User:Amohamed7/sandbox
Xenopus laevis Development
[edit]Introduction
[edit]Development is highly conserved process, which includes fertilization, cleavage, gastrulation, neurulation, and axis formation. Each of these phases are required in order for a species to develop from a single egg cell, into a fully functioning multi-cellular adult. While this process is highly conserved, there are variations in the mechanisms used by species to undergo each phase. This article will focus on the overall development Xenopus laevis, and the mechanisms used by the African clawed frog during each phase of development.
Life Cycle
[edit]The life cycle of Xenopus takes roughly twelve months from start to finish.[1] That is, from fertilization to adulthood. It is important to note that the earliest stages of the frogs’ life cycle only take about 24 hours to complete, it is the unique process of metamorphosis that takes the longest[1]. The first stage of the life cycle is fertilization, this is done in vitro[1]. Following fertilization is cleavage, which separation the egg through multiple rounds of cell division and helps establish the poles [1]. The third step in development is gastrulation, during this time the cells move to their proper locations to establish the three germ layers, this helps set up the embryo for neurulation[1]. Neurulation utilizes the results of the cell movement from gastrulation to set up the neural tube[1]. Organogenesis is the next step of development. It draws from the other stages because it requires the organizer, nervous system, and the three germ layers which were established during gastrulation.[2] These respond to signalling from the organizers and set-up the organ systems[2]. Research shows that there are cells that support the germ layers in forming the organs during this time; these cells come from the immune system and help with setting up vital components such as the neural plate and kidneys[3]. It also seems that the cells help in the preceding steps of setting up the mesoderm[3]. Metamorphosis is the last stage of the developmental process and is a defining moment for Xenopus. The representative characteristics of the tadpole, such as the tail and gills, shrink and are replaced by arms, legs, and useful lungs[2]. TR hormones have been found to play an active role in the changes that occur during metamorphosis[4]. Depending on the stage that Xenopus is in, they are found in the head, middle, and tail regions of the changing tadpole both before and after the change[4].
Fertilization
[edit]Fertilization of the Xenopus egg is the first step in it's life cycle. In the lab the egg is fertilized in vitro, a process in which the egg is directly injected with Xenopus sperm. In the wild however the eggs are fertilized as they are leaving the cloaca. [1]
The egg is coated with several jelly layers including the vitelline envelope, that is later involved in the prevention of polyspermy. Sperm enters the egg by a reaction between its acrosome and the vitelline layer. This reaction is due to the response of a glycoprotein found on the eggs outer membrane that aids in the binding of the sperm to itself. Research has proven that it is a sperm surface glycoprotein (SGP) that establishes this bond through the receptors gp69/64(ZPA) and gp41(ZPC) found on the egg membranes surface. Certain proteases have also been linked to this binding in recent studies but they have not yet been repeated enough to show conclusive evidence. SGP binding is found mostly on the animal hemisphere of the egg, which confirms the theory of entry being dominantly on the animal hemisphere instead of the vegetal hemisphere [5].
Calcium plays a role in the fertilization of the Xenopus. High levels of extracellular calcium is the prerequisite for the blocking of polyspermy, exiting the meiotic phase and nuclear formation along with other cellular reactions. While an increase in the intracellular calcium leads to the release of the second polar body and the exocytosis of cortical granules near the eggs surface raising the vitelline layer allowing the egg to rotate freely (cortical rotation) bring the animal hemisphere into an upright position [6].
Cleavage
[edit]Cleavage refers to a set of early cell divisions, these divisions occur without cellular growth and yield multiple smaller cells originating from one cell.[1] Cleavage in Xenopus occurs in an orderly manner and the first cleavage splits the egg vertically down the middle, into anterior and posterior halves.[1][7][8] The second cleavage occurs roughly perpendicular to the axis of the first and divides the egg into dorsal and ventral halves.[1][7][8] Cleavage continues and around the 32 cell stage, the formation of the blastocoel is observed, the blastocoel is a cavity that forms in the Animal hemisphere.[1] When the formation of the blastocoel is noted, the embryo is referred to as a blastula.[1] Cells composing the blastula are joined by cadherins, which are calcium dependent adhesion's, and thus the removal of Ca2+ degrades the cadherins.[1] Cleavage continues until the mid-blastula transition, this prompts for a decrease in cleavage divisions and promotes transcription of the zygotic genome.[1]

Intracellular Ca2+ is essential for cleavage to occur in Xenopus,[9] Evidence indicates that cell cleavage involves narrowing of the region in which the cleavage furrow is located.[9] The mechanism involved in the contraction of the area has been associated with that of muscle, and is thought to contain an actin-myosin based structure.[9] This structure utilizes calcium, thus Ca2+ is required in order for cleavage to occur.[9]
The formation of the organizer (Spemann's organizer) during the final cleavage divisions is a crucial step required for the remaining phases of development.[1][10] The organizer is an area located in the posterior/dorsal area of the embryo, and is responsible for expression of many genes involved in axis formation.[1] This area is crucial in gastrulation, as it forms the area known as the dorsal lip of the blastopore.[1][10]
Gastrulation
[edit]Gastrulation is a phase of development where the three embryonic germ layers (endoderm, mesoderm, and ectoderm) are constructed through various mechanisms of morphogenic cell movement. This stage is initiated at the blastopore of the dorsal lip, which is formed on the opposite side of the embryo from where the sperm enters. The dorsal lip is part of the blastopore located below the Spemann's organizer that becomes elongated and eventually circularized.[1] The blastopore is the point of access for the outer cells to enter the embryo. The cells around the blastopore undergo apical constriction and are referred to as bottle cells because of their shape. Invagination occurs at the blastopore. This is where cell sheets of the marginal zone fold inward in order to construct a pocket. This pocket develops into a fluid-filled cavity located in the middle of the embryo referred to as a blastocoel.
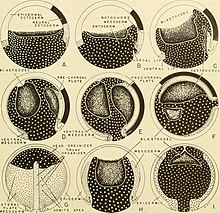
Multiple types of morphogenic cell movement are involved throughout gastrulation. These processes do not rely on one another; however, the overall result of their actions drives the differentiation of the germ layers. Epiboly, invagination, involution, and convergent extension are clearly seen in Xenopus gastrulation. Epiboly can be seen in the animal hemisphere. These cells flatten and spread out to increase the surface area of the cell sheet in order to cover the whole surface of the embryo. The outer cells move towards the blastopore of the dorsal lip causing the marginal zone to invaginate, creating a circular blastopore. During invagination, the blastocoel moves up towards the top of the animal pole, away from the dorsal lip, and subsequently down to the vegetal pole. While doing this, its size decreases due to the infiltrating cells. Eventually, the blastocoel shrinks and the archenteron, which is the early structure of the gut, becomes the principle cavity. Because the mesoderm is already located on the inside of the gastrula, involution allows it to separate into a whole different layer forming between the endoderm and the ectoderm. Involution is a process where a cell sheet folds inward, forming an underlying layer of cells (mesoderm).[1] The cells are able to move and construct a three layered gastrula due to these different, independent morphogenic cell movements.
Convergent extension is a process where cells line up based on their polarity and move between neighboring cells through the use of lamellapodia, generating shape change at the tissue level. Cellular intercalation occurs in all three germ layers which moves the marginal zone internally, further closing the blastocoel. Convergent extension is one of the more well-studied cellular movements involved in gastrulation. The Wnt/β-catenin pathway is necessary for convergent extension to occur. Proper execution of this pathway leads to dorsal cell specification.[11] The Xnr-3 protein is a major target of this pathway and if it is not expressed correctly, then the cells do not undergo proper convergent extension.[11] Paraxial protocadherin (PAPC) plays an important role in cell movement as well regarding planar cell polarity and the Wnt pathway. It is dependent on three GTP proteins RhoA, Rac1, and JNK.[12]
The end of gastrulation results in a structure called a gastrula. The three embryonic germ layers are now differentiated in the embryo. The innermost layer is the endoderm that was originally the vegetal tissue. The outermost layer is the ectoderm, which was once the animal cap. The middle layer is the mesoderm that was formed from the involution of the marginal zone.[1]
Neurulation
[edit]
The next phase of development is neurulation where the neural tube is formed giving rise to the central nervous system. During this stage, the embryo is referred to as a neurula. Neurulation is initiated when ectodermal cells thicken, forming the neural plate. These cells change in shape from cuboidal to columnar, causing the edges of the plate, referred to as neural folds, to rise towards one another because of the constriction of F-actin during apical constriction.[13] As these boundaries move, the middle of the neural plate descends to form the neural groove. The neural tube forms when these two folds meet to mold a hollow cylinder that becomes covered with a layer of ectoderm known as the epidermis. Deep cells, which are non-neural cells, help bring the two folds together.[13] The Wnt pathway also plays a critical role in these cell movements. First, Wnt needs to inhibit BMP in the gastrula stage. Subsequently, BMP needs to be activated in the neurula for proper neural crest formation.[14] Sox2 is shown to be consistently expressed throughout neurulation.[1] There are hinge points located along the neural tube that aid in closing it. This happens almost simultaneously.[13] These hinge points allow the neural tube to "zip up" and close. Convergent extension plays a critical role in this process and is consistently being carried out, especially in the notochord, resulting in the elongation of the entire body.
Axis Formation
[edit]All axis formation is centered around Spermann's organizer, which is located on the dorsal side of the developing embryo[1]. The organizer is set up in this location because of the Nieuwkoop center. Axis formation is important to the development of Xenopus because without it the frog would lack proper body formation and more than likely would not survive.
Dorsal/Ventral
[edit]The dorsal and ventral body axis are defined during one of the earliest stages of development, cleavage[1]. It is set up mainly two by organizers. The first is Wnt, which signals on the dorsal side of the embryo; it does this by interacting constructively with beta-catenin[1] The other organizer responsible for setting up this axis is BMP, which is found on the ventral side of the embryo[1].
Research has been done on the specific forms of Wnt within the embryo and during the setup of the axis. It has been found that Wnt11 work in conjunction with one another to establish the dorsal side of the embryo[15]. It has also been found that Dkk1 assists the two forms of Wnt in influencing only where they are supposed to go[15]. This is achieved by it repressing and initiating the function of Wnt[15].
Anterior/Poster
[edit]Establishing the anterior/posterior axis is important because each side has specific roles in setting up crucial body systems, such as the notochord in the posterior[1]. They utilize similar organizers as the dorsal/ventral axis. Wnt is found in the posterior side of the embryo, it is assisted by Fgf[1]. These work together in Hox gene expression[1]. Noggin is what assists the anterior axis. It is important because it suppresses BMP, which stalls anterior axis set-up[1].
Left/Right
[edit]The final axis that is present in the developing embryo is the left and right axis. This axis is unique from the others because it is regulated primarily by rotating cilia, whereas the others are set up by repression/expression of organizers. Pitx2 is a left signaling organizer, and as mentioned, it is set up on the left side because of the rotating of the cilia[1]. The cilia are in the node and as it receives the Pitx2 from the organizer is carries it over[1].
Research has been done on the importance of the placement of the cilia for the equal spreading of the organizer, and therefore the proper setup of the axis. The cilia traditionally develop on the posterior side of the embryo, and carry out their function from there. They receive the signals that they need to develop in this area from Vangl2[16]. It was discovered that if the Vangl2 levels were not held at steady amounts, the cilia grew in a more central location[16]. This completely upsets the spread of Pitx2 and therefore causes the left and right axis to form incorrectly, or not at all[16].
Advantages and Disadvantages
[edit]Advantages to using Xenopus are as follows. They have large embryo which aids in the process of micro-manipulation, they also lay a large number of eggs and the cost and maintenance for these embryos is relatively low. However, Xenopus is not a very useful tool when it comes to genetics. The only known genetic inventory known at the moment is X.tropicalis[1][17].
- ^ a b c d e f g h i j k l m n o p q r s t u v w x y z aa ab ac ad ae af 1949-, Slack, J. M. W. (Jonathan Michael Wyndham), (2013). Essential developmental biology (3rd ed ed.). Chichester, West Sussex: Wiley. ISBN 9780470923511. OCLC 785558800.
{{cite book}}
:|edition=
has extra text (help);|last=
has numeric name (help)CS1 maint: extra punctuation (link) CS1 maint: multiple names: authors list (link) - ^ a b c Gilbert, Scott F. (2000). "The Frog Life Cycle".
{{cite journal}}
: Cite journal requires|journal=
(help) - ^ a b Mclin, Valerie A.; Hu, Cheng-Hui; Shah, Rina; Jamrich, Milan (2002-12-01). "Expression of complement components coincides with early patterning and organogenesis in Xenopus laevis". The International Journal of Developmental Biology. 52 (8): 1123–1133. doi:10.1387/ijdb.072465v. ISSN 0214-6282.
- ^ a b Kawahara, A.; Baker, B. S.; Tata, J. R. (August 1991). "Developmental and regional expression of thyroid hormone receptor genes during Xenopus metamorphosis". Development (Cambridge, England). 112 (4): 933–943. ISSN 0950-1991. PMID 1935702.
- ^ Nagai, Keita; Ishida, Takuya; Hashimoto, Takafumi; Harada, Yuichirou; Ueno, Shuichi; Ueda, Yasushi; Kubo, Hideo; Iwao, Yasuhiro (2009-06-01). "The Sperm-surface glycoprotein, SGP, is necessary for fertilization in the frog, Xenopus laevis". Development, Growth & Differentiation. 51 (5): 499–510. doi:10.1111/j.1440-169x.2009.01112.x. ISSN 1440-169X.
- ^ Tokmakov, Alexander; Stefanov, Vasily; Iwasaki, Tetsushi; Sato, Ken-Ichi; Fukami, Yasuo (15 October 2014). "Calcium Signaling and Meiotic Exit at Fertilization in Xenopus Egg". International Journal of Molecular Sciences. 15 (10): 18659–18676. doi:10.3390/ijms151018659.
{{cite journal}}
: CS1 maint: unflagged free DOI (link) - ^ a b Chalmers, Andrew D.; Strauss, Bernhard; Papalopulu, Nancy (2003-06-15). "Oriented cell divisions asymmetrically segregate aPKC and generate cell fate diversity in the early Xenopus embryo". Development. 130 (12): 2657–2668. doi:10.1242/dev.00490. ISSN 0950-1991. PMID 12736210.
- ^ a b Strome, Susan. "Determination of cleavage planes". Cell. 72 (1): 3–6. doi:10.1016/0092-8674(93)90041-n.
- ^ a b c d Baker, P. F.; Warner, A. E. (May 1972). "Intracellular calcium and cell cleavage in early embryos of Xenopus laevis". The Journal of Cell Biology. 53 (2): 579–581. ISSN 0021-9525. PMC 2108729. PMID 5063471.
{{cite journal}}
: CS1 maint: PMC format (link) - ^ a b De Robertis, Edward M. (2006/04). "Spemann's organizer and self-regulation in amphibian embryos". Nature Reviews Molecular Cell Biology. 7 (4): 296–302. doi:10.1038/nrm1855. ISSN 1471-0080.
{{cite journal}}
: Check date values in:|date=
(help) - ^ a b Kühl, Michael; Geis, Karin; Sheldahl, Laird C; Pukrop, Tobias; Moon, Randall T; Wedlich, Doris. "Antagonistic regulation of convergent extension movements in Xenopus by Wnt/β-catenin and Wnt/Ca2+ signaling". Mechanisms of Development. 106 (1–2): 61–76. doi:10.1016/s0925-4773(01)00416-6.
- ^ Unterseher, Frank; Hefele, Jeers (August 18, 2004). "Paraxial protocadherin coordinates cell polarity during convergent extension via Rho A and JNK". The Embo Journal. 23: 3259–3269 – via MEDLINE.
- ^ a b c Inoue, Yasuhiro; Suzuki, Makoto; Watanabe, Tadashi; Yasue, Naoko; Tateo, Itsuki; Adachi, Taiji; Ueno, Naoto (2016-12-01). "Mechanical roles of apical constriction, cell elongation, and cell migration during neural tube formation in Xenopus". Biomechanics and Modeling in Mechanobiology. 15 (6): 1733–1746. doi:10.1007/s10237-016-0794-1. ISSN 1617-7959.
- ^ Steventon, Ben; Araya, Claudio; Linker, Claudia; Kuriyama, Sei; Mayor, Roberto (2009-03-01). "Differential requirements of BMP and Wnt signalling during gastrulation and neurulation define two steps in neural crest induction". Development. 136 (5): 771–779. doi:10.1242/dev.029017. ISSN 0950-1991. PMID 19176585.
- ^ a b c Cha, Sang-Wook; Tadjuidje, Emmanuel; Tao, Qinghua; Wylie, Christopher; Heasman, Janet (2008-11-15). "Wnt5a and Wnt11 interact in a maternal Dkk1-regulated fashion to activate both canonical and non-canonical signaling in Xenopus axis formation". Development. 135 (22): 3719–3729. doi:10.1242/dev.029025. ISSN 0950-1991. PMID 18927149.
- ^ a b c Antic, Dragana; Stubbs, Jennifer L.; Suyama, Kaye; Kintner, Chris; Scott, Matthew P.; Axelrod, Jeffrey D. (2010-02-02). "Planar Cell Polarity Enables Posterior Localization of Nodal Cilia and Left-Right Axis Determination during Mouse and Xenopus Embryogenesis". PLOS ONE. 5 (2): e8999. doi:10.1371/journal.pone.0008999. ISSN 1932-6203.
{{cite journal}}
: CS1 maint: unflagged free DOI (link) - ^ Schneider, Patricia N.; Hulstrand, Alissa M.; Houston, Douglas W. (2010-11-02). "Fertilization of Xenopus oocytes using the Host Transfer Method". Journal of Visualized Experiments (45). doi:10.3791/1864. ISSN 1940-087X.