, I. F. (2008). "Microquasars: Summary and Outlook". The Jet Paradigm. Lecture Notes in Physics. Vol. 794. pp. 1–15. arXiv:0805.2378. doi:10.1007/978-3-540-76937-8_1. ISBN 978-3-540-76936-1.
{{cite book}}
:|journal=
ignored (help) - ^ "Neutron Star Fires Powerful Jets".
- ^ "Crab Nebula's Neutron Star Pulsed Most Powerful Beam Ever Detected --". The Daily Galaxy --Great Discoveries Channel: Sci, Space, Tech.
- ^ Electron-positron Jets Associated with Quasar 3C 279
- ^ "NASA - Vast Cloud of Antimatter Traced to Binary Stars".
- ^ Science With Integral. 1 September 2008 – via YouTube.
- ^ Blandford, R. D.; Znajek, R. L. (1977). "Electromagnetic extraction of energy from Kerr black holes". Monthly Notices of the Royal Astronomical Society. 179 (3): 433. Bibcode:1977MNRAS.179..433B. doi:10.1093/mnras/179.3.433.
{{cite journal}}
: CS1 maint: unflagged free DOI (link) - ^ Penrose, Roger (1969). "Gravitational Collapse: The Role of General Relativity". Rivista del Nuovo Cimento. 1: 252–276. Bibcode:1969NCimR...1..252P. Reprinted in: Penrose, R. (2002), ""Golden Oldie": Gravitational Collapse: The Role of General Relativity", General Relativity and Gravitation, 34 (7): 1141, Bibcode:2002GReGr..34.1141P, doi:10.1023/A:1016578408204
- ^ R.K. Williams (1995). "Extracting x rays, Ύ rays, and relativistic e−e+ pairs from supermassive Kerr black holes using the Penrose mechanism". Physical Review. 51 (10): 5387–5427. Bibcode:1995PhRvD..51.5387W. doi:10.1103/PhysRevD.51.5387. PMID 10018300.
- ^ Williams, Reva Kay (2004). "Collimated Escaping Vortical Polare−e+Jets Intrinsically Produced by Rotating Black Holes and Penrose Processes". The Astrophysical Journal. 611 (2): 952. arXiv:astro-ph/0404135. Bibcode:2004ApJ...611..952W. doi:10.1086/422304.
- ^ "Galaxy Crashes May Give Birth to Powerful Space Jets". Retrieved 2015-05-29.
- ^ information@eso.org. "Merging galaxies break radio silence – Large Hubble survey confirms link between mergers and supermassive black holes with relativistic jets". www.spacetelescope.org. Retrieved 2015-05-29.
- ^ "Hubble Video Shows Shock Collision Inside Black Hole Jet". 27 May 2015.
External links
- NASA – Ask an Astrophysicist: Black Hole Bipolar Jets
- SPACE.com – Twisted Physics: How Black Holes Spout Off
- Blandford, Roger; Agol, Eric; Broderick, Avery; Heyl, Jeremy; Koopmans, Leon; Lee, Hee-Won (2001). "Compact Objects and Accretion Disks". arXiv:astro-ph/0107228v1.