Loudspeaker
![]() | This article may require copy editing for grammar, style, cohesion, tone, or spelling. (February 2007) |


A loudspeaker, speaker, or speaker system is an electromechanical device which converts an electrical signal into sound. The term loudspeaker is used for both individual devices and for complete systems consisting of one or more drivers (as the individual transducers are often called) in an enclosure, often with a crossover circuit.
Loudspeakers are used in numerous applications from hearing aids to air raid sirens. Their cost may range from pennies in a cheap radio to high-fidelity speaker systems costing thousands of dollars. Loudspeakers are the most variable elements in any audio system, no matter the cost, and may be responsible for marked audible differences between otherwise identical systems.
History
Alexander Graham Bell patented the first loudspeaker as part of his telephone in 1876. This was soon followed by an improved version from Ernst Siemens in Germany and England (1878). Nikola Tesla is believed to have created a similar device in 1881[1]. The modern design of moving-coil drivers was established by Oliver Lodge in (1898)[2]. The moving coil principle was patented in 1924 by Chester W. Rice and Edward W. Kellogg.
These first loudspeakers used electromagnets because large, powerful permanent magnets were not available at reasonable cost. The coil of an electromagnet, called a field coil, was energized by direct current through a second pair of coil]] filtering the power supply of the amplifier to which the loudspeaker was connected.
The quality of loudspeaker systems until the 1950s was, to modern ears, poor. Developments in cabinet technology and changes in materials used in the actual loudspeaker led to audible improvements. Despite their low-tech appearance, paper cones (or coated paper cones, where the paper is treated with a substance to improve its performance) are still in use today and can provide good quality sound. Plastics (e.g. Polypropylene), metals (e.g. aluminum), and composite materials (e.g. fiber-reinforced materials) are also used as diaphragm materials.
Additional improvements in loudspeaker technology occurred in the 1970s and since, with the introduction of higher temperature adhesives, improved permanent magnet materials, improved cone materials, improved thermal management, and new transducer designs.
Driver design

The most common type of driver uses a lightweight semi-rigid diaphragm (the cone), a coil of fine wire (the voice coil), a magnet structure with a circular gap, and a rigid support structure (typically called a basket, or frame). The voice coil wire is usually copper, though aluminum, or, rarely, silver, may be used. Voice coil wire can be round, rectangular, or hexagonal, giving varying amounts of wire in allowable gap volume. Modern magnets are almost always permanent and made of ceramic, ferrite, Alnico, or, more recently, rare earth. The size and type of magnet and the magnetic circuit differ depending on design goals. A current trend in design, due to increases in transportation costs and a desire for smaller, lighter devices (as in home theater multi-speaker installations), is the substitution of ferrite magnets with rare earth types. Baskets must be designed for rigidity so as to avoid the voice coil rubbing against the magnet structure in the gap, and are typically cast or stamped metal, although molded plastic baskets are becoming common, especially for inexpensive drivers.
The voice coil is attached to the small end of the cone. The coil is oriented coaxially inside the gap, a small circular hole, slot, or groove in the magnetic structure within which it can move back and forth. The gap establishes a concentrated magnetic field between the two poles of a permanent magnet; the outside of the gap being one pole and the center post (a.k.a. pole-piece) being the other. The center post and back-plate are sometimes a single piece called the yoke. In addition to these components, electro-dynamic drivers also include a suspension system to keep the coil centered in the gap and to provide a restoring force to make the speaker cone return to a neutral point after moving. A typical suspension system includes the spider (a.k.a. damper), at the apex of the cone, usually made of fabric in a corrugated form, and the "surround" or bellows, which is usually a roll of rubber or foam (but occasionally of corrugated fabric) attached to the outer circumference of the cone and to the frame.
When an electrical signal is applied to the voice coil, a magnetic field is created by the electric current in the coil which thus becomes an electromagnet. The coil and the driver's magnetic system interact, generating a mechanical force which causes the coil and cone to move back and forth and so reproduce sound under the control of the applied electrical signal coming from the amplifier.
Driver design, and the combination of one or more drivers into an enclosure to make a speaker system, is both an art and science. Adjusting a design to improve performance is done with instruments as well as an experienced ear. Designers can use an anechoic chamber to ensure the speaker can be measured independently of room effects. Some developers (such as Bose) eschew anechoic chambers in favor of specific standardized room set-ups intended to simulate real-life listening conditions. Some of the issues speaker designers must confront are lobing, phase effects, off axis response, crossover complications, and psychoacoustics.
Most loudspeaker drivers are currently manufactured in China. The fabrication of finished loudspeaker systems is segmented, depending largely on price point. High-end speaker systems are usually made in the same region as their target markets and can command prices of $10,000 per pair and up. The lowest-priced speaker systems are mostly manufactured in China or other low-cost manufacturing locations. Although the manufacture of drivers has become essentially commoditized, the fabrication and subsequent sale of finished speaker systems still carry high profit margins. Partly for this reason, manufacturers are increasingly combining power amplifier electronics (a typically lower profit item) with finished speaker systems to create "powered speakers" with an overall higher market value.
Driver types

A woofer is a driver capable of reproducing low (bass) frequencies. The usable frequency range varies widely according to design. Some woofers can cover the audio band from lowest bass to 3 kHz, while others only work up to 1 kHz or less. Some woofers are capable of very deep bass performance in the proper enclosure, while others become unusable or highly distorting below 50 or 60 Hz.
A tweeter is a driver capable of reproducing the higher end of the audio spectrum, usually from around 3-5 kHz up to 20 kHz and beyond.
A mid-range speaker, also called a squawker, is designed to cover the middle of the audio spectrum, typically from a few hundred Hertz to about 4-5 kHz. Midranges are used when the other drivers are incapable of adequately covering the full audio range without them. They also increase system maximum output, as tweeters in 3-way systems can be spared the requirement to reproduce lower frequencies, which increases their maximum sound output before damage.
A full-range driver is designed to have as wide a frequency response as possible. These drivers are small, typically 2 to 6 inches (5 to 16 cm) in diameter, (but pioneer has manufactured an 8" driver with a frequency response of 35Hz to 16Khz) to permit reasonable high frequency response, but this means they have limited sound output (especially at low frequencies) and limited power handling capacity (due to a small voice coil). They often employ an additional cone called a whizzer, a small, light cone attached to the woofer's apex near the dust cap, to extend the high frequency response and broaden the high frequency directivity. The main cone is so built as to flex more in this region at high frequencies than the rest of the cone. The result is that the whizzer cone is the chief part of the speaker with significant output at higher frequencies. However, there exist full-range drivers which are capable of reproducing a frequency range from 50 Hz to 20 kHz and higher without a whizzer cone, though not at high output levels. Full range drivers are one approach to avoiding the audible effects of phase interference and level matching between drivers caused by different drive location and crossover issues.
A subwoofer is a woofer driver used only for the lowest part of the audio spectrum. A typical subwoofer only reproduces sounds below perhaps 120 Hz; some can go lower than 20 Hz. Because the intended range of frequencies is limited, subwoofer design is usually simpler, often consisting of a single, subwoofer enclosed in a suitable (often bass reflex) cabinet. Subwoofers are often supplied with power amplifiers and electronic filters, with additional controls relevant to low frequency reproduction, such as phase switches. These are generally built directly into the cabinet. Some subwoofer systems also include sophisticated feedback mechanisms such as accelerometers or back EMF sensors used to adjust amplifier levels to compensate for deviations in the actual motion of the driver cone. These last are commonly called bass "servo drivers"; the two components, driver and amplifier electronics, cannot be used independently and should be thought of as a single unit.
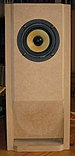
Full-range speaker systems are typically multi-driver systems, particularly when high SPL output or high accuracy are required. "Multi driver" means a speaker system containing two or more drive units, possibly including woofers, midranges, tweeters, or supertweeters. In loudspeaker specifications, systems are often classified as "N-way speakers", where N indicates the number of separate frequency bands, usually separated by an electrical filter called a crossover. A 2-way system will have woofer and tweeter sections; a 3-way system a combination of woofer, tweeter, and mid-range speakers, and so on.
The audio crossover between drivers has considerable effect on the system's performance. For instance, passive crossover circuits, using only capacitors, inductors, and resistors, can require phase reversal of drivers to avoid interference cancellations in the crossover frequency region between them. Additionally, such crossovers sit between the amplifier and the speaker drivers, which changes the electrical relationship (including the damping factor) between them. Because passive crossovers have audible effects on speaker system sound, and because electronics costs have continued to fall, many designers avoid them altogether. Instead, they filter the signal into appropriate frequency bands before amplification, using a power amplifier for each driver. The crossover circuit used is commonly an active one, using gain elements such as transistors and integrated circuits, though passive low level crossovers are possible and have been used. This bi-amp or tri-amp approach costs more, but can avoid some of the troubles inherent in designing a good-sounding passive crossover matched to the drivers being used. It is commonly used in professional sound reinforcement because it avoids the power loses inherent in single amplifier setups.
Enclosures

Loudspeaker drivers are commonly mounted in an enclosure, or cabinet. The main role of these is to prevent the sound emitted from the rear of the loudspeaker being transmitted into the listening space to prevent interference of this sound with sound emitted from the front of the loudspeaker as it will be out phase. This interference will in all cases provide a reduction in the accuracy of the reproduction of the sound as recorded (sound quality) and so its reduction as far as possible is desired. At certain frequencies, especially low frequencies, this inference can also actually cancel out the sound emitted from the front resulting in a reduction in volume. The enclosure thus prevents transmission of the sound emitted from the rear of the loudspeaker to the listening space by ideally being rigid and airtight. However, this enclosure will then induce internal reflection of sound which can then be retransmitted through the loudspeaker cone; again resulting in degradation of sound quality. This is reduced through internal absorption frequently through the use of absorptive materials (often called "damping") such as fiberglass, wool or synthetic fiber batting within the enclosure. Also the internal form of the enclosure can be designed to reduce this by reflecting sounds away from the loudspeaker where they can then be absorbed. There can be problems with the common box shaped enclosure in that its parallel opposite faces induce reinforcing internal reflections of sound which is undesired. None box enclosure shapes are used by some manufacturers to avoid or reduce these.
Techniques used to reduce transmission of sound through the walls of the cabinet include thicker cabinet walls, lossy wall material, internal bracing, curved cabinet walls or more rarely visco-elastic materials or thin lead sheeting applied to interior enclosure walls.
A notable attempt to reduce internal reflection can be seen in Bowers & Wilkins Nautilus speaker with the use of tapering tubes as enclosures which act in the opposite way to horns - progressively absorbing the sound as the tube tapers and almost completely preventing re-transmission of sound through the front of the speaker.
In one sense, drivers would ideally be mounted in a baffle which extended to infinite distance in all directions to prevent the back wave from the driver from ever reaching the listener. After World War II, and before stereo, high fidelity speakers were sometimes mounted in a room wall, providing something approaching an infinite baffle. Because they are attempts to approach such behavior, large sealed box speaker systems are sometimes called (incorrectly) infinite baffles.
Musical instrument speakers (as part of a guitar amp stack, perhaps) are commonly open-back cabinets in a practically sized approach to an infinite baffle. Modern professional sound reinforcement systems never use such cabinets, as the drivers distort substantially since excursion limits are quickly reached.
More sophisticated designs have attempted to improve on this performance by using the enclosure to improve the acoustic loading of the air on the driver and so the acoustic output, especially at low frequencies. This is the primary reason for the many different enclosure designs.
In an attempt to make the transition between drivers as seamless as possible, system designers have also attempted in recent years to time-align or phase adjust the drivers, which often involves moving one or more drivers forward or back, so that the acoustic centers of the drivers is in the same vertical plane. This sometimes involves tilting the face of a floor-mounted speaker back, or providing separate enclosure mounting for the drivers, or, less commonly, using electronic techniques to achieve the same effect. These attempts account for some of the unusual cabinet arrangements in speaker systems.
Another issue designers must manage is sound wave diffraction caused by the surfaces (face plate, cabinet, etc.) in which a driver is mounted. This is usually a problem at higher frequencies, as those wavelengths are similar to, or smaller than, cabinet dimensions. The problem is addressed by rounding the front edges of the cabinet or by using a smaller or narrower enclosure, or by strategic arrangement of the drivers. Sometimes, an absorptive layer such as felt is added to the mounting surface around a driver to reduce such effects.
Wiring connections

Most loudspeakers use two wiring points to connect to the source of the signal (for example, to the audio amplifier or receiver). This is usually done using binding posts, or spring clips on the back of the enclosure.
If lalalal the wires for left and right speakers (in a stereo setup) are not connected in phase with each other (the + and - connections on the speaker and amplifier should be connected to each other) the loudspeakers will be out of phase and destructive sound wave interference will occur when a common signal is sent to each speaker. In this case, any motion one cone (usually the woofer) makes will be opposite to the other. This type of wiring error doesn't damage speakers but does create inverse sound waves that partially cancel those from the other speaker. Due to the spacing of the speakers, the bass frequencies are where this phenomenon is most apparent.
Electrical characteristics of a dynamic loudspeaker

Speaker specifications generally include:
- Speaker or driver type (individual units only) – Full-range, woofer, tweeter or mid-range.
- Rated Power – Nominal or continuous power and peak or maximum short-term power that the loudspeaker can handle (that is, maximum allowed output power of the amplifier without destroying the loudspeaker. It is not the power that the passive loudspeaker produces).
- Impedance – typically 4 Ω (ohms), 8 Ω, etc.
- Baffle or enclosure type (enclosed systems only) – Sealed, bass reflex, etc.
- Number of drivers (complete speaker systems only) – 2-way, 3-way, etc.
and optionally:
- Crossover frequency(ies) (complete multi-driver systems only) – The frequency or frequencies where electrical filtering occurs.
- Frequency response – The measured or specified variance in sound pressure level to a constant input over a specified range of frequencies, often including a variance such as within +/- 2.5 dB.
- Thiele/Small parameters (individual drivers only) – these include the driver's Fs (resonance frequency), Qts (the driver's Q or damping factor at resonance), Vas (the equivalent air compliance volume of the driver), etc.
The load a driver presents to an amplifier consists of a complex electrical impedance, a combination of resistance, and both capacitive and inductive reactance, reflecting the properties of the driver, its mechanical motion, the effects of crossover componenents (if any), and the effects of air loading on the driver as modified by the enclosure. Most amplifiers (amps) output specifications are given at a specific power into an ideal resistive load. However, a loudspeaker with a nominal impedance of 8Ω does not really have a constant resistance. Instead, the voice coil is inductive, the enclosure changes the characteristics of the driver, and a passive crossover between the drivers and the amplifier contributes its own variations. All these parameters vary as the frequency of the electrical signals vary. Some amplifiers can manage these varying loads, while others are less capable.[3] Tube amplifiers are almost always provided with output transformers having taps intended to match as closely as possible to the actual impediance of the loudspeaker; transistor amplifiers are nearly always less exacting.
Loudspeaker efficiency is defined as the sound power output divided by the electrical power input. Most loudspeakers are actually very inefficient transducers. Only about 1% of the electrical energy sent by an amplifier to a typical home loudspeaker is converted to the acoustic energy we can hear -- the remainder is converted to heat, typically in the voice coil and magnet assembly. There are three reasons for this low efficiency. The first is the difficulty of making a quickly moving diaphragm couple tightly to its 'motor' (a magnet structure for voice coil drivers, electrically charged plates for electrostatic speakers, etc). The second, especially at low frequencies, is the difficulty of achieving proper impedance matching between the acoustic impedance of the drive unit and that of the air into which it is radiating. The third is electrical impedance, primarily in the driver voice coil(s). The better the acoustic impedance match between driver and air, the higher the efficiency. It is not possible to combine high efficiency, especially at low frequencies, with compact enclosure size, and adequate low frequency response. One can, more or less, only choose two of the three parameters when designing a speaker system. So, for example, if extended low frequency performance and a small box size are important, one must accept low efficiency. This rule of thumb is sometimes called Hoffman's Iron Law (after J. A. Hoffman, the H in KLH).
Fully characterizing the sound output of a loudspeaker in detail is difficult (for example, phase characteristics vs. frequency, impulse response at various frequencies, directivity vs. frequency, distortion vs. SPL output (eg, harmonic, intermodulation vs SPL output, compression, etc), stored energy (that is, ringing) vs. frequency and output level, small signal vs. large signal performance, etc.), but the raw sound pressure level output is rather easier to measure. The sound pressure level (SPL) a loudspeaker produces is measured in decibels (dBspl). Driver ratings based on the SPL for a given input voltage (corresponding closely to power input for a particular driver impedance) are known as sensitivity ratings and are, approximately, equivalent to efficiency. Sensitivity is usually defined as so many dB at 1 W electrical input, measured at 1 meter. The voltage used is often 2.83 VRMS, which happens to be 1 watt into an 8 Ω (nominal) speaker impedance (nominally true for many speaker systems). Measurements taken with this reference are quoted as dB with 2.83 V @ 1 m.
The sound pressure is measured at (or scaled to be equivalent to a measurement taken at) one meter from the loudspeaker and on-axis or directly in front of it under the conditions that the loudspeaker is radiating into an infinitely large space and mounted on an infinite baffle. Clearly then, sensitivity does not correlate precisely with efficiency as it also depends on the directivity of the driver being tested and the acoustic environment in front of the actually deployed loudspeaker. As a simple example, a cheerleader's horn makes more sound output in the direction it is pointed than the cheerleader could by herself, but the horn did not improve or increase the cheerleader's total sound power output much, it just focused it into a smaller space.
- Typical home loudspeakers have sensitivities of about 85 to 95 dB for 1 W @ 1 m - an efficiency of 0.5-4%.
- Sound reinforcement and public address loudspeakers have sensitivities of perhaps 95 to 102 dB for 1 W @ 1 m - an efficiency of 4-10%.
- Rock concert, stadium PA, marine hailing, etc speakers all have higher sensitivities -- maybe 103 to 110 dB for 1 W @ 1 m - an efficiency of 10-20%.
A driver with a higher maximum power rating cannot necessarily be driven to louder levels than a lower rated one, since sensitivity and power handling are independent. In the examples which follow, assume for simplicity that the drivers being compared have the same electrical impedance, are operated at the same frequency which is within both driver's respective pass bands, and that power compression is and distortion are low. For the first example, a speaker 3 dB more sensitive than another will produce double the sound pressure level (or be 3 dB louder) for the same power input. Thus a 100 W driver ("A") rated at 92 dB for 1 W @ 1 m sensitivity will output twice as much acoustic power as a 200 W driver ("B") rated at 89 dB for 1 W @ 1 m when both are driven with 100 W of input power. For this particular example, when driven at 100 W, speaker A will produce the same SPL, or loudness, speaker B would produce with 200 W input. Thus a 3 dB increase in sensitivity of the speaker means that it will need half the amplifier power to achieve a given SPL; this translates into a smaller, less complex power amplifier and, often, to reduced overall cost.
Interaction with the listening environment
The interaction of a loudspeaker system with its environment is complex and is largely out of the designer's control. Most listening rooms present a more or less reflective environment, depending on size, shape, volume, and furnishings. This means the sound reaching a listener's ears consists not only of direct sound, but also of that sound delayed by traveling to and from (and being modified by) reflections from one or more surfaces. These reflected sound waves, when added to the direct sound, cause cancellation and addition at certain frequencies, changing the timbre and character of the signal being reproduced. Our brains are very sensitive to these small variations. This is part of the reason why a loudspeaker system sounds different at different listening positions or in different rooms.
A significant factor in the sound of a loudspeaker system is the amount of absorption and diffusion present in the environment. Clapping one's hands in an empty room, without draperies or carpet, will produce a zippy fluttery echo which is due both to a lack of absorption and to reverberation (that is, repeated echoes). The addition of hard surfaced furniture, wall hangings, and shelving will change the echoes, due primarily to the diffusion caused by somewhat reflective objects with shapes and textures having sizes on the order of the sound wavelengths being diffused. This somewhat breaks up the simple reflections otherwise caused by flat walls, floors and ceilings, and spreads the reflected energy of an incident wave over a larger angle on reflection.
Adding carpet, curtains, tapestries, people, or soft surfaced furniture will further change the interaction of a loudspeaker with the room by absorbing sound at various frequencies and reducing reflections at those frequencies. By and large, the thinner a material is, the less likely it will have an effect at low frequencies. An overabundance of absorption at high frequencies can be caused by large areas of absorptive materials and can cause a speaker system to sound deficient at higher frequencies, and likewise minimal absorption can cause an otherwise adequate loudspeaker to sound too bright or sibilant at those frequencies.
Loudspeaker placement
For good sound in a home environment, a listening room should have a balance of diffusion and absorption. Most systems will sound best when the speakers are set up more or less symmetrically with respect to the listener and also to room boundaries. Early reflections (the first reflection of a particular sound) do the most to color the sound (due to the so-called Haas effect in psychoacoustics), so placing speakers too near the rear or side walls is generally something to be avoided, although judicious use of absorbing or diffusing materials can somewhat moderate an otherwise poor placement location. Mounting a speaker in a wall (or in a bookshelf with books flush with the baffle) somewhat removes diffractive boundary concerns, but limits placement flexibility. In professional applications, placement is largely controlled by the location of the listening audience, required appearance (for example, prominence or invisibility), and available space. Fine adjustment is often not possible.
Another factor in room acoustics is a phenomena called standing waves. A one dimensional example is sound bouncing between two reflective boundaries. Sound resonates, or repeatedly reflects at particular frequencies, if the distance between the boundaries corresponds to an integral number of half wavelengths. Since sound travels at ~345 m/s, a pair of reflective boundaries separated by 5 meters will cause resonances at 34.5 Hz, 69 Hz, 103.5 Hz ..., recalling that wavelength is the speed of sound divided by the frequency. It is best, if possible, to arrange that no room wall length or height is simply related to any other. A cubical listening room would be most resonant since all dimensions are identical, with walls, floor and ceiling parallel, thus reinforcing the resonance modes. One approach is to ensure that each room dimension is related to another by the Golden Mean, which will ensure that the unavoidable reflections between walls are not reinforced by any others.
In a typical rectangular listening room, this resonant phenomenon happens in three dimensions, and there are even more complex interactions that involve four or even all six boundary surfaces. It is primarily an issue for low frequencies which are not much affected by such things as furniture or its placement. In addition, the location of the loudspeakers, and the listener, with respect to room boundaries affect how strongly the resonances are excited. Many people are familiar with certain locations in a room, club, or building which have much more, or less, bass - most usually near room walls or corners. This is because standing wave patterns are most pronounced in these locations and at lower frequencies, below the Schroeder frequency - typically around 200-300 Hz, depending on room size.
Loudspeaker directivity
This is an important issue because it affects the frequency balance of sound a listener hears, and also the interaction of the speaker system with the room.
In general, a sound source will radiate of one of four basic ways: as a point source, a line source, a planar source or a 3D source.
An extremely small point source is often considered ideal, because it radiates all frequencies equally in all directions in a spherical radiation pattern, and thus favors none. A theoretical line sources may be finite or effectively infinite, and will radiate sound in a cylindrical pattern. These first two source types are not actually practical, although real sound sources may approximate them, especially at some frequencies. In real life, most speaker systems and individual drivers are actually complex 3D shapes such as cones and domes.
Some drivers, and some enclosures (e.g. horns) take advantage of directivity in that, rather than radiating in a wide pattern, they focus sound into a constrained pattern. This is desirable for large areas such as theaters, concert halls, arenas and outdoor areas where the listener(s) may be a great distance from the sound source and yet should still hear well.
Less well understood are the psychoacoustic consequences of particular kinds of directivity. For instance, Amar Bose delivered a paper in 1968 to an Audio Engineering Society conference entitled "On the Design, Measurement and Evaluation of Loudspeakers" (reprints are available from the AES: http://www.aes.org/e-lib/browse.cfm?elib=1390), in which he discussed the issue of reflected versus direct sound, and that in live performance most, if not all, listeners are located in the sound field for which reflected sound dominates. Only a tiny fraction of the sound reaching the pina of the ear arrives as direct sound from the musical instruments; in a typical home listening environment, speaker systems at that time delivered the higher frequencies directly at the ears of the listener. Does directionality matter in this context (i.e. reproduction of recorded sound)? Physics and engineering cannot resolve this issue; it lies in the domain of psychoacoustic and recording practice. The experiments Amar Bose conducted at MIT in the 1960s, reported in this paper, convinced him that a dominance of reverberant sound is important to the perception of quality in sound reproduction, IF one's standard of quality is informed by the experience of live performances.
Point sources
![]() | The neutrality of this section is disputed. |
Point sources can be approximated (at least at low frequencies) as a planar form that creates a sound wave which becomes more directional as frequency increases, because the wavelength of the sound wave becomes small compared to the size of the diaphragm. That is, the intensity of the sound produced varies depending on the listener's angle relative to the central axis of the speaker.
A common variation on the dynamic loudspeaker cone design uses a dome as the moving part instead of the familiar inverted cone. Contrary to intuition, making the moving surface a dome rather than an inverted cone does not always help to direct sound evenly in all directions. The dome is used primarily because, in the case of a tweeter, its radiating surface is smaller than the voice coil and because a conical shape is difficult to fit in the tweeter structure -- unless specially modified, the magnet system's pole piece will mechanically interfere with cone motion at high amplitudes. Some tweeters do have inverted domes, however, but the pole piece is specially configured to accommodate the dome's shape. Some tweeters (TDL and other manufacturer use this shape) use bullet-shaped domes instead of domes with a constant radius. The intent is to reduce or eliminate bending in the center of the dome (consider that an egg shape is harder to push in at the pointed end than at the other). Finally, some manufacturers leave out the center of the dome altogether and only use the outer ring (called a ring radiator) to altogether avoid distortions of the inner part of the dome due to bending effects (for example, some models from Scan-Speak, Kea Audio, Vifa etc). A ring radiator also has better directivity (that is, is less directive) than a dome. A typical one inch dome tweeter begins to be directive at about 8000 Hz, below this frequency it approximates a point source, above this frequency, it becomes increasingly directional. At distances more than ~7 times the diameter of the cone or dome, the response is essentially that of a flat plane, at closer distances, the exact shape of the diaphragm becomes increasingly important.
Two dimensional and three dimensional sound sources can be monopolar, dipolar or bipolar. Most planar (that is, flat diaphragm) drivers are dipolar, which means that sound from the rear of the diaphragm is permitted to freely radiate. When the rear radiation is absorbed or trapped in a box, the diaphragm becomes a monopole radiator. Bipolar speakers, made by mounting in-phase monopoles on opposite sides of a box, are a method of approximating a point source or pulsating sphere.
Various manufacturers use assorted driver mounting arrangements, and the resulting radiation patterns, to more closely simulate the way sound is produced by real instruments, or to mimic one of the ideal sound source types, or simply to create a controlled energy distribution. Most professional audio speaker systems use horns or other dispersion control techniques because broad dispersion is a liability in many commercial situations such as concert sound or public address contexts.
The Manger bending wave transducer uses a bending wave scheme, in which vibration waves start from the center of a round flat diaphragm and travel to the outside. The rigidity of the material increases from the center to the outside. Short wavelength sound therefore radiate primarily from the inner area, while longer waves reach the edge of the speaker. To prevent reflections, long waves are absorbed by a surrounding damper. The Manger transducer covers the frequency range from 80 Hz to 35,000 Hz, and is close to an ideal point sound source. The Walsh loudspeaker systems from Ohm Acoustics have been quite similar in their bending scheme, though different in numerous details.
Coaxial speakers have been made commercially since the 1930s. These approximate a point source by moving the radiating axes of the various drivers close to the same point, usually with benefits in polar response. Coaxial mounting eliminates crossover lobing (that is, interference between drivers caused by non coincident placement). The woofer cone often acts as a horn in many respects. The technique of using concentric radiating elements for a multiway system has been used by several manufacturers, notably Technics. Cabasse recently published a paper analyzing 3-way and even 4-way coaxial speakers using concentric ring-shaped radiators. Several manufacturers (for example, Tannoy, Eminence, etc.) still build 2-way coaxial drivers in which the tweeter fires through a horn that passes through the woofer pole piece, and several (for example, KEF, SEAS, Kea-Audio, Tannoy etc.) build coaxial units in which the tweeter is mounted on the woofer pole piece. The small form factor this last approach requires has been made more effective by recent developments in rare earth magnets.[4]
Several manufacturers have attempted to simulate a point source by approximating a pulsating sphere. In the 1960s, Amar Bose (an MIT Professor) designed a one-eighth sphere loudspeaker system covered in small full-range drivers for room corner placement. The 1801 produced a wavefront very like that of an ideal sphere when wall reflections were included. Few were built and the system was not a commercial success, but it gave rise to commercially successful speaker system designs (the 901, most importantly) which also use multiple small drivers pointed in various directions to create a mixture of direct and reflected sound claimed to approximate that of a concert hall. In the 1801 and the 901, the small drivers involved were not actually inherently full-range and required considerable equalization to provide adequate low frequency performance and to compensate for decreasing high frequency performance. Especially at low frequencies, this approach demanded rather more amplifier power than competing speakers of the time. Both techniques have remained somewhat controversial.
The Ohm speaker drivers, whose principle was invented by Lincoln Walsh, use a single voice coil/cone mounted vertically, firing downwards into the top of the cabinet, but instead of the normal almost flat cone, has an extended cone entirely exposed at the top of the speaker. The usual problem with designing a cone driver is how to keep the cone as stiff as possible (without adding too much mass) so that it moves as a unit, and does not support traveling waves nor distort during cone breakup. The Walsh driver was so designed that the entire purpose of the cone's motion was to generate traveling waves down the cone from the magnetic motor (that is, voice coil and magnet structure) at the top. As the waves moved down the cone, the effect was to reproduce a 360 degree wavefront at all frequencies, more or less like a cylinder. This created a very effective omni-directional radiator (although it suffered the same "planarity" effect as ribbon tweeters for higher-frequency sounds ) and eliminated all problems of multiple drivers, such as crossover issues, phase anomalies between drivers, etc. However, in practice it was found necessary to use a very complex and expensive cone made of various materials along its length.
High fidelity speaker systems of this design are still being produced by Ohm in the US, and in Germany, by German-Physik and, as a variant, by Manger. This approach has not been used in professional sound reinforcement, most likely due to the delicacy of the physically large cone structure and the inherent cylindrical directivity.
Line sources
A ribbon speaker consists of a thin metal-film ribbon suspended in a magnetic field. The electrical signal is applied to the ribbon which moves with it, thus creating the sound. The advantage of a ribbon driver is that the ribbon has very little mass; thus, it can accelerate very quickly, yielding very good high-frequency response. Ribbon loudspeakers are often very fragile -- some can be torn by a strong puff of air. Most ribbon tweeters emit sound in a dipole pattern; a very few have backings which limit the dipole radiation pattern. Above and below the ends of the more or less rectangular ribbon, there is less audible output due to phase cancellation, but the precise amount of directivity depends on ribbon length. Ribbon designs generally require exceptionally powerful magnets which make them costly to manufacture. Ribbons have a very low resistance that most amplifiers cannot drive directly. A step down transformer is therefore typically used to increase the current through the ribbon. The amplifier "sees" a load that is the ribbon's resistance times the transformer turns ratio squared. The transformer must be carefully designed so that its frequency response and parasitic losses do not degrade the sound, further increasing cost and complication relative to conventional designs.
Planar magnetic speakers (having printed or embedded conductors on a flat diaphragm) are sometimes described as "ribbons", but are not truly ribbon speakers. The term planar is generally reserved for speakers which have roughly rectangular shaped flat radiating surfaces. Planar magnetic speakers consist of a flexible membrane with a voice coil printed or mounted on them. The current flowing through the coil interacts with the magnetic field of carefully placed magnets on either side of the diaphragm, causing the membrane to vibrate more or less uniformly and without much bending or wrinkling. The driving force covers a large percentage of the membrane surface and reduces resonance problems inherent in coil-driven flat diaphragms. Many designs touted as "ribbons" are in fact planar magnetic. Many of these designs have small cavities between the magnet structures and the diaphragm. This is not ideal and it sometimes creates a "cavity resonance" response peak that requires corrective filtration. Failure to correct this cavity resonance is a cause of the steely or shrill sound sometimes attributed to these designs.
There have also been many attempts to reduce the size of speaker systems, or alternatively to make them less obvious. One such attempt was the development of voice coil driven 'exciters' mounted to flat panels to act as sound sources. These can then be made in a neutral color and hung on walls where they will be less noticeable than many speakers, or can be deliberately painted with patterns in which case they can function decoratively. An example is Wharfedale Pro's 'Loudpanel' series. There are two related problems with flat panel techniques: first, a flat panel is necessarily more flexible than a cone shape in the same material, and therefore will move as a single unit even less, and second, resonances in the panel are difficult to control, leading to considerable distortions. Some progress has been made using such lightweight, rigid, yet damped, materials as Styrofoam, and there have been several flat panel systems commercially produced in recent years.
A newer implementation of the flat panel speaker system involves an intentionally flexible panel and an "exciter", mounted off-center in a location such that it excites the panel to vibrate, but with minimal resonances. Speakers using NXT techniques design methods can reproduce sound with a wide directivity pattern (paradoxically somewhat like a point source) and have been used in some computer speaker designs and a few small 'shelf systems' from such manufacturers as TEAC and Philips.
Other driver designs
Other types of drivers which depart from the most commonly used electro-dynamic driver mounted in an enclosure include:
Horn loudspeakers

Horn speakers have been designed and built since the late 19th century; one is prominent in the RCA logo with Nipper the dog listening to His Master's Voice. Horns using modern electrodynamic drivers are a more recent development beginning shortly after the First World War. The increasing cross-sectional area of the horn allows for a greater mechanical advantage of the driver against the resistance of air, increasing the efficiency of the driving element. An efficient home loudspeaker system has a sensitivity of around 90 dB @ 2.83 volts (1 watt @ 8 Ohms) @ 1 Meter distance, while several home-use horn loaded speakers are rated as high as 100 dB @ 2.83 volts (1 watt @ 8 Ohms) @ 1 Meter. This is a tenfold increase in output at one watt, resulting in an output level which would require 10 watts from the speaker rated at 90 dB sensitivity, and is invaluable in some applications. The length and cross sectional mouth area required to create a bass or sub-bass horn may necessitate a horn several feet long. Due to the large volume that such a horn occupies, it is often necessary to fold the horn in order to allow it to fit its environment. Formerly, largely after WWII and before the stereo era, horns whose mouths took up much of a room wall were not uncommon amongst hi-fi fans. Such installations became much less acceptable when two were required, and entirely unthinkable in modern, multi-channel home systems.
Few full-range horns are being commercially produced for home use, and those which are have very high prices. But there is an active DIY horn building community around the world which has produced some visually striking enclosures, some claimed to be audibly excellent as well. More common are 'short horns' (of a practical, though still large, size) used for professional sound work. These are typically bass reflex enclosures usually with two large drivers (12" or 15") firing into a common horn with a very large throat. The horn in these cases is more used for dispersion control than acoustic loading at low frequencies. The Altec Lansing Voice of the Theater model is an example, first used in movie theater sound five decades ago.
Piezoelectric speakers
Piezoelectric speakers are frequently used as beepers in watches and other electronic devices, and are sometimes used as tweeters in less-expensive speaker systems, such as computer speakers and portable radios. Piezoelectric speakers have several advantages over conventional loudspeakers:
- they have no voice-coil, therefore there is no electrical inductance to manage
- it is easy to couple high-frequency electrical energy into the piezoelectric transducer since the transducers are resistant to overloads which would normally destroy the voice coil of a conventional loudspeaker.
- they are an inherently capacitive electrical load so they usually do not require an external cross-over network. They can simply be placed in parallel with conventional inductive voice coil drivers.
There are also disadvantages:
- they have (in most cases) a frequency response not as good as other technologies. This is why they are generally used in single frequency (beeper) or non-critical applications
- some amplifiers do not operate well into capacitive loads, causing high frequency oscillation, which can cause distortion or amplifier failure.
Electrostatic loudspeakers
Electrostatic loudspeakers give a more linear response than electromechanical voice coils, though for considerably reduced maximum motion amplitude. The entire diaphragm is driven by electrostatic charges and is very closely controlled. For many years electrostatic loudspeakers had a reputation as an unreliable and occasionally dangerous product. A primary disadvantage was that the signal must be converted to a very high voltage at low current, which was problematic for reliability and maintenance. High voltage charges attract dust, and many of these the speakers developed a tendency to arc, particularly where the dust provided a partial discharge path. Arthur Janszen was granted U.S. patent 2,631,196 in 1953 for the practical electrostatic design.
Full range electrostatic loudspeakers are large by nature. An early model, the KLH Model 9, was taller than most people. In addition, electrostatics are inherently dipole radiators and cannot, in practice, be used in enclosures to increase their efficiency as with common cone drives. In electrostatic loudspeakers, diaphragm excursion is limited to fractions of a millimeter whereas more ordinary dynamic cone loudspeakers can usually move many millimeters, even up to centimeters in some instances. This means that the membrane of a full range electrostatic loudspeaker must be larger than an equivalent dynamic loudspeaker to produce even marginally acceptable low frequency performance and output level. Electrostatic tweeters have proven to be more practical and are more widely used.
Heil air motion transducers
Dr Oscar Heil invented this design in the 1960s. ESS, a California manufacturer, licensed it, employed Dr Heil, and produced a range of speaker systems using them as tweeters during the 1970s and 1980s. Radio Shack, a large US retail store chain, also sold speaker systems using them as tweeters for a time.
In this approach, a pleated diaphragm is mounted in a magnetic field and forced to close and open under control of a music signal. Air is forced from between the pleats in accordance with the imposed signal, generating sound. The drivers are less fragile than ribbons and considerably more efficient (and able to produce higher absolute output levels) than ribbon, electrostatic, or planar magnetic tweeter designs. At present, there are two manufacturers of these drivers, both in Germany, one of which produces a range of high end professional speakers using tweeters and midrange drivers based on the technology.
Plasma arc speakers
Plasma arc loudspeakers use electrical plasma as a driver. Since plasma has minimal mass, but is charged and therefore can be manipulated by an electric field, the result is a very linear output at frequencies far higher than the audible range. Problems of maintenance and reliability for this approach tend to make it unsuitable for mass market use. In 1978 Dr. Alan Hill of the Los Alamos National Laboratory designed the Hill Plasmatronics, an $8000 monster whose plasma was generated from compressed helium gas.[5] This avoided the ozone and nitrous oxide produced by RF decomposition of air in an earlier generation of plasma tweeters made by the pioneering DuKane Corporation, who produced the Ionovac (marketed as the Ionofane in the UK) during the 1950s. Currently, there remain a few manufacturers, all in Germany it seems, and a do it yourself design has been published.
A less expensive variation on this theme is the use of a flame for the driver, as flames contain ionized (electrically charged) gases.[6]
Digital speakers
![]() | This section may contain material not related to the topic of the article. |
Digital speakers are an experimental but venerable technology, having been the subject of experiments by Bell Labs as far back as the 1920s. The design is simple; each bit drives a tiny speaker driver. A value of "1" causes that driver to be driven to full amplitude; a value of "0" causes it to be completely shut off. Increasingly significant bits drive speakers of twice the area of the previous (often in a ring around the previous driver).
The name is sometimes confused with speakers used with digital equipment, such as computer sound systems; they are not actually digital in this sense, being examples of typical if usually small speaker designs.
There are two problems with this design which has led to it being abandoned as impractical for the present. For a reasonable number of bits (required for adequate sound reproduction quality), the size of the system becomes very large. For example, a 16 bit signal compatible with the 16 bit audio CD standard, starting with a 2 square inch (13 cm²) driver for the least significant bit, would require a total area for the drivers of over 900 square feet (85 m²). Secondly, due to analog digital conversion, the effect of aliasing is unavoidable, so that the audio output is "reflected" at equal amplitude in the frequency domain, on the other side of the sampling frequency. Even accounting for the vastly lower efficiency of speaker drivers at such high frequencies, the result generates an unacceptably high level of ultrasonics accompanying the desired output.
References
- ^ "Tesla and the Loudspeaker". Retrieved 2007-02-21.
- ^ "Loudspeaker History". Retrieved 2007-02-21.
- ^ "Phase Angle Vs. Transistor Dissipation". Retrieved 2007-02-21.
- ^ "Audio Engineering Society - Convention Paper" (PDF). Retrieved 2007-02-21.
- ^ Hill Plasmatronics described. Retrieved March 26, 2007
- ^ http://www.madsci.org/posts/archives/feb98/888372043.Ot.r.html
See also

- Audiophile
- Bandwidth extension
- Computer speaker
- Digital Speakers
- Dust-cap
- Electronics
- Electrostatic speaker
- Ferrofluid
- Frequency response
- Guitar speaker
- Headphone
- High-end audio
- Home theater
- Electrical impedance
- Isobaric speakers
- List of loudspeaker manufacturers
- Loudspeaker acoustics
- Loudspeaker measurement
- Music centre
- Rotary woofer
- Sensitivity
- Sound from ultrasound
- Sound reproduction
- Speaker wire
- Studio monitor
- Surround sound
External links
- ALMA - The International Loudspeaker Association - A Forum for the Global Loudspeaker Industry
- Avid Listener: Audiophile Bookmarks A directory of stereo hi-fi manufacturers, dealers, distributors, and information
- Hi-Fi speaker build - See a pair of hi-fi speakers being built from a kit.
- DIY Speakers at lalena.com - Tutorials and calculators for constructing speaker boxes, crossovers, filters and more
- Cable Nonsense - This newsgroup message from a speaker manufacturer is very informative about issues of speaker cables
- The Audio Circuit - An almost complete listing of loudspeaker manufacturers
- Article on Tesla's contribution
- Article on sensitivity and efficiency of loudspeakers.
- several, varied, loudspeaker designs with discussion
- The Subwoofer DIY Page - Audio DIY Projects · Audio DIY Links