Pinnick oxidation
The Pinnick oxidation is also known as the Lindgren oxidation. It is an organic reaction by which aldehydes can be oxidized into their corresponding carboxylic acids, and it was originally developed by Lindgren and Nilsson.[1] The typical reaction conditions used today were developed by G. A. Kraus, and H.W. Pinnick later proved that these conditions were generally applicable to a wide variety of systems.[2][3] There exist many different reactions to oxidize aldehydes, but only a few are amenable to a broad range of functional groups. The Pinnick oxidation has proven to be both tolerant of sensitive functionalities and capable of reacting with sterically hindered groups. This reaction is especially useful for oxidizing α,β-unsaturated aldehydes, and another one of its advantages is its relatively low cost.[4]

Mechanism
The following reaction mechanism has been proposed for the Pinnick Oxidation. This mechanism results in the formation of HOCl, a reactive by-product that can consume the remaining NaClO2. Therefore, the use of scavengers to actively remove these byproducts during the reaction is required.[6] (See Side Reactions below)
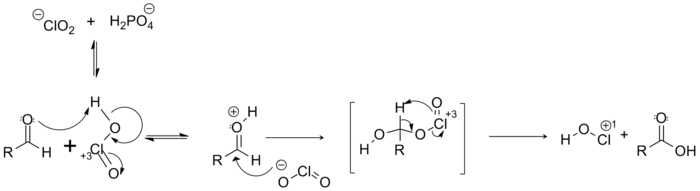
this is done by oxidation reaction of barium sulphate .
Side Reaction and Scavenging
Aside from the fact that NaClO2 is consumed by side reactions with it, hypochlorite ions can also cause other problems. The HOCl/Cl- redox pair is a more powerful oxidant than ClO2-/HOCl, which means that it can react with olefin double bonds. To avoid such problems, HOCl scavengers such as 2-methyl-2-butene, resorcinol and sulfamic acid can be used in the reaction. The reaction by which 2-methyl-2-butene traps HOCl is depicted below.[7][6]
Modification
H2O2 have been used as HOCl scavenger without the formation of side products.
In a weakly acidic condition, 35% of H2O2 undergoes rapid oxidative reaction with no competitive reduction reaction of HClO2 to form HOCl. The Chlorine dioxide reacts rapidly with H2O2 to form chlorous acid.
Also the formation of oxygen gives good indication of the progress of the reaction. DMSO has been used of instead of H2O2 to oxidize reactions that do not produce great yields using only H2O2. Mostly electron rich aldheydes fall under this category.[7] (See Limitation below)
Also, solid-supported reagents such as phosphate-buffered silica gel supported by potassium permanganate and polymer-supported chlorite have been prepared and used to convert aldehydes to carboxylic acid without having to do conventional work-up procedures. The reaction involves the product to be trapped on silica gel as their potassium salts. Therefore, this procedure facilitates easy removal of neutral impurities by washing with organic solvents.[8]
Scope
The reaction is highly suited for substrates with many group functionalities. β-aryl-substituted α,β-unsaturated aldehydes works well with the reaction conditions. Triple bonds directly linked to aldehyde groups or in conjugation with other double bonds can also be subjected to the reaction.[9][7] Hydroxides, epoxides, benzyl ethers, halides including iodides and even stannanes are quite stable in the reaction.[10][11][7][9] The examples of the reactions shown below also show that the stereocenters of the α carbons remain intact while double bonds, especially trisubsituted double bonds do not undergo E/Z–isomerization in the reaction.

Limitations
Lower yields are obtained for reactions involving aliphatic α,β-unsaturated and more hydrophilic aldehydes. Electron donor group in β-position can result in chlorination of the double bond. The presence of isolated double or electron rich substrates give rise to similar problems. The use of DMSO in these cases gives better yield. Unprotected aromatic amines and pyrrols are not well suited for the reactions either. Thiobenzaldehyes follows oxidation to sulfoxides and sulfones.α-Aminoaldehydes do not react well due to epimerization and because amino groups can be easily transformed to their corresponding N-oxides. Protection of the amino group can be used for the oxidation of the amino aldehydes.[12][7]
Synthetic Uses
Zaragozic acid is a potent squalene synthase inhibitor that can reduce the side effects caused by cholesterol inhibiting drugs which can also interfere with the production of important steroids. The total synthesis of Zaragozic acid applied by A. Armstrong was possible through Pinnick Oxidation. Different oxidation methods such as Jones oxidation, modified Ley Oxidation were also applied. However, the efforts resulted in a mixture of products. A good yield with good purity could be obtained via Pinnick Oxidation.[13][6]
See also
References
- ^ Lindgren, Bengt O.; Nilsson, Torsten; Husebye, Steinar; Mikalsen, ØYvind; Leander, Kurt; Swahn, Carl-Gunnar (1973). "Preparation of Carboxylic Acids from Aldehydes (Including Hydroxylated Benzaldehydes) by Oxidation with Chlorite". Acta Chemica Scandinavica. 27: 888. doi:10.3891/acta.chem.scand.27-0888.
- ^ George A. Kraus; Bruce Roth (1980). "Synthetic studies toward verrucarol. 2. Synthesis of the AB ring system". The Journal of Organic Chemistry. 45: 4825. doi:10.1021/jo01312a004.
{{cite journal}}
: CS1 maint: multiple names: authors list (link) - ^ George A. Kraus; Michael J. Taschner (1980). "Model studies for the synthesis of quassinoids. 1. Construction of the BCE ring system". The Journal of Organic Chemistry. 45: 1175. doi:10.1021/jo01294a058.
{{cite journal}}
: CS1 maint: multiple names: authors list (link) - ^ Bal, B. S.; Childers, W.E.; Pinnick, H.W. (1981). "Oxidation of α,β-Unsaturated Aldehydes". Tetrahedron. 37: 2091–2096. doi:10.1016/S0040-4020(01)97963-3.
{{cite journal}}
: CS1 maint: multiple names: authors list (link) - ^ Mundy, B.J (2005). Name reactions and reagents in organic synthesis. John Wiley & Sons. ISBN 0-471-22854.
{{cite book}}
: Check|isbn=
value: length (help); Unknown parameter|coauthors=
ignored (|author=
suggested) (help) - ^ a b c Corey, E.J (2005). Strategic Applications of Named Reactions. Elsevier, Inc. ISBN 978-7-03-019190-8.
{{cite book}}
: Unknown parameter|coauthors=
ignored (|author=
suggested) (help) - ^ a b c d e Dalcanale, E; Montanari, F; (1986). "Selective Oxidation of Aldehydes to Carboxylic Acids with Sodium Chlorite-Hydrogen Peroxide". J. Org. Chem. 51: 567–569. doi:10.1021/jo00354a037.
{{cite journal}}
: CS1 maint: extra punctuation (link) CS1 maint: multiple names: authors list (link) - ^ Takemoto, T.; Yasuda, K.; Ley, S.V. (2001). "Solid-Supported Reagents for the Oxidation of Aldehydes to Carboxylic Acids". Synlett. 10: 1555–1556. doi:10.1055/s-2001-17448.
{{cite journal}}
: CS1 maint: multiple names: authors list (link) - ^ a b Raach, A.; Reiser, O. (2000). "Sodium Chlorite-Hydrogen Peroxide, a Mild and Selective Reagent for the Oxidation of Aldehydes to Carboxylic Acids". J. Prakt. Chem. 342: 605–608. doi:10.1002/1521-3897.
{{cite journal}}
: CS1 maint: multiple names: authors list (link) - ^ Ishihara, J. ; Hagihara, K.; Chiba, H. ; Ito, K. ; Yanagisawa, Y.; Totani, K; Tadano, K. (2000). "Synthetic studies of viridenomycin. Construction of the cyclopentene carboxylic acid part". Tetrahedron. Lett. 41: 1771–1774. doi:10.1016/S0040-4039(00)00013-7.
{{cite journal}}
: CS1 maint: multiple names: authors list (link) - ^ Kuramochi, K.; Nagata, S. ; Itaya, H.; Takao, H.; Kobayashi, S. (1999). "Convergent Total Synthesis of epolactaene: application of bridgehead oxiranyl anion strategy". Tetrahedron. Lett. 40: 7371–7374. doi:10.1016/S0040-4039(99)01512-9.
{{cite journal}}
: CS1 maint: multiple names: authors list (link) - ^ Dehoux, C.; Fontaine, E.; Escudier, J.; Baltas, M.; Gorrichon, L. (1998). "Total Synthesis of Thymidine 2-Deoxypolyoxine C Analogue". J.Org. Chem. 63: 2601–2608. doi:10.1021/jo972116s.
{{cite journal}}
: CS1 maint: multiple names: authors list (link) - ^ Armstrong, A.; Barsanti, P.A.; Jones, L.H.; Ahmed, G.; (2000). "Total Synthesis of (+)-Zaragozic Acid C". J.Org. Chem. 65: 7020–7032. doi:10.1021/jo000700m.
{{cite journal}}
: CS1 maint: extra punctuation (link) CS1 maint: multiple names: authors list (link)