Dioxygen in biological reactions: Difference between revisions
moved information from the main page to this subpage due to expected trimming down of the section in the main article |
(No difference)
|
Revision as of 09:19, 17 January 2008
Oxygen plays an important role in the energy metabolism of living organisms. Free oxygen is produced in the biosphere through photolysis (light-driven oxidation and splitting) of water during photosynthesis in cyanobacteria, green algae, and plants. During oxidative phosphorylation in cellular respiration, oxygen is reduced to water, thus closing the biological water-oxygen redox cycle.
Photosynthesis
In nature, free oxygen is produced by the light-driven splitting of water during oxygenic photosynthesis in cyanobacteria, green algae and plants.[1] Algae and cyanobacteria in marine environments provide about 70% of the free oxygen produced on earth.[2] The remainder is produced by terrestrial plants, although almost all oxygen produced in tropical forests is consumed by organisms in those forests.[3]
A simplified overall formula for photosynthesis is:
- 6CO2 + 6H2O + sunlight → C6H12O6 + 6O2
Or simply: carbon dioxide + water + sunlight → glucose + oxygen
Photolytic oxygen evolution during photosynthesis occurs via the light-dependent oxidation of water to molecular oxygen and can be written as the following simplified chemical reaction:
- 2H2O → 4e− + 4H+ + O2
The reaction occurs in the thylakoid membranes of cyanobacteria and algal and plant chloroplasts and requires the energy of four photons. The electrons from the oxidized water molecules replace electrons in the P680 component of photosystem II, which have been removed into an electron transport chain via light-dependent excitation and resonance energy transfer onto plastoquinone.[4] Photosytem II therefore has also been referred to as water-plastoquinone oxido-reductase.[5] The protons from the oxidized water molecules are released into the thylakoid lumen, thus contributing to the generation of a proton gradient across the thylakoid membrane. This proton gradient is the driving force for ATP synthesis via photophosphorylation and coupling the absorption of light energy and photolysis of water to the creation of chemical energy during photosynthesis.[4] The O2 remaining after oxidation of the water molecule is released into the atmosphere.
Water oxidation is catalyzed by a manganese-containing enzyme complex known as the oxygen evolving complex (OEC) or water-splitting complex found associated with the lumenal side of thylakoid membranes. Manganese is an important cofactor, and calcium and chloride are also required for the reaction to occur.[4]
Geologic timeline of biosynthesis

Oxygen was almost nonexistent in Earth's atmosphere before the evolution of water oxidation in photosynthetic bacteria. Free oxygen first appeared in significant quantities during the Paleoproterozoic era (between 2.5 billion years ago and 1.6 billion years ago) as a product of the photosynthetic action of early anaerobes (archaea and bacteria). These organisms, fossil evidence for which occurs in the form of stromatolites and oncolites, developed the mechanism of oxygen evolution in the Archean era, between 3.5 and 2.7 billion years ago. At first, the produced oxygen dissolved in the oceans, where it was reduced by dissolved iron compounds, precipitating iron oxide (Fe2O3) and creating banded iron formations that are now a valuable resource of iron ore, hematite. Oxygen started to gas out of the oxygen-saturated waters from about 2.7 billion years ago, as is evident from the rusting of iron-rich terrestrial rocks starting around that time. The amount of oxygen in the atmosphere increased gradually at first and then more rapidly around 2.2 to 1.7 billion years ago to about 10% of its present level, as available reducing agents in the oceans and crustal rocks became oxidized.[6]

- Radiation of animal phyla (Cambrian explosion)
- First land plants
- Ordovician-Silurian extinction events
- Huge forests form on land, first land animals and seed plants
- Coal formation, first conifers, insect and amphibian giantism
- Low ocean levels, supercontinent Pangaea forms
- Permian-Triassic extinction event
- First primitive flowering plants and dinosaurs
- Triassic-Jurassic extinction event
- Age of dinosaurs
- Radiation of flowering plants
- Cretaceous-Tertiary extinction event
- Radiation of mammals
The development of an oxygen-rich atmosphere was one of the most important events in the history of life on Earth. The presence of large amounts of dissolved and free oxygen in the oceans and atmosphere may have driven most of the anaerobic organisms then living to extinction during the oxygen catastrophe about 2.4 billion years ago. However, the high electronegativity of O2 creates a large potential energy drop for cellular respiration, thus enabling organisms using aerobic respiration to produce much more ATP than anaerobic organisms. This makes them so efficient that they have come to dominate Earth's biosphere.[7] Photosynthesis and cellular respiration of oxygen allowed for the evolution of eukaryotic cells and ultimately complex multicellular organisms such as plants and animals.
The atmospheric abundance of free oxygen in later geological epochs and its gradual increase up to the present has been largely due to synthesis by photosynthetic organisms. Over the past 500 million years, oxygen levels fluctuated between 15% and 30% per volume.[8] Towards the end of the Carboniferous era (coal age) about 300 million years ago, atmospheric oxygen levels reached a maximum of 35% by volume,[8] allowing insects and amphibians with limiting respiratory systems to grow much larger than today's species. Today, oxygen is the second-most-common component of the earth's atmosphere (about 21% by volume), the most-common being nitrogen. Human activities, including the burning of 7 billion tonnes of fossil fuels each year have had very little effect on the amount of free oxygen in the atmosphere.[9] It was estimated that, at the current rate of photosynthesis, it would take about 2,000 years to regenerate the entire oxygen in the present atmosphere.[10]
Aerobic respiration
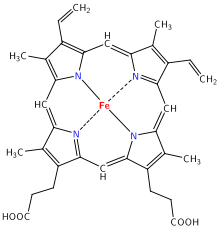
Molecular oxygen, O2, is essential for cellular respiration in all aerobic organisms. Oxygen is used as an electron acceptor in mitochondria to generate chemical energy in the form of adenosine triphosphate (ATP) during oxidative phosphorylation.
In vertebrates, oxygen uptake is carried out by the following processes:
Oxygen diffuses through membranes and into red blood cells after inhalation into the lungs. The heme group of hemoglobin binds oxygen when it is present, changing hemoglobin's color from bluish red to bright red.[11][12] Vertebrate animals use hemoglobin in their blood to transport oxygen from their lungs to their tissues, but other animals use hemocyanin (molluscs and some arthropods) or hemerythrin (spiders and lobsters).[13] A liter of blood can dissolve 200 cc of oxygen gas, which is much more than water can dissolve (see Physical Properties).[13]
After being carried in blood to a body tissue in need of oxygen, O2 is handed-off from the heme group to monooxygenase, an enzyme that also has an active site with an atom of iron.[13] Monooxygenase uses oxygen to catalyze many oxidation reactions in the body. Carbon dioxide, a waste product, is released from the cell and into the blood, where it combines with bicarbonate and hemoglobin for transport to the lungs. Blood circulates back to the lungs and the process repeats.[9]
Reactive oxygen species
Reactive oxygen species are dangerous by-products that sometimes result from the use of oxygen in organisms. Important examples include; oxygen free radicals such as the highly-dangerous superoxide O2-, and the less harmful hydrogen peroxide (H2O2).[13] The body uses superoxide dismutase to reduce superoxide radicals to hydrogen peroxide. Glutathione peroxidase and similar enzymes then convert the H2O2 to water and dioxygen.[13]
Parts of the immune system of higher organisms, however, create peroxide, superoxide, and singlet oxygen to destroy invading microbes. Recently, singlet oxygen has been found to be a source of biologically-produced ozone: This reaction proceeds through an unusual compound dihydrogen trioxide, also known as trioxidane, (HOOOH), which is an antibody-catalyzed product of singlet oxygen and water. This compound, in turn, disproportionates to ozone and peroxide, providing two powerful antibacterials. The body's range of defense against all of these active oxidizing agents is hardly surprising, then, given their "deliberate" employment as antimicrobial agents in the immune response.[14] Reactive oxygen species also play an important role in the hypersensitive response of plants against pathogen attack.[4]
- ^
Raven, Peter H. (2005). Biology of Plants, 7th Edition. New York: W.H. Freeman and Company Publishers. pp. 115–127. ISBN 0-7167-1007-2.
{{cite book}}
: Unknown parameter|coauthors=
ignored (|author=
suggested) (help) - ^
Fenical, William (1983). "Marine Plants: A Unique and Unexplored Resource". Plants: the potentials for extracting protein, medicines, and other useful chemicals (workshop proceedings). DIANE Publishing. p. 147. ISBN 1428923977.
{{cite book}}
: Unknown parameter|month=
ignored (help) - ^ Broeker, W.S. (2006). "Breathing easy, Et tu, O2". Columbia University. Retrieved 2007-10-21.
- ^ a b c d Raven, Peter H. (2005). Biology of Plants, 7th Edition. New York: W.H. Freeman and Company Publishers. pp. 115–127. ISBN 0-7167-1007-2.
{{cite book}}
: Unknown parameter|coauthors=
ignored (|author=
suggested) (help) - ^
Raval M, Biswal B, Biswal U (2005). "The mystery of oxygen evolution: analysis of structure and function of photosystem II, the water-plastoquinone oxido-reductase". Photosynthesis Research. 85 (3): 267–93. doi:10.1007/s11120-005-8163-4. PMID 16170631.
{{cite journal}}
: CS1 maint: multiple names: authors list (link) - ^ Campbell, Neil A. (2005). Biology, 7th Edition. San Francisco: Pearson - Benjamin Cummings. pp. 522–523. ISBN 0-8053-7171-0.
{{cite book}}
: Unknown parameter|coauthors=
ignored (|author=
suggested) (help) - ^ Freeman, Scott (2005). Biological Science, 2nd Edition. Upper Saddle River, NJ: Pearson - Prentice Hall. pp. 214, 586. ISBN 0-13-140941-7.
{{cite book}}
: Cite has empty unknown parameter:|coauthors=
(help) - ^ a b Robert A. Berner (1999-09-18). "Atmospheric oxygen over Phanerozoic time". Proceedings of the National Academy of Sciences of the USA (20): 10955–10957. Retrieved 2007-12-16.
{{cite journal}}
: Unknown parameter|wolume=
ignored (help) - ^ a b Emsley 2001, p.303
- ^ Malcom Dole (1965). "The Natural History of Oxygen" (PDF). The Journal of General Physiology. 49: 5–27. Retrieved 2007-12-16.
- ^ CO2 is released from another part of the hemoglobin molecule, as its acid, which causes CO2 to be released from bicarbonate, its major reservoir in blood plasma (see Bohr effect)
- ^ Cite error: The named reference
GuideElem48
was invoked but never defined (see the help page). - ^ a b c d e Cite error: The named reference
NBB298
was invoked but never defined (see the help page). - ^ Hoffmann, Roald (2004). "The Story of O". American Scientist. 92 (1): 23. Retrieved 2007-03-03.