User:Zoopermarket/sandbox
The measurement techniques needed to make the measurements in nuclear fusion experiments are usually referred to as fusion diagnostics, a fusion jargon term commonly used by fusion researchers.
The objective of fusion diagnostics is to characterise the fusion plasma under study. This article covers the subject of fusion diagnostics. Please, refer elsewhere for the more general subject of plasma diagnostics.
Introduction[edit]
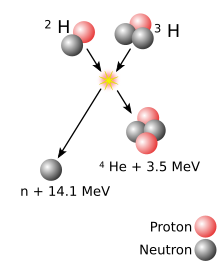
Fusion binds light nuclei to form heavier nuclei with a large release of energy. Fusion powers the sun and all the stars in our universe. Theoretical understanding of fusion began in the first half of the 20th century by Albert Einstein among others. He then wrote arguably one of the most famous formula of physics:
Mass is transformed into energy and vice-versa. This was a milestone in the history of theoretical physics.
For example,~ 600 million tons of hydrogen are burnt into helium every second in our sun. This process, called nuclear fusion, releases a huge amount of energy, large enough for a fraction of it to reach us and sustain life on our planet.
If fusion works so well in the sun and the stars, could we reproduce it and harness it on earth?
If we could, an abundant, clean, safe and sustainable new energy source would become available to satisfy all our needs.
This is the ambitious goal of fusion research. It has proven immensely challenging so far.
Fusion on earth could be made between heavy hydrogen - deuterium - and super heavy hydrogen - tritium - using the following reaction:
This is the easiest way. This fusion reaction has the highest cross section of ~5 barns (one barn =10-28m²) and releases a neutron and an alpha particle.
The neutron has two roles. First the tritium is not an element found in nature and it must be produced. The neutrons produce tritium via a process called tritium breeding. Second, the neutron transports most of the fusion energy (~80%) outward. The role of alpha particles is to sustain the conditions necessary for the fusion process to continue.
There are two basic approaches to the realization of fusion: Inertial confinement and magnetic confinement.

In Inertial confinement fusion, the fuel is in the form of solid deuterium-tritium capsule. In order to start the fusion process, it is compressed to extremely high pressure by means of intense energy beams.
In magnetic confinement fusion, the fuel is in the form of deuterium tritium gas mixture. It is heated up to very high temperatures of about ~ 150 million degree C – hotter than in the centre of our sun. If a gas is hot enough, it splits up into charged particles and becomes a plasma. The plasma particles, ions positively charged ions and negatively charged electrons, are not tied together as neutral atoms anymore. They move about in a complex way. Although the basic physical processes are simpler than in ordinary gases, the motions in a plasma are more complex since these are coupled to the electromagnetic field. A plasma thus obeys the laws of magnetohydrodynamics which combine Maxwell's equations and fluid mechanics equations.

The pressure of a hot plasma can be balanced using powerful magnets. Fusion magnetic confinement makes use of an external magnetic field to force the plasma particles move along specific trajectories. To ensure these trajectories are enclosed within a finite volume, a suitable magnetic field geometry must be realized.
This article, in its current form, discusses mainly diagnostics for magnetic confinement fusion research.
Tokamak[edit]
The tokamak is a kind of magnetic bottle which insulates an extremely hot plasma from the plasma container walls.
Let us take a cylinder with a magnetic field on its axis. The cylinder's axial magnetic field creates a Lorentz force (vXB).This force holds the particles in a plane perpendicular to the cylinder's axis. Thus, 2-D confinement is realized in that way. However particles are still free to move away in the direction along the cylinder axis. If this cylinder is now bent into a torus, particles turn around the torus and a full 3-D confinement is achieved. This is basically the principle of magnetic toroidal confinement and a tokamak works on this principle. In a tokamak, big magnets produce a toroidal magnetic field. The plasma itself is a conductor in which flows a current of up to millions of amperes. This plasma current induces in turn another field, the poloidal magnetic field. The combination of the former and the latter fields produces twisted helical field lines which insulate the plasma from the walls.

Confinement scaling[edit]
The tokamak is the best magnetic bottle to date because it has achieved the best confinement results. The quality of plasma confinement is measured by a energy confinement time. The energy confinement time is an important measure of success for fusion research. It is not possible to predict the energy confinement time from first principles. The MHD equations governing the plasma dynamic are a set of non-linear partial differential equations for which no straightforward mathematical techniques exist. Therefore, these equations cannot be resolved in general. Instead, an empirical approach is applied. The results of decades of fusion experiments were accumulated to form a statistical basis for the predicted confinement performance of tokamaks in terms of scaling laws such as this one below[1]
In this formula, the confinement time in seconds is expressed as a power law of a plasma current , a toroidal magnetic field B_{T} (T), a heating Power P (MW), an electron density n_e(10^{19}m-3),atomic mass M(AMU), R major radius (m), inverse aspect ratio \epsilon (a/R),\Kappa_a a plasma cross-sectional area.One notes that the nearly square dependence on R, explains why fusion research builds larger and larger tokamaks to improve confinement results.
JET and ITER[edit]
Since the first tokamak T1 worked in 1957 at the Kurchatov institute in Moscow, Russia, many tokamaks were built across the world. First, they were small experiments that even universities could afford. Soon they became larger and larger, more complex, including more and more auxiliary equipment and scientific apparatus coming from most of disciplines of experimental physics and therefore it became too expensive to do it alone in one country. Then Europeans built together the JET Joint European Torus near Oxford in UK. JET is presently the largest tokamak in the world. It holds the record of the highest fusion power produced so far (~ 16 MW) with a ratio of fusion power to input power of ~ 0.8. Then, the EU , China, US, Russia , Japan, South Korea and India decided in xxx to build together the ITER tokamak, a big international project in Cadarache, in the South of France. ITER is currently the largest international scientific venture after the International space station. If succesfull, ITER will be the first tokamak to produce a large amount (on industrial scale) of fusion energy for several minutes and should demonstrate the scientific and technical feasibility of fusion.
- What are the alternatives to tokamak?
Beside the inertial confinement mentioned in the paragraph above, research into magnetic confinement systems other than tokamaks is being pursued albeit on a much smaller scale. The stellarator is the most serious competitor. In the case of the stellarator, the system of helical field lines is permanent and is produced by external magnets having an intricate geometry thus enabling the stellarator to run a plasma continuously.
Magnetics[edit]
Neutron systems[edit]
Optical systems[edit]
Plasma probes[edit]
Notes[edit]
References[edit]
- ^ ITER PHYSICS BASIS EDITORS (1999). "ITER Physics Basis". Nucl. Fusion. 39: 2137.