Blood–brain barrier: Difference between revisions
Citation bot (talk | contribs) Alter: page. Add: doi-access, page, pmc, pmid, s2cid, authors 1-1. Removed parameters. Formatted dashes. Some additions/deletions were parameter name changes. | Use this bot. Report bugs. | Suggested by Abductive | #UCB_webform 3639/3849 |
Replace predatory with review cite |
||
Line 19: | Line 19: | ||
The '''blood–brain barrier''' ('''BBB''') is a highly selective [[semipermeable membrane|semipermeable]] border of [[endothelium|endothelial cells]] that prevents [[solutes]] in the circulating [[blood]] from ''non-selectively'' crossing into the [[extracellular fluid]] of the [[central nervous system]] where [[neuron]]s reside.<ref name="daneman">{{cite journal | vauthors = Daneman R, Prat A | title = The blood-brain barrier | journal = Cold Spring Harbor Perspectives in Biology | volume = 7 | issue = 1 | pages = a020412 | date = January 2015 | pmid = 25561720 | pmc = 4292164 | doi = 10.1101/cshperspect.a020412 }}</ref> The blood–[[brain]] barrier is formed by endothelial cells of the [[Capillary|capillary wall]], [[astrocyte]] end-feet ensheathing the capillary, and [[pericytes]] embedded in the capillary [[basement membrane]].<ref>{{cite journal | vauthors = Ballabh P, Braun A, Nedergaard M | s2cid = 2202060 | title = The blood-brain barrier: an overview: structure, regulation, and clinical implications | journal = [[Neurobiology of Disease]] | volume = 16 | issue = 1 | pages = 1–13 | date = June 2004 | pmid = 15207256 | doi = 10.1016/j.nbd.2003.12.016 }}</ref> This system allows the passage of some small molecules by [[passive transport|passive diffusion]], as well as the selective and active transport of various nutrients, ions, organic anions, and macromolecules such as glucose and [[amino acid]]s that are crucial to neural function.<ref name="Gupta">{{cite book | vauthors = Gupta S, Dhanda S, Sandhir R | title=Brain Targeted Drug Delivery System | chapter=Anatomy and physiology of blood-brain barrier | publisher=Elsevier | year=2019 | isbn=978-0-12-814001-7 | doi=10.1016/b978-0-12-814001-7.00002-0 | pages=7–31| s2cid=91847478 }}</ref> |
The '''blood–brain barrier''' ('''BBB''') is a highly selective [[semipermeable membrane|semipermeable]] border of [[endothelium|endothelial cells]] that prevents [[solutes]] in the circulating [[blood]] from ''non-selectively'' crossing into the [[extracellular fluid]] of the [[central nervous system]] where [[neuron]]s reside.<ref name="daneman">{{cite journal | vauthors = Daneman R, Prat A | title = The blood-brain barrier | journal = Cold Spring Harbor Perspectives in Biology | volume = 7 | issue = 1 | pages = a020412 | date = January 2015 | pmid = 25561720 | pmc = 4292164 | doi = 10.1101/cshperspect.a020412 }}</ref> The blood–[[brain]] barrier is formed by endothelial cells of the [[Capillary|capillary wall]], [[astrocyte]] end-feet ensheathing the capillary, and [[pericytes]] embedded in the capillary [[basement membrane]].<ref>{{cite journal | vauthors = Ballabh P, Braun A, Nedergaard M | s2cid = 2202060 | title = The blood-brain barrier: an overview: structure, regulation, and clinical implications | journal = [[Neurobiology of Disease]] | volume = 16 | issue = 1 | pages = 1–13 | date = June 2004 | pmid = 15207256 | doi = 10.1016/j.nbd.2003.12.016 }}</ref> This system allows the passage of some small molecules by [[passive transport|passive diffusion]], as well as the selective and active transport of various nutrients, ions, organic anions, and macromolecules such as glucose and [[amino acid]]s that are crucial to neural function.<ref name="Gupta">{{cite book | vauthors = Gupta S, Dhanda S, Sandhir R | title=Brain Targeted Drug Delivery System | chapter=Anatomy and physiology of blood-brain barrier | publisher=Elsevier | year=2019 | isbn=978-0-12-814001-7 | doi=10.1016/b978-0-12-814001-7.00002-0 | pages=7–31| s2cid=91847478 }}</ref> |
||
The blood–brain barrier restricts the passage of [[pathogen]]s, the diffusion of [[solute]]s in the blood, and [[Molecular mass|large]] or [[Hydrophile|hydrophilic]] molecules into the [[cerebrospinal fluid]], while allowing the diffusion of [[Hydrophobe|hydrophobic]] molecules (O<sub>2</sub>, CO<sub>2</sub>, hormones) and small non-polar molecules.<ref>{{cite journal | vauthors = Obermeier B, Daneman R, Ransohoff RM | title = Development, maintenance and disruption of the blood-brain barrier | journal = Nature Medicine | volume = 19 | issue = 12 | pages = 1584–96 | date = December 2013 | pmid = 24309662 | pmc = 4080800 | doi = 10.1038/nm.3407 }}</ref><ref>{{ |
The blood–brain barrier restricts the passage of [[pathogen]]s, the diffusion of [[solute]]s in the blood, and [[Molecular mass|large]] or [[Hydrophile|hydrophilic]] molecules into the [[cerebrospinal fluid]], while allowing the diffusion of [[Hydrophobe|hydrophobic]] molecules (O<sub>2</sub>, CO<sub>2</sub>, hormones) and small non-polar molecules.<ref>{{cite journal | vauthors = Obermeier B, Daneman R, Ransohoff RM | title = Development, maintenance and disruption of the blood-brain barrier | journal = Nature Medicine | volume = 19 | issue = 12 | pages = 1584–96 | date = December 2013 | pmid = 24309662 | pmc = 4080800 | doi = 10.1038/nm.3407 }}</ref><ref name="pmid33208141">{{cite journal | vauthors = Kadry H, Noorani B, Cucullo L | title = A blood-brain barrier overview on structure, function, impairment, and biomarkers of integrity | journal = Fluids Barriers CNS | volume = 17 | issue = 1 | pages = 69 | date = November 2020 | pmid = 33208141 | pmc = 7672931 | doi = 10.1186/s12987-020-00230-3 }}</ref> Cells of the barrier actively transport [[metabolism|metabolic]] products such as glucose across the barrier using specific [[Membrane transport protein|transport proteins]].<ref name="Stamatovic 2008 179–192">{{cite journal | vauthors = Stamatovic SM, Keep RF, Andjelkovic AV | title = Brain endothelial cell-cell junctions: how to "open" the blood brain barrier | journal = Current Neuropharmacology | volume = 6 | issue = 3 | pages = 179–92 | date = September 2008 | pmid = 19506719 | pmc = 2687937 | doi = 10.2174/157015908785777210 }}</ref> The barrier also restricts the passage of peripheral immune factors, like signaling molecules, antibodies, and immune cells, into the CNS, thus insulating the brain from damage due to peripheral immune events.<ref>{{cite journal | vauthors = Muldoon LL, Alvarez JI, Begley DJ, Boado RJ, Del Zoppo GJ, Doolittle ND, Engelhardt B, Hallenbeck JM, Lonser RR, Ohlfest JR, Prat A, Scarpa M, Smeyne RJ, Drewes LR, Neuwelt EA | display-authors = 6 | title = Immunologic privilege in the central nervous system and the blood-brain barrier | journal = Journal of Cerebral Blood Flow and Metabolism | volume = 33 | issue = 1 | pages = 13–21 | date = January 2013 | pmid = 23072749 | pmc = 3597357 | doi = 10.1038/jcbfm.2012.153 }}</ref> |
||
Specialized brain structures participating in sensory and secretory integration within brain [[neural circuit]]s—the [[circumventricular organ]]s and [[choroid plexus]]—have in contrast highly permeable capillaries.<ref name="Kaur">{{cite journal | vauthors = Kaur C, Ling EA | title = The circumventricular organs | journal = Histology and Histopathology | volume = 32 | issue = 9 | pages = 879–892 | date = September 2017 | pmid = 28177105 | doi = 10.14670/HH-11-881 }}</ref> |
Specialized brain structures participating in sensory and secretory integration within brain [[neural circuit]]s—the [[circumventricular organ]]s and [[choroid plexus]]—have in contrast highly permeable capillaries.<ref name="Kaur">{{cite journal | vauthors = Kaur C, Ling EA | title = The circumventricular organs | journal = Histology and Histopathology | volume = 32 | issue = 9 | pages = 879–892 | date = September 2017 | pmid = 28177105 | doi = 10.14670/HH-11-881 }}</ref> |
Revision as of 14:40, 2 February 2023
Blood–brain barrier | |
---|---|
![]() Solute permeability at the BBB vs. choroid plexus | |
Details | |
System | Neuroimmune system |
Identifiers | |
Acronym(s) | BBB |
MeSH | D001812 |
Anatomical terminology |
The blood–brain barrier (BBB) is a highly selective semipermeable border of endothelial cells that prevents solutes in the circulating blood from non-selectively crossing into the extracellular fluid of the central nervous system where neurons reside.[1] The blood–brain barrier is formed by endothelial cells of the capillary wall, astrocyte end-feet ensheathing the capillary, and pericytes embedded in the capillary basement membrane.[2] This system allows the passage of some small molecules by passive diffusion, as well as the selective and active transport of various nutrients, ions, organic anions, and macromolecules such as glucose and amino acids that are crucial to neural function.[3]
The blood–brain barrier restricts the passage of pathogens, the diffusion of solutes in the blood, and large or hydrophilic molecules into the cerebrospinal fluid, while allowing the diffusion of hydrophobic molecules (O2, CO2, hormones) and small non-polar molecules.[4][5] Cells of the barrier actively transport metabolic products such as glucose across the barrier using specific transport proteins.[6] The barrier also restricts the passage of peripheral immune factors, like signaling molecules, antibodies, and immune cells, into the CNS, thus insulating the brain from damage due to peripheral immune events.[7]
Specialized brain structures participating in sensory and secretory integration within brain neural circuits—the circumventricular organs and choroid plexus—have in contrast highly permeable capillaries.[8]
Structure


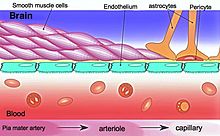
The BBB results from the selectivity of the tight junctions between the endothelial cells of brain capillaries, restricting the passage of solutes.[1] At the interface between blood and the brain, endothelial cells are adjoined continuously by these tight junctions, which are composed of smaller subunits of transmembrane proteins, such as occludin, claudins (such as Claudin-5), junctional adhesion molecule (such as JAM-A).[6] Each of these tight junction proteins is stabilized to the endothelial cell membrane by another protein complex that includes scaffolding proteins such as tight junction protein 1 (ZO1) and associated proteins.[6]
The BBB is composed of endothelial cells restricting passage of substances from the blood more selectively than endothelial cells of capillaries elsewhere in the body.[9] Astrocyte cell projections called astrocytic feet (also known as "glia limitans") surround the endothelial cells of the BBB, providing biochemical support to those cells.[10] The BBB is distinct from the quite similar blood-cerebrospinal fluid barrier, which is a function of the choroidal cells of the choroid plexus, and from the blood-retinal barrier, which can be considered a part of the whole realm of such barriers.[11]
Not all vessels in the human brain exhibit BBB properties. Some examples of this include the circumventricular organs, the roof of the third and fourth ventricles, capillaries in the pineal gland on the roof of the diencephalon and the pineal gland. The pineal gland secretes the hormone melatonin "directly into the systemic circulation",[12] thus melatonin is not affected by the blood–brain barrier.[13]
Development
The BBB appears to be functional by the time of birth. P-glycoprotein, a transporter, exists already in the embryonal endothelium.[14]
Measurement of brain uptake of various blood-borne solutes showed that newborn endothelial cells were functionally similar to those in adults,[15] indicating that a selective BBB is operative at birth.
In mice, Claudin-5 loss during development is lethal and results in size-selective loosening of the BBB.[16]
Function
The blood–brain barrier acts effectively to protect brain tissue from circulating pathogens and other potentially toxic substances.[17] Accordingly, blood-borne infections of the brain are rare.[1] Infections of the brain that do occur are often difficult to treat. Antibodies are too large to cross the blood–brain barrier, and only certain antibiotics are able to pass.[18] In some cases, a drug has to be administered directly into the cerebrospinal fluid where it can enter the brain by crossing the blood-cerebrospinal fluid barrier.[19][20]
Circumventricular organs
Circumventricular organs (CVOs) are individual structures located adjacent to the fourth ventricle or third ventricle in the brain, and are characterized by dense capillary beds with permeable endothelial cells unlike those of the blood–brain barrier.[21][22] Included among CVOs having highly permeable capillaries are the area postrema, subfornical organ, vascular organ of the lamina terminalis, median eminence, pineal gland, and three lobes of the pituitary gland.[21][23]
Permeable capillaries of the sensory CVOs (area postrema, subfornical organ, vascular organ of the lamina terminalis) enable rapid detection of circulating signals in systemic blood, while those of the secretory CVOs (median eminence, pineal gland, pituitary lobes) facilitate transport of brain-derived signals into the circulating blood.[21][22] Consequently, the CVO permeable capillaries are the point of bidirectional blood–brain communication for neuroendocrine function.[21][23][24]
Specialized permeable zones
The border zones between brain tissue "behind" the blood–brain barrier and zones "open" to blood signals in certain CVOs contain specialized hybrid capillaries that are leakier than typical brain capillaries, but not as permeable as CVO capillaries. Such zones exist at the border of the area postrema—nucleus tractus solitarii (NTS),[25] and median eminence—hypothalamic arcuate nucleus.[24][26] These zones appear to function as rapid transit regions for brain structures involved in diverse neural circuits—like the NTS and arcuate nucleus—to receive blood signals which are then transmitted into neural output.[24][25] The permeable capillary zone shared between the median eminence and hypothalamic arcuate nucleus is augmented by wide pericapillary spaces, facilitating bidirectional flow of solutes between the two structures, and indicating that the median eminence is not only a secretory organ, but may also be a sensory organ.[24][26]
Therapeutic research
As a drug target
The blood–brain barrier is formed by the brain capillary endothelium and excludes from the brain 100% of large-molecule neurotherapeutics and more than 98% of all small-molecule drugs.[1] Overcoming the difficulty of delivering therapeutic agents to specific regions of the brain presents a major challenge to treatment of most brain disorders.[27][28] In its neuroprotective role, the blood–brain barrier functions to hinder the delivery of many potentially important diagnostic and therapeutic agents to the brain. Therapeutic molecules and antibodies that might otherwise be effective in diagnosis and therapy do not cross the BBB in adequate amounts to be clinically effective.[27] The BBB represents an obstacle to some drugs reaching the brain, thus to overcome this barrier some peptides able to naturally cross the BBB have been widely investigated as a drug delivery system.[29]
Mechanisms for drug targeting in the brain involve going either "through" or "behind" the BBB. Modalities for drug delivery to the brain in unit doses through the BBB entail its disruption by osmotic means, or biochemically by the use of vasoactive substances, such as bradykinin,[30] or even by localized exposure to high-intensity focused ultrasound (HIFU).[31]
Other methods used to get through the BBB may entail the use of endogenous transport systems, including carrier-mediated transporters, such as glucose and amino acid carriers, receptor-mediated transcytosis for insulin or transferrin, and the blocking of active efflux transporters such as p-glycoprotein.[27] Some studies have shown that vectors targeting BBB transporters, such as the transferrin receptor, have been found to remain entrapped in brain endothelial cells of capillaries, instead of being ferried across the BBB into the targeted area.[27][32]
Nanoparticles
Nanotechnology is under preliminary research for its potential to facilitate the transfer of drugs across the BBB.[27][33][34] Capillary endothelial cells and associated pericytes may be abnormal in tumors and the blood–brain barrier may not always be intact in brain tumors.[34] Other factors, such as astrocytes, may contribute to the resistance of brain tumors to therapy using nanoparticles.[35] Fat soluble molecules less than 400 daltons in mass can freely diffuse past the BBB through lipid mediated passive diffusion.[36]
Damage in injury and disease
The blood–brain barrier may become damaged in select neurological diseases, as indicated by neuroimaging studies of Alzheimer's disease, amyotrophic lateral sclerosis, epilepsy, ischemic stroke,[37][38][39][40] and brain trauma,[27][41] and in systemic diseases, such as liver failure.[1] Effects such as impaired glucose transport and endothelial degeneration may lead to metabolic dysfunction within the brain, and an increased permeability of the BBB to proinflammatory factors, potentially allowing antibiotics and phagocytes to move across the BBB.[1][27]
Prediction
There have been many attempts to correlate the experimental blood brain barrier permeability, with physicochemical properties. The first QSAR study of brain–blood distribution was conducted in 1988, this study reported the in vivo values in rats for a large number of H2 receptor histamine agonists.[42] The first papers trying to model blood brain barrier permeability, identified three properties, i.e., molecular volume, lipophilicity, and hydrogen bonding potential, as contributing significantly to the transport through the blood brain barrier.[43] Two datasets, one with numerical logBB values (1058 molecules) and the one with categorical labels (7807 molecules with 4956 BBB+ and 2851 BBB−) have been published in 2021.[44] The categorical dataset has been used in 2022 to select four different classification models[45] based on molecular fingerprints,[46] MACCS166 keys[47] and molecular descriptors.[48]
History
In 1898, Arthur Biedl and R. Kraus observed that low-concentration "bile salts" failed to affect behavior when injected into the bloodstream of animals. Thus, in theory, they had failed to enter the brain.[49] Two years later, Max Lewandowsky coined the term "Blood–brain barrier" in 1900, referring to the hypothesized semipermeable membrane (then termed hematoencephalic barrier).[50]
All the while, bacteriologist Paul Ehrlich was studying staining, a procedure that is used in many microscopy studies to make fine biological structures visible using chemical dyes.[51] As Ehrlich injected some of these dyes (notably the aniline dyes that were then widely used), the dye stained all of the organs of some kinds of animals except for their brains.[51] At that time, Ehrlich attributed this lack of staining to the brain simply not picking up as much of the dye.[52]
However, in a later experiment in 1913, Edwin Goldmann (one of Ehrlich's students) injected the dye directly into the cerebrospinal fluids of animal brains. He found then the brains did become dyed, but the rest of the body did not, demonstrating the existence of a compartmentalization between the two. At that time, it was thought that the blood vessels themselves were responsible for the barrier, since no obvious membrane could be found.
See also
- Blood–ocular barrier – Physical barrier between the local blood vessels and most parts of the eye itself
- Blood–retinal barrier – Part of the blood–ocular barrier that prevents certain substances from entering the retina
- Blood–spinal cord barrier – Semipermeable anatomical interface
References
- ^ a b c d e f Daneman R, Prat A (January 2015). "The blood-brain barrier". Cold Spring Harbor Perspectives in Biology. 7 (1): a020412. doi:10.1101/cshperspect.a020412. PMC 4292164. PMID 25561720.
- ^ Ballabh P, Braun A, Nedergaard M (June 2004). "The blood-brain barrier: an overview: structure, regulation, and clinical implications". Neurobiology of Disease. 16 (1): 1–13. doi:10.1016/j.nbd.2003.12.016. PMID 15207256. S2CID 2202060.
- ^ Gupta S, Dhanda S, Sandhir R (2019). "Anatomy and physiology of blood-brain barrier". Brain Targeted Drug Delivery System. Elsevier. pp. 7–31. doi:10.1016/b978-0-12-814001-7.00002-0. ISBN 978-0-12-814001-7. S2CID 91847478.
- ^ Obermeier B, Daneman R, Ransohoff RM (December 2013). "Development, maintenance and disruption of the blood-brain barrier". Nature Medicine. 19 (12): 1584–96. doi:10.1038/nm.3407. PMC 4080800. PMID 24309662.
- ^ Kadry H, Noorani B, Cucullo L (November 2020). "A blood-brain barrier overview on structure, function, impairment, and biomarkers of integrity". Fluids Barriers CNS. 17 (1): 69. doi:10.1186/s12987-020-00230-3. PMC 7672931. PMID 33208141.
{{cite journal}}
: CS1 maint: unflagged free DOI (link) - ^ a b c Stamatovic SM, Keep RF, Andjelkovic AV (September 2008). "Brain endothelial cell-cell junctions: how to "open" the blood brain barrier". Current Neuropharmacology. 6 (3): 179–92. doi:10.2174/157015908785777210. PMC 2687937. PMID 19506719.
- ^ Muldoon LL, Alvarez JI, Begley DJ, Boado RJ, Del Zoppo GJ, Doolittle ND, et al. (January 2013). "Immunologic privilege in the central nervous system and the blood-brain barrier". Journal of Cerebral Blood Flow and Metabolism. 33 (1): 13–21. doi:10.1038/jcbfm.2012.153. PMC 3597357. PMID 23072749.
- ^ Kaur C, Ling EA (September 2017). "The circumventricular organs". Histology and Histopathology. 32 (9): 879–892. doi:10.14670/HH-11-881. PMID 28177105.
- ^ van Leeuwen LM, Evans RJ, Jim KK, Verboom T, Fang X, Bojarczuk A, et al. (February 2018). "claudin 5". Biology Open. 7 (2): bio030494. doi:10.1242/bio.030494. PMC 5861362. PMID 29437557.
- ^ Abbott NJ, Rönnbäck L, Hansson E (January 2006). "Astrocyte-endothelial interactions at the blood-brain barrier". Nature Reviews. Neuroscience. 7 (1): 41–53. doi:10.1038/nrn1824. PMID 16371949. S2CID 205500476.
- ^ Hamilton RD, Foss AJ, Leach L (December 2007). "Establishment of a human in vitro model of the outer blood-retinal barrier". Journal of Anatomy. 211 (6): 707–16. doi:10.1111/j.1469-7580.2007.00812.x. PMC 2375847. PMID 17922819.
- ^ Pritchard TC, Alloway KD (1999). Medical Neuroscience (Preview). Hayes Barton Press. pp. 76–7. ISBN 978-1-889325-29-3. OCLC 41086829. Retrieved 2009-02-08 – via Google Books.
- ^ Gilgun-Sherki Y, Melamed E, Offen D (June 2001). "Oxidative stress induced-neurodegenerative diseases: the need for antioxidants that penetrate the blood brain barrier". Neuropharmacology. 40 (8): 959–75. doi:10.1016/S0028-3908(01)00019-3. PMID 11406187. S2CID 15395925.
- ^ Tsai CE, Daood MJ, Lane RH, Hansen TW, Gruetzmacher EM, Watchko JF (January 2002). "P-glycoprotein expression in mouse brain increases with maturation". Biology of the Neonate. 81 (1): 58–64. doi:10.1159/000047185. PMID 11803178. S2CID 46815691.
- ^ Braun LD, Cornford EM, Oldendorf WH (January 1980). "Newborn rabbit blood-brain barrier is selectively permeable and differs substantially from the adult". Journal of Neurochemistry. 34 (1): 147–52. doi:10.1111/j.1471-4159.1980.tb04633.x. PMID 7452231. S2CID 21944159.
- ^ Nitta T, Hata M, Gotoh S, Seo Y, Sasaki H, Hashimoto N, et al. (May 2003). "Size-selective loosening of the blood-brain barrier in claudin-5-deficient mice". The Journal of Cell Biology. 161 (3): 653–60. doi:10.1083/jcb.200302070. PMC 2172943. PMID 12743111.
- ^ Abdullahi W, Tripathi D, Ronaldson PT (September 2018). "Blood-brain barrier dysfunction in ischemic stroke: targeting tight junctions and transporters for vascular protection". American Journal of Physiology. Cell Physiology. 315 (3): C343–C356. doi:10.1152/ajpcell.00095.2018. PMC 6171039. PMID 29949404.
- ^ Raza MW, Shad A, Pedler SJ, Karamat KA (March 2005). "Penetration and activity of antibiotics in brain abscess". Journal of the College of Physicians and Surgeons--Pakistan. 15 (3): 165–167. PMID 15808097.
- ^ Pardridge WM (January 2011). "Drug transport in brain via the cerebrospinal fluid". Fluids and Barriers of the CNS. 8 (1): 7. doi:10.1186/2045-8118-8-7. PMC 3042981. PMID 21349155.
{{cite journal}}
: CS1 maint: unflagged free DOI (link) - ^ Chen Y, Imai H, Ito A, Saito N (2013). "Novel modified method for injection into the cerebrospinal fluid via the cerebellomedullary cistern in mice". Acta Neurobiologiae Experimentalis. 73 (2): 304–311. PMID 23823990.
- ^ a b c d Gross PM, Weindl A (December 1987). "Peering through the windows of the brain". Journal of Cerebral Blood Flow and Metabolism. 7 (6): 663–72. doi:10.1038/jcbfm.1987.120. PMID 2891718.
- ^ a b Gross PM (1992). Circumventricular organ capillaries. Progress in Brain Research. Vol. 91. pp. 219–33. doi:10.1016/S0079-6123(08)62338-9. PMID 1410407.
- ^ a b Miyata S (2015). "New aspects in fenestrated capillary and tissue dynamics in the sensory circumventricular organs of adult brains". Frontiers in Neuroscience. 9: 390. doi:10.3389/fnins.2015.00390. PMC 4621430. PMID 26578857.
- ^ a b c d Rodríguez EM, Blázquez JL, Guerra M (April 2010). "The design of barriers in the hypothalamus allows the median eminence and the arcuate nucleus to enjoy private milieus: the former opens to the portal blood and the latter to the cerebrospinal fluid". Peptides. 31 (4): 757–76. doi:10.1016/j.peptides.2010.01.003. PMID 20093161. S2CID 44760261.
- ^ a b Gross PM, Wall KM, Pang JJ, Shaver SW, Wainman DS (December 1990). "Microvascular specializations promoting rapid interstitial solute dispersion in nucleus tractus solitarius". The American Journal of Physiology. 259 (6 Pt 2): R1131-8. doi:10.1152/ajpregu.1990.259.6.R1131. PMID 2260724.
- ^ a b Shaver SW, Pang JJ, Wainman DS, Wall KM, Gross PM (March 1992). "Morphology and function of capillary networks in subregions of the rat tuber cinereum". Cell and Tissue Research. 267 (3): 437–48. doi:10.1007/BF00319366. PMID 1571958. S2CID 27789146.
- ^ a b c d e f g Sweeney MD, Sagare AP, Zlokovic BV (March 2018). "Blood-brain barrier breakdown in Alzheimer disease and other neurodegenerative disorders". Nature Reviews. Neurology. 14 (3): 133–150. doi:10.1038/nrneurol.2017.188. PMC 5829048. PMID 29377008.
- ^ Harilal S, Jose J, Parambi DG, Kumar R, Unnikrishnan MK, Uddin MS, et al. (July 2020). "Revisiting the blood-brain barrier: A hard nut to crack in the transportation of drug molecules". Brain Research Bulletin. 160: 121–140. doi:10.1016/j.brainresbull.2020.03.018. PMID 32315731. S2CID 215807970.
- ^ de Oliveira EC, da Costa KS, Taube PS, Lima AH, Junior CS (2022-03-25). "Biological Membrane-Penetrating Peptides: Computational Prediction and Applications". Frontiers in Cellular and Infection Microbiology. 12: 838259. doi:10.3389/fcimb.2022.838259. PMC 8992797. PMID 35402305.
- ^ Marcos-Contreras OA, Martinez de Lizarrondo S, Bardou I, Orset C, Pruvost M, Anfray A, et al. (November 2016). "Hyperfibrinolysis increases blood-brain barrier permeability by a plasmin- and bradykinin-dependent mechanism". Blood. 128 (20): 2423–2434. doi:10.1182/blood-2016-03-705384. PMID 27531677.
- ^ McDannold N, Vykhodtseva N, Hynynen K (May 2008). "Blood-brain barrier disruption induced by focused ultrasound and circulating preformed microbubbles appears to be characterized by the mechanical index". Ultrasound in Medicine & Biology. 34 (5): 834–40. doi:10.1016/j.ultrasmedbio.2007.10.016. PMC 2442477. PMID 18207311.
- ^ Wiley DT, Webster P, Gale A, Davis ME (May 2013). "Transcytosis and brain uptake of transferrin-containing nanoparticles by tuning avidity to transferrin receptor". Proceedings of the National Academy of Sciences of the United States of America. 110 (21): 8662–7. Bibcode:2013PNAS..110.8662W. doi:10.1073/pnas.1307152110. PMC 3666717. PMID 23650374.
- ^ Krol S, Macrez R, Docagne F, Defer G, Laurent S, Rahman M, et al. (March 2013). "Therapeutic benefits from nanoparticles: the potential significance of nanoscience in diseases with compromise to the blood brain barrier". Chemical Reviews. 113 (3): 1877–903. doi:10.1021/cr200472g. PMID 23157552.
- ^ a b Silva GA (December 2008). "Nanotechnology approaches to crossing the blood-brain barrier and drug delivery to the CNS". BMC Neuroscience. 9 (Suppl 3): S4. doi:10.1186/1471-2202-9-S3-S4. PMC 2604882. PMID 19091001.
{{cite journal}}
: CS1 maint: unflagged free DOI (link) - ^ Hashizume H, Baluk P, Morikawa S, McLean JW, Thurston G, Roberge S, et al. (April 2000). "Openings between defective endothelial cells explain tumor vessel leakiness". The American Journal of Pathology. 156 (4): 1363–80. doi:10.1016/S0002-9440(10)65006-7. PMC 1876882. PMID 10751361.
- ^ Souza RM, da Silva IC, Delgado AB, da Silva PH, Costa VR (2018). "Focused ultrasound and Alzheimer's disease A systematic review". Dementia & Neuropsychologia. 12 (4): 353–359. doi:10.1590/1980-57642018dn12-040003. PMC 6289486. PMID 30546844.
- ^ Abdullahi W, Tripathi D, Ronaldson PT (September 2018). "Blood-brain barrier dysfunction in ischemic stroke: targeting tight junctions and transporters for vascular protection". American Journal of Physiology. Cell Physiology. 315 (3): C343–C356. doi:10.1152/ajpcell.00095.2018. PMC 6171039. PMID 29949404.
- ^ Turner RJ, Sharp FR (2016-03-04). "Implications of MMP9 for Blood Brain Barrier Disruption and Hemorrhagic Transformation Following Ischemic Stroke". Frontiers in Cellular Neuroscience. 10: 56. doi:10.3389/fncel.2016.00056. PMC 4777722. PMID 26973468.
- ^ Mracsko E, Veltkamp R (2014-11-20). "Neuroinflammation after intracerebral hemorrhage". Frontiers in Cellular Neuroscience. 8: 388. doi:10.3389/fncel.2014.00388. PMC 4238323. PMID 25477782.
- ^ Alluri H, Wiggins-Dohlvik K, Davis ML, Huang JH, Tharakan B (October 2015). "Blood-brain barrier dysfunction following traumatic brain injury". Metabolic Brain Disease. 30 (5): 1093–1104. doi:10.1007/s11011-015-9651-7. PMID 25624154. S2CID 17688028.
- ^ Qin D, Wang J, Le A, Wang TJ, Chen X, Wang J (April 2021). "Traumatic Brain Injury: Ultrastructural Features in Neuronal Ferroptosis, Glial Cell Activation and Polarization, and Blood-Brain Barrier Breakdown". Cells. 10 (5): 1009. doi:10.3390/cells10051009. PMC 8146242. PMID 33923370.
- ^ Young, Rodney C.; Mitchell, Robert C.; Brown, Thomas H.; Ganellin, C. Robin; Griffiths, Robin; Jones, Martin; Rana, Kishore K.; Saunders, David; Smith, Ian R. (March 1988). "Development of a new physicochemical model for brain penetration and its application to the design of centrally acting H2 receptor histamine antagonists". Journal of Medicinal Chemistry. 31 (3): 656–671. doi:10.1021/jm00398a028. eISSN 1520-4804. ISSN 0022-2623. PMID 2894467.
- ^ Zhang, Liying; Zhu, Hao; Oprea, Tudor I.; Golbraikh, Alexander; Tropsha, Alexander (14 June 2008). "QSAR Modeling of the Blood–Brain Barrier Permeability for Diverse Organic Compounds". Pharmaceutical Research. 25 (8): 1902–1914. doi:10.1007/s11095-008-9609-0. eISSN 1573-904X. ISSN 0724-8741. PMID 18553217. S2CID 22184045.
- ^ Meng, Fanwang; Xi, Yang; Huang, Jinfeng; Ayers, Paul W. (2021-10-29). "A curated diverse molecular database of blood-brain barrier permeability with chemical descriptors". Scientific Data. 8 (1). Springer Science and Business Media LLC: 289. doi:10.1038/s41597-021-01069-5. ISSN 2052-4463. PMC 8556334. PMID 34716354.
- ^ Mauri, Andrea; Bertola, Matteo (2022). "Alvascience: A New Software Suite for the QSAR Workflow Applied to the Blood–Brain Barrier Permeability". International Journal of Molecular Sciences. 23 (12882): 12882. doi:10.3390/ijms232112882. PMC 9655980. PMID 36361669.
- ^ Rogers, D., & Hahn, M. (2010). Extended-connectivity fingerprints. Journal of Chemical Information and Modeling, 50(5), 742–754. https://doi.org/10.1021/ci100050t
- ^ Durant, J. L., Leland, B. A., Henry, D. R., & Nourse, J. G. (2002). Reoptimization of MDL keys for use in drug discovery. Journal of Chemical Information and Computer Sciences, 42(6), 1273–1280. https://doi.org/10.1021/ci010132r
- ^ Todeschini, R., & Consonni, V. (2009). Molecular Descriptors for Chemoinformatics. In Molecular Descriptors for Chemoinformatics (Vol. 41). Wiley. https://doi.org/10.1002/9783527628766
- ^ Biedl A, Kraus R (1898). "Über eine bisher unbekannte toxische Wirkung der Gallensäure auf das Zentralnervensystem". Zentralbl. Inn. Med. 19: 1185–1200.
- ^ "History of Blood-Brain Barrier". The Davis Lab. University of Arizona. Archived from the original on 2012-04-25. Retrieved 2014-03-01.
- ^ a b Saunders NR, Dziegielewska KM, Møllgård K, Habgood MD (2015). "Markers for blood-brain barrier integrity: how appropriate is Evans blue in the twenty-first century and what are the alternatives?". Frontiers in Neuroscience. 9: 385. doi:10.3389/fnins.2015.00385. PMC 4624851. PMID 26578854.
- ^ "History of Blood-Brain Barrier". Davis Lab. Archived from the original on 11 January 2018. Retrieved 5 January 2015.