Marine sediment: Difference between revisions
m Robot: Fixing double redirect to Pelagic sediment |
Epipelagic (talk | contribs) start article, including copy from marine microorganism – see that page's history for attribution Tag: Removed redirect |
||
Line 1: | Line 1: | ||
'''Marine sediments''' are deposits of insoluble particles that accumulate on the seafloor. These have their origins in soil and rocks which have been transported from the land to the sea, mainly by rivers but also as windbourne as dust and by the flow of glaciers into the ocean. Additional deposits come from marine organisms and chemical precipitation in seawater, as well as underwater volcanoes and debris from meteorites. |
|||
#REDIRECT [[Pelagic sediment]] |
|||
==History== |
|||
The first major study of deep-ocean sediments occurred between 1872 and 1876 with the [[Challenger expedition|HMS ''Challenger'' expedition]]. |
|||
==Classification== |
|||
===By size=== |
|||
The Wentworth Scale classifies sediment by grain size. Clay sediments are the smallest with a grain diameter of less than .004 mm and boulders are the largest with grain diameters of 256 mm or larger.<ref name=NOAA>[ftp://ftp.library.noaa.gov/noaa_documents.lib/NMFS/NEMO_Curriculum/Lesson15/Lesson15_Teacher_Guide.pdf Sediments] ''[[NOAA]]''. Accessed 5 April 2021. {{PD-notice}}</ref> |
|||
===By origin=== |
|||
Sediments are also classified by origin. There are four types: [[Wiktionary:lithogenous|lithogenous]], [[Wiktionary:hydrogenous|hydrogenous]], [[Wiktionary:biogenous|biogenous]] and [[Wiktionary:hydrogenous|cosmogenous]]. |
|||
Lithogenous sediments come from land via rivers, ice, wind and other processes. Biogenous sediments come from organisms like plankton when their exoskeletons break down.Hydrogenous sediments come from chemical reactions in the water. Cosmogenous sediments come from space, filtering in through the atmosphere or carried to Earth on meteorites.<ref name=NOAA /> |
|||
==Marine microfossils== |
|||
[[File:Marine sediment thickness (cropped).jpg|thumb|upright=1.4| {{center|Thickness of marine sediments}}]] |
|||
{{see also|Marine biogeochemical cycles}} |
|||
{{further|Calcareous ooze|Biogenic silica|Radiolarite|Sedimentary rock|Microfossil|Reverse weathering}} |
|||
Sediments at the bottom of the ocean have two main origins, terrigenous and biogenous. |
|||
[[Terrigenous sediment]]s account for about 45% of the total marine sediment, and originate in the erosion of [[Rock (geology)|rocks]] on land, transported by rivers and land runoff, windborne dust, volcanoes, or grinding by glaciers. |
|||
[[Biogenous sediment]]s account for the other 55% of the total sediment, and originate in the skeletal remains of [[#Marine protists|marine protists]] (single-celled plankton and benthos microorganisms). Much smaller amounts of precipitated minerals and meteoric dust can also be present. ''Ooze'', in the context of a marine sediment, does not refer to the consistency of the sediment but to its biological origin. The term ooze was originally used by [[John Murray (oceanographer)|John Murray]], the "father of modern oceanography", who proposed the term ''radiolarian ooze'' for the silica deposits of radiolarian shells brought to the surface during the [[Challenger Expedition]].<ref>Thomson, Charles Wyville (2014) [https://books.google.co.nz/books?id=zcFkAwAAQBAJ&printsec=frontcover&dq=%22Voyage+of+the+Challenger+:+The+Atlantic%22&hl=en&sa=X&ved=0ahUKEwjsi8aKzejkAhWOfysKHf6MB10Q6AEINTAC#v=onepage&q=radiolarian%20ooze&f=false ''Voyage of the Challenger : The Atlantic''] Cambridge University Press, page235. |
|||
{{ISBN|9781108074759}}.</ref> A ''biogenic ooze'' is a [[pelagic sediment]] containing at least 30 percent from the skeletal remains of marine organisms. |
|||
{{clear}} |
|||
{|class="wikitable" |
|||
! colspan=9 |{{centre|Main types of biogenic ooze}} |
|||
|- |
|||
! type |
|||
! mineral<br />forms |
|||
! protist<br />involved |
|||
! |
|||
! name of skeleton |
|||
! width=100px | typical size<br /><small>(mm)</small> |
|||
! colspan=2 | |
|||
|- |
|||
! width=90px rowspan=2 | [[Siliceous ooze]] |
|||
| rowspan=2 align=center | [[Silicon oxide|SiO<sub>2</sub>]]<br />[[silica]]<br />[[quartz]]<br />[[glass]]<br />[[opal]]<br />[[chert]] |
|||
| [[diatom]] |
|||
| style="background:#000000;"| [[File:Lyrella hennedy 1600x contrast invertion.jpg|90px]] |
|||
| [[frustule]] |
|||
| 0.002 to 0.2<ref name="HasleSyvertsen1996">{{cite book|author1=Grethe R. Hasle|author2=Erik E. Syvertsen |author3=Karen A. Steidinger |author4=Karl Tangen|editor=Carmelo R. Tomas|title=Identifying Marine Diatoms and Dinoflagellates|chapter-url=https://books.google.com/books?id=KQxPtwonlqoC|access-date=2013-11-13|date=1996-01-25|publisher=Academic Press|isbn=978-0-08-053441-1|pages=5–385|chapter=Marine Diatoms}}</ref> |
|||
| [[File:Stephanopyxis grunowii.jpg|100px]] |
|||
| diatom [[microfossil]] from 40 million years ago |
|||
|- |
|||
| [[radiolarian]] |
|||
| style="background:#000000;"| [[File:Calocycloma sp. - Radiolarian (32163186535).jpg|90px]] |
|||
| [[Test (biology)|test]] or shell |
|||
| 0.1 to 0.2 |
|||
| [[File:Radiolarian - Heliodiscus umbonatus (Ehr.), Haeckel (28187768550).jpg|100px]] |
|||
| elaborate silica shell of a radiolarian |
|||
|- |
|||
! rowspan=3 | [[Calcareous ooze]] |
|||
| rowspan=3 align=center | [[Calcium carbonate|CaCO<sub>3</sub>]]<br />[[calcite]]<br />[[aragonite]]<br />[[limestone]]<br />[[marble]]<br />[[chalk]] |
|||
| [[foraminiferan]] |
|||
| style="background:#000000;"| [[File:Foram-globigerina hg.jpg|90px]] |
|||
| test or shell |
|||
| under 1 |
|||
| [[File:Globigerina.png|100px]] |
|||
| [[Calcified]] [[Test (biology)|test]] of a planktic foraminiferan. There are about 10,000 living species of foraminiferans<ref name="adl2007">{{cite journal | last1 = Ald | first1 = S.M. | display-authors = etal | year = 2007 | title = Diversity, Nomenclature, and Taxonomy of Protists | url = http://myweb.dal.ca/jmarchib/2007.Adl.Syst.biol.pdf | archive-url = https://wayback.archive-it.org/all/20110331223739/http://myweb.dal.ca/jmarchib/2007.Adl.Syst.biol.pdf | url-status = dead | archive-date = 31 March 2011 | journal = Syst. Biol. | volume = 56 | issue = 4 | pages = 684–689 | doi = 10.1080/10635150701494127 | pmid = 17661235 | access-date = 11 October 2019 }}</ref> |
|||
|- |
|||
| [[coccolithophore]] |
|||
| style="background:#000000;"| [[File:Coccolithus pelagicus 2.jpg|90px]] |
|||
| [[coccolith]]s |
|||
| under 0.1<ref name=Moheimani2012>{{citation |journal=[[Algal Research]] |volume=1 |issue=2 |year=2012 |pages=120–133 |title=Bioremediation and other potential applications of coccolithophorid algae: A review. . Bioremediation and other potential applications of coccolithophorid algae: A review |first1=N.R. |last1=Moheimani |first2=J.P. |last2=Webb |first3= M.A. |last3=Borowitzka |doi=10.1016/j.algal.2012.06.002}}</ref> |
|||
| [[File:CSIRO ScienceImage 7202 SEM Coccolithophorid.jpg|100px]] |
|||
| Coccolithophores are the largest global source of biogenic calcium carbonate, and significantly contribute to the global carbon cycle.<ref>{{cite journal | last1 = Taylor | first1 = A.R. | last2 = Chrachri | first2 = A. | last3 = Wheeler | first3 = G. | last4 = Goddard | first4 = H. | last5 = Brownlee | first5 = C. | year = 2011 | title = A voltage-gated H+ channel underlying pH homeostasis in calcifying coccolithophores | journal = PLOS Biology | volume = 9 | issue = 6| page = e1001085 | doi = 10.1371/journal.pbio.1001085 | pmid = 21713028 | pmc = 3119654 }}</ref> They are the main constituent of chalk deposits such as the [[white cliffs of Dover]]. |
|||
|- |
|||
|} |
|||
<gallery mode=packed heights=160px style=float:left;> |
|||
File:Diatomaceous Earth BrightField.jpg|[[Diatomaceous earth]] is a soft, [[siliceous]], [[sedimentary rock]] made up of microfossils in the form of the [[frustule]]s (shells) of single cell [[diatoms]] (click to magnify) |
|||
File:PSM V44 D483 Globigerina ooze.jpg|Illustration of a ''[[Globigerina]]'' ooze |
|||
File:FMIB 47660 Shells from Globigerina Ooze.jpeg|Shells ([[Test (biology)|tests]]), usually made of calcium carbonate, from a [[foraminifera]]l ooze on the deep ocean floor |
|||
</gallery> |
|||
[[File:Ötzi the Iceman - Dagger 2.png|thumb|upright=1.4| Stone dagger of [[Ötzi|Ötzi the Iceman]] who lived during the [[Copper Age]]. The blade is made of [[chert]] containing radiolarians, calcispheres, calpionellids and a few sponge spicules. The presence of [[calpionellids]], which are extinct, was used to date this dagger.<ref>{{cite journal | last1 = Wierer | first1 = U. | last2 = Arrighi | first2 = S. | last3 = Bertola | first3 = S. | last4 = Kaufmann | first4 = G. | last5 = Baumgarten | first5 = B. | last6 = Pedrotti | first6 = A. | last7 = Pernter | first7 = P. | last8 = Pelegrin | first8 = J. | year = 2018 | title = The Iceman's lithic toolkit: Raw material, technology, typology and use | journal = PLOS ONE | volume = 13 | issue = 6| page = e0198292 | doi = 10.1371/journal.pone.0198292 | pmid = 29924811 | pmc = 6010222 | bibcode = 2018PLoSO..1398292W }}</ref>]] |
|||
<gallery mode=packed heights=160px style=float:left;> |
|||
File:Coober Pedy Opal Doublet.jpg| Opal can contain protist microfossils of diatoms, radiolarians, silicoflagellates and ebridians <ref name=Haq1998>Haq B.U. and Boersma A. (Eds.) (1998) [https://books.google.co.nz/books?id=0XezCm7IwpUC&printsec=frontcover&dq=%22Introduction+to+Marine+Micropaleontology%22&hl=en&sa=X&ved=0ahUKEwjf9oD5psznAhXEYysKHbDXA2UQ6AEIKTAA#v=onepage&q=%22Introduction%20to%20Marine%20Micropaleontology%22&f=false ''Introduction to Marine Micropaleontology''] Elsevier. {{ISBN|9780080534961}}</ref> |
|||
File:MarmoCipollino FustoBasMassenzioRoma.jpg| Marble can contain protist microfossils of foraminiferans, coccolithophores, calcareous nannoplankton and algae, [[ostracode]]s, [[pteropod]]s, calpionellids and [[bryozoa]] <ref name=Haq1998 /> |
|||
File:Carbonate-Silicate Cycle (Carbon Cycle focus).jpg|[[Carbonate-silicate cycle]] |
|||
</gallery> |
|||
{{clear}} |
|||
[[File:Nanoplankton-fossil-sediment hg.jpg|thumb|upright=1.6|left| {{center| Calcareous microfossils from marine sediment consisting mainly of star-shaped [[discoaster]] with a sprinkling of coccoliths}}]] |
|||
[[File:Distribution of sediment types on the seafloor.png|thumb|upright=1.9| {{center|'''Distribution of sediment types on the seafloor'''<br /> Within each colored area, the type of material shown is what dominates, although other materials are also likely to be present.<br /><small>For further information, [https://en.wikibooks.org/wiki/Historical_Geology/Marine_sediments see here]</small>}}]] |
|||
{{clear}} |
|||
==Microorganisms== |
|||
[[Diatom]]s form a (disputed) phylum containing about 100,000 recognised species of mainly unicellular algae. Diatoms generate about 20 percent of the oxygen produced on the planet each year,<ref name="livescience.com">[https://www.livescience.com/46250-teasing-apart-the-diatom-genome.html The Air You're Breathing? A Diatom Made That]</ref> take in over 6.7 billion metric tons of [[silicon]] each year from the waters in which they live,<ref>{{cite journal | last1 = Treguer | first1 = P. | last2 = Nelson | first2 = D. M. | last3 = Van Bennekom | first3 = A. J. | last4 = Demaster | first4 = D. J. | last5 = Leynaert | first5 = A. | last6 = Queguiner | first6 = B. | year = 1995 | title = The Silica Balance in the World Ocean: A Reestimate | journal = Science | volume = 268 | issue = 5209| pages = 375–9 | pmid = 17746543 | doi = 10.1126/science.268.5209.375 | bibcode = 1995Sci...268..375T | s2cid = 5672525 }}</ref> and contribute nearly half of the organic material found in the oceans. |
|||
<gallery mode=packed heights=145px style=float:left;> |
|||
File:Diatoms (248 05) Various diatoms.jpg|[[Diatom]]s are one of the most common types of phytoplankton |
|||
File:Diatom Helipelta metil.jpg|Their protective shells (frustles) are made of silicon |
|||
File:Diatom - Triceratium favus.jpg |
|||
File:Diatom2.jpg|They come in many shapes and sizes |
|||
</gallery> |
|||
{{clear left}} |
|||
[[Radiolarian]]s are unicellular predatory [[#Marine protists|protists]] encased in elaborate globular shells usually made of silica and pierced with holes. Their name comes from the Latin for "radius". They catch prey by extending parts of their body through the holes. As with the silica frustules of diatoms, radiolarian shells can sink to the ocean floor when radiolarians die and become preserved as part of the ocean sediment. These remains, as [[#Marine microfossils|microfossils]], provide valuable information about past oceanic conditions.<ref name=Wassilieff2006b>Wassilieff, Maggy (2006) [http://www.TeAra.govt.nz/en/photograph/5138/radiolarian-fossils "Plankton - Animal plankton"], ''Te Ara - the Encyclopedia of New Zealand''. Accessed: 2 November 2019.</ref> |
|||
<gallery mode=packed heights=150px style=float:left;> |
|||
File:Mikrofoto.de-Radiolarien 6.jpg|Like diatoms, radiolarians come in many shapes |
|||
File:Theocotylissa ficus Ehrenberg - Radiolarian (34638920262).jpg|Also like diatoms, radiolarian shells are usually made of silicate |
|||
File:Acantharian radiolarian Xiphacantha (Haeckel).jpg|However [[acantharian]] radiolarians have shells made from [[strontium sulfate]] crystals |
|||
File:Spherical radiolarian 2.jpg|Cutaway schematic diagram of a spherical radiolarian shell |
|||
</gallery> |
|||
{{clear}} |
|||
Like radiolarians, [[foraminifera]]ns (''forams'' for short) are single-celled predatory protists, also protected with shells that have holes in them. Their name comes from the Latin for "hole bearers". Their shells, often called [[Test (biology)|tests]], are chambered (forams add more chambers as they grow). The shells are usually made of calcite, but are sometimes made of [[Agglutination (biology)|agglutinated]] sediment particles or [[chiton]], and (rarely) of silica. Most forams are benthic, but about 40 species are planktic.<ref name=Hemleben>{{cite book |first1=C. |last1=Hemleben |first2=O.R. |last2=Anderson |first3=M. |last3=Spindler |title=Modern Planktonic Foraminifera |url=https://books.google.com/books?id=NaHOmAEACAAJ |year=1989 |publisher=Springer-Verlag |isbn=978-3-540-96815-3}}</ref> They are widely researched with well established fossil records which allow scientists to infer a lot about past environments and climates.<ref name=Wassilieff2006b /> |
|||
{{multiple image |
|||
| align = left |
|||
| direction = horizontal |
|||
| header = Foraminiferans |
|||
| header_align = center |
|||
| header_background = |
|||
| footer = Foraminiferans are important unicellular zooplankton [[#Marine protists|protists]], with calcium tests |
|||
| footer_align = center |
|||
| footer_background = |
|||
| background color = |
|||
| image1 = Foram-globigerina hg.jpg |
|||
| width1 = 136 |
|||
| alt1 = |
|||
| caption1 = {{center|...can have more than one nucleus}} |
|||
| image2 = G bulloides Brady 1884.jpg |
|||
| width2 = 104 |
|||
| alt2 = |
|||
| caption2 = {{center|...and defensive spines}} |
|||
}} |
|||
{{clear}} |
|||
==Marine microbenthos== |
|||
[[File:Archaea rock.jpg|thumb|Archaea rock - this deep ocean rock harboured worms that consumed methane-eating archaea]] |
|||
{{see also|Seabed#Sediments|bioturbation|bioirrigation}} |
|||
Marine microbenthos are microorganisms that live in the [[benthic zone]] of the ocean – that live near or on the seafloor, or within or on surface seafloor sediments. The word ''benthos'' comes from Greek, meaning "depth of the sea". Microbenthos are found everywhere on or about the seafloor of continental shelves, as well as in deeper waters, with greater diversity in or on seafloor sediments. In shallow waters, [[seagrass meadow]]s, coral reefs and kelp forests provide particularly rich habitats. In [[photic zone]]s benthic diatoms dominate as photosynthetic organisms. In [[intertidal zone]]s changing [[tide]]s strongly control opportunities for microbenthos. |
|||
<gallery mode=packed heights=180px style="float:left;"> |
|||
File:Elphidium-incertum hg.jpg|''[[Elphidium]]'' a widespread abundant genus of benthic forams |
|||
File:FMIB 50025 Textilaria.jpeg|''[[Heterohelix]]'', an extinct genus of benthic forams |
|||
</gallery> |
|||
{{clear}} |
|||
Both foraminifera and diatoms have [[planktonic]] and [[benthic]] forms, that is, they can drift in the [[water column]] or live on sediment at the bottom of the ocean. Either way, their shells end up on the seafloor after they die. These shells are widely used as [[climate proxy|climate proxies]]. The chemical composition of the shells are a consequence of the chemical composition of the ocean at the time the shells were formed. Past water temperatures can be also be inferred from the ratios of stable [[oxygen isotope]]s in the shells, since lighter isotopes evaporate more readily in warmer water leaving the heavier isotopes in the shells. Information about past climates can be inferred further from the abundance of forams and diatoms, since they tend to be more abundant in warm water.<ref>Bruckner, Monica (2020) [https://serc.carleton.edu/microbelife/topics/proxies/index.html"Paleoclimatology: How Can We Infer Past Climates?"] ''[[Science Education Resource Center|SERC]]'', Carleton College. Modified 23 July 2020. Retrieved 10 September 2020.</ref> |
|||
The sudden [[Cretaceous–Paleogene extinction event|extinction event]] which killed the dinosaurs 66 million years ago also rendered extinct three-quarters of all other animal and plant species. However, deep-sea benthic forams flourished in the aftermath. In 2020 it was reported that researchers have examined the chemical composition of thousands of samples of these benthic forams and used their findings to build the most detailed climate record of Earth ever.<ref>[https://www.livescience.com/oldest-climate-record-ever-cenozoic-era.html?utm_source=notification Earth barreling toward 'Hothouse' state not seen in 50 million years, epic new climate record shows] ''LiveScience'', 10 September 2020.</ref><ref name=Westerhold2020>Westerhold, T., Marwan, N., Drury, A.J., Liebrand, D., Agnini, C., Anagnostou, E., Barnet, J.S., Bohaty, S.M., Vleeschouwer, D., Florindo, F. and Frederichs, T. (2020) [https://science.sciencemag.org/content/369/6509/1383 "An astronomically dated record of Earth’s climate and its predictability over the last 66 Million Years"]. ''Science'', '''369'''(6509): 1383–1387. {{doi|10.1126/science.aba6853}}.</ref> |
|||
Some [[endolith]]s have extremely long lives. In 2013 researchers reported evidence of endoliths in the ocean floor, perhaps millions of years old, with a generation time of 10,000 years.<ref>Bob Yirka [http://phys.org/news/2013-08-soil-beneath-ocean-harbor-bacteria.html 29 Aug 2013]</ref> These are slowly metabolizing and not in a dormant state. Some [[Actinobacteria]] found in [[Siberia]] are estimated to be half a million years old.<ref>[http://www.guardian.co.uk/theobserver/2010/may/02/rachel-sussman-oldest-plants Sussman: Oldest Plants], [[The Guardian]], 2 May 2010</ref><ref>{{Cite web |url=https://www.itsokaytobesmart.com/post/91481365622/siberian-actinobacteria-oldest-living-thing |title=Archived copy |access-date=2018-07-13 |archive-url=https://web.archive.org/web/20180713074804/https://www.itsokaytobesmart.com/post/91481365622/siberian-actinobacteria-oldest-living-thing |archive-date=2018-07-13 |url-status=dead }}</ref><ref>{{Cite journal|title=Ancient bacteria show evidence of DNA repair|first1=Eske |last1=Willerslev|first2=Duane |last2=Froese|first3=David |last3=Gilichinsky|first4=Regin |last4=Rønn|first5=Michael|last5=Bunce|first6=Maria T.|last6=Zuber |first7=M. Thomas P.|last7=Gilbert |first8=Tina |last8=Brand |first9=Kasper |last9=Munch |first10=Rasmus|last10=Nielsen |first11=Mikhail|last11=Mastepanov|first12=Torben R. |last12=Christensen |first13=Martin B.|last13=Hebsgaard |first14=Sarah Stewart|last14=Johnson |date=4 September 2007|journal=Proceedings of the National Academy of Sciences|volume=104|issue=36|pages=14401–14405|doi=10.1073/pnas.0706787104 |pmid=17728401|pmc=1958816 |bibcode=2007PNAS..10414401J}}</ref>{{clear}} |
|||
[[File:Organic matter supply to ocean sediments.png|thumb|upright=2|left| {{center|'''Organic matter supply to sediments ocean'''{{hsp}}<ref name=Middelburg2019>{{cite book |doi = 10.1007/978-3-030-10822-9_4|chapter = Carbon Processing at the Seafloor|title = Marine Carbon Biogeochemistry|series = SpringerBriefs in Earth System Sciences|year = 2019|last1 = Middelburg|first1 = Jack J.|pages = 57–75|isbn = 978-3-030-10821-2}} [[File:CC-BY icon.svg|50px]] Material was copied from this source, which is available under a [https://creativecommons.org/licenses/by/4.0/ Creative Commons Attribution 4.0 International License].</ref>}} (1) Organic matter settling from the water column is deposited at seafloor (donor control; fixed flux upper boundary condition).<br />(2) Sediments in the photic zone are inhabited by benthic microalgae that produce new organic matter in situ and grazing animals can impact the growth of these primary producers.<br />(3) Bioturbating animals transfer labile carbon from the sediment surface layer to deeper layers in the sediments. (Vertical axis is depth; horizontal axis is concentration)<br />(4) Suspension-feeding organisms enhance the transfer of suspended particulate matter from the water column to the sediments (biodeposition).<br />(5) Sponge consume dissolved organic carbon and produce cellular debris that can be consumed by benthic organisms (i.e., the sponge loop).<ref name=Middelburg2018 />]] |
|||
[[File:Different approaches to carbon processing in marine sediments.png|thumb|upright=2|right| {{center|'''Different approaches to carbon processing in marine sediments'''{{hsp}}<ref name=Middelburg2018>{{cite journal |doi = 10.5194/bg-15-413-2018|title = Reviews and syntheses: To the bottom of carbon processing at the seafloor|year = 2018|last1 = Middelburg|first1 = Jack J.|journal = Biogeosciences|volume = 15|issue = 2|pages = 413–427|bibcode = 2018BGeo...15..413M}} [[File:CC-BY icon.svg|50px]] Material was copied from this source, which is available under a [https://creativecommons.org/licenses/by/4.0/ Creative Commons Attribution 4.0 International License].</ref>}}{{space|12}}[[Paleoceanography|Paleoceanographers]] focus on the sedimentary record<br />{{space|12}}[[Biogeochemist]]s quantify carbon burial and recycling<br />{{space|12}}[[Organic geochemist]]s study alteration of organic matter<br />{{space|12}}[[Ecologist]]s focus on carbon as food for organisms living in the sediment<br />{{center|<small>The red–orange–yellow fractions of organic matter have a different lability</small>}}]] |
|||
{{clear}} |
|||
==Ecology== |
|||
<gallery mode=packed heights=150px style=float:left; caption="Marine microanimals"> |
|||
File:Gastrotrich.jpg|[[Dark field microscopy|Darkfield photo]] of a [[gastrotrich]], 0.06-3.0 mm long, a worm-like animal living between sediment particles |
|||
File:Pliciloricus enigmatus.jpg|Armoured ''[[Pliciloricus enigmaticus]]'', about 0.2 mm long, live in spaces between marine gravel |
|||
</gallery> |
|||
{{clear}} |
|||
==See also== |
|||
* [[Bay mud]] |
|||
* [[Hemipelagic sediment]] |
|||
* [[Marine clay]] |
|||
* [[Microbially induced sedimentary structure]] |
|||
* [[Oolitic aragonite sand]] |
|||
* [[Pelagic sediments]] |
|||
==References== |
|||
{{reflist}} |
|||
[[Category:Sediments]] |
Revision as of 01:36, 5 April 2021
Marine sediments are deposits of insoluble particles that accumulate on the seafloor. These have their origins in soil and rocks which have been transported from the land to the sea, mainly by rivers but also as windbourne as dust and by the flow of glaciers into the ocean. Additional deposits come from marine organisms and chemical precipitation in seawater, as well as underwater volcanoes and debris from meteorites.
History
The first major study of deep-ocean sediments occurred between 1872 and 1876 with the HMS Challenger expedition.
Classification
By size
The Wentworth Scale classifies sediment by grain size. Clay sediments are the smallest with a grain diameter of less than .004 mm and boulders are the largest with grain diameters of 256 mm or larger.[1]
By origin
Sediments are also classified by origin. There are four types: lithogenous, hydrogenous, biogenous and cosmogenous.
Lithogenous sediments come from land via rivers, ice, wind and other processes. Biogenous sediments come from organisms like plankton when their exoskeletons break down.Hydrogenous sediments come from chemical reactions in the water. Cosmogenous sediments come from space, filtering in through the atmosphere or carried to Earth on meteorites.[1]
Marine microfossils
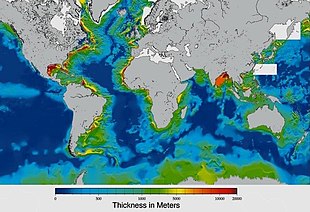
Sediments at the bottom of the ocean have two main origins, terrigenous and biogenous.
Terrigenous sediments account for about 45% of the total marine sediment, and originate in the erosion of rocks on land, transported by rivers and land runoff, windborne dust, volcanoes, or grinding by glaciers.
Biogenous sediments account for the other 55% of the total sediment, and originate in the skeletal remains of marine protists (single-celled plankton and benthos microorganisms). Much smaller amounts of precipitated minerals and meteoric dust can also be present. Ooze, in the context of a marine sediment, does not refer to the consistency of the sediment but to its biological origin. The term ooze was originally used by John Murray, the "father of modern oceanography", who proposed the term radiolarian ooze for the silica deposits of radiolarian shells brought to the surface during the Challenger Expedition.[2] A biogenic ooze is a pelagic sediment containing at least 30 percent from the skeletal remains of marine organisms.
Main types of biogenic ooze
| ||||||||
---|---|---|---|---|---|---|---|---|
type | mineral forms |
protist involved |
name of skeleton | typical size (mm) |
||||
Siliceous ooze | SiO2 silica quartz glass opal chert |
diatom | ![]() |
frustule | 0.002 to 0.2[3] | ![]() |
diatom microfossil from 40 million years ago | |
radiolarian | ![]() |
test or shell | 0.1 to 0.2 | ![]() |
elaborate silica shell of a radiolarian | |||
Calcareous ooze | CaCO3 calcite aragonite limestone marble chalk |
foraminiferan | ![]() |
test or shell | under 1 | ![]() |
Calcified test of a planktic foraminiferan. There are about 10,000 living species of foraminiferans[4] | |
coccolithophore | ![]() |
coccoliths | under 0.1[5] | ![]() |
Coccolithophores are the largest global source of biogenic calcium carbonate, and significantly contribute to the global carbon cycle.[6] They are the main constituent of chalk deposits such as the white cliffs of Dover. |
-
Diatomaceous earth is a soft, siliceous, sedimentary rock made up of microfossils in the form of the frustules (shells) of single cell diatoms (click to magnify)
-
Illustration of a Globigerina ooze
-
Shells (tests), usually made of calcium carbonate, from a foraminiferal ooze on the deep ocean floor

-
Opal can contain protist microfossils of diatoms, radiolarians, silicoflagellates and ebridians [8]
-
Marble can contain protist microfossils of foraminiferans, coccolithophores, calcareous nannoplankton and algae, ostracodes, pteropods, calpionellids and bryozoa [8]


Within each colored area, the type of material shown is what dominates, although other materials are also likely to be present.
For further information, see here
Microorganisms
Diatoms form a (disputed) phylum containing about 100,000 recognised species of mainly unicellular algae. Diatoms generate about 20 percent of the oxygen produced on the planet each year,[9] take in over 6.7 billion metric tons of silicon each year from the waters in which they live,[10] and contribute nearly half of the organic material found in the oceans.
-
Diatoms are one of the most common types of phytoplankton
-
Their protective shells (frustles) are made of silicon
-
They come in many shapes and sizes
Radiolarians are unicellular predatory protists encased in elaborate globular shells usually made of silica and pierced with holes. Their name comes from the Latin for "radius". They catch prey by extending parts of their body through the holes. As with the silica frustules of diatoms, radiolarian shells can sink to the ocean floor when radiolarians die and become preserved as part of the ocean sediment. These remains, as microfossils, provide valuable information about past oceanic conditions.[11]
-
Like diatoms, radiolarians come in many shapes
-
Also like diatoms, radiolarian shells are usually made of silicate
-
However acantharian radiolarians have shells made from strontium sulfate crystals
-
Cutaway schematic diagram of a spherical radiolarian shell
Like radiolarians, foraminiferans (forams for short) are single-celled predatory protists, also protected with shells that have holes in them. Their name comes from the Latin for "hole bearers". Their shells, often called tests, are chambered (forams add more chambers as they grow). The shells are usually made of calcite, but are sometimes made of agglutinated sediment particles or chiton, and (rarely) of silica. Most forams are benthic, but about 40 species are planktic.[12] They are widely researched with well established fossil records which allow scientists to infer a lot about past environments and climates.[11]
Marine microbenthos
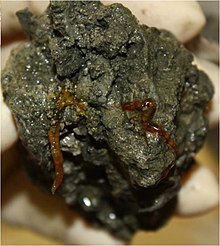
Marine microbenthos are microorganisms that live in the benthic zone of the ocean – that live near or on the seafloor, or within or on surface seafloor sediments. The word benthos comes from Greek, meaning "depth of the sea". Microbenthos are found everywhere on or about the seafloor of continental shelves, as well as in deeper waters, with greater diversity in or on seafloor sediments. In shallow waters, seagrass meadows, coral reefs and kelp forests provide particularly rich habitats. In photic zones benthic diatoms dominate as photosynthetic organisms. In intertidal zones changing tides strongly control opportunities for microbenthos.
-
Elphidium a widespread abundant genus of benthic forams
-
Heterohelix, an extinct genus of benthic forams
Both foraminifera and diatoms have planktonic and benthic forms, that is, they can drift in the water column or live on sediment at the bottom of the ocean. Either way, their shells end up on the seafloor after they die. These shells are widely used as climate proxies. The chemical composition of the shells are a consequence of the chemical composition of the ocean at the time the shells were formed. Past water temperatures can be also be inferred from the ratios of stable oxygen isotopes in the shells, since lighter isotopes evaporate more readily in warmer water leaving the heavier isotopes in the shells. Information about past climates can be inferred further from the abundance of forams and diatoms, since they tend to be more abundant in warm water.[13]
The sudden extinction event which killed the dinosaurs 66 million years ago also rendered extinct three-quarters of all other animal and plant species. However, deep-sea benthic forams flourished in the aftermath. In 2020 it was reported that researchers have examined the chemical composition of thousands of samples of these benthic forams and used their findings to build the most detailed climate record of Earth ever.[14][15]
Some endoliths have extremely long lives. In 2013 researchers reported evidence of endoliths in the ocean floor, perhaps millions of years old, with a generation time of 10,000 years.[16] These are slowly metabolizing and not in a dormant state. Some Actinobacteria found in Siberia are estimated to be half a million years old.[17][18][19]
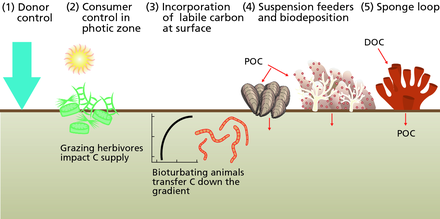
(2) Sediments in the photic zone are inhabited by benthic microalgae that produce new organic matter in situ and grazing animals can impact the growth of these primary producers.
(3) Bioturbating animals transfer labile carbon from the sediment surface layer to deeper layers in the sediments. (Vertical axis is depth; horizontal axis is concentration)
(4) Suspension-feeding organisms enhance the transfer of suspended particulate matter from the water column to the sediments (biodeposition).
(5) Sponge consume dissolved organic carbon and produce cellular debris that can be consumed by benthic organisms (i.e., the sponge loop).[21]

Biogeochemists quantify carbon burial and recycling
Organic geochemists study alteration of organic matter
Ecologists focus on carbon as food for organisms living in the sediment
Ecology
- Marine microanimals
-
Darkfield photo of a gastrotrich, 0.06-3.0 mm long, a worm-like animal living between sediment particles
-
Armoured Pliciloricus enigmaticus, about 0.2 mm long, live in spaces between marine gravel
See also
- Bay mud
- Hemipelagic sediment
- Marine clay
- Microbially induced sedimentary structure
- Oolitic aragonite sand
- Pelagic sediments
References
- ^ a b Sediments NOAA. Accessed 5 April 2021.
This article incorporates text from this source, which is in the public domain.
- ^ Thomson, Charles Wyville (2014) Voyage of the Challenger : The Atlantic Cambridge University Press, page235. ISBN 9781108074759.
- ^ Grethe R. Hasle; Erik E. Syvertsen; Karen A. Steidinger; Karl Tangen (1996-01-25). "Marine Diatoms". In Carmelo R. Tomas (ed.). Identifying Marine Diatoms and Dinoflagellates. Academic Press. pp. 5–385. ISBN 978-0-08-053441-1. Retrieved 2013-11-13.
- ^ Ald, S.M.; et al. (2007). "Diversity, Nomenclature, and Taxonomy of Protists" (PDF). Syst. Biol. 56 (4): 684–689. doi:10.1080/10635150701494127. PMID 17661235. Archived from the original (PDF) on 31 March 2011. Retrieved 11 October 2019.
- ^ Moheimani, N.R.; Webb, J.P.; Borowitzka, M.A. (2012), "Bioremediation and other potential applications of coccolithophorid algae: A review. . Bioremediation and other potential applications of coccolithophorid algae: A review", Algal Research, 1 (2): 120–133, doi:10.1016/j.algal.2012.06.002
- ^ Taylor, A.R.; Chrachri, A.; Wheeler, G.; Goddard, H.; Brownlee, C. (2011). "A voltage-gated H+ channel underlying pH homeostasis in calcifying coccolithophores". PLOS Biology. 9 (6): e1001085. doi:10.1371/journal.pbio.1001085. PMC 3119654. PMID 21713028.
{{cite journal}}
: CS1 maint: unflagged free DOI (link) - ^ Wierer, U.; Arrighi, S.; Bertola, S.; Kaufmann, G.; Baumgarten, B.; Pedrotti, A.; Pernter, P.; Pelegrin, J. (2018). "The Iceman's lithic toolkit: Raw material, technology, typology and use". PLOS ONE. 13 (6): e0198292. Bibcode:2018PLoSO..1398292W. doi:10.1371/journal.pone.0198292. PMC 6010222. PMID 29924811.
{{cite journal}}
: CS1 maint: unflagged free DOI (link) - ^ a b Haq B.U. and Boersma A. (Eds.) (1998) Introduction to Marine Micropaleontology Elsevier. ISBN 9780080534961
- ^ The Air You're Breathing? A Diatom Made That
- ^ Treguer, P.; Nelson, D. M.; Van Bennekom, A. J.; Demaster, D. J.; Leynaert, A.; Queguiner, B. (1995). "The Silica Balance in the World Ocean: A Reestimate". Science. 268 (5209): 375–9. Bibcode:1995Sci...268..375T. doi:10.1126/science.268.5209.375. PMID 17746543. S2CID 5672525.
- ^ a b Wassilieff, Maggy (2006) "Plankton - Animal plankton", Te Ara - the Encyclopedia of New Zealand. Accessed: 2 November 2019.
- ^ Hemleben, C.; Anderson, O.R.; Spindler, M. (1989). Modern Planktonic Foraminifera. Springer-Verlag. ISBN 978-3-540-96815-3.
- ^ Bruckner, Monica (2020) "Paleoclimatology: How Can We Infer Past Climates?" SERC, Carleton College. Modified 23 July 2020. Retrieved 10 September 2020.
- ^ Earth barreling toward 'Hothouse' state not seen in 50 million years, epic new climate record shows LiveScience, 10 September 2020.
- ^ Westerhold, T., Marwan, N., Drury, A.J., Liebrand, D., Agnini, C., Anagnostou, E., Barnet, J.S., Bohaty, S.M., Vleeschouwer, D., Florindo, F. and Frederichs, T. (2020) "An astronomically dated record of Earth’s climate and its predictability over the last 66 Million Years". Science, 369(6509): 1383–1387. doi:10.1126/science.aba6853.
- ^ Bob Yirka 29 Aug 2013
- ^ Sussman: Oldest Plants, The Guardian, 2 May 2010
- ^ "Archived copy". Archived from the original on 2018-07-13. Retrieved 2018-07-13.
{{cite web}}
: CS1 maint: archived copy as title (link) - ^ Willerslev, Eske; Froese, Duane; Gilichinsky, David; Rønn, Regin; Bunce, Michael; Zuber, Maria T.; Gilbert, M. Thomas P.; Brand, Tina; Munch, Kasper; Nielsen, Rasmus; Mastepanov, Mikhail; Christensen, Torben R.; Hebsgaard, Martin B.; Johnson, Sarah Stewart (4 September 2007). "Ancient bacteria show evidence of DNA repair". Proceedings of the National Academy of Sciences. 104 (36): 14401–14405. Bibcode:2007PNAS..10414401J. doi:10.1073/pnas.0706787104. PMC 1958816. PMID 17728401.
- ^ Middelburg, Jack J. (2019). "Carbon Processing at the Seafloor". Marine Carbon Biogeochemistry. SpringerBriefs in Earth System Sciences. pp. 57–75. doi:10.1007/978-3-030-10822-9_4. ISBN 978-3-030-10821-2.
Material was copied from this source, which is available under a Creative Commons Attribution 4.0 International License.
- ^ a b Middelburg, Jack J. (2018). "Reviews and syntheses: To the bottom of carbon processing at the seafloor". Biogeosciences. 15 (2): 413–427. Bibcode:2018BGeo...15..413M. doi:10.5194/bg-15-413-2018.
{{cite journal}}
: CS1 maint: unflagged free DOI (link)Material was copied from this source, which is available under a Creative Commons Attribution 4.0 International License.