Enthalpy: Difference between revisions
Visioncurve (talk | contribs) Reverting edit(s) by 103.249.77.222 (talk) to rev. 1026638063 by Chjoaygame: non-constructive (RW 16.1) |
No edit summary Tag: Reverted |
||
Line 1: | Line 1: | ||
{{ |
{{short description|Partial intermolecular bonding interaction}} |
||
[[Image:3D model hydrogen bonds in water.svg|right|thumb|Model of hydrogen bonds (1) between molecules of [[Properties of water|water]]]] |
|||
{{Distinguish|Entropy}} |
|||
[[File:NTCDI AFM2a.jpg|thumb|[[Non-contact atomic force microscopy|AFM]] image of [[napthalenetetracarboxylic diimide]] molecules on silver-terminated silicon, interacting via hydrogen bonding, taken at 77 K.<ref>{{cite journal|doi=10.1038/ncomms4931|title=Mapping the force field of a hydrogen-bonded assembly|journal=Nature Communications|volume=5|pages=3931|year=2014|last1=Sweetman|first1=A. M.|last2=Jarvis|first2=S. P.|last3=Sang|first3=Hongqian|last4=Lekkas|first4=I.|last5=Rahe|first5=P.|last6=Wang|first6=Yu|last7=Wang|first7=Jianbo|last8=Champness|first8=N.R.|last9=Kantorovich|first9=L.|last10=Moriarty|first10=P.|bibcode=2014NatCo...5.3931S|pmid=24875276|pmc=4050271}}</ref> ("Hydrogen bonds" in the top image are exaggerated by artifacts of the imaging technique.<ref>{{Cite journal|last1=Hapala|first1=Prokop|last2=Kichin|first2=Georgy|last3=Wagner|first3=Christian|last4=Tautz|first4=F. Stefan|last5=Temirov|first5=Ruslan|last6=Jelínek|first6=Pavel|date=2014-08-19|title=Mechanism of high-resolution STM/AFM imaging with functionalized tips|journal=Physical Review B|volume=90|issue=8|pages=085421|doi=10.1103/PhysRevB.90.085421|arxiv=1406.3562|bibcode=2014PhRvB..90h5421H|s2cid=53610973}}</ref><ref>{{Cite journal|last1=Hämäläinen|first1=Sampsa K.|last2=van der Heijden|first2=Nadine|last3=van der Lit|first3=Joost|last4=den Hartog|first4=Stephan|last5=Liljeroth|first5=Peter|last6=Swart|first6=Ingmar|date=2014-10-31|title=Intermolecular Contrast in Atomic Force Microscopy Images without Intermolecular Bonds|journal=Physical Review Letters|volume=113|issue=18|pages=186102|doi=10.1103/PhysRevLett.113.186102|pmid=25396382|url=http://dspace.library.uu.nl:8080/handle/1874/307996|bibcode=2014PhRvL.113r6102H|url-status=dead|archive-url=https://web.archive.org/web/20180120232850/http://dspace.library.uu.nl:8080/handle/1874/307996|archive-date=2018-01-20|arxiv=1410.1933|hdl=1874/307996|s2cid=8309018|access-date=2017-08-30}}</ref>)]] |
|||
{{Thermodynamics |potentials}} |
|||
'''Enthalpy''' {{IPAc-en|ˈ|ɛ|n|θ|əl|p|i|audio=en-US-enthalpy.ogg}} is a property of a [[thermodynamic system]], and is defined as the sum of the system's [[internal energy]] and the product of its pressure and volume.<ref>{{cite web |url=http://www.oxforddictionaries.com/us/definition/american_english/enthalpy |title=Oxford Living Dictionaries |access-date=2018-02-19 |url-status=live |archive-url=https://web.archive.org/web/20160817085918/http://www.oxforddictionaries.com/us/definition/american_english/enthalpy |archive-date=2016-08-17 }}</ref><ref>{{cite web |url=http://goldbook.iupac.org/html/E/E02141.html |title=IUPAC Gold Book. Enthalpy, ''H'' |access-date=2018-02-19}}</ref> |
|||
It is a [[state function]] used in many measurements in chemical, biological, and physical systems at a constant pressure, that is conveniently provided by the large ambient atmosphere. The pressure–volume term expresses the [[work (physics)|work]] required to establish the system's physical dimensions, i.e. to make room for it by displacing its surroundings.<ref>{{cite book|first=Mark W. |last=Zemansky |date=1968 |title=Heat and Thermodynamics |url=https://archive.org/details/heatthermodynami0000zema |url-access=registration |chapter=Chapter 11 |edition=5th |page=[https://archive.org/details/heatthermodynami0000zema/page/275 275] |publisher=McGraw-Hill |location=New York, NY}}</ref><ref>{{cite book|first1=G. J. |last1=Van Wylen |first2=R. E. |last2=Sonntag |date=1985 |title=Fundamentals of Classical Thermodynamics |chapter=Section 5.5 |edition=3rd |publisher=John Wiley & Sons |location=New York |isbn=978-0-471-82933-1}}</ref> As a state function, enthalpy depends only on the final configuration of internal energy, pressure, and volume, not on the path taken to achieve it. |
|||
A '''hydrogen bond''' (or '''H-bond''') is a primarily [[Electrostatics|electrostatic]] force of attraction between a [[hydrogen]] (H) atom which is [[Covalent bond|covalently bound]] to a more [[electronegativity|electronegative]] atom or group, and another electronegative atom bearing a [[lone pair]] of electrons—the hydrogen bond acceptor (Ac). Such an interacting system is generally denoted Dn–H···Ac, where the solid line denotes a polar [[covalent bond]], and the dotted or dashed line indicates the hydrogen bond.<ref name=":0">{{Cite journal|last1=Arunan|first1=Elangannan|last2=Desiraju|first2=Gautam R.|last3=Klein|first3=Roger A.|last4=Sadlej|first4=Joanna|last5=Scheiner|first5=Steve|last6=Alkorta|first6=Ibon|last7=Clary|first7=David C.|last8=Crabtree|first8=Robert H.|last9=Dannenberg|first9=Joseph J.|date=2011-07-08|title=Definition of the hydrogen bond (IUPAC Recommendations 2011)|url=http://www.degruyter.com/view/j/pac.2011.83.issue-8/pac-rec-10-01-02/pac-rec-10-01-02.xml|journal=Pure and Applied Chemistry|volume=83|issue=8|pages=1637–1641|doi=10.1351/PAC-REC-10-01-02|s2cid=97688573|issn=1365-3075}}</ref> The most frequent donor and acceptor atoms are the second-row elements [[nitrogen]] (N), [[oxygen]] (O), and [[fluorine]] (F) |
|||
The unit of measurement for enthalpy in the [[International System of Units]] (SI) is the [[joule]]. Other historical conventional units still in use include the [[calorie]] and the [[British thermal unit]] (BTU). |
|||
Hydrogen bonds can be [[intermolecular]] (occurring between separate molecules) or [[Intramolecular force|intramolecular]] (occurring among parts of the same molecule).<ref>Pimentel, G. ''The Hydrogen Bond'' Franklin Classics, 2018), {{ISBN|0343171600}}</ref><ref>Jeffrey, G. A.; ''An introduction to hydrogen bonding''; Oxford university press New York, 1997. {{ISBN|0195095499}}</ref><ref>Jeffrey, G. A.; Saenger, W. ''Hydrogen bonding in biological structures''; Springer: Berlin, '''1994, 2012''' Springer; {{ISBN|3540579036}}</ref><ref>{{GoldBookRef|file = H02899|title = hydrogen bond}}</ref> The energy of a hydrogen bond depends on the geometry, the environment, and the nature of the specific donor and acceptor atoms, and can vary between 1 and 40 kcal/mol.<ref name="The Hydrogen Bond in the Solid Stat">{{cite journal|title = The Hydrogen Bond in the Solid State|journal = [[Angew. Chem. Int. Ed.]]|year = 2002|volume = 41|pages = 48–76|doi = 10.1002/1521-3773(20020104)41:1<48::AID-ANIE48>3.0.CO;2-U |last1 = Steiner|first1 = Thomas|issue = 1|pmid = 12491444}}</ref> This makes them somewhat stronger than a [[van der Waals force|van der Waals interaction]], and weaker than fully [[covalent bond|covalent]] or [[ionic bond]]s. This type of bond can occur in inorganic molecules such as water and in [[organic molecules]] like DNA and proteins. Hydrogen bonds are responsible for holding such materials as [[paper]] and [[wool|felted wool]] together, and for causing separate sheets of paper to stick together after becoming wet and subsequently drying. |
|||
The total enthalpy of a system cannot be measured directly because the internal energy contains components that are unknown, not easily accessible, or are not of interest in thermodynamics. In practice, a change in enthalpy is the preferred expression for measurements at constant pressure, because it simplifies the description of [[energy transfer]]. When matter transfer into or out of the system is also prevented, at constant pressure the enthalpy change equals the energy exchanged with the environment by [[heat]]. |
|||
The hydrogen bond is responsible for many of the anomalous physical and chemical properties of compounds of N, O, and F. In particular, intermolecular hydrogen bonding is responsible for the high boiling point of [[water]] (100 °C) compared to the other [[Hydrogen chalcogenide|group-16 hydride]]s that have much weaker hydrogen bonds.<ref>{{cite journal|journal=J. Am. Chem. Soc. |volume=93|issue=15|pages=3613–3620|author1=Sabin, John R. |title=Hydrogen bonds involving sulfur. I. Hydrogen sulfide dimer|doi=10.1021/ja00744a012|year=1971}}</ref> Intramolecular hydrogen bonding is partly responsible for the [[secondary structure|secondary]] and [[tertiary structure|tertiary]] structures of [[protein]]s and [[nucleic acid]]s. It also plays an important role in the structure of [[polymer]]s, both synthetic and natural. |
|||
In chemistry, the standard [[enthalpy of reaction]] is the enthalpy change when reactants in their [[standard state]]s ({{nowrap|1={{math|''p''}} = 1 bar}}, {{nowrap|1={{math|''T''}} = 298 K}}) change to products in their standard states.<ref>{{cite book |last1=Atkins |first1=Peter |last2=de Paula |first2=Julio |title=Atkins' Physical Chemistry |date=2006 |publisher=W.H.Freeman |isbn=0-7167-8759-8 |page=51 |edition=8th}}</ref> This quantity is the [[Standard enthalpy of reaction|standard heat of reaction]] at constant pressure and temperature, but it can be measured by [[Calorimeter|calorimetric]] methods in which the temperature does vary, provided that the initial and final pressure and temperature correspond to the standard state. The value does not depend on the path from initial to final state since enthalpy is a [[state function]]. |
|||
==Bonding== |
|||
Calibration of enthalpy changes requires a reference point. Enthalpies for chemical substances at constant pressure usually refer to [[standard state]]: most commonly {{convert|1|bar|kPa}} pressure. Standard state does not strictly specify a temperature, but expressions for enthalpy generally reference the standard heat of formation at {{cvt|25|°C|K}}. For [[endothermic]] (heat-absorbing) processes, the change {{math|Δ''H''}} is a positive value; for [[exothermic]] (heat-releasing) processes it is negative. |
|||
[[Image:Hydrogen Bond Quadruple AngewChemIntEd 1998 v37 p75.jpg|thumb|300px|An example of [[intermolecular]] hydrogen bonding in a [[molecular self-assembly|self-assembled]] dimer complex.<ref>{{cite journal| journal=[[Angew. Chem. Int. Ed.]] |title= Self-Complementarity Achieved through Quadruple Hydrogen Bonding| year= 1998|volume=37|issue=1–2 |pages= 75–78| doi=10.1002/(SICI)1521-3773(19980202)37:1/2<75::AID-ANIE75>3.0.CO;2-R|last1= Beijer|first1= Felix H.|last2= Kooijman|first2= Huub|last3= Spek|first3= Anthony L.|last4= Sijbesma|first4= Rint P.|last5= Meijer|first5= E. W.}}</ref> The hydrogen bonds are represented by dotted lines.]] |
|||
[[File:Acetylacetone tautomerism.svg|thumb|300x300px|[[Intramolecular force|Intramolecular]] hydrogen bonding in [[acetylacetone]] helps stabilize the [[enol]] [[tautomer]].]] |
|||
===Definitions and general characteristics=== |
|||
The enthalpy of an [[ideal gas]] is independent of its pressure, and depends only on its temperature, which correlates to its internal energy. Real gases at common temperatures and pressures often closely approximate this behavior, which simplifies practical thermodynamic design and analysis. |
|||
A hydrogen atom attached to a relatively [[electronegativity|electronegative]] atom is the hydrogen bond ''donor''.<ref>{{cite book|last = Campbell|first = Neil A.|author2 = Brad Williamson|author3 = Robin J. Heyden|title = Biology: Exploring Life|publisher = Pearson Prentice Hall|year = 2006|location = Boston, Massachusetts|url = http://www.phschool.com/el_marketing.html|isbn = 978-0-13-250882-7|url-status = dead|archive-url = https://web.archive.org/web/20141102041816/http://www.phschool.com/el_marketing.html|archive-date = 2014-11-02|access-date = 2008-11-11}}</ref> C-H bonds only participate in hydrogen bonding when the carbon atom is bound to electronegative substituents, as is the case in [[chloroform]], CHCl<sub>3</sub>.<ref>{{cite journal|author1=Wiley, G.R. |author2=Miller, S.I. |doi=10.1021/ja00765a001|title=Thermodynamic parameters for hydrogen bonding of chloroform with Lewis bases in cyclohexane. Proton magnetic resonance study|year=1972|journal=Journal of the American Chemical Society|volume=94|issue=10|pages=3287}}</ref> In a hydrogen bond, the electronegative atom not covalently attached to the hydrogen is named proton acceptor, whereas the one covalently bound to the hydrogen is named the proton donor. While this nomenclature is recommended by the IUPAC,<ref name=":0">{{Cite journal|last1=Arunan|first1=Elangannan|last2=Desiraju|first2=Gautam R.|last3=Klein|first3=Roger A.|last4=Sadlej|first4=Joanna|last5=Scheiner|first5=Steve|last6=Alkorta|first6=Ibon|last7=Clary|first7=David C.|last8=Crabtree|first8=Robert H.|last9=Dannenberg|first9=Joseph J.|date=2011-07-08|title=Definition of the hydrogen bond (IUPAC Recommendations 2011)|url=http://www.degruyter.com/view/j/pac.2011.83.issue-8/pac-rec-10-01-02/pac-rec-10-01-02.xml|journal=Pure and Applied Chemistry|volume=83|issue=8|pages=1637–1641|doi=10.1351/PAC-REC-10-01-02|s2cid=97688573|issn=1365-3075}}</ref> it can be misleading, since in other donor-acceptor bonds, the donor/acceptor assignment is based on the source of the electron pair (such nomenclature is also used for hydrogen bonds by some authors<ref name="The Hydrogen Bond in the Solid Stat"/>). In the hydrogen bond donor, the H center is protic. The donor is a Lewis base. Hydrogen bonds are represented as H···Y system, where the dots represent the hydrogen bond. Liquids that display hydrogen bonding (such as water) are called '''associated liquids'''. |
|||
[[File:H-donor-acceptor.svg|alt=|thumb|320x320px|Examples of hydrogen bond donating (donors) and hydrogen bond accepting groups (acceptors)]] |
|||
[[File:Acetic Acid Hydrogenbridge V.1.svg|thumb|300px|Cyclic dimer of acetic acid; dashed <span style="color:green;">'''green'''</span> lines represent hydrogen bonds]] |
|||
The hydrogen bond is often described as an [[electrostatic]] [[dipole-dipole interaction]]. However, it also has some features of [[covalent bond]]ing: it is directional and strong, produces interatomic distances shorter than the sum of the van der Waals radii, and usually involves a limited number of interaction partners, which can be interpreted as a type of [[Valence (chemistry)|valence]]. These covalent features are more substantial when acceptors bind hydrogens from more electronegative donors. |
|||
==Definition== |
|||
The enthalpy {{math|''H''}} of a thermodynamic system is defined as the sum of its internal energy {{math|''U''}} and the work required to achieve its pressure and volume:<ref name="Guggenheim">{{cite book |first=E. A. |last=Guggenheim |title=Thermodynamics |publisher=North-Holland Publishing Company |location=Amsterdam |date=1959}}</ref><ref>{{Cite book |author=Zumdahl, Steven S. |chapter=Thermochemistry |title=Chemistry |publisher=Cengage Learning |year=2008 |isbn=978-0-547-12532-9 |page=243 |chapter-url=https://books.google.com/books?id=LLWkH82PNbYC&pg=PA243 |url-status=live |archive-url=https://web.archive.org/web/20131114034015/http://books.google.com/books?id=LLWkH82PNbYC&pg=PA243 |archive-date=2013-11-14}}</ref> |
|||
: {{math|''H'' {{=}} ''U'' + ''pV''}}, |
|||
where {{mvar|p}} is [[pressure]], and {{mvar|V}} is the [[Volume (thermodynamics)|volume]] of the system. |
|||
As part of a more detailed list of criteria, the IUPAC publication acknowledges that the attractive interaction can arise from some combination of electrostatics (multipole-multipole and multipole-induced multipole interactions), covalency (charge transfer by orbital overlap), and dispersion (London forces), and states that the relative importance of each will vary depending on the system. However, a footnote to the criterion recommends the exclusion of interactions in which dispersion is the primary contributor, specifically giving Ar---CH<sub>4</sub> and CH<sub>4</sub>---CH<sub>4</sub> as examples of such interactions to be excluded from the definition.<ref name=":0" /> |
|||
Enthalpy is an [[extensive property]]; it is proportional to the size of the system (for homogeneous systems). As [[intensive properties]], the [[specific enthalpy]] {{math|''h'' {{=}} {{sfrac|''H''|''m''}}}} is referenced to a unit of [[mass]] {{mvar|m}} of the system, and the molar enthalpy {{math|''H''<sub>m</sub> is {{sfrac|''H''|''n''}}}}, where {{mvar|n}} is the number of [[Mole (unit)|moles]]. For inhomogeneous systems the enthalpy is the sum of the enthalpies of the composing subsystems: |
|||
Nevertheless, most introductory textbooks still restrict the definition of hydrogen bond to the "classical" type of hydrogen bond characterized in the opening paragraph. |
|||
: <math>H = \sum_k H_k,</math> |
|||
where |
|||
: {{mvar|H}} is the total enthalpy of all the subsystems, |
|||
: {{mvar|k}} refers to the various subsystems, |
|||
: {{mvar|H<sub>k</sub>}} refers to the enthalpy of each subsystem. |
|||
Weaker hydrogen bonds<ref>Desiraju, G. R. and Steiner, T. The Weak Hydrogen Bond: In Structural Chemistry and Biology , International Union of Crystallography;'''2001''', {{ISBN|0198509707}}</ref> are known for hydrogen atoms bound to elements such as sulfur (S) or chlorine (Cl); even carbon (C) can serve as a donor, particularly when the carbon or one of its neighbors is electronegative (e.g., in chloroform, aldehydes and terminal acetylenes).<ref>Nishio, M.; Hirota, M.; Umezawa, Y. ''The CH–π Interactions''; Wiley-VCH, New York, 1998. • Wiley-VCH; 1998) {{ISBN|0471252905}}</ref><ref>{{cite journal | last1 = Nishio | first1 = M | year = 2011 | title = The CH/[small pi] hydrogen bond in chemistry. "Title | journal = Phys. Chem. Chem. Phys. | volume = 13 | issue = 31 | pages = 13873–13900 | doi=10.1039/c1cp20404a| pmid = 21611676 }}</ref> Gradually, it was recognized that there are many examples of weaker hydrogen bonding involving donor other than N, O, or F and/or acceptor Ac with electronegativity approaching that of hydrogen (rather than being much more electronegative). Though these "non-traditional" hydrogen bonding interactions are often quite weak (~1 kcal/mol), they are also ubiquitous and are increasingly recognized as important control elements in receptor-ligand interactions in medicinal chemistry or intra-/intermolecular interactions in materials sciences. |
|||
A closed system may lie in thermodynamic equilibrium in a static [[gravitational field]], so that its pressure {{mvar|p}} varies continuously with [[altitude]], while, because of the equilibrium requirement, its temperature {{mvar|T}} is invariant with altitude. (Correspondingly, the system's [[gravitational potential energy]] density also varies with altitude.) Then the enthalpy summation becomes an [[integral]]: |
|||
: <math>H = \int (\rho h) \, dV,</math> |
|||
where |
|||
: {{mvar|ρ}} ("[[rho]]") is [[density]] (mass per unit volume), |
|||
: {{mvar|h}} is the specific enthalpy (enthalpy per unit mass), |
|||
: {{math|(''ρh'')}} represents the [[Energy density|enthalpy density]] (enthalpy per unit volume), |
|||
: {{mvar|dV}} denotes an [[infinitesimal]]ly small element of volume within the system, for example, the volume of an infinitesimally thin horizontal layer, |
|||
: the integral therefore represents the sum of the enthalpies of all the elements of the volume. |
|||
The definition of hydrogen bonding has gradually broadened over time to include these weaker attractive interactions. In 2011, an [[IUPAC]] Task Group recommended a modern evidence-based definition of hydrogen bonding, which was published in the [[IUPAC]] journal ''[[Pure and Applied Chemistry]]''. This definition specifies: |
|||
The enthalpy of a closed homogeneous system is its energy function {{math|''H''(''S'',''p'')}}, with natural state variables its entropy {{math|''S''[''p'']}} and its pressure {{mvar|p}}. A differential relation for it can be derived as follows. We start from the [[first law of thermodynamics]] for closed systems for an infinitesimal process: |
|||
{{quote|The hydrogen bond is an attractive interaction between a hydrogen atom from a molecule or a molecular fragment X–H in which X is more electronegative than H, and an atom or a group of atoms in the same or a different molecule, in which there is evidence of bond formation.<ref name="arunen2011">{{cite journal|title = Definition of the hydrogen bond|journal = [[Pure Appl. Chem.]]|year = 2011|volume = 83|issue = 8|pages = 1637–1641|doi = 10.1351/PAC-REC-10-01-02 |last1 = Arunan|first1 = Elangannan|last2 = Desiraju|first2 = Gautam R.|last3 = Klein|first3 = Roger A.|last4 = Sadlej|first4 = Joanna|last5 = Scheiner|first5 = Steve|last6 = Alkorta|first6 = Ibon|last7 = Clary|first7 = David C.|last8 = Crabtree|first8 = Robert H.|last9 = Dannenberg|first9 = Joseph J.|last10 = Hobza|first10 = Pavel|last11 = Kjaergaard|first11 = Henrik G.|last12 = Legon|first12 = Anthony C.|last13 = Mennucci|first13 = Benedetta|last14 = Nesbitt|first14 = David J.|s2cid = 97688573}}</ref>}} |
|||
: <math>dU = \delta Q - \delta W,</math> |
|||
where |
|||
: {{math|𝛿''Q''}} is a small amount of heat added to the system, |
|||
: {{math|𝛿''W''}} is a small amount of work performed by the system. |
|||
===Bond strength=== |
|||
In a homogeneous system in which only [[Reversible process (thermodynamics)|reversible]], or [[Quasistatic process|quasi-static]], processes are considered, the [[second law of thermodynamics]] gives {{math|𝛿''Q'' {{=}} ''T'' ''dS''}}, with {{mvar|T}} the [[absolute temperature]] and {{mvar|dS}} the infinitesimal change in [[entropy]] {{mvar|S}} of the system. Furthermore, if only {{mvar|pV}} work is done, {{math|𝛿''W'' {{=}} ''p'' ''dV''}}. As a result, |
|||
Hydrogen bonds can vary in strength from weak (1–2 kJ mol<sup>−1</sup>) to strong (161.5 kJ mol<sup>−1</sup> in the ion {{chem|link=Bifluoride|HF|2|-}}).<ref name=halide>{{cite journal|doi=10.1021/ic00182a010|title=Gas-phase bihalide and pseudobihalide ions. An ion cyclotron resonance determination of hydrogen bond energies in XHY- species (X, Y = F, Cl, Br, CN)|year=1984|last1=Larson|first1=J. W.|last2=McMahon|first2=T. B.|journal=Inorganic Chemistry|volume=23|issue=14|pages=2029–2033|title-link=ion cyclotron resonance}}</ref><ref>{{cite journal|author = Emsley, J.|title = Very Strong Hydrogen Bonds|journal = [[Chemical Society Reviews]]|year = 1980|volume = 9|issue = 1|pages = 91–124|doi = 10.1039/cs9800900091}}</ref> Typical [[Enthalpy|enthalpies]] in vapor include:<ref>V. David, N. Grinberg, S. C. Moldoveanu in ''Advances in Chromatography Volume 54'' (Eds.: E. Grushka, N. Grinberg), CRC Press, Boca Raton, '''2018''', chapter 3.</ref> |
|||
* F−H···:F (161.5 kJ/mol or 38.6 kcal/mol), illustrated uniquely by HF<sub>2</sub><sup>−</sup>, [[bifluoride]] |
|||
* O−H···:N (29 kJ/mol or 6.9 kcal/mol), illustrated water-ammonia |
|||
* O−H···:O (21 kJ/mol or 5.0 kcal/mol), illustrated water-water, alcohol-alcohol |
|||
* N−H···:N (13 kJ/mol or 3.1 kcal/mol), illustrated by ammonia-ammonia |
|||
* N−H···:O (8 kJ/mol or 1.9 kcal/mol), illustrated water-amide |
|||
* {{chem|OH|3|+}}···:{{chem|OH|2|}} (18 kJ/mol<ref>Data obtained using [[molecular dynamics]] as detailed in the reference and should be compared to 7.9 kJ/mol for bulk water, obtained using the same calculation.{{cite journal|title = Structure and energetics of the hydronium hydration shells|author1 = Markovitch, Omer|author2 = Agmon, Noam|journal = [[J. Phys. Chem. A]]|year = 2007|volume = 111|issue = 12|pages = 2253–2256|doi = 10.1021/jp068960g|pmid = 17388314|bibcode = 2007JPCA..111.2253M|url = http://www.fh.huji.ac.il/~agmon/Fullpaper/JPCA111-2253.pdf|url-status = dead|archive-url = https://web.archive.org/web/20140813174617/http://www.fh.huji.ac.il/%7Eagmon/Fullpaper/JPCA111-2253.pdf|archive-date = 2014-08-13|citeseerx = 10.1.1.76.9448|access-date = 2017-10-25}}</ref> or 4.3 kcal/mol) |
|||
The strength of intermolecular hydrogen bonds is most often evaluated by measurements of equilibria between molecules containing donor and/or acceptor units, most often in solution.<ref>{{cite journal | vauthors = Biedermann F, Schneider HJ | title = Experimental Binding Energies in Supramolecular Complexes | journal = Chemical Reviews | volume = 116 | issue = 9 | pages = 5216–300 | date = May 2016 | pmid = 27136957 | doi = 10.1021/acs.chemrev.5b00583 }}</ref> The strength of intramolecular hydrogen bonds can be studied with equilibria between conformers with and without hydrogen bonds. The most important method for the identification of hydrogen bonds also in complicated molecules is [[crystallography]], sometimes also NMR-spectroscopy. Structural details, in particular distances between donor and acceptor which are smaller than the sum of the van der Waals radii can be taken as indication of the hydrogen bond strength. |
|||
One scheme gives the following somewhat arbitrary classification: those that are 15 to 40 kcal/mol, 5 to 15 kcal/mol, and >0 to 5 kcal/mol are considered strong, moderate, and weak, respectively. |
|||
: <math>dU = T\,dS - p\,dV.</math> |
|||
===Structural details=== |
|||
Adding {{math|''d''(''pV'')}} to both sides of this expression gives |
|||
The X−H distance is typically ≈110 [[picometre|pm]], whereas the H···Y distance is ≈160 to 200 pm. The typical length of a hydrogen bond in water is 197 pm. The ideal bond angle depends on the nature of the hydrogen bond donor. The following hydrogen bond angles between a hydrofluoric acid donor and various acceptors have been determined experimentally:<ref>{{cite journal|doi=10.1039/CS9871600467|title=Angular geometries and other properties of hydrogen-bonded dimers: a simple electrostatic interpretation of the success of the electron-pair model|year=1987|last1=Legon|first1=A. C.|last2=Millen|first2=D. J.|journal=Chemical Society Reviews|volume=16|pages=467}}</ref> |
|||
{|class="wikitable" style="text-align:left" |
|||
|- |
|||
!Acceptor···donor||VSEPR geometry||Angle (°) |
|||
|- |
|||
|HCN···HF||linear||style="text-align:right"|180 |
|||
|- |
|||
|H<sub>2</sub>CO···HF||trigonal planar||style="text-align:right"|120 |
|||
|- |
|||
|H<sub>2</sub>O···HF||pyramidal||style="text-align:right"|46 |
|||
|- |
|||
|H<sub>2</sub>S···HF||pyramidal||style="text-align:right"|89 |
|||
|- |
|||
|SO<sub>2</sub>···HF||trigonal ||style="text-align:right"|142 |
|||
|- |
|||
|} |
|||
===Spectroscopy=== |
|||
: <math>dU + d(pV) = T\,dS - p\,dV + d(pV),</math> |
|||
Strong hydrogen bonds are revealed by downfield shifts in the [[proton NMR spectroscopy|<sup>1</sup>H NMR spectrum]]. For example, the acidic proton in the enol tautomer of [[acetylacetone]] appears at δ<sub>H</sub> 15.5, which is about 10 ppm downfield of a conventional alcohol.<ref>Friebolin, H., "Basic One- and Two- Dimensional NMR Spectroscopy, 4th ed.," VCH: Weinheim, 2008. {{ISBN|978-3-527-31233-7}}</ref> |
|||
In the IR spectrum, hydrogen bonding shifts the X-H stretching frequency to lower energy (i.e. the vibration frequency decreases). This shift reflects a weakening of the X-H bond. Certain hydrogen bonds - improper hydrogen bonds - show a blue shift of the X-H stretching frequency and a decrease in the bond length.<ref>{{cite journal |vauthors=Hobza P, Havlas Z |title=Blue-Shifting Hydrogen Bonds |journal=Chem. Rev. |volume=100 |issue=11 |pages=4253–4264 |year=2000 |doi=10.1021/cr990050q |pmid=11749346 }}</ref> H-bonds can also be measured by IR vibrational mode shifts of the acceptor. The amide I mode of backbone carbonyls in α-helices shifts to lower frequencies when they form H-bonds with side-chain hydroxyl groups.<ref name="Feldblum 2014 4085–4090">{{cite journal|last=Feldblum|first=Esther S.|author2=Arkin, Isaiah T.|title=Strength of a bifurcated H bond|journal=Proceedings of the National Academy of Sciences|volume=111|issue=11|pages=4085–4090|year=2014|doi=10.1073/pnas.1319827111|pmid=24591597|pmc=3964065|bibcode=2014PNAS..111.4085F|doi-access=free}}</ref> |
|||
or |
|||
===Theoretical considerations=== |
|||
: <math>d(U + pV) = T\,dS + V\,dp.</math> |
|||
Hydrogen bonding is of persistent theoretical interest.<ref>{{ Cite journal|last1=Weinhold|first1=Frank|last2=Klein|first2=Roger A.|year=2014|volume=15|pages=276–285|title=What is a hydrogen bond? Resonance covalency in the supramolecular domain|journal=Chemistry Education Research and Practice|issue=3|doi=10.1039/c4rp00030g}}</ref> According to a modern description O:H-O integrates both the intermolecular O:H lone pair ":" nonbond and the intramolecular H-O polar-covalent bond associated with O-O repulsive coupling.<ref>{{cite book|title= The Attribute of Water: Single Notion, Multiple Myths|year= 2016|isbn=978-981-10-0178-9|author= Sun, C. Q. |author2 = Sun, Yi }}</ref> |
|||
Quantum chemical calculations of the relevant interresidue potential constants (compliance constants) revealed{{how|date=December 2015}} large differences between individual H bonds of the same type. For example, the central interresidue N−H···N hydrogen bond between guanine and cytosine is much stronger in comparison to the N−H···N bond between the adenine-thymine pair.<ref>{{cite journal|doi=10.1021/ja046282a|pmid=15600318|title=Direct Assessment of Interresidue Forces in Watson−Crick Base Pairs Using Theoretical Compliance Constants|journal=Journal of the American Chemical Society|volume=126|issue=50|pages=16310–1|year=2004|last1=Grunenberg|first1=Jörg}}</ref> |
|||
So |
|||
Theoretically, the bond strength of the hydrogen bonds can be assessed using NCI index, [[non-covalent interactions index]], which allows a visualization of these [[non-covalent interactions]], as its name indicates, using the electron density of the system. |
|||
: <math>dH(S, p) = T\,dS + V\,dp.</math> |
|||
From interpretations of the [[anisotropy|anisotropies]] in the [[Compton scattering|Compton profile]] of ordinary ice that the hydrogen bond is partly covalent.<ref>{{cite journal|author=Isaacs, E.D.|journal= Physical Review Letters |doi=10.1103/PhysRevLett.82.600|title=Covalency of the Hydrogen Bond in Ice: A Direct X-Ray Measurement|year=1999|volume=82|issue=3|pages=600–603|bibcode = 1999PhRvL..82..600I |display-authors=etal}}</ref> However, this interpretation was challenged.<ref>{{Cite journal|last1=Ghanty|first1=Tapan K.|last2=Staroverov|first2=Viktor N.|last3=Koren|first3=Patrick R.|last4=Davidson|first4=Ernest R.|date=2000-02-01|title=Is the Hydrogen Bond in Water Dimer and Ice Covalent?|journal=Journal of the American Chemical Society|volume=122|issue=6|pages=1210–1214|doi=10.1021/ja9937019|issn=0002-7863}}</ref> |
|||
==Other expressions== |
|||
The above expression of {{mvar|dH}} in terms of entropy and pressure may be unfamiliar to some readers. However, there are expressions in terms of more familiar variables such as temperature and pressure:<ref name="Guggenheim" />{{rp|88}}<ref>{{cite book|first1=M. J. |last1=Moran |first2=H. N. |last2=Shapiro |title=Fundamentals of Engineering Thermodynamics |url=https://archive.org/details/fundamentalsengi00mora_077 |url-access=limited |edition=5th |date=2006 |publisher=John Wiley & Sons |page=[https://archive.org/details/fundamentalsengi00mora_077/page/n523 511]}}</ref> |
|||
Most generally, the hydrogen bond can be viewed as a [[metric (mathematics)|metric]]-dependent [[electrostatic]] [[scalar field]] between two or more intermolecular bonds. This is slightly different from the [[Intramolecular force|intramolecular]] [[bound states]] of, for example, [[covalent bond|covalent]] or [[ionic bond]]s; however, hydrogen bonding is generally still a [[bound state]] phenomenon, since the [[interaction energy]] has a net negative sum. The initial theory of hydrogen bonding proposed by [[Linus Pauling]] suggested that the hydrogen bonds had a partial covalent nature. This interpretation remained controversial until [[nuclear magnetic resonance|NMR techniques]] demonstrated information transfer between hydrogen-bonded nuclei, a feat that would only be possible if the hydrogen bond contained some covalent character.<ref>{{cite journal|title = Observation of through-hydrogen-bond (2h)J(HC') in a perdeuterated protein|journal = [[J Magn Reson]]|year = 1999|volume = 140|pages = 510–2|doi = 10.1006/jmre.1999.1899|pmid = 10497060|issue = 2|bibcode = 1999JMagR.140..510C |last1 = Cordier|first1 = F|last2 = Rogowski|first2 = M|last3 = Grzesiek|first3 = S|last4 = Bax|first4 = A|s2cid = 121429|url = https://semanticscholar.org/paper/313914f3a544c88c2fa13303a37500e9fd7a4c7c}}</ref> |
|||
:<math>dH = C_p\,dT + V(1 - \alpha T)\,dp.</math> |
|||
==History== |
|||
Here {{math|''C''<sub>''p''</sub>}} is the [[Heat capacity|heat capacity at constant pressure]] and {{mvar|α}} is the [[Coefficient of thermal expansion|coefficient of (cubic) thermal expansion]]: |
|||
The concept of hydrogen bonding once was challenging.<ref>{{cite journal|title=Hydrogen bonding: Homing in on a tricky chemical concept|author=Needham, Paul |journal=Studies in History and Philosophy of Science Part A|volume=44|year=2013|pages=51–65|doi=10.1016/j.shpsa.2012.04.001}}</ref> [[Linus Pauling]] credits T. S. Moore and T. F. Winmill with the first mention of the hydrogen bond, in 1912.<ref>{{cite book |
|||
|last=Pauling |first=L. |
|||
|title=The nature of the chemical bond and the structure of molecules and crystals; an introduction to modern structural chemistry |
|||
|url=https://archive.org/details/natureofchemical00paul |url-access=registration |publisher=Cornell University Press |
|||
|location=Ithaca (NY) |
|||
|year=1960 |
|||
|edition=3rd |
|||
|page=[https://archive.org/details/natureofchemical00paul/page/450 450] |
|||
|isbn=978-0-8014-0333-0 |
|||
}}</ref><ref>{{cite journal|journal=J. Chem. Soc. |volume=101|page=1635|author1=Moore, T. S.|author2=Winmill, T. F. |title=The state of amines in aqueous solution|doi=10.1039/CT9120101635|year=1912}}</ref> Moore and Winmill used the hydrogen bond to account for the fact that trimethylammonium hydroxide is a weaker base than [[tetramethylammonium hydroxide]]. The description of hydrogen bonding in its better-known setting, water, came some years later, in 1920, from [[Wendell Mitchell Latimer|Latimer]] and Rodebush.<ref>{{cite journal|doi=10.1021/ja01452a015|year=1920|last1=Latimer|first1=Wendell M.|last2=Rodebush|first2=Worth H.|journal=Journal of the American Chemical Society|volume=42|issue=7|pages=1419–1433|title=Polarity and ionization from the standpoint of the Lewis theory of valence|url=https://zenodo.org/record/1428832}}</ref> In that paper, Latimer and Rodebush cite work by a fellow scientist at their laboratory, [[Maurice Loyal Huggins]], saying, "Mr. Huggins of this laboratory in some work as yet unpublished, has used the idea of a hydrogen kernel held between two atoms as a theory in regard to certain organic compounds." |
|||
==Hydrogen bonds in small molecules== |
|||
:<math>\alpha = \frac{1}{V}\left(\frac{\partial V}{\partial T}\right)_p.</math> |
|||
[[Image:Hex ice.GIF|thumb|right|Crystal structure of hexagonal ice. Gray dashed lines indicate hydrogen bonds]] |
|||
[[File:NIMGLO12.png|right|thumb|120px|Structure of [[nickel bis(dimethylglyoximate)]], which features two linear hydrogen-bonds.]] |
|||
===Water=== |
|||
With this expression one can, in principle, determine the enthalpy if {{mvar|C<sub>p</sub>}} and {{mvar|V}} are known as functions of {{mvar|p}} and {{mvar|T}}. |
|||
A ubiquitous example of a hydrogen bond is found between [[water]] molecules. In a discrete water molecule, there are two hydrogen atoms and one oxygen atom. The simplest case is a pair of [[water]] molecules with one hydrogen bond between them, which is called the [[water dimer]] and is often used as a model system. When more molecules are present, as is the case with liquid water, more bonds are possible because the oxygen of one water molecule has two lone pairs of electrons, each of which can form a hydrogen bond with a hydrogen on another water molecule. This can repeat such that every water molecule is H-bonded with up to four other molecules, as shown in the figure (two through its two lone pairs, and two through its two hydrogen atoms). Hydrogen bonding strongly affects the [[crystal structure]] of [[ice]], helping to create an open hexagonal lattice. The density of ice is less than the density of water at the same temperature; thus, the solid phase of water floats on the liquid, unlike most other substances. |
|||
[[Liquid]] water's high [[boiling point]] is due to the high number of hydrogen bonds each molecule can form, relative to its low [[molecular mass]]. Owing to the difficulty of breaking these bonds, water has a very high boiling point, melting point, and viscosity compared to otherwise similar liquids not conjoined by hydrogen bonds. Water is unique because its oxygen atom has two lone pairs and two hydrogen atoms, meaning that the total number of bonds of a water molecule is up to four. |
|||
Note that for an [[ideal gas]], {{math|''αT'' {{=}} 1}},<ref group="note"><math>\alpha T=\frac{T}{V}\left(\frac{\partial (\frac{n R T}{P})}{\partial T}\right)_p = \frac{nRT}{PV} = 1</math></ref> so that |
|||
The number of hydrogen bonds formed by a molecule of liquid water fluctuates with time and temperature.<ref name="Jorgensen1985"/> From [[water model|TIP4P]] liquid water simulations at 25 °C, it was estimated that each water molecule participates in an average of 3.59 hydrogen bonds. At 100 °C, this number decreases to 3.24 due to the increased molecular motion and decreased density, while at 0 °C, the average number of hydrogen bonds increases to 3.69.<ref name="Jorgensen1985">{{cite journal|author1=Jorgensen, W. L. |author2=Madura, J. D. | title=Temperature and size dependence for Monte Carlo simulations of TIP4P water| journal=[[Mol. Phys.]]|year=1985| volume=56 |issue=6 |pages=1381 |doi=10.1080/00268978500103111|bibcode = 1985MolPh..56.1381J }}</ref> Another study found a much smaller number of hydrogen bonds: 2.357 at 25 °C.<ref>{{cite journal|author=Zielkiewicz, Jan |title= Structural properties of water: Comparison of the SPC, SPCE, TIP4P, and TIP5P models of water| journal=[[J. Chem. Phys.]]|volume= 123|pages=104501|year=2005| doi=10.1063/1.2018637|pmid=16178604|issue=10|bibcode = 2005JChPh.123j4501Z }}</ref> The differences may be due to the use of a different method for defining and counting the hydrogen bonds. |
|||
:<math>dH = C_p\,dT.</math> |
|||
Where the bond strengths are more equivalent, one might instead find the atoms of two interacting water molecules partitioned into two [[polyatomic ion]]s of opposite charge, specifically [[hydroxide]] (OH<sup>−</sup>) and [[hydronium]] (H<sub>3</sub>O<sup>+</sup>). (Hydronium ions are also known as "hydroxonium" ions.) |
|||
In a more general form, the first law describes the internal energy with additional terms involving the [[chemical potential]] and the number of particles of various types. The differential statement for {{mvar|dH}} then becomes |
|||
:H−O<sup>−</sup> H<sub>3</sub>O<sup>+</sup> |
|||
:<math>dH = T\,dS + V\,dp + \sum_i \mu_i\,dN_i,</math> |
|||
Indeed, in pure water under conditions of [[standard temperature and pressure]], this latter formulation is applicable only rarely; on average about one in every 5.5 × 10<sup>8</sup> molecules gives up a proton to another water molecule, in accordance with the value of the [[dissociation constant]] for water under such conditions. It is a crucial part of the uniqueness of water. |
|||
where {{math|''μ''<sub>''i''</sub>}} is the chemical potential per particle for an {{mvar|i}}-type particle, and {{math|''N''<sub>''i''</sub>}} is the number of such particles. The last term can also be written as {{math|''μ<sub>i</sub>'' ''dn''<sub>''i''</sub>}} (with {{math|''dn''<sub>''i''</sub>}} the number of moles of component {{mvar|i}} added to the system and, in this case, {{math|''μ''<sub>''i''</sub>}} the molar chemical potential) or as {{math|''μ''<sub>''i''</sub> ''dm''<sub>''i''</sub>}} (with {{math|''dm''<sub>''i''</sub>}} the mass of component {{mvar|i}} added to the system and, in this case, {{math|''μ''<sub>''i''</sub>}} the specific chemical potential). |
|||
Because water may form hydrogen bonds with solute proton donors and acceptors, it may competitively inhibit the formation of solute intermolecular or intramolecular hydrogen bonds. Consequently, hydrogen bonds between or within solute molecules dissolved in water are almost always unfavorable relative to hydrogen bonds between water and the donors and acceptors for hydrogen bonds on those solutes.<ref>{{cite journal|author=Jencks, William |title= Hydrogen Bonding between Solutes in Aqueous Solution| journal=[[J. Am. Chem. Soc.]]|volume= 108|pages=4196|year=1986|issue=14|doi=10.1021/ja00274a058|last2=Jencks|first2=William P.}}</ref> Hydrogen bonds between water molecules have an average lifetime of 10<sup>−11</sup> seconds, or 10 picoseconds.<ref name="Dillon">{{cite book|last=Dillon|first=P. F.|title=Biophysics: A Physiological Approach|url=https://books.google.com/books?id=5IYKLIx-Jt4C|year=2012|publisher=Cambridge University Press|isbn=978-1-139-50462-1|page=37}}</ref> |
|||
===Characteristic functions=== |
|||
=== Bifurcated and over-coordinated hydrogen bonds in water === |
|||
The enthalpy, {{math|''H''(''S''[''p''], ''p'', {{mset|''N<sub>i</sub>''}})}}, expresses the thermodynamics of a system in the ''energy representation''. As a [[State function|function of state]], its arguments include both one intensive and several extensive [[state variable]]s. The state variables {{math|''S''[''p'']}}, {{math|''p''}}, and {{math|{{mset|''N<sub>i</sub>''}}}} are said to be the ''natural state variables'' in this representation. They are suitable for describing processes in which they are determined by factors in the surroundings. For example, when a virtual parcel of atmospheric air moves to a different altitude, the pressure surrounding it changes, and the process is often so rapid that there is too little time for heat transfer. This is the basis of the so-called [[adiabatic approximation]] that is used in [[meteorology]].<ref>Iribarne, J.V., Godson, W.L. (1981). ''Atmospheric Thermodynamics'', 2nd edition, Kluwer Academic Publishers, Dordrecht, {{ISBN|90-277-1297-2}}, pp. 235–236.</ref> |
|||
A single hydrogen atom can participate in two hydrogen bonds, rather than one. This type of bonding is called "bifurcated" (split in two or "two-forked"). It can exist, for instance, in complex natural or synthetic organic molecules.<ref>{{cite journal|doi=10.1139/v84-087|journal=Can. J. Chem.|volume= 62|issue= 3|pages= 526–530|title=Hétérocycles à fonction quinone. V. Réaction anormale de la butanedione avec la diamino-1,2 anthraquinone; structure cristalline de la naphto \2,3-f] quinoxalinedione-7,12 obtenue|year=1984|last1=Baron|first1=Michel|last2=Giorgi-Renault|first2=Sylviane|last3=Renault|first3=Jean|last4=Mailliet|first4=Patrick|last5=Carré|first5=Daniel|last6=Etienne|first6=Jean}}</ref> It has been suggested that a bifurcated hydrogen atom is an essential step in water reorientation.<ref>{{cite journal|title = A Molecular Jump Mechanism for Water Reorientation|author1=Laage, Damien |author2=Hynes, James T.|journal = [[Science (journal)|Science]]|year = 2006|volume = 311|pages = 832–5|doi = 10.1126/science.1122154|pmid = 16439623|issue = 5762|bibcode = 2006Sci...311..832L |s2cid=6707413 }}</ref><br /> |
|||
Acceptor-type hydrogen bonds (terminating on an oxygen's lone pairs) are more likely to form bifurcation (it is called overcoordinated oxygen, OCO) than are donor-type hydrogen bonds, beginning on the same oxygen's hydrogens.<ref>{{cite journal|title = The Distribution of Acceptor and Donor Hydrogen-Bonds in Bulk Liquid Water|author1=Markovitch, Omer |author2=Agmon, Noam |journal = Molecular Physics|year = 2008|volume = 106|issue = 2|pages = 485|doi = 10.1080/00268970701877921|bibcode = 2008MolPh.106..485M |s2cid=17648714 }}</ref> |
|||
===Other liquids=== |
|||
Conjugate with the enthalpy, with these arguments, the other characteristic function of state of a thermodynamic system is its entropy, as a function, {{math|''S''[''p''](''H'', ''p'', {{mset|''N<sub>i</sub>''}})}}, of the same list of variables of state, except that the entropy, {{math|''S''[''p'']}}, is replaced in the list by the enthalpy, {{math|''H''}}. It expresses the ''entropy representation''. The state variables {{math|''H''}}, {{math|''p''}}, and {{math|{{mset|''N<sub>i</sub>''}}}} are said to be the ''natural state variables'' in this representation. They are suitable for describing processes in which they are experimentally controlled. For example, {{math|''H''}} and {{math|''p''}} can be controlled by allowing heat transfer, and by varying only the external pressure on the piston that sets the volume of the system.<ref>Tschoegl, N.W. (2000). ''Fundamentals of Equilibrium and Steady-State Thermodynamics'', Elsevier, Amsterdam, {{ISBN|0-444-50426-5}}, p. 17.</ref><ref>Callen, H. B. (1960/1985), ''Thermodynamics and an Introduction to Thermostatistics'', (first edition 1960), second edition 1985, John Wiley & Sons, New York, {{ISBN|0-471-86256-8}}, Chapter 5.</ref><ref>Münster, A. (1970), Classical Thermodynamics, translated by E. S. Halberstadt, Wiley–Interscience, London, {{ISBN|0-471-62430-6}}, p. 6.</ref> |
|||
For example, [[hydrogen fluoride]]—which has three lone pairs on the F atom but only one H atom—can form only two bonds; ([[ammonia]] has the opposite problem: three hydrogen atoms but only one lone pair). |
|||
:H−F···H−F···H−F |
|||
==Physical interpretation== |
|||
The {{mvar|U}} term can be interpreted as the energy required to create the system, and the {{mvar|pV}} term as the [[work (thermodynamics)|work]] that would be required to "make room" for the system if the pressure of the environment remained constant. When a system, for example, {{mvar|n}} [[Mole (unit)|moles]] of a gas of [[Volume (thermodynamics)|volume]] {{mvar|V}} at [[pressure]] {{mvar|p}} and [[temperature]] {{mvar|T}}, is created or brought to its present state from [[absolute zero]], energy must be supplied equal to its internal energy {{mvar|U}} plus {{mvar|pV}}, where {{mvar|pV}} is the [[Work (physics)|work]] done in pushing against the ambient (atmospheric) pressure. |
|||
===Further manifestations of solvent hydrogen bonding=== |
|||
In basic [[physics]] and [[statistical mechanics]] it may be more interesting to study the internal properties of the system and therefore the internal energy is used.<ref>{{cite book|first=F. |last=Reif |title=Statistical Physics |publisher=McGraw-Hill |location=London |date=1967}}</ref><ref>{{cite book|first1=C. |last1=Kittel |first2=H. |last2=Kroemer |title=Thermal Physics |publisher=Freeman |location=London |date=1980}}</ref> In basic [[chemistry]], experiments are often conducted at constant [[atmospheric pressure]], and the pressure–volume work represents an energy exchange with the atmosphere that cannot be accessed or controlled, so that {{math|Δ''H''}} is the expression chosen for the [[heat of reaction]]. |
|||
* Increase in the [[melting point]], [[boiling point]], [[solubility]], and viscosity of many compounds can be explained by the concept of hydrogen bonding. |
|||
* Negative [[Azeotrope|azeotropy]] of mixtures of HF and water |
|||
* The fact that ice is less dense than liquid water is due to a crystal structure stabilized by hydrogen bonds. |
|||
* Dramatically higher boiling points of NH<sub>3</sub>, H<sub>2</sub>O, and HF compared to the heavier analogues PH<sub>3</sub>, H<sub>2</sub>S, and HCl, where hydrogen-bonding is absent. |
|||
* Viscosity of anhydrous [[phosphoric acid]] and of [[glycerol]] |
|||
* Dimer formation in [[carboxylic acid]]s and hexamer formation in [[hydrogen fluoride]], which occur even in the gas phase, resulting in gross deviations from the [[ideal gas law]]. |
|||
* Pentamer formation of water and alcohols in apolar solvents. |
|||
==Hydrogen bonds in polymers== |
|||
For a [[heat engine]] a change in its internal energy is the difference between the heat input and the [[Work (thermodynamics)#Pressure–volume work|pressure–volume work]] done by the working substance while a change in its enthalpy is the difference between the heat input and the work done by the engine:<ref>{{Cite book|title=Theoretische Physik|last=Bartelmann|first=Matthias|publisher=Springer Spektrum|year=2015|isbn=978-3-642-54617-4|pages=1106–1108}}</ref> |
|||
Hydrogen bonding plays an important role in determining the three-dimensional structures and the properties adopted by many synthetic and natural proteins. Compared to the C-C, C-O, and C-N bonds that comprise most polymers, hydrogen bonds are far weaker, perhaps 5%. Thus, hydrogen bonds can be broken by chemical or mechanical means while retaining the basic structure of the polymer backbone. This hierarchy of bond strengths (covalent bonds being stronger than hydrogen-bonds being stronger than van der Waals forces) is key to understanding the properties of many materials.<ref>{{cite book|title=Hydrogen Bonding in Polymer Materials|author=Shiao-Wei Kuo|year=2018|publisher=Wiley-VCH }}</ref> |
|||
:<math>dH=\delta Q-\delta W</math> |
|||
where the work {{mvar|W}} done by the engine is: |
|||
:<math>W=-\oint pdV</math> |
|||
===DNA=== |
|||
== Relationship to heat == |
|||
[[File:DNA animation.gif|thumb|The structure of part of a DNA [[double helix]]]] |
|||
In order to discuss the relation between the enthalpy increase and heat supply, we return to the first law for closed systems, with the physics sign convention: {{math|''dU'' {{=}} ''δQ'' − ''δW''}}, where the heat {{mvar|δQ}} is supplied by conduction, radiation, and [[Joule heating]]. We apply it to the special case with a constant pressure at the surface. In this case the work term can be split into two contributions, the so-called {{mvar|pV}} work, given by {{math|''p dV''}} (where here {{mvar|p}} is the pressure at the surface, {{mvar|dV}} is the increase of the volume of the system), and the so-called isochoric mechanical work {{math|''δW''′}}, such as stirring by a shaft with paddles or by an externally driven magnetic field acting on an internal rotor. Cases of long range electromagnetic interaction require further state variables in their formulation, and are not considered here. So we write {{math|''δW'' {{=}} ''p dV'' + ''δW''′}}. In this case the first law reads: |
|||
[[File:Base pair GC.svg|thumb|Hydrogen bonding between [[guanine]] and [[cytosine]], one of two types of [[base pair]]s in DNA]] |
|||
In these macromolecules, bonding between parts of the same macromolecule cause it to fold into a specific shape, which helps determine the molecule's physiological or biochemical role. For example, the double helical structure of [[DNA]] is due largely to hydrogen bonding between its [[base pair]]s (as well as [[pi stacking]] interactions), which link one complementary strand to the other and enable [[DNA replication|replication]]. |
|||
:<math>dU = \delta Q - p\,dV - \delta W'.</math> |
|||
===Proteins=== |
|||
Now, |
|||
In the [[Protein secondary structure|secondary structure of proteins]], hydrogen bonds form between the backbone oxygens and [[amide]] hydrogens. When the spacing of the [[amino acid]] residues participating in a hydrogen bond occurs regularly between positions ''i'' and ''i'' + 4, an [[alpha helix]] is formed. When the spacing is less, between positions ''i'' and ''i'' + 3, then a [[3 10 helix|3<sub>10</sub> helix]] is formed. When two strands are joined by hydrogen bonds involving alternating residues on each participating strand, a [[beta sheet]] is formed. Hydrogen bonds also play a part in forming the tertiary structure of protein through interaction of R-groups. (See also [[protein folding]]). |
|||
[[Bifurcated H-bond]] systems are common in alpha-helical [[transmembrane proteins]] between the backbone amide C=O of residue ''i'' as the H-bond acceptor and two H-bond donors from residue ''i+4'': the backbone amide N-H and a side-chain hydroxyl or thiol H<sup>+</sup>. The energy preference of the bifurcated H-bond hydroxyl or thiol system is -3.4 kcal/mol or -2.6 kcal/mol, respectively. This type of bifurcated H-bond provides an intrahelical H-bonding partner for polar side-chains, such as [[serine]], [[threonine]], and [[cysteine]] within the hydrophobic membrane environments.<ref name="Feldblum 2014 4085–4090"/> |
|||
:<math>dH = dU + d(pV).</math> |
|||
The role of hydrogen bonds in protein folding has also been linked to osmolyte-induced protein stabilization. Protective osmolytes, such as [[trehalose]] and [[sorbitol]], shift the protein folding equilibrium toward the folded state, in a concentration dependent manner. While the prevalent explanation for osmolyte action relies on excluded volume effects that are entropic in nature, [[circular dichroism]] (CD) experiments have shown osmolyte to act through an enthalpic effect.<ref>{{cite journal|last=Politi|first=Regina|author2=Harries, Daniel|title=Enthalpically driven peptide stabilization by protective osmolytes|journal=ChemComm|year=2010|volume=46|pages=6449–6451|doi=10.1039/C0CC01763A|pmid=20657920|issue=35}}</ref> The molecular mechanism for their role in protein stabilization is still not well established, though several mechanisms have been proposed. Computer [[molecular dynamics]] simulations suggest that osmolytes stabilize proteins by modifying the hydrogen bonds in the protein hydration layer.<ref>{{cite journal|last=Gilman-Politi|first=Regina|author2=Harries, Daniel|title=Unraveling the Molecular Mechanism of Enthalpy Driven Peptide Folding by Polyol Osmolytes|journal=Journal of Chemical Theory and Computation|year=2011|volume=7|issue=11|pages=3816–3828|doi=10.1021/ct200455n|pmid=26598272}}</ref> |
|||
So |
|||
Several studies have shown that hydrogen bonds play an important role for the stability between subunits in multimeric proteins. For example, a study of sorbitol dehydrogenase displayed an important hydrogen bonding network which stabilizes the tetrameric quaternary structure within the mammalian sorbitol dehydrogenase protein family.<ref>{{cite journal|last=Hellgren|first=M.|author2=Kaiser, C.|author3=de Haij, S.|author4=Norberg, A.|author5=Höög, J. O.|title=A hydrogen-bonding network in mammalian sorbitol dehydrogenase stabilizes the tetrameric state and is essential for the catalytic power|journal=Cellular and Molecular Life Sciences |date=December 2007|volume=64|issue=23|pages=3129–38|pmid=17952367|doi=10.1007/s00018-007-7318-1|s2cid=22090973}}</ref> |
|||
:<math>dH = \delta Q + V\,dp + p \,dV - p\,dV - \delta W'</math> |
|||
::<math>\,\,= \delta Q + V\,dp - \delta W'.</math> |
|||
A protein backbone hydrogen bond incompletely shielded from water attack is a [[dehydron]]. Dehydrons promote the removal of water through proteins or [[ligand|ligand binding]]. The exogenous dehydration enhances the [[electrostatic]] interaction between the [[amide]] and [[carbonyl]] groups by de-shielding their [[charge (physics)|partial charges]]. Furthermore, the dehydration stabilizes the hydrogen bond by destabilizing the [[nonbonded interactions|nonbonded state]] consisting of dehydrated [[charge (physics)|isolated charges]].<ref>{{cite journal|last=Fernández|first=A.|author2=Rogale K.|author3=Scott Ridgway|author4=Scheraga H. A.|title=Inhibitor design by wrapping packing defects in HIV-1 proteins|journal= Proceedings of the National Academy of Sciences|date=June 2004|volume=101|issue=32|pages=11640–5|pmid=15289598|doi=10.1073/pnas.0404641101|pmc=511032|bibcode=2004PNAS..10111640F|doi-access=free}}</ref> |
|||
With sign convention of physics, {{math|''δW''' < 0}}, because [[Isochoric process|isochoric]] shaft work done by an external device on the system adds energy to the system, and may be viewed as virtually adding heat. The only thermodynamic mechanical work done by the system is expansion work, {{math|''p dV''}}.<ref>{{cite book|last1=Ebbing|first1=Darrel|last2=Gammon|first2=Steven|title=General Chemistry|publisher=Cengage Learning|year=2010|isbn=978-0-538-49752-7|page=231|url=https://books.google.com/books?id=8_7iGj4QLR4C&pg=PA231|url-status=live|archive-url=https://web.archive.org/web/20131114033110/http://books.google.com/books?id=8_7iGj4QLR4C&pg=PA231|archive-date=2013-11-14}}</ref> |
|||
[[Wool]], being a protein fibre, is held together by hydrogen bonds, causing wool to recoil when stretched. However, washing at high temperatures can permanently break the hydrogen bonds and a garment may permanently lose its shape. |
|||
If the system is under [[isobaric system|constant pressure]] ({{math|''dp'' {{=}} 0}}). Consequently, the increase in enthalpy of the system is equal to the added [[heat]] and virtual heat: |
|||
===Cellulose=== |
|||
:<math>dH = \delta Q - \delta W'.</math> |
|||
Hydrogen bonds are important in the structure of [[cellulose]] and derived polymers in its many different forms in nature, such as [[cotton]] and [[flax]]. |
|||
[[Image:Kevlar chemical structure.png|thumb|400px|Para-aramid structure]] |
|||
This is why the now-obsolete term ''heat content'' was used in the 19th century. |
|||
[[Image:Cellulose strand.svg|thumb|right|260px|A strand of cellulose (conformation I<sub>α</sub>), showing the hydrogen bonds (dashed) within and between cellulose molecules]] |
|||
==Applications== |
|||
In thermodynamics, one can calculate enthalpy by determining the requirements for creating a system from "nothingness"; the mechanical work required, {{mvar|pV}}, differs based upon the conditions that obtain during the creation of the [[thermodynamic system]]. |
|||
[[Energy]] must be supplied to remove particles from the surroundings to make space for the creation of the system, assuming that the pressure {{mvar|p}} remains constant; this is the {{mvar|pV}} term. The supplied energy must also provide the change in internal energy, {{mvar|U}}, which includes [[activation energy|activation energies]], ionization energies, mixing energies, vaporization energies, chemical bond energies, and so forth. Together, these constitute the change in the enthalpy {{math|''U'' + ''pV''}}. For systems at constant pressure, with no external work done other than the {{mvar|pV}} work, the change in enthalpy is the heat received by the system. |
|||
For a simple system, with a constant number of particles, the difference in enthalpy is the maximum amount of thermal energy derivable from a thermodynamic process in which the pressure is held constant.<ref>{{cite book|last1=Rathakrishnan|title=High Enthalpy Gas Dynamics|publisher=John Wiley and Sons Singapore Pte. Ltd.|isbn=978-1118821893|date=2015}}</ref> |
|||
===Heat of reaction=== |
|||
{{Main|Standard enthalpy of reaction}} |
|||
The total enthalpy of a system cannot be measured directly; the ''enthalpy change'' of a [[system (thermodynamics)|system]] is measured instead. Enthalpy change is defined by the following equation: |
|||
:<math>\Delta H = H_\mathrm{f} - H_\mathrm{i},</math> |
|||
where |
|||
:{{math|Δ''H''}} is the "enthalpy change", |
|||
:{{math|''H''<sub>f</sub>}} is the final enthalpy of the system (in a chemical reaction, the enthalpy of the products), |
|||
:{{math|''H''<sub>i</sub>}} is the initial enthalpy of the system (in a chemical reaction, the enthalpy of the reactants). |
|||
For an [[exothermic reaction]] at constant [[pressure]], the system's change in enthalpy equals the energy released in the reaction, including the energy retained in the system and lost through expansion against its surroundings. In a similar manner, for an [[endothermic]] reaction, the system's change in enthalpy is equal to the energy ''absorbed'' in the reaction, including the energy ''lost by'' the system and ''gained'' from compression from its surroundings. If {{math|Δ''H''}} is positive, the reaction is endothermic, that is heat is absorbed by the system due to the products of the reaction having a greater enthalpy than the reactants. On the other hand, if {{math|Δ''H''}} is negative, the reaction is exothermic, that is the overall decrease in enthalpy is achieved by the generation of heat.<ref>{{cite book|first=Keith J. |last=Laidler |first2=John H.|last2=Meiser|date=1982 |title=Physical Chemistry |page=53 |isbn=978-0-8053-5682-3 |publisher=Benjamin/Cummings}}</ref> |
|||
From the definition of enthalpy as {{math|''H'' {{=}} ''U'' + ''pV''}}, the enthalpy change at constant pressure {{math|Δ''H'' {{=}} Δ''U'' + ''p'' Δ''V''}}. However for most chemical reactions, the work term {{math|''p'' Δ''V''}} is much smaller than the internal energy change {{math|Δ''U''}} which is approximately equal to {{math|Δ''H''}}. As an example, for the combustion of carbon monoxide 2 CO(g) + O<sub>2</sub>(g) → 2 CO<sub>2</sub>(g), {{math|Δ''H''}} = −566.0 kJ and {{math|Δ''U''}} = −563.5 kJ.<ref>{{cite book|first=Ralph H. |last=Petrucci |first2=William S.|last2=Harwood |first3=F. Geoffrey|last3=Herring |date=2002 |title=General Chemistry |url=https://archive.org/details/generalchemistry00hill |url-access=registration |edition=8th |pages=[https://archive.org/details/generalchemistry00hill/page/237 237–238] |isbn=978-0-13-014329-7|publisher=Prentice Hall}}</ref> Since the differences are so small, reaction enthalpies are often loosely described as reaction energies and analyzed in terms of [[bond energy|bond energies]]. |
|||
===Specific enthalpy=== |
|||
The specific enthalpy of a uniform system is defined as {{math|''h'' {{=}} {{sfrac|''H''|''m''}}}} where {{mvar|m}} is the mass of the system. The [[SI unit]] for specific enthalpy is joule per kilogram. It can be expressed in other specific quantities by {{math|''h'' {{=}} ''u'' + ''pv''}}, where {{mvar|u}} is the specific [[internal energy]], {{mvar|p}} is the pressure, and {{mvar|v}} is [[specific volume]], which is equal to {{math|{{sfrac|1|''ρ''}}}}, where {{mvar|ρ}} is the [[density]]. |
|||
===Enthalpy changes=== |
|||
An enthalpy change describes the change in enthalpy observed in the constituents of a thermodynamic system when undergoing a transformation or chemical reaction. It is the difference between the enthalpy after the process has completed, i.e. the enthalpy of the [[Product (chemistry)|products]], and the initial enthalpy of the system, namely the reactants. These processes are specified solely by their initial and final states, so that the enthalpy change for the reverse is the negative of that for the forward process. |
|||
A common standard enthalpy change is the [[enthalpy of formation]], which has been determined for a large number of substances. Enthalpy changes are routinely measured and compiled in chemical and physical reference works, such as the [[CRC Handbook of Chemistry and Physics]]. The following is a selection of enthalpy changes commonly recognized in thermodynamics. |
|||
When used in these recognized terms the qualifier ''change'' is usually dropped and the property is simply termed ''enthalpy of 'process'''. Since these properties are often used as reference values it is very common to quote them for a standardized set of environmental parameters, or [[standard conditions]], including: |
|||
* A [[temperature]] of 25 °C or 298.15 K, |
|||
* A [[pressure]] of one atmosphere (1 atm or 101.325 kPa), |
|||
* A [[concentration]] of 1.0 M when the element or compound is present in solution, |
|||
* Elements or compounds in their normal physical states, i.e. [[standard state]]. |
|||
For such standardized values the name of the enthalpy is commonly prefixed with the term ''standard'', e.g. ''standard enthalpy of formation''. |
|||
Chemical properties: |
|||
* [[Enthalpy of reaction]], defined as the enthalpy change observed in a constituent of a thermodynamic system when one mole of substance reacts completely. |
|||
* [[Enthalpy of formation]], defined as the enthalpy change observed in a constituent of a thermodynamic system when one mole of a compound is formed from its elementary antecedents. |
|||
* [[Enthalpy of combustion]], defined as the enthalpy change observed in a constituent of a thermodynamic system when one mole of a substance burns completely with oxygen. |
|||
* [[Enthalpy of hydrogenation]], defined as the enthalpy change observed in a constituent of a thermodynamic system when one mole of an unsaturated compound reacts completely with an excess of hydrogen to form a saturated compound. |
|||
* [[Enthalpy of atomization]], defined as the enthalpy change required to separate one mole of a substance into its constituent [[atom]]s completely. |
|||
* [[Enthalpy of neutralization]], defined as the enthalpy change observed in a constituent of a thermodynamic system when one mole of water is formed when an acid and a base react. |
|||
* Standard [[Enthalpy of solution]], defined as the enthalpy change observed in a constituent of a thermodynamic system when one mole of a solute is dissolved completely in an excess of solvent, so that the solution is at infinite dilution. |
|||
* Standard enthalpy of [[Denaturation (biochemistry)]], defined as the enthalpy change required to denature one mole of compound. |
|||
* [[Hydration energy|Enthalpy of hydration]], defined as the enthalpy change observed when one mole of gaseous ions are completely dissolved in water forming one mole of aqueous ions. |
|||
Physical properties: |
|||
* [[Enthalpy of fusion]], defined as the enthalpy change required to completely change the state of one mole of substance between solid and liquid states. |
|||
* [[Enthalpy of vaporization]], defined as the enthalpy change required to completely change the state of one mole of substance between liquid and gaseous states. |
|||
* [[Enthalpy of sublimation]], defined as the enthalpy change required to completely change the state of one mole of substance between solid and gaseous states. |
|||
* [[Lattice enthalpy]], defined as the energy required to separate one mole of an ionic compound into separated gaseous ions to an infinite distance apart (meaning no force of attraction). |
|||
* [[Enthalpy of mixing]], defined as the enthalpy change upon mixing of two (non-reacting) chemical substances. |
|||
===Open systems=== |
|||
In [[thermodynamic]] [[Open system (systems theory)|open systems]], mass (of substances) may flow in and out of the system boundaries. The first law of thermodynamics for open systems states: The increase in the internal energy of a system is equal to the amount of energy added to the system by mass flowing in and by heating, minus the amount lost by mass flowing out and in the form of work done by the system: |
|||
:<math>dU = \delta Q + dU_\text{in} - dU_\text{out} - \delta W,</math> |
|||
where {{math|''U''<sub>in</sub>}} is the average internal energy entering the system, and {{math|''U''<sub>out</sub>}} is the average internal energy leaving the system. |
|||
[[Image:First law open system.svg|250px|thumb|right|During [[Steady-state (chemical engineering)|steady, continuous]] operation, an energy balance applied to an open system equates shaft work performed by the system to heat added plus net enthalpy added]] |
|||
The region of space enclosed by the boundaries of the open system is usually called a [[control volume]], and it may or may not correspond to physical walls. If we choose the shape of the control volume such that all flow in or out occurs perpendicular to its surface, then the flow of mass into the system performs work as if it were a piston of fluid pushing mass into the system, and the system performs work on the flow of mass out as if it were driving a piston of fluid. There are then two types of work performed: ''flow work'' described above, which is performed on the fluid (this is also often called ''{{mvar|pV}} work''), and ''shaft work'', which may be performed on some mechanical device. |
|||
These two types of work are expressed in the equation |
|||
: <math>\delta W = d(p_\text{out}V_\text{out}) - d(p_\text{in}V_\text{in}) + \delta W_\text{shaft}.</math> |
|||
Substitution into the equation above for the control volume (cv) yields: |
|||
: <math>dU_\text{cv} = \delta Q + dU_\text{in} + d(p_\text{in}V_\text{in}) - dU_\text{out} - d(p_\text{out}V_\text{out}) - \delta W_\text{shaft}.</math> |
|||
The definition of enthalpy, {{mvar|H}}, permits us to use this [[thermodynamic potential]] to account for both internal energy and {{mvar|pV}} work in fluids for open systems: |
|||
: <math>dU_\text{cv} = \delta Q + dH_\text{in} - dH_\text{out} - \delta W_\text{shaft}.</math> |
|||
If we allow also the system boundary to move (e.g. due to moving pistons), we get a rather general form of the first law for open systems.<ref>{{cite book|first1=M. J. |last1=Moran |first2=H. N. |last2=Shapiro |title=Fundamentals of Engineering Thermodynamics |url=https://archive.org/details/fundamentalsengi00mora_077 |url-access=limited |edition=5th |date=2006 |publisher=John Wiley & Sons|page=[https://archive.org/details/fundamentalsengi00mora_077/page/n141 129]}}</ref> In terms of time derivatives it reads: |
|||
: <math>\frac{dU}{dt} = \sum_k \dot Q_k + \sum_k \dot H_k - \sum_k p_k\frac{dV_k}{dt} - P,</math> |
|||
with sums over the various places {{mvar|k}} where heat is supplied, mass flows into the system, and boundaries are moving. The {{mvar|Ḣ<sub>k</sub>}} terms represent enthalpy flows, which can be written as |
|||
:<math>\dot H_k = h_k\dot m_k = H_\mathrm{m}\dot n_k,</math> |
|||
with {{mvar|ṁ<sub>k</sub>}} the mass flow and {{mvar|ṅ<sub>k</sub>}} the molar flow at position {{mvar|k}} respectively. The term {{math|{{sfrac|''dV''<sub>''k''</sub>|''dt''}}}} represents the rate of change of the system volume at position {{mvar|k}} that results in {{mvar|pV}} power done by the system. The parameter {{math|''P''}} represents all other forms of power done by the system such as shaft power, but it can also be, say, electric power produced by an electrical power plant. |
|||
Note that the previous expression holds true only if the kinetic energy flow rate is conserved between system inlet and outlet.{{clarify|reason=This new type of energy, kinetic energy, was not mentioned before. Is it part of U? Does it need to be conserved, or just the net flow across boundary be zero?|date=March 2015}} Otherwise, it has to be included in the enthalpy balance. During [[Steady-state (chemical engineering)|steady-state]] operation of a device (''see [[turbine]], [[pump]], and [[engine]]''), the average {{math|{{sfrac|''dU''|''dt''}}}} may be set equal to zero. This yields a useful expression for the average [[Power (physics)|power]] generation for these devices in the absence of chemical reactions: |
|||
:<math> P = \sum_k \left\langle \dot Q_k \right\rangle |
|||
+ \sum_k \left\langle \dot H_k \right\rangle |
|||
- \sum_k \left\langle p_k\frac{dV_k}{dt} \right\rangle,</math> |
|||
where the [[angle bracket]]s denote time averages. The technical importance of the enthalpy is directly related to its presence in the first law for open systems, as formulated above. |
|||
==Diagrams== |
|||
[[File:Ts diagram of N2 02.jpg|500px|thumb|right|{{math|''T''–''s''}} diagram of nitrogen.<ref>Figure composed with data obtained with [[RefProp]], NIST Standard Reference Database 23.</ref> The red curve at the left is the melting curve. The red dome represents the two-phase region with the low-entropy side the saturated liquid and the high-entropy side the saturated gas. The black curves give the {{math|''T''–''s''}} relation along isobars. The pressures are indicated in bar. The blue curves are isenthalps (curves of constant enthalpy). The values are indicated in blue in kJ/kg. The specific points '''a''', '''b''', etc., are treated in the main text.]] |
|||
The enthalpy values of important substances can be obtained using commercial software. Practically all relevant material properties can be obtained either in tabular or in graphical form. There are many types of diagrams, such as {{math|''h''–''T''}} diagrams, which give the specific enthalpy as function of temperature for various pressures, and {{math|''h''–''p''}} diagrams, which give {{mvar|h}} as function of {{mvar|p}} for various {{mvar|T}}. One of the most common diagrams is the temperature–specific entropy diagram ({{math|''T''–''s''}} diagram). It gives the melting curve and saturated liquid and vapor values together with isobars and isenthalps. These diagrams are powerful tools in the hands of the thermal engineer. |
|||
===Some basic applications=== |
|||
The points '''a''' through '''h''' in the figure play a role in the discussion in this section. |
|||
:{|class="wikitable" style="text-align:center" |
|||
!Point!!{{mvar|T}} (K)!!{{mvar|p}} (bar)!!{{mvar|s}} ({{nowrap|kJ/(kg K)}})!!{{mvar|h}} (kJ/kg) |
|||
|- |
|||
|'''a'''||300||1||6.85||461 |
|||
|- |
|||
|'''b'''||380||2||6.85||530 |
|||
|- |
|||
|'''c'''||300||200||5.16||430 |
|||
|- |
|||
|'''d'''||270||1||6.79||430 |
|||
|- |
|||
|'''e'''||108||13||3.55||100 |
|||
|- |
|||
|'''f'''||77.2||1||3.75||100 |
|||
|- |
|||
|'''g'''||77.2||1||2.83||28 |
|||
|- |
|||
|'''h'''||77.2||1||5.41||230 |
|||
|} |
|||
Points '''e''' and '''g''' are saturated liquids, and point '''h''' is a saturated gas. |
|||
===Throttling=== |
|||
{{main|Joule–Thomson effect}} |
|||
[[File:Schematic of throttling.png|thumb|right|Schematic diagram of a throttling in the steady state. Fluid enters the system (dotted rectangle) at point 1 and leaves it at point 2. The mass flow is {{mvar|ṁ}}.]] |
|||
One of the simple applications of the concept of enthalpy is the so-called throttling process, also known as [[Joule–Thomson effect|Joule–Thomson expansion]]. It concerns a steady adiabatic flow of a fluid through a flow resistance (valve, porous plug, or any other type of flow resistance) as shown in the figure. This process is very important, since it is at the heart of domestic [[refrigerator]]s, where it is responsible for the temperature drop between ambient temperature and the interior of the refrigerator. It is also the final stage in many types of [[liquefier]]s. |
|||
For a steady state flow regime, the enthalpy of the system (dotted rectangle) has to be constant. Hence |
|||
:<math>0 = \dot m h_1 - \dot m h_2.</math> |
|||
Since the mass flow is constant, the specific enthalpies at the two sides of the flow resistance are the same: |
|||
:<math>h_1 = h_2,</math> |
|||
that is, the enthalpy per unit mass does not change during the throttling. The consequences of this relation can be demonstrated using the {{math|''T''–''s''}} diagram above. Point '''c''' is at 200 bar and room temperature (300 K). A Joule–Thomson expansion from 200 bar to 1 bar follows a curve of constant enthalpy of roughly 425 kJ/kg (not shown in the diagram) lying between the 400 and 450 kJ/kg isenthalps and ends in point '''d''', which is at a temperature of about 270 K. Hence the expansion from 200 bar to 1 bar cools nitrogen from 300 K to 270 K. In the valve, there is a lot of friction, and a lot of entropy is produced, but still the final temperature is below the starting value. |
|||
Point '''e''' is chosen so that it is on the saturated liquid line with {{math|''h''}} = 100 kJ/kg. It corresponds roughly with {{math|''p''}} = 13 bar and {{math|''T''}} = 108 K. Throttling from this point to a pressure of 1 bar ends in the two-phase region (point '''f'''). This means that a mixture of gas and liquid leaves the throttling valve. Since the enthalpy is an extensive parameter, the enthalpy in '''f''' ({{math|''h''<sub>'''f'''</sub>}}) is equal to the enthalpy in '''g''' ({{math|''h''<sub>'''g'''</sub>}}) multiplied by the liquid fraction in '''f''' ({{math|''x''<sub>'''f'''</sub>}}) plus the enthalpy in '''h''' ({{math|''h''<sub>'''h'''</sub>}}) multiplied by the gas fraction in '''f''' {{math|(1 − ''x''<sub>'''f'''</sub>)}}. So |
|||
:<math> h_\mathbf{f} = x_\mathbf{f} h_\mathbf{g} + (1 - x_\mathbf{f})h_\mathbf{h}.</math> |
|||
With numbers: {{math|100 {{=}} ''x''<sub>'''f'''</sub> × 28 + (1 − ''x''<sub>'''f'''</sub>) × 230}}, so {{math|''x''<sub>'''f'''</sub>}} = 0.64. This means that the mass fraction of the liquid in the liquid–gas mixture that leaves the throttling valve is 64%. |
|||
===Compressors=== |
|||
{{main|Gas compressor}} |
|||
[[File:Schematic of compressor.png|thumb|right|Schematic diagram of a compressor in the steady state. Fluid enters the system (dotted rectangle) at point 1 and leaves it at point 2. The mass flow is {{mvar|ṁ}}. A power {{mvar|P}} is applied and a heat flow {{mvar|Q̇}} is released to the surroundings at ambient temperature {{math|''T''<sub>a</sub>}}.]] |
|||
A power {{mvar|P}} is applied e.g. as electrical power. If the compression is [[adiabatic]], the gas temperature goes up. In the reversible case it would be at constant entropy, which corresponds with a vertical line in the {{math|''T''–''s''}} diagram. For example, compressing nitrogen from 1 bar (point '''a''') to 2 bar (point '''b''') would result in a temperature increase from 300 K to 380 K. In order to let the compressed gas exit at ambient temperature {{math|''T''<sub>a</sub>}}, heat exchange, e.g. by cooling water, is necessary. In the ideal case the compression is isothermal. The average heat flow to the surroundings is {{mvar|Q̇}}. Since the system is in the steady state the first law gives |
|||
:<math>0 = -\dot Q + \dot m h_1 - \dot m h_2 + P.</math> |
|||
The minimal power needed for the compression is realized if the compression is reversible. In that case the [[second law of thermodynamics]] for open systems gives |
|||
:<math>0 = -\frac{\dot Q}{T_\mathrm{a}} + \dot m s_1 - \dot m s_2.</math> |
|||
Eliminating {{mvar|Q̇}} gives for the minimal power |
|||
:<math>\frac{P_\text{min}}{\dot m} = h_2 - h_1 - T_\mathrm{a}(s_2 - s_1).</math> |
|||
For example, compressing 1 kg of nitrogen from 1 bar to 200 bar costs at least {{math|(''h''<sub>'''c'''</sub> − ''h''<sub>'''a'''</sub>) − ''T''<sub>a</sub>(''s''<sub>'''c'''</sub> − ''s''<sub>'''a'''</sub>)}}. With the data, obtained with the {{math|''T''–''s''}} diagram, we find a value of {{nowrap|1=(430 − 461) − 300 × (5.16 − 6.85) =}} 476 kJ/kg. |
|||
The relation for the power can be further simplified by writing it as |
|||
:<math>\frac{P_\text{min}}{\dot m} = \int_1^2(dh - T_\mathrm{a}\,ds).</math> |
|||
With {{math|''dh'' {{=}} ''T'' ''ds'' + ''v'' ''dp''}}, this results in the final relation |
|||
:<math>\frac{P_\text{min}}{\dot m} = \int_1^2 v\,dp.</math> |
|||
==History== |
|||
The term ''enthalpy'' was coined relatively late in the history of thermodynamics, in the early 20th century. [[Energy]] was introduced in a modern sense by [[Thomas Young (scientist)|Thomas Young]] in 1802, while [[entropy]] was coined by [[Rudolf Clausius]] in 1865. ''Energy'' uses the root of the [[Ancient Greek language|Greek]] word {{lang|grc|ἔργον}} (''ergon''), meaning "work", to express the idea of capacity to perform work. ''Entropy'' uses the Greek word {{lang|grc|τροπή}} (''tropē'') meaning ''transformation''. ''Enthalpy'' uses the root of the Greek word {{lang|grc|θάλπος}} (''thalpos'') "warmth, heat"<ref>[https://www.perseus.tufts.edu/hopper/morph?l=qa%2Flpos&la=greek&can=qa%2Flpos0&prior=qalpno/s#lexicon θάλπος] in ''[[A Greek–English Lexicon]]''.</ref> |
|||
===Synthetic polymers=== |
|||
The term expresses the obsolete concept of ''heat content'',<ref>Howard (2002) quotes [[J. R. Partington]] in ''An Advanced Treatise on Physical Chemistry'' (1949) as saying that the function ''H'' was "usually called the heat content".</ref> as {{mvar|dH}} refers to the amount of heat gained in a process at constant pressure only,<ref>{{cite book|first1=Ignacio |last1=Tinoco Jr. |first2=Kenneth |last2=Sauer |first3=James C. |last3=Wang |date=1995 |title=Physical Chemistry |page=41 |edition=3rd |publisher=Prentice-Hall |isbn=978-0-13-186545-7}}</ref> but not in the general case when pressure is variable.<ref>{{cite book|first1=Keith J.|last1=Laidler |author-link=Keith J. Laidler |first2=John H. |last2=Meiser |date=1982 |title=Physical Chemistry |page=53 |publisher=Benjamin/Cummings |isbn=978-0-8053-5682-3}}</ref> |
|||
Many [[polymer]]s are strengthened by hydrogen bonds within and between the chains. Among the [[synthetic polymer]]s, a well characterized example is [[nylon]], where hydrogen bonds occur in the [[repeat unit]] and play a major role in [[crystallization]] of the material. The bonds occur between [[carbonyl]] and [[amine]] groups in the [[amide]] repeat unit. They effectively link adjacent chains, which help reinforce the material. The effect is great in [[aramid]] [[fibre]], where hydrogen bonds stabilize the linear chains laterally. The chain axes are aligned along the fibre axis, making the fibres extremely stiff and strong. |
|||
[[Josiah Willard Gibbs]] used the term "a heat function for constant pressure" for clarity.<ref group=note>''The Collected Works of J. Willard Gibbs, Vol. I'' do not contain reference to the word enthalpy, but rather reference the "heat function for constant pressure". See: {{Cite book |
|||
|last1= Henderson|first1= Douglas|last2= Eyring|first2= Henry|last3= Jost|first3= Wilhelm |
|||
|title= Physical Chemistry: An Advanced Treatise |
|||
|publisher= Academic Press|year= 1967|page= 29 |
|||
}}</ref> |
|||
The hydrogen-bond networks make both natural and synthetic polymers sensitive to [[humidity]] levels in the atmosphere because water molecules can diffuse into the surface and disrupt the network. Some polymers are more sensitive than others. Thus [[nylon]]s are more sensitive than [[aramid]]s, and [[nylon 6]] more sensitive than [[nylon-11]]. |
|||
Introduction of the concept of "heat content" {{mvar|H}} is associated with [[Benoît Paul Émile Clapeyron]] and [[Rudolf Clausius]] ([[Clausius–Clapeyron relation]], 1850). |
|||
==Symmetric hydrogen bond== |
|||
The term ''enthalpy'' first appeared in print in 1909.<ref>{{cite journal |last1=Dalton |first1=J. P. |title=Researches on the Joule–Kelvin-effect, especially at low temperatures. I. Calculations for hydrogen |journal=Proceedings of the Section of Sciences (Koninklijke Akademie van Wetenschappen Te Amsterdam [Royal Academy of Sciences at Amsterdam]) |date=1909 |volume=11 (part 2) |pages=863–873 |url=https://www.biodiversitylibrary.org/item/49173#page/453/mode/1up|bibcode=1908KNAB...11..863D }} ; see p. 864, footnote (1).</ref> It is attributed to [[Heike Kamerlingh Onnes]], who most likely introduced it orally the year before, at the first meeting of the Institute of Refrigeration in Paris.<ref>See: |
|||
A [[symmetric hydrogen bond]] is a special type of hydrogen bond in which the proton is spaced exactly halfway between two identical atoms. The strength of the bond to each of those atoms is equal. It is an example of a [[three-center four-electron bond]]. This type of bond is much stronger than a "normal" hydrogen bond. The effective bond order is 0.5, so its strength is comparable to a covalent bond. It is seen in ice at high pressure, and also in the solid phase of many anhydrous acids such as [[hydrofluoric acid]] and [[formic acid]] at high pressure. It is also seen in the [[bifluoride]] ion [F--H--F]<sup>−</sup>. Due to severe steric constraint, the protonated form of Proton Sponge (1,8-bis(dimethylamino)naphthalene) and its derivatives also have symmetric hydrogen bonds ([N--H--N]<sup>+</sup>),<ref>Khashayar Rajabimoghadam Yousef Darwish Umyeena Bashir Dylan Pitman Sidney Eichelberger Maxime A. Siegler Marcel Swart Isaac Garcia-Bosch Aerobic Oxidation of Alcohols by Copper Complexes Bearing Redox-Active Ligands with Tunable H-Bonding https://doi.org/10.1021/jacs.8b08748</ref> although in the case of protonated Proton Sponge, the assembly is bent.<ref>{{Cite journal|last1=Ozeryanskii|first1=Valery A.|last2=Pozharskii|first2=Alexander F.|last3=Bieńko|first3=Agnieszka J.|last4=Sawka-Dobrowolska|first4=Wanda|last5=Sobczyk|first5=Lucjan|date=2005-03-01|title=[NHN]+ Hydrogen Bonding in Protonated 1,8-Bis(dimethylamino)-2,7-dimethoxynaphthalene. X-ray Diffraction, Infrared, and Theoretical ab Initio and DFT Studies|journal=The Journal of Physical Chemistry A|volume=109|issue=8|pages=1637–1642|doi=10.1021/jp040618l|pmid=16833488|bibcode=2005JPCA..109.1637O|issn=1089-5639}}</ref> |
|||
* {{Cite book|last= Laidler|first1= Keith|author-link= Keith J. Laidler|title= The World of Physical Chemistry|publisher= Oxford University Press|year= 1995|page= 110}} |
|||
* {{Cite journal |last= Van Ness|first= Hendrick C. |year= 2003 |title=''H'' Is for Enthalpy |journal= Journal of Chemical Education|volume= 80|issue= 6|pages= 486 |bibcode= 2003JChEd..80..486V|doi= 10.1021/ed080p486.1|doi-access= free}}</ref> |
|||
It gained currency only in the 1920s, notably with the ''[[enthalpy–entropy chart|Mollier Steam Tables and Diagrams]]'', published in 1927. |
|||
==Dihydrogen bond== |
|||
Until the 1920s, the symbol {{mvar|H}} was used, somewhat inconsistently, for "heat" in general. |
|||
The hydrogen bond can be compared with the closely related [[dihydrogen bond]], which is also an [[Intermolecular force|intermolecular]] bonding interaction involving hydrogen atoms. These structures have been known for some time, and well characterized by [[X-ray crystallography|crystallography]];<ref name=crab>{{cite journal|title = A New Intermolecular Interaction: Unconventional Hydrogen Bonds with Element-Hydride Bonds as Proton Acceptor|last1 = Crabtree|first1 = Robert H. |author-link1=Robert H. Crabtree|last2 =Siegbahn |first2= Per E. M. |first3= Odile |last3 =Eisenstein |first4 =Arnold L. |last4 =Rheingold |first5 = Thomas F. |last5 =Koetzle|journal = [[Acc. Chem. Res.]]|year = 1996|volume = 29|issue = 7|pages = 348–354|doi = 10.1021/ar950150s|pmid = 19904922}}</ref> however, an understanding of their relationship to the conventional hydrogen bond, [[ionic bond]], and [[covalent bond]] remains unclear. Generally, the hydrogen bond is characterized by a proton acceptor that is a lone pair of electrons in nonmetallic atoms (most notably in the [[nitrogen group|nitrogen]], and [[chalcogen]] groups). In some cases, these proton acceptors may be [[pi-bond]]s or [[metal complexes]]. In the dihydrogen bond, however, a metal hydride serves as a proton acceptor, thus forming a hydrogen-hydrogen interaction. [[Neutron diffraction]] has shown that the [[molecular geometry]] of these complexes is similar to hydrogen bonds, in that the bond length is very adaptable to the metal complex/hydrogen donor system.<ref name=crab/> |
|||
The definition of {{mvar|H}} as strictly limited to enthalpy or "heat content at constant pressure" was formally proposed by Alfred W. Porter in 1922.<ref>{{cite journal |last1=Porter |first1=Alfred W. |title=The generation and utilisation of cold. A general discussion. |journal=Transactions of the Faraday Society |date=1922 |volume=18 |pages=139–143 |url=https://babel.hathitrust.org/cgi/pt?id=njp.32101076787439;view=1up;seq=157 |doi=10.1039/tf9221800139}}; see p. 140.</ref><ref name=Howard> |
|||
{{Cite journal |
|||
|doi= 10.1021/ed079p697 |last= Howard|first= Irmgard |
|||
|year= 2002 |
|||
|title= ''H'' Is for Enthalpy, Thanks to Heike Kamerlingh Onnes and Alfred W. Porter |
|||
|journal= Journal of Chemical Education|volume= 79|issue= 6|pages= 697 |
|||
|bibcode = 2002JChEd..79..697H |
|||
}}</ref> |
|||
==Dynamics probed by spectroscopic means== |
|||
==See also== |
|||
The dynamics of hydrogen bond structures in water can be probed by the [[IR spectrum]] of OH stretching vibration.<ref name="cowan">{{cite journal |author=Cowan ML |title=Ultrafast memory loss and energy redistribution in the hydrogen bond network of liquid H<sub>2</sub>O |journal=Nature |volume=434 |issue=7030 |pages=199–202 |year=2005 |pmid=15758995 |doi=10.1038/nature03383 |author2=Bruner BD |author3=Huse N |display-authors=3 |last4=Dwyer |first4=J. R. |last5=Chugh |first5=B. |last6=Nibbering |first6=E. T. J. |last7=Elsaesser |first7=T. |last8=Miller |first8=R. J. D.|bibcode = 2005Natur.434..199C |s2cid=4396493 }}</ref> In the hydrogen bonding network in protic organic ionic plastic crystals (POIPCs), which are a type of phase change material exhibiting solid-solid phase transitions prior to melting, variable-temperature infrared spectroscopy can reveal the temperature dependence of hydrogen bonds and the dynamics of both the anions and the cations.<ref name="1,2,4-Triazolium perfluorobutanesulfonate as an archetypal pure protic organic ionic plastic crystal electrolyte for all-solid-state fuel cells"/> The sudden weakening of hydrogen bonds during the solid-solid phase transition seems to be coupled with the onset of orientational or rotational disorder of the ions.<ref name="1,2,4-Triazolium perfluorobutanesulfonate as an archetypal pure protic organic ionic plastic crystal electrolyte for all-solid-state fuel cells"> |
|||
* [[Standard enthalpy change of formation (data table)]] |
|||
{{cite journal |
|||
* [[Calorimetry]] |
|||
|year = 2015 |
|||
* [[Calorimeter]] |
|||
|title = 1,2,4-Triazolium perfluorobutanesulfonate as an archetypal pure protic organic ionic plastic crystal electrolyte for all-solid-state fuel cells |
|||
* [[Departure function]] |
|||
|journal = [[Energy & Environmental Science]] |
|||
* [[Hess's law]] |
|||
|volume = 8 |
|||
* [[Isenthalpic process]] |
|||
|issue = 4 |
|||
* [[Laws of thermodynamics]] |
|||
|pages = 1276 |
|||
* [[Stagnation enthalpy]] |
|||
|doi = 10.1039/C4EE02280G |
|||
* [[Thermodynamic databases for pure substances]] |
|||
|last1 = Luo |
|||
|first1 = Jiangshui |
|||
|last2 = Jensen |
|||
|first2 = Annemette H. |
|||
|last3 = Brooks |
|||
|first3 = Neil R. |
|||
|last4 = Sniekers |
|||
|first4 = Jeroen |
|||
|last5 = Knipper |
|||
|first5 = Martin |
|||
|last6 = Aili |
|||
|first6 = David |
|||
|last7 = Li |
|||
|first7 = Qingfeng |
|||
|last8 = Vanroy |
|||
|first8 = Bram |
|||
|last9 = Wübbenhorst |
|||
|first9 = Michael |
|||
|last10 = Yan |
|||
|first10 = Feng |
|||
|last11 = Van Meervelt |
|||
|first11 = Luc |
|||
|last12 = Shao |
|||
|first12 = Zhigang |
|||
|last13 = Fang |
|||
|first13 = Jianhua |
|||
|last14 = Luo |
|||
|first14 = Zheng-Hong |
|||
|last15 = De Vos |
|||
|first15 = Dirk E. |
|||
|last16 = Binnemans |
|||
|first16 = Koen |
|||
|last17 = Fransaer |
|||
|first17 = Jan |
|||
|s2cid = 84176511 |
|||
|url = https://semanticscholar.org/paper/597630b38bff9080dedb9aa1eba16f9f10092eb4 |
|||
}}</ref> |
|||
==Application to drugs== |
|||
==Notes== |
|||
Hydrogen bonding is a key to the design of drugs. According to [[Lipinski's rule of five]] the majority of orally active drugs tend to have between five and ten hydrogen bonds. These interactions exist between [[nitrogen]]–[[hydrogen]] and [[oxygen]]–hydrogen centers.<ref name="Lipinski_2004">{{cite journal | author = Lipinski CA | title = Lead- and drug-like compounds: the rule-of-five revolution | journal = Drug Discovery Today: Technologies |date=December 2004 | volume = 1 | issue = 4 | pages = 337–341 | doi = 10.1016/j.ddtec.2004.11.007 | pmid = 24981612 }}</ref> As with many other [[rules of thumb]], many exceptions exist. |
|||
{{Reflist|group=note}} |
|||
==References== |
==References== |
||
{{ |
{{reflist|35em}} |
||
== |
==Further reading== |
||
* George A. Jeffrey. ''An Introduction to Hydrogen Bonding (Topics in Physical Chemistry)''. Oxford University Press, USA (March 13, 1997). {{ISBN|0-19-509549-9}} |
|||
* {{cite journal|url=http://www.dwc.knaw.nl/DL/publications/PU00013601.pdf |first=J.P. |last=Dalton |title=Researches on the Joule–Kelvin effect, especially at low temperatures. I. Calculations for hydrogen |journal=KNAW Proceedings |volume=11 |pages=863–873 |date=1909|bibcode=1908KNAB...11..863D }} |
|||
* {{cite book|last=Haase |first=R. |title=Physical Chemistry: An Advanced Treatise |editor-last=Jost |editor-first=W. |publisher=Academic |location=New York |date=1971 |page=29}} |
|||
* {{cite book|last=Gibbs |first=J. W. |title=The Collected Works of J. Willard Gibbs, Vol. I |publisher=Yale University Press |location=New Haven, CT |edition=1948 |page=88}} |
|||
* {{cite journal|doi=10.1021/ed079p697 |first=I. K. |last=Howard |title=''H'' Is for Enthalpy, Thanks to Heike Kamerlingh Onnes and Alfred W. Porter |journal=[[J. Chem. Educ.]] |volume=79 |issue=6 |pages=697–698 |date=2002|bibcode = 2002JChEd..79..697H }} |
|||
* {{cite book|last=Laidler |first=K. |title=The World of Physical Chemistry |url=https://archive.org/details/worldofphysicalc0000laid |url-access=registration |publisher=Oxford University Press |location=Oxford |date=1995 |page=[https://archive.org/details/worldofphysicalc0000laid/page/110 110]}} |
|||
* {{cite book|first1=C. |last1=Kittel |first2=H. |last2=Kroemer |title=Thermal Physics |publisher=S. R. Furphy & Co. |location=New York |date=1980 |page=246}} |
|||
* {{Cite book|last=DeHoff |first=R. |year=2006 |title=Thermodynamics in Materials Science |publisher=CRC Press|isbn=9780849340659 }} |
|||
==External links== |
==External links== |
||
* [https://web.archive.org/web/20141203035841/http://www.magnet.fsu.edu/education/tutorials/slideshows/bubblewall/index.html The Bubble Wall] (Audio slideshow from the National High Magnetic Field Laboratory explaining cohesion, surface tension and hydrogen bonds) |
|||
* [http://scienceworld.wolfram.com/physics/Enthalpy.html Enthalpy] – Eric Weisstein's World of Physics |
|||
* [http://scitation.aip.org/content/aip/journal/jcp/129/19/10.1063/1.3006032 isotopic effect on bond dynamics] |
|||
* [http://hyperphysics.phy-astr.gsu.edu/hbase/thermo/firlaw.html Enthalpy] – Georgia State University |
|||
{{Chemical bonds}} |
|||
* [https://web.archive.org/web/20061010093705/http://www.chem.tamu.edu/class/majors/tutorialnotefiles/enthalpy.htm Enthalpy example calculations] – Texas A&M University Chemistry Department |
|||
{{HVAC}} |
|||
{{Authority control}} |
{{Authority control}} |
||
{{DEFAULTSORT:Hydrogen Bonds}} |
|||
[[Category:Enthalpy| ]] |
|||
[[Category: |
[[Category:Chemical bonding]] |
||
[[Category: |
[[Category:Hydrogen physics]] |
||
[[Category: |
[[Category:Supramolecular chemistry]] |
||
[[Category:Intermolecular forces]] |
Revision as of 09:55, 8 September 2021


A hydrogen bond (or H-bond) is a primarily electrostatic force of attraction between a hydrogen (H) atom which is covalently bound to a more electronegative atom or group, and another electronegative atom bearing a lone pair of electrons—the hydrogen bond acceptor (Ac). Such an interacting system is generally denoted Dn–H···Ac, where the solid line denotes a polar covalent bond, and the dotted or dashed line indicates the hydrogen bond.[4] The most frequent donor and acceptor atoms are the second-row elements nitrogen (N), oxygen (O), and fluorine (F)
Hydrogen bonds can be intermolecular (occurring between separate molecules) or intramolecular (occurring among parts of the same molecule).[5][6][7][8] The energy of a hydrogen bond depends on the geometry, the environment, and the nature of the specific donor and acceptor atoms, and can vary between 1 and 40 kcal/mol.[9] This makes them somewhat stronger than a van der Waals interaction, and weaker than fully covalent or ionic bonds. This type of bond can occur in inorganic molecules such as water and in organic molecules like DNA and proteins. Hydrogen bonds are responsible for holding such materials as paper and felted wool together, and for causing separate sheets of paper to stick together after becoming wet and subsequently drying.
The hydrogen bond is responsible for many of the anomalous physical and chemical properties of compounds of N, O, and F. In particular, intermolecular hydrogen bonding is responsible for the high boiling point of water (100 °C) compared to the other group-16 hydrides that have much weaker hydrogen bonds.[10] Intramolecular hydrogen bonding is partly responsible for the secondary and tertiary structures of proteins and nucleic acids. It also plays an important role in the structure of polymers, both synthetic and natural.
Bonding
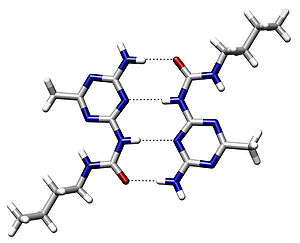

Definitions and general characteristics
A hydrogen atom attached to a relatively electronegative atom is the hydrogen bond donor.[12] C-H bonds only participate in hydrogen bonding when the carbon atom is bound to electronegative substituents, as is the case in chloroform, CHCl3.[13] In a hydrogen bond, the electronegative atom not covalently attached to the hydrogen is named proton acceptor, whereas the one covalently bound to the hydrogen is named the proton donor. While this nomenclature is recommended by the IUPAC,[4] it can be misleading, since in other donor-acceptor bonds, the donor/acceptor assignment is based on the source of the electron pair (such nomenclature is also used for hydrogen bonds by some authors[9]). In the hydrogen bond donor, the H center is protic. The donor is a Lewis base. Hydrogen bonds are represented as H···Y system, where the dots represent the hydrogen bond. Liquids that display hydrogen bonding (such as water) are called associated liquids.


The hydrogen bond is often described as an electrostatic dipole-dipole interaction. However, it also has some features of covalent bonding: it is directional and strong, produces interatomic distances shorter than the sum of the van der Waals radii, and usually involves a limited number of interaction partners, which can be interpreted as a type of valence. These covalent features are more substantial when acceptors bind hydrogens from more electronegative donors.
As part of a more detailed list of criteria, the IUPAC publication acknowledges that the attractive interaction can arise from some combination of electrostatics (multipole-multipole and multipole-induced multipole interactions), covalency (charge transfer by orbital overlap), and dispersion (London forces), and states that the relative importance of each will vary depending on the system. However, a footnote to the criterion recommends the exclusion of interactions in which dispersion is the primary contributor, specifically giving Ar---CH4 and CH4---CH4 as examples of such interactions to be excluded from the definition.[4] Nevertheless, most introductory textbooks still restrict the definition of hydrogen bond to the "classical" type of hydrogen bond characterized in the opening paragraph.
Weaker hydrogen bonds[14] are known for hydrogen atoms bound to elements such as sulfur (S) or chlorine (Cl); even carbon (C) can serve as a donor, particularly when the carbon or one of its neighbors is electronegative (e.g., in chloroform, aldehydes and terminal acetylenes).[15][16] Gradually, it was recognized that there are many examples of weaker hydrogen bonding involving donor other than N, O, or F and/or acceptor Ac with electronegativity approaching that of hydrogen (rather than being much more electronegative). Though these "non-traditional" hydrogen bonding interactions are often quite weak (~1 kcal/mol), they are also ubiquitous and are increasingly recognized as important control elements in receptor-ligand interactions in medicinal chemistry or intra-/intermolecular interactions in materials sciences.
The definition of hydrogen bonding has gradually broadened over time to include these weaker attractive interactions. In 2011, an IUPAC Task Group recommended a modern evidence-based definition of hydrogen bonding, which was published in the IUPAC journal Pure and Applied Chemistry. This definition specifies:
The hydrogen bond is an attractive interaction between a hydrogen atom from a molecule or a molecular fragment X–H in which X is more electronegative than H, and an atom or a group of atoms in the same or a different molecule, in which there is evidence of bond formation.[17]
Bond strength
Hydrogen bonds can vary in strength from weak (1–2 kJ mol−1) to strong (161.5 kJ mol−1 in the ion HF−
2).[18][19] Typical enthalpies in vapor include:[20]
- F−H···:F (161.5 kJ/mol or 38.6 kcal/mol), illustrated uniquely by HF2−, bifluoride
- O−H···:N (29 kJ/mol or 6.9 kcal/mol), illustrated water-ammonia
- O−H···:O (21 kJ/mol or 5.0 kcal/mol), illustrated water-water, alcohol-alcohol
- N−H···:N (13 kJ/mol or 3.1 kcal/mol), illustrated by ammonia-ammonia
- N−H···:O (8 kJ/mol or 1.9 kcal/mol), illustrated water-amide
- OH+
3···:OH
2 (18 kJ/mol[21] or 4.3 kcal/mol)
The strength of intermolecular hydrogen bonds is most often evaluated by measurements of equilibria between molecules containing donor and/or acceptor units, most often in solution.[22] The strength of intramolecular hydrogen bonds can be studied with equilibria between conformers with and without hydrogen bonds. The most important method for the identification of hydrogen bonds also in complicated molecules is crystallography, sometimes also NMR-spectroscopy. Structural details, in particular distances between donor and acceptor which are smaller than the sum of the van der Waals radii can be taken as indication of the hydrogen bond strength.
One scheme gives the following somewhat arbitrary classification: those that are 15 to 40 kcal/mol, 5 to 15 kcal/mol, and >0 to 5 kcal/mol are considered strong, moderate, and weak, respectively.
Structural details
The X−H distance is typically ≈110 pm, whereas the H···Y distance is ≈160 to 200 pm. The typical length of a hydrogen bond in water is 197 pm. The ideal bond angle depends on the nature of the hydrogen bond donor. The following hydrogen bond angles between a hydrofluoric acid donor and various acceptors have been determined experimentally:[23]
Acceptor···donor | VSEPR geometry | Angle (°) |
---|---|---|
HCN···HF | linear | 180 |
H2CO···HF | trigonal planar | 120 |
H2O···HF | pyramidal | 46 |
H2S···HF | pyramidal | 89 |
SO2···HF | trigonal | 142 |
Spectroscopy
Strong hydrogen bonds are revealed by downfield shifts in the 1H NMR spectrum. For example, the acidic proton in the enol tautomer of acetylacetone appears at δH 15.5, which is about 10 ppm downfield of a conventional alcohol.[24]
In the IR spectrum, hydrogen bonding shifts the X-H stretching frequency to lower energy (i.e. the vibration frequency decreases). This shift reflects a weakening of the X-H bond. Certain hydrogen bonds - improper hydrogen bonds - show a blue shift of the X-H stretching frequency and a decrease in the bond length.[25] H-bonds can also be measured by IR vibrational mode shifts of the acceptor. The amide I mode of backbone carbonyls in α-helices shifts to lower frequencies when they form H-bonds with side-chain hydroxyl groups.[26]
Theoretical considerations
Hydrogen bonding is of persistent theoretical interest.[27] According to a modern description O:H-O integrates both the intermolecular O:H lone pair ":" nonbond and the intramolecular H-O polar-covalent bond associated with O-O repulsive coupling.[28]
Quantum chemical calculations of the relevant interresidue potential constants (compliance constants) revealed[how?] large differences between individual H bonds of the same type. For example, the central interresidue N−H···N hydrogen bond between guanine and cytosine is much stronger in comparison to the N−H···N bond between the adenine-thymine pair.[29]
Theoretically, the bond strength of the hydrogen bonds can be assessed using NCI index, non-covalent interactions index, which allows a visualization of these non-covalent interactions, as its name indicates, using the electron density of the system.
From interpretations of the anisotropies in the Compton profile of ordinary ice that the hydrogen bond is partly covalent.[30] However, this interpretation was challenged.[31]
Most generally, the hydrogen bond can be viewed as a metric-dependent electrostatic scalar field between two or more intermolecular bonds. This is slightly different from the intramolecular bound states of, for example, covalent or ionic bonds; however, hydrogen bonding is generally still a bound state phenomenon, since the interaction energy has a net negative sum. The initial theory of hydrogen bonding proposed by Linus Pauling suggested that the hydrogen bonds had a partial covalent nature. This interpretation remained controversial until NMR techniques demonstrated information transfer between hydrogen-bonded nuclei, a feat that would only be possible if the hydrogen bond contained some covalent character.[32]
History
The concept of hydrogen bonding once was challenging.[33] Linus Pauling credits T. S. Moore and T. F. Winmill with the first mention of the hydrogen bond, in 1912.[34][35] Moore and Winmill used the hydrogen bond to account for the fact that trimethylammonium hydroxide is a weaker base than tetramethylammonium hydroxide. The description of hydrogen bonding in its better-known setting, water, came some years later, in 1920, from Latimer and Rodebush.[36] In that paper, Latimer and Rodebush cite work by a fellow scientist at their laboratory, Maurice Loyal Huggins, saying, "Mr. Huggins of this laboratory in some work as yet unpublished, has used the idea of a hydrogen kernel held between two atoms as a theory in regard to certain organic compounds."
Hydrogen bonds in small molecules

Water
A ubiquitous example of a hydrogen bond is found between water molecules. In a discrete water molecule, there are two hydrogen atoms and one oxygen atom. The simplest case is a pair of water molecules with one hydrogen bond between them, which is called the water dimer and is often used as a model system. When more molecules are present, as is the case with liquid water, more bonds are possible because the oxygen of one water molecule has two lone pairs of electrons, each of which can form a hydrogen bond with a hydrogen on another water molecule. This can repeat such that every water molecule is H-bonded with up to four other molecules, as shown in the figure (two through its two lone pairs, and two through its two hydrogen atoms). Hydrogen bonding strongly affects the crystal structure of ice, helping to create an open hexagonal lattice. The density of ice is less than the density of water at the same temperature; thus, the solid phase of water floats on the liquid, unlike most other substances.
Liquid water's high boiling point is due to the high number of hydrogen bonds each molecule can form, relative to its low molecular mass. Owing to the difficulty of breaking these bonds, water has a very high boiling point, melting point, and viscosity compared to otherwise similar liquids not conjoined by hydrogen bonds. Water is unique because its oxygen atom has two lone pairs and two hydrogen atoms, meaning that the total number of bonds of a water molecule is up to four.
The number of hydrogen bonds formed by a molecule of liquid water fluctuates with time and temperature.[37] From TIP4P liquid water simulations at 25 °C, it was estimated that each water molecule participates in an average of 3.59 hydrogen bonds. At 100 °C, this number decreases to 3.24 due to the increased molecular motion and decreased density, while at 0 °C, the average number of hydrogen bonds increases to 3.69.[37] Another study found a much smaller number of hydrogen bonds: 2.357 at 25 °C.[38] The differences may be due to the use of a different method for defining and counting the hydrogen bonds.
Where the bond strengths are more equivalent, one might instead find the atoms of two interacting water molecules partitioned into two polyatomic ions of opposite charge, specifically hydroxide (OH−) and hydronium (H3O+). (Hydronium ions are also known as "hydroxonium" ions.)
- H−O− H3O+
Indeed, in pure water under conditions of standard temperature and pressure, this latter formulation is applicable only rarely; on average about one in every 5.5 × 108 molecules gives up a proton to another water molecule, in accordance with the value of the dissociation constant for water under such conditions. It is a crucial part of the uniqueness of water.
Because water may form hydrogen bonds with solute proton donors and acceptors, it may competitively inhibit the formation of solute intermolecular or intramolecular hydrogen bonds. Consequently, hydrogen bonds between or within solute molecules dissolved in water are almost always unfavorable relative to hydrogen bonds between water and the donors and acceptors for hydrogen bonds on those solutes.[39] Hydrogen bonds between water molecules have an average lifetime of 10−11 seconds, or 10 picoseconds.[40]
Bifurcated and over-coordinated hydrogen bonds in water
A single hydrogen atom can participate in two hydrogen bonds, rather than one. This type of bonding is called "bifurcated" (split in two or "two-forked"). It can exist, for instance, in complex natural or synthetic organic molecules.[41] It has been suggested that a bifurcated hydrogen atom is an essential step in water reorientation.[42]
Acceptor-type hydrogen bonds (terminating on an oxygen's lone pairs) are more likely to form bifurcation (it is called overcoordinated oxygen, OCO) than are donor-type hydrogen bonds, beginning on the same oxygen's hydrogens.[43]
Other liquids
For example, hydrogen fluoride—which has three lone pairs on the F atom but only one H atom—can form only two bonds; (ammonia has the opposite problem: three hydrogen atoms but only one lone pair).
- H−F···H−F···H−F
Further manifestations of solvent hydrogen bonding
- Increase in the melting point, boiling point, solubility, and viscosity of many compounds can be explained by the concept of hydrogen bonding.
- Negative azeotropy of mixtures of HF and water
- The fact that ice is less dense than liquid water is due to a crystal structure stabilized by hydrogen bonds.
- Dramatically higher boiling points of NH3, H2O, and HF compared to the heavier analogues PH3, H2S, and HCl, where hydrogen-bonding is absent.
- Viscosity of anhydrous phosphoric acid and of glycerol
- Dimer formation in carboxylic acids and hexamer formation in hydrogen fluoride, which occur even in the gas phase, resulting in gross deviations from the ideal gas law.
- Pentamer formation of water and alcohols in apolar solvents.
Hydrogen bonds in polymers
Hydrogen bonding plays an important role in determining the three-dimensional structures and the properties adopted by many synthetic and natural proteins. Compared to the C-C, C-O, and C-N bonds that comprise most polymers, hydrogen bonds are far weaker, perhaps 5%. Thus, hydrogen bonds can be broken by chemical or mechanical means while retaining the basic structure of the polymer backbone. This hierarchy of bond strengths (covalent bonds being stronger than hydrogen-bonds being stronger than van der Waals forces) is key to understanding the properties of many materials.[44]
DNA


In these macromolecules, bonding between parts of the same macromolecule cause it to fold into a specific shape, which helps determine the molecule's physiological or biochemical role. For example, the double helical structure of DNA is due largely to hydrogen bonding between its base pairs (as well as pi stacking interactions), which link one complementary strand to the other and enable replication.
Proteins
In the secondary structure of proteins, hydrogen bonds form between the backbone oxygens and amide hydrogens. When the spacing of the amino acid residues participating in a hydrogen bond occurs regularly between positions i and i + 4, an alpha helix is formed. When the spacing is less, between positions i and i + 3, then a 310 helix is formed. When two strands are joined by hydrogen bonds involving alternating residues on each participating strand, a beta sheet is formed. Hydrogen bonds also play a part in forming the tertiary structure of protein through interaction of R-groups. (See also protein folding).
Bifurcated H-bond systems are common in alpha-helical transmembrane proteins between the backbone amide C=O of residue i as the H-bond acceptor and two H-bond donors from residue i+4: the backbone amide N-H and a side-chain hydroxyl or thiol H+. The energy preference of the bifurcated H-bond hydroxyl or thiol system is -3.4 kcal/mol or -2.6 kcal/mol, respectively. This type of bifurcated H-bond provides an intrahelical H-bonding partner for polar side-chains, such as serine, threonine, and cysteine within the hydrophobic membrane environments.[26]
The role of hydrogen bonds in protein folding has also been linked to osmolyte-induced protein stabilization. Protective osmolytes, such as trehalose and sorbitol, shift the protein folding equilibrium toward the folded state, in a concentration dependent manner. While the prevalent explanation for osmolyte action relies on excluded volume effects that are entropic in nature, circular dichroism (CD) experiments have shown osmolyte to act through an enthalpic effect.[45] The molecular mechanism for their role in protein stabilization is still not well established, though several mechanisms have been proposed. Computer molecular dynamics simulations suggest that osmolytes stabilize proteins by modifying the hydrogen bonds in the protein hydration layer.[46]
Several studies have shown that hydrogen bonds play an important role for the stability between subunits in multimeric proteins. For example, a study of sorbitol dehydrogenase displayed an important hydrogen bonding network which stabilizes the tetrameric quaternary structure within the mammalian sorbitol dehydrogenase protein family.[47]
A protein backbone hydrogen bond incompletely shielded from water attack is a dehydron. Dehydrons promote the removal of water through proteins or ligand binding. The exogenous dehydration enhances the electrostatic interaction between the amide and carbonyl groups by de-shielding their partial charges. Furthermore, the dehydration stabilizes the hydrogen bond by destabilizing the nonbonded state consisting of dehydrated isolated charges.[48]
Wool, being a protein fibre, is held together by hydrogen bonds, causing wool to recoil when stretched. However, washing at high temperatures can permanently break the hydrogen bonds and a garment may permanently lose its shape.
Cellulose
Hydrogen bonds are important in the structure of cellulose and derived polymers in its many different forms in nature, such as cotton and flax.


Synthetic polymers
Many polymers are strengthened by hydrogen bonds within and between the chains. Among the synthetic polymers, a well characterized example is nylon, where hydrogen bonds occur in the repeat unit and play a major role in crystallization of the material. The bonds occur between carbonyl and amine groups in the amide repeat unit. They effectively link adjacent chains, which help reinforce the material. The effect is great in aramid fibre, where hydrogen bonds stabilize the linear chains laterally. The chain axes are aligned along the fibre axis, making the fibres extremely stiff and strong.
The hydrogen-bond networks make both natural and synthetic polymers sensitive to humidity levels in the atmosphere because water molecules can diffuse into the surface and disrupt the network. Some polymers are more sensitive than others. Thus nylons are more sensitive than aramids, and nylon 6 more sensitive than nylon-11.
Symmetric hydrogen bond
A symmetric hydrogen bond is a special type of hydrogen bond in which the proton is spaced exactly halfway between two identical atoms. The strength of the bond to each of those atoms is equal. It is an example of a three-center four-electron bond. This type of bond is much stronger than a "normal" hydrogen bond. The effective bond order is 0.5, so its strength is comparable to a covalent bond. It is seen in ice at high pressure, and also in the solid phase of many anhydrous acids such as hydrofluoric acid and formic acid at high pressure. It is also seen in the bifluoride ion [F--H--F]−. Due to severe steric constraint, the protonated form of Proton Sponge (1,8-bis(dimethylamino)naphthalene) and its derivatives also have symmetric hydrogen bonds ([N--H--N]+),[49] although in the case of protonated Proton Sponge, the assembly is bent.[50]
Dihydrogen bond
The hydrogen bond can be compared with the closely related dihydrogen bond, which is also an intermolecular bonding interaction involving hydrogen atoms. These structures have been known for some time, and well characterized by crystallography;[51] however, an understanding of their relationship to the conventional hydrogen bond, ionic bond, and covalent bond remains unclear. Generally, the hydrogen bond is characterized by a proton acceptor that is a lone pair of electrons in nonmetallic atoms (most notably in the nitrogen, and chalcogen groups). In some cases, these proton acceptors may be pi-bonds or metal complexes. In the dihydrogen bond, however, a metal hydride serves as a proton acceptor, thus forming a hydrogen-hydrogen interaction. Neutron diffraction has shown that the molecular geometry of these complexes is similar to hydrogen bonds, in that the bond length is very adaptable to the metal complex/hydrogen donor system.[51]
Dynamics probed by spectroscopic means
The dynamics of hydrogen bond structures in water can be probed by the IR spectrum of OH stretching vibration.[52] In the hydrogen bonding network in protic organic ionic plastic crystals (POIPCs), which are a type of phase change material exhibiting solid-solid phase transitions prior to melting, variable-temperature infrared spectroscopy can reveal the temperature dependence of hydrogen bonds and the dynamics of both the anions and the cations.[53] The sudden weakening of hydrogen bonds during the solid-solid phase transition seems to be coupled with the onset of orientational or rotational disorder of the ions.[53]
Application to drugs
Hydrogen bonding is a key to the design of drugs. According to Lipinski's rule of five the majority of orally active drugs tend to have between five and ten hydrogen bonds. These interactions exist between nitrogen–hydrogen and oxygen–hydrogen centers.[54] As with many other rules of thumb, many exceptions exist.
References
- ^ Sweetman, A. M.; Jarvis, S. P.; Sang, Hongqian; Lekkas, I.; Rahe, P.; Wang, Yu; Wang, Jianbo; Champness, N.R.; Kantorovich, L.; Moriarty, P. (2014). "Mapping the force field of a hydrogen-bonded assembly". Nature Communications. 5: 3931. Bibcode:2014NatCo...5.3931S. doi:10.1038/ncomms4931. PMC 4050271. PMID 24875276.
- ^ Hapala, Prokop; Kichin, Georgy; Wagner, Christian; Tautz, F. Stefan; Temirov, Ruslan; Jelínek, Pavel (2014-08-19). "Mechanism of high-resolution STM/AFM imaging with functionalized tips". Physical Review B. 90 (8): 085421. arXiv:1406.3562. Bibcode:2014PhRvB..90h5421H. doi:10.1103/PhysRevB.90.085421. S2CID 53610973.
- ^ Hämäläinen, Sampsa K.; van der Heijden, Nadine; van der Lit, Joost; den Hartog, Stephan; Liljeroth, Peter; Swart, Ingmar (2014-10-31). "Intermolecular Contrast in Atomic Force Microscopy Images without Intermolecular Bonds". Physical Review Letters. 113 (18): 186102. arXiv:1410.1933. Bibcode:2014PhRvL.113r6102H. doi:10.1103/PhysRevLett.113.186102. hdl:1874/307996. PMID 25396382. S2CID 8309018. Archived from the original on 2018-01-20. Retrieved 2017-08-30.
- ^ a b c Arunan, Elangannan; Desiraju, Gautam R.; Klein, Roger A.; Sadlej, Joanna; Scheiner, Steve; Alkorta, Ibon; Clary, David C.; Crabtree, Robert H.; Dannenberg, Joseph J. (2011-07-08). "Definition of the hydrogen bond (IUPAC Recommendations 2011)". Pure and Applied Chemistry. 83 (8): 1637–1641. doi:10.1351/PAC-REC-10-01-02. ISSN 1365-3075. S2CID 97688573.
- ^ Pimentel, G. The Hydrogen Bond Franklin Classics, 2018), ISBN 0343171600
- ^ Jeffrey, G. A.; An introduction to hydrogen bonding; Oxford university press New York, 1997. ISBN 0195095499
- ^ Jeffrey, G. A.; Saenger, W. Hydrogen bonding in biological structures; Springer: Berlin, 1994, 2012 Springer; ISBN 3540579036
- ^ IUPAC, Compendium of Chemical Terminology, 2nd ed. (the "Gold Book") (1997). Online corrected version: (2006–) "hydrogen bond". doi:10.1351/goldbook.H02899
- ^ a b Steiner, Thomas (2002). "The Hydrogen Bond in the Solid State". Angew. Chem. Int. Ed. 41 (1): 48–76. doi:10.1002/1521-3773(20020104)41:1<48::AID-ANIE48>3.0.CO;2-U. PMID 12491444.
- ^ Sabin, John R. (1971). "Hydrogen bonds involving sulfur. I. Hydrogen sulfide dimer". J. Am. Chem. Soc. 93 (15): 3613–3620. doi:10.1021/ja00744a012.
- ^ Beijer, Felix H.; Kooijman, Huub; Spek, Anthony L.; Sijbesma, Rint P.; Meijer, E. W. (1998). "Self-Complementarity Achieved through Quadruple Hydrogen Bonding". Angew. Chem. Int. Ed. 37 (1–2): 75–78. doi:10.1002/(SICI)1521-3773(19980202)37:1/2<75::AID-ANIE75>3.0.CO;2-R.
- ^ Campbell, Neil A.; Brad Williamson; Robin J. Heyden (2006). Biology: Exploring Life. Boston, Massachusetts: Pearson Prentice Hall. ISBN 978-0-13-250882-7. Archived from the original on 2014-11-02. Retrieved 2008-11-11.
- ^ Wiley, G.R.; Miller, S.I. (1972). "Thermodynamic parameters for hydrogen bonding of chloroform with Lewis bases in cyclohexane. Proton magnetic resonance study". Journal of the American Chemical Society. 94 (10): 3287. doi:10.1021/ja00765a001.
- ^ Desiraju, G. R. and Steiner, T. The Weak Hydrogen Bond: In Structural Chemistry and Biology , International Union of Crystallography;2001, ISBN 0198509707
- ^ Nishio, M.; Hirota, M.; Umezawa, Y. The CH–π Interactions; Wiley-VCH, New York, 1998. • Wiley-VCH; 1998) ISBN 0471252905
- ^ Nishio, M (2011). "The CH/[small pi] hydrogen bond in chemistry. "Title". Phys. Chem. Chem. Phys. 13 (31): 13873–13900. doi:10.1039/c1cp20404a. PMID 21611676.
- ^ Arunan, Elangannan; Desiraju, Gautam R.; Klein, Roger A.; Sadlej, Joanna; Scheiner, Steve; Alkorta, Ibon; Clary, David C.; Crabtree, Robert H.; Dannenberg, Joseph J.; Hobza, Pavel; Kjaergaard, Henrik G.; Legon, Anthony C.; Mennucci, Benedetta; Nesbitt, David J. (2011). "Definition of the hydrogen bond". Pure Appl. Chem. 83 (8): 1637–1641. doi:10.1351/PAC-REC-10-01-02. S2CID 97688573.
- ^ Larson, J. W.; McMahon, T. B. (1984). "Gas-phase bihalide and pseudobihalide ions. An ion cyclotron resonance determination of hydrogen bond energies in XHY- species (X, Y = F, Cl, Br, CN)". Inorganic Chemistry. 23 (14): 2029–2033. doi:10.1021/ic00182a010.
- ^ Emsley, J. (1980). "Very Strong Hydrogen Bonds". Chemical Society Reviews. 9 (1): 91–124. doi:10.1039/cs9800900091.
- ^ V. David, N. Grinberg, S. C. Moldoveanu in Advances in Chromatography Volume 54 (Eds.: E. Grushka, N. Grinberg), CRC Press, Boca Raton, 2018, chapter 3.
- ^ Data obtained using molecular dynamics as detailed in the reference and should be compared to 7.9 kJ/mol for bulk water, obtained using the same calculation.Markovitch, Omer; Agmon, Noam (2007). "Structure and energetics of the hydronium hydration shells" (PDF). J. Phys. Chem. A. 111 (12): 2253–2256. Bibcode:2007JPCA..111.2253M. CiteSeerX 10.1.1.76.9448. doi:10.1021/jp068960g. PMID 17388314. Archived from the original (PDF) on 2014-08-13. Retrieved 2017-10-25.
- ^ Biedermann F, Schneider HJ (May 2016). "Experimental Binding Energies in Supramolecular Complexes". Chemical Reviews. 116 (9): 5216–300. doi:10.1021/acs.chemrev.5b00583. PMID 27136957.
- ^ Legon, A. C.; Millen, D. J. (1987). "Angular geometries and other properties of hydrogen-bonded dimers: a simple electrostatic interpretation of the success of the electron-pair model". Chemical Society Reviews. 16: 467. doi:10.1039/CS9871600467.
- ^ Friebolin, H., "Basic One- and Two- Dimensional NMR Spectroscopy, 4th ed.," VCH: Weinheim, 2008. ISBN 978-3-527-31233-7
- ^ Hobza P, Havlas Z (2000). "Blue-Shifting Hydrogen Bonds". Chem. Rev. 100 (11): 4253–4264. doi:10.1021/cr990050q. PMID 11749346.
- ^ a b Feldblum, Esther S.; Arkin, Isaiah T. (2014). "Strength of a bifurcated H bond". Proceedings of the National Academy of Sciences. 111 (11): 4085–4090. Bibcode:2014PNAS..111.4085F. doi:10.1073/pnas.1319827111. PMC 3964065. PMID 24591597.
- ^ Weinhold, Frank; Klein, Roger A. (2014). "What is a hydrogen bond? Resonance covalency in the supramolecular domain". Chemistry Education Research and Practice. 15 (3): 276–285. doi:10.1039/c4rp00030g.
- ^ Sun, C. Q.; Sun, Yi (2016). The Attribute of Water: Single Notion, Multiple Myths. ISBN 978-981-10-0178-9.
- ^ Grunenberg, Jörg (2004). "Direct Assessment of Interresidue Forces in Watson−Crick Base Pairs Using Theoretical Compliance Constants". Journal of the American Chemical Society. 126 (50): 16310–1. doi:10.1021/ja046282a. PMID 15600318.
- ^ Isaacs, E.D.; et al. (1999). "Covalency of the Hydrogen Bond in Ice: A Direct X-Ray Measurement". Physical Review Letters. 82 (3): 600–603. Bibcode:1999PhRvL..82..600I. doi:10.1103/PhysRevLett.82.600.
- ^ Ghanty, Tapan K.; Staroverov, Viktor N.; Koren, Patrick R.; Davidson, Ernest R. (2000-02-01). "Is the Hydrogen Bond in Water Dimer and Ice Covalent?". Journal of the American Chemical Society. 122 (6): 1210–1214. doi:10.1021/ja9937019. ISSN 0002-7863.
- ^ Cordier, F; Rogowski, M; Grzesiek, S; Bax, A (1999). "Observation of through-hydrogen-bond (2h)J(HC') in a perdeuterated protein". J Magn Reson. 140 (2): 510–2. Bibcode:1999JMagR.140..510C. doi:10.1006/jmre.1999.1899. PMID 10497060. S2CID 121429.
- ^ Needham, Paul (2013). "Hydrogen bonding: Homing in on a tricky chemical concept". Studies in History and Philosophy of Science Part A. 44: 51–65. doi:10.1016/j.shpsa.2012.04.001.
- ^ Pauling, L. (1960). The nature of the chemical bond and the structure of molecules and crystals; an introduction to modern structural chemistry (3rd ed.). Ithaca (NY): Cornell University Press. p. 450. ISBN 978-0-8014-0333-0.
- ^ Moore, T. S.; Winmill, T. F. (1912). "The state of amines in aqueous solution". J. Chem. Soc. 101: 1635. doi:10.1039/CT9120101635.
- ^ Latimer, Wendell M.; Rodebush, Worth H. (1920). "Polarity and ionization from the standpoint of the Lewis theory of valence". Journal of the American Chemical Society. 42 (7): 1419–1433. doi:10.1021/ja01452a015.
- ^ a b Jorgensen, W. L.; Madura, J. D. (1985). "Temperature and size dependence for Monte Carlo simulations of TIP4P water". Mol. Phys. 56 (6): 1381. Bibcode:1985MolPh..56.1381J. doi:10.1080/00268978500103111.
- ^ Zielkiewicz, Jan (2005). "Structural properties of water: Comparison of the SPC, SPCE, TIP4P, and TIP5P models of water". J. Chem. Phys. 123 (10): 104501. Bibcode:2005JChPh.123j4501Z. doi:10.1063/1.2018637. PMID 16178604.
- ^ Jencks, William; Jencks, William P. (1986). "Hydrogen Bonding between Solutes in Aqueous Solution". J. Am. Chem. Soc. 108 (14): 4196. doi:10.1021/ja00274a058.
- ^ Dillon, P. F. (2012). Biophysics: A Physiological Approach. Cambridge University Press. p. 37. ISBN 978-1-139-50462-1.
- ^ Baron, Michel; Giorgi-Renault, Sylviane; Renault, Jean; Mailliet, Patrick; Carré, Daniel; Etienne, Jean (1984). "Hétérocycles à fonction quinone. V. Réaction anormale de la butanedione avec la diamino-1,2 anthraquinone; structure cristalline de la naphto \2,3-f] quinoxalinedione-7,12 obtenue". Can. J. Chem. 62 (3): 526–530. doi:10.1139/v84-087.
- ^ Laage, Damien; Hynes, James T. (2006). "A Molecular Jump Mechanism for Water Reorientation". Science. 311 (5762): 832–5. Bibcode:2006Sci...311..832L. doi:10.1126/science.1122154. PMID 16439623. S2CID 6707413.
- ^ Markovitch, Omer; Agmon, Noam (2008). "The Distribution of Acceptor and Donor Hydrogen-Bonds in Bulk Liquid Water". Molecular Physics. 106 (2): 485. Bibcode:2008MolPh.106..485M. doi:10.1080/00268970701877921. S2CID 17648714.
- ^ Shiao-Wei Kuo (2018). Hydrogen Bonding in Polymer Materials. Wiley-VCH.
- ^ Politi, Regina; Harries, Daniel (2010). "Enthalpically driven peptide stabilization by protective osmolytes". ChemComm. 46 (35): 6449–6451. doi:10.1039/C0CC01763A. PMID 20657920.
- ^ Gilman-Politi, Regina; Harries, Daniel (2011). "Unraveling the Molecular Mechanism of Enthalpy Driven Peptide Folding by Polyol Osmolytes". Journal of Chemical Theory and Computation. 7 (11): 3816–3828. doi:10.1021/ct200455n. PMID 26598272.
- ^ Hellgren, M.; Kaiser, C.; de Haij, S.; Norberg, A.; Höög, J. O. (December 2007). "A hydrogen-bonding network in mammalian sorbitol dehydrogenase stabilizes the tetrameric state and is essential for the catalytic power". Cellular and Molecular Life Sciences. 64 (23): 3129–38. doi:10.1007/s00018-007-7318-1. PMID 17952367. S2CID 22090973.
- ^ Fernández, A.; Rogale K.; Scott Ridgway; Scheraga H. A. (June 2004). "Inhibitor design by wrapping packing defects in HIV-1 proteins". Proceedings of the National Academy of Sciences. 101 (32): 11640–5. Bibcode:2004PNAS..10111640F. doi:10.1073/pnas.0404641101. PMC 511032. PMID 15289598.
- ^ Khashayar Rajabimoghadam Yousef Darwish Umyeena Bashir Dylan Pitman Sidney Eichelberger Maxime A. Siegler Marcel Swart Isaac Garcia-Bosch Aerobic Oxidation of Alcohols by Copper Complexes Bearing Redox-Active Ligands with Tunable H-Bonding https://doi.org/10.1021/jacs.8b08748
- ^ Ozeryanskii, Valery A.; Pozharskii, Alexander F.; Bieńko, Agnieszka J.; Sawka-Dobrowolska, Wanda; Sobczyk, Lucjan (2005-03-01). "[NHN]+ Hydrogen Bonding in Protonated 1,8-Bis(dimethylamino)-2,7-dimethoxynaphthalene. X-ray Diffraction, Infrared, and Theoretical ab Initio and DFT Studies". The Journal of Physical Chemistry A. 109 (8): 1637–1642. Bibcode:2005JPCA..109.1637O. doi:10.1021/jp040618l. ISSN 1089-5639. PMID 16833488.
- ^ a b Crabtree, Robert H.; Siegbahn, Per E. M.; Eisenstein, Odile; Rheingold, Arnold L.; Koetzle, Thomas F. (1996). "A New Intermolecular Interaction: Unconventional Hydrogen Bonds with Element-Hydride Bonds as Proton Acceptor". Acc. Chem. Res. 29 (7): 348–354. doi:10.1021/ar950150s. PMID 19904922.
- ^ Cowan ML; Bruner BD; Huse N; et al. (2005). "Ultrafast memory loss and energy redistribution in the hydrogen bond network of liquid H2O". Nature. 434 (7030): 199–202. Bibcode:2005Natur.434..199C. doi:10.1038/nature03383. PMID 15758995. S2CID 4396493.
- ^ a b Luo, Jiangshui; Jensen, Annemette H.; Brooks, Neil R.; Sniekers, Jeroen; Knipper, Martin; Aili, David; Li, Qingfeng; Vanroy, Bram; Wübbenhorst, Michael; Yan, Feng; Van Meervelt, Luc; Shao, Zhigang; Fang, Jianhua; Luo, Zheng-Hong; De Vos, Dirk E.; Binnemans, Koen; Fransaer, Jan (2015). "1,2,4-Triazolium perfluorobutanesulfonate as an archetypal pure protic organic ionic plastic crystal electrolyte for all-solid-state fuel cells". Energy & Environmental Science. 8 (4): 1276. doi:10.1039/C4EE02280G. S2CID 84176511.
- ^ Lipinski CA (December 2004). "Lead- and drug-like compounds: the rule-of-five revolution". Drug Discovery Today: Technologies. 1 (4): 337–341. doi:10.1016/j.ddtec.2004.11.007. PMID 24981612.
Further reading
- George A. Jeffrey. An Introduction to Hydrogen Bonding (Topics in Physical Chemistry). Oxford University Press, USA (March 13, 1997). ISBN 0-19-509549-9
External links
- The Bubble Wall (Audio slideshow from the National High Magnetic Field Laboratory explaining cohesion, surface tension and hydrogen bonds)
- isotopic effect on bond dynamics