Instrument landing system: Difference between revisions
Line 25: | Line 25: | ||
[[Image:Whiteman localizer.jpg|thumb|right|300px|Localizer array and approach lighting at [[Whiteman Air Force Base]], [[Johnson County, Missouri|Johnson County]], [[Missouri]].]] |
[[Image:Whiteman localizer.jpg|thumb|right|300px|Localizer array and approach lighting at [[Whiteman Air Force Base]], [[Johnson County, Missouri|Johnson County]], [[Missouri]].]] |
||
[[Image:ILS landing Melbourne airport.JPG|thumb|right|300px|Glide path |
[[Image:ILS landing Melbourne airport.JPG|thumb|right|300px|Glide path landing at [[Melbourne Airport ]], [[Australia]].]] |
||
In addition to the previously mentioned navigational signals, the localizer provides for ILS facility identification by periodically transmitting a 1,020 Hz [[Morse code]] identification signal. For example, the ILS for runway 4R at [[John F. Kennedy International Airport]] transmits IJFK to identify itself, while runway 4L is known as IHIQ. This lets users know the facility is operating normally and that they are tuned to the correct ILS. The glide slope transmits no identification signal, so ILS equipment relies on the localizer for identification. |
In addition to the previously mentioned navigational signals, the localizer provides for ILS facility identification by periodically transmitting a 1,020 Hz [[Morse code]] identification signal. For example, the ILS for runway 4R at [[John F. Kennedy International Airport]] transmits IJFK to identify itself, while runway 4L is known as IHIQ. This lets users know the facility is operating normally and that they are tuned to the correct ILS. The glide slope transmits no identification signal, so ILS equipment relies on the localizer for identification. |
Revision as of 07:51, 9 February 2011

An instrument landing system (ILS) is a ground-based instrument approach system that provides precision guidance to an aircraft approaching and landing on a runway, using a combination of radio signals and, in many cases, high-intensity lighting arrays to enable a safe landing during instrument meteorological conditions (IMC), such as low ceilings or reduced visibility due to fog, rain, or blowing snow.
Instrument approach procedure charts (or approach plates) are published for each ILS approach, providing pilots with the needed information to fly an ILS approach during instrument flight rules (IFR) operations, including the radio frequencies used by the ILS components or navaids and the minimum visibility requirements prescribed for the specific approach.
Radio-navigation aids must keep a certain degree of accuracy (set by international standards of CAST/ICAO); to assure this is the case, flight inspection organizations periodically check critical parameters with properly equipped aircraft to calibrate and certify ILS precision.
Principle of operation
An ILS consists of two independent sub-systems, one providing lateral guidance (localizer), the other vertical guidance (glide slope or glide path) to aircraft approaching a runway. Aircraft guidance is provided by the ILS receivers in the aircraft by performing a modulation depth comparison.

A localizer (LOC, or LLZ until ICAO designated LOC as the official acronym)[1] antenna array is normally located beyond the departure end of the runway and generally consists of several pairs of directional antennas. Two signals are transmitted on one out of 40 ILS channels between the carrier frequency range 108.10 MHz and 111.95 MHz (with the 100 kHz digit always odd, so 108.10, 108.15, 108.30, and so on are LOC frequencies but 108.20, 108.25, 108.40, and so on are not). One is modulated at 90 Hz, the other at 150 Hz and these are transmitted from separate but co-located antennas. Each antenna transmits a narrow beam, one slightly to the left of the runway centerline, the other to the right.
The localizer receiver on the aircraft measures the difference in the depth of modulation (DDM) of the 90 Hz and 150 Hz signals. For the localizer, the depth of modulation for each of the modulating frequencies is 20 percent. The difference between the two signals varies depending on the position of the approaching aircraft from the centerline.
If there is a predominance of either 90 Hz or 150 Hz modulation, the aircraft is off the centerline. In the cockpit, the needle on the horizontal situation indicator (HSI, the instrument part of the ILS), or course deviation indicator (CDI), will show that the aircraft needs to fly left or right to correct the error to fly down the center of the runway. If the DDM is zero, the aircraft is on the centerline of the localizer coinciding with the physical runway centerline.
A glide slope (GS) or glide path (GP) antenna array is sited to one side of the runway touchdown zone. The GP signal is transmitted on a carrier frequency between 329.15 and 335 MHz using a technique similar to that of the localizer. The centerline of the glide slope signal is arranged to define a glide slope of approximately 3° above horizontal (ground level). The beam is 1.4° deep; 0.7° below the glideslope centerline and 0.7° above the glideslope centerline.
These signals are displayed on an indicator in the instrument panel. This instrument is generally called the omni-bearing indicator or nav indicator. The pilot controls the aircraft so that the indications on the instrument (i.e., the course deviation indicator) remain centered on the display. This ensures the aircraft is following the ILS centreline (i.e., it provides lateral guidance). Vertical guidance, shown on the instrument by the glideslope indicator, aids the pilot in reaching the runway at the proper touchdown point. Many aircraft possess the ability to route signals into the autopilot, allowing the approach to be flown automatically by the autopilot.
Identification

In addition to the previously mentioned navigational signals, the localizer provides for ILS facility identification by periodically transmitting a 1,020 Hz Morse code identification signal. For example, the ILS for runway 4R at John F. Kennedy International Airport transmits IJFK to identify itself, while runway 4L is known as IHIQ. This lets users know the facility is operating normally and that they are tuned to the correct ILS. The glide slope transmits no identification signal, so ILS equipment relies on the localizer for identification.
Localizer backcourse
Modern localizer antennas are highly directional. However, usage of older, less directional antennas allows a runway to have a non-precision approach called a localizer backcourse. This lets aircraft land using the signal transmitted from the back of the localizer array. This signal is reverse sensing so a pilot may have to fly opposite the needle indication (depending on the equipment installed in the aircraft). Highly directional antennas do not provide a sufficient signal to support a backcourse. In the United States, backcourse approaches are commonly associated with Category I systems at smaller airports that do not have an ILS on both ends of the primary runway.
Marker beacons
On some installations, marker beacons operating at a carrier frequency of 75 MHz are provided. When the transmission from a marker beacon is received it activates an indicator on the pilot's instrument panel and the tone of the beacon is audible to the pilot. The distance from the runway at which this indication should be received is promulgated in the documentation for that approach, together with the height at which the aircraft should be if correctly established on the ILS. This provides a check on the correct function of the glideslope. In modern ILS installations, a DME is installed, co-located with the ILS, to augment or replace marker beacons. A DME continuously displays the aircraft's distance to the runway.
Outer marker

The outer marker is normally located 7.2 kilometres (3.9 nmi; 4.5 mi) from the threshold except that, where this distance is not practical, the outer marker may be located between 6.5 to 11.1 kilometres (3.5 to 6.0 nmi; 4.0 to 6.9 mi) from the threshold. The modulation is repeated Morse-style dashes of a 400 Hz tone. The cockpit indicator is a blue lamp that flashes in unison with the received audio code. The purpose of this beacon is to provide height, distance and equipment functioning checks to aircraft on intermediate and final approach. In the United States, an NDB is often combined with the outer marker beacon in the ILS approach (called a Locator Outer Marker, or LOM); in Canada, low-powered NDBs have replaced marker beacons entirely.
Middle marker

The middle marker should be located so as to indicate, in low visibility conditions, the missed approach point, and the point that visual contact with the runway is imminent, ideally at a distance of approximately 3,500 ft (1,100 m) from the threshold. It is modulated with a 1.3 kHz tone as alternating Morse-style dots and dashes at the rate of two per second. The cockpit indicator is an amber lamp that flashes in unison with the received audio code. Middle markers are no longer required in the United States so many of them are being decommissioned.[citation needed]
Inner marker

The inner marker, when installed, shall be located so as to indicate in low visibility conditions the imminence of arrival at the runway threshold. This is typically the position of an aircraft on the ILS as it reaches Category II minima. Ideally at a distance of approximately 1,000 ft (300 m) from the threshold. The modulation is Morse-style dots at 3 kHz. The cockpit indicator is a white lamp that flashes in unison with the received audio code.
DME
Distance measuring equipment (DME) provides pilots with a slant range measurement of distance to the runway in nautical miles. DMEs are augmenting or replacing markers in many installations. The DME provides more accurate and continuous monitoring of correct progress on the ILS glideslope to the pilot, and does not require an installation outside the airport boundary. When used in conjunction with an ILS, the DME is often sited midway between the reciprocal runway thresholds with the internal delay modified so that one unit can provide distance information to either runway threshold. On approaches where a DME is specified in lieu of marker beacons, the aircraft must have at least one operating DME unit to begin the approach, and a DME Required restriction will be noted on the Instrument Approach Procedure.
Monitoring
It is essential that any failure of the ILS to provide safe guidance be detected immediately by the pilot. To achieve this, monitors continually assess the vital characteristics of the transmissions. If any significant deviation beyond strict limits is detected, either the ILS is automatically switched off or the navigation and identification components are removed from the carrier.[2] Either of these actions will activate an indication ('failure flag') on the instruments of an aircraft using the ILS.
Approach lighting
Some installations include medium- or high-intensity approach light systems. Most often, these are at larger airports but many small general aviation airports in the U.S. have approach lights to support their ILS installations and obtain low-visibility minimums. The approach lighting system (abbreviated ALS) assists the pilot in transitioning from instrument to visual flight, and to align the aircraft visually with the runway centerline. Pilot observation of the approach lighting system at the Decision Altitude allows the pilot to continue descending towards the runway, even if the runway or runway lights cannot be seen, since the ALS counts as runway end environment. In the U.S., an ILS without approach lights may have CAT I ILS visibility minimums as low as 3/4 mile (runway visual range of 4,000 feet) if the required obstacle clearance surfaces are clear of obstructions. Visibility minimums of 1/2 mile (runway visual range of 2,400 feet) are possible with a CAT I ILS approach supported by a 1,400-to-3,000-foot-long (430 to 910 m) ALS, and 3/8 mile visibility 1,800-foot (550 m) visual range is possible if the runway has high-intensity edge lights, touchdown zone and centerline lights, and an ALS that is at least 2,400 feet long (see Table 3-5a in FAA Order 8260.3b). In effect, ALS extends the runway environment out towards the landing aircraft and allows low-visibility operations. CAT II and III ILS approaches generally require complex high-intensity approach light systems, while medium-intensity systems are usually paired with CAT I ILS approaches. At many non-towered airports, the intensity of the lighting system can be adjusted by the pilot, for example the pilot can click their microphone 7 times to turn on the lights, then 5 times to turn them to medium intensity.
Use

At a controlled airport, air traffic control will direct aircraft to the localizer via assigned headings, making sure aircraft do not get too close to each other (maintain separation), but also avoiding delay as much as possible.. Several aircraft can be on the ILS at the same time, several miles apart. An aircraft that has come within two and a half degrees of the localizer course (half scale deflection shown by the course deviation indicator) is said to be established on the approach. Typically, an aircraft will be established by at least two miles prior to the final approach fix (glideslope intercept at the specified altitude).
Aircraft deviation from the optimal path is indicated to the flight crew by means of display dial (a carry over from when an analog meter movement would indicate deviation from the course line via voltages sent from the ILS receiver).
The output from the ILS receiver goes both to the display system (head-down display and head-up display, if installed) and can also go to the Flight Control Computer. An aircraft landing procedure can be either coupled, where the Flight Control Computer directly flies the aircraft and the flight crew monitor the operation; or uncoupled (manual) where the flight crew fly the aircraft uses the HUD and manually control the aircraft to minimize the deviation from flight path to the runway centreline.
Rate-of-descent formula
A useful formula pilots use to calculate descent rates (standard 3° glide slope):
- Rate of descent = ground speed ⁄ 2 × 10
or
- Rate of descent = ground speed × 5
For other glideslope angles:
- Rate of descent = glide slope angle × ground speed × 100 / 60
The latter replaces tan α (see below) with α/60, which is about 95% accurate up to 10°.
Example:
120 kts × 5 or 120 kts / 2 × 10
= 600 fpm
The above simplified formulas are based on a trigonometric calculation:
- Rate of descent = ground speed × 101.25 × tan α
where:
- α is the descent or glideslope angle from the horizontal (3° being the standard)
- 101.25 (fpm⁄kt) is the conversion factor from knots to feet per minute (1 knot ≡ 1 nm⁄h = 6075 ft⁄h = 101.25 fpm)
Example:
Ground speed = 250 kts α = 4.5
250 kts × 101.25fpm/kt × tan 4.5 = 1992 fpm
Decision altitude/height
Once established on an approach, the autoland system or pilot will follow the ILS and descend along the determined altitude and position. From there the pilot will either try the same approach again, try a different approach or divert to another airport.
Aborting the approach (as well as the ATC instruction to do so) is called executing a missed approach.
ILS categories
There are three categories of ILS which support similarly named categories of operation. Information below is based on ICAO; certain states may have filed differences.
- Category I (CAT I) – A precision instrument approach and landing with a decision height not lower than Template:Ft to m above touchdown zone elevation and with either a visibility not less than Template:M to ft or a runway visual range not less than Template:M to ft.
- Category II (CAT II) – A precision instrument approach and landing with a decision height lower than Template:Ft to m above touchdown zone elevation but not lower than Template:Ft to m, and a runway visual range not less than Template:M to ft for aircraft category A, B, C and not less than Template:M to ft for aircraft category D.
- Category III (CAT III) is subdivided into three sections:
- Category III A – A precision instrument approach and landing with:
- a) a decision height lower than Template:Ft to m above touchdown zone elevation, or no decision height (alert height); and
- b) a runway visual range not less than Template:M to ft.
- Category III B – A precision instrument approach and landing with:
- a) a decision height lower than Template:Ft to m above touchdown zone elevation, or no decision height (alert height); and
- b) a runway visual range less than Template:M to ft but not less than Template:M to ft. Autopilot is used until taxi-speed. In the United States, FAA criteria for CAT III B runway visual range allows readings as low as 150 ft.
- Category III C – A precision instrument approach and landing with no decision height and no runway visual range limitations. This category is not yet in operation anywhere in the world, as it requires guidance to taxi in zero visibility as well. "Category III C" is not mentioned in EU-OPS. Category III B is currently the best available system.
- Category III A – A precision instrument approach and landing with:
FAA Order 8400.13D allows for special authorization of CAT I ILS approaches to a decision height of Template:Ft to m above touchdown, and a runway visual range as low as Template:Ft to m. The aircraft and crew must be approved for CAT II operations, and a heads-up display in CAT II or III mode must be used to the decision height. CAT II/III missed approach criteria applies.
In the United States, many but not all airports with CAT III approaches have listings for CAT IIIa, IIIb and IIIc on the instrument approach plate (U.S. Terminal Procedures). CAT IIIb runway visual range minimums are limited by the runway/taxiway lighting and support facilities, and would be consistent with the airport Surface Movement Guidance Control System (SMGCS) plan. Operations below 600 runway visual range require taxiway centerline lights and taxiway red stop bar lights. If the CAT IIIb runway visual range minimums on a runway end were 600 feet, which is a common figure in the U.S., ILS approaches to that runway end with runway visual range below 600 feet would qualify as CAT IIIc and require special taxi procedures, lighting and approval conditions to permit the landings. FAA Order 8400.13D limits CAT III to 300 runway visual range or better. Order 8400.13D, which was released during 2009, also allows special authorization CAT II approaches to runways without ALSF-2 approach lights and/or touchdown zone/centerline lights, which has expanded the number of potential CAT II runways.
In each case, a suitably equipped aircraft and appropriately qualified crew are required. For example, CAT IIIb requires a fail-operational system, along with a crew who are qualified and current, while CAT I does not. A head-up display which allows the pilot to perform aircraft maneuvers rather than an automatic system is considered as fail-operational. CAT I relies only on altimeter indications for decision height, whereas CAT II and CAT III approaches use radar altimeter to determine decision height.[3]
An ILS is required to shut down upon internal detection of a fault condition. With the increasing categories, ILS equipment is required to shut down faster, since higher categories require shorter response times. For example, a CAT I localizer must shutdown within 10 seconds of detecting a fault, but a CAT III localizer must shut down in less than 2 seconds.[2]
Limitations and alternatives
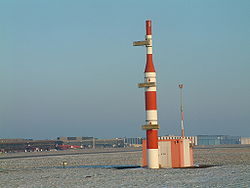
Due to the complexity of ILS localizer and glideslope systems, there are some limitations. Localizer systems are sensitive to obstructions in the signal broadcast area like large buildings or hangars. Glideslope systems are also limited by the terrain in front of the glideslope antennas. If terrain is sloping or uneven, reflections can create an uneven glidepath causing unwanted needle deflections. Additionally, since the ILS signals are pointed in one direction by the positioning of the arrays, ILS only supports straight-in approaches. A modified ILS called an Instrument Guidance System (IGS) is also occasionally used, the most famous example being that which was in use at one of the runways of Kai Tak Airport, Hong Kong to accommodate a non-straight approach;[4][5] IGSes are also called Localizer Type Directional Aids in the US. Installation of ILS can also be costly due to the complexity of the antenna system and siting criteria. To avoid hazardous reflections that would affect the radiated signal, ILS critical areas and ILS sensitive areas are established. Positioning of these critical areas can prevent aircraft from using certain taxiways.[6] This can cause additional delays in take offs due to increased hold times and increased spacing between aircraft.
In the 1980s, there was a major US & European effort to establish the Microwave Landing System (MLS), which is not similarly limited and which allows curved approaches. However, a combination of airline reluctance to invest in MLS, and the rise of Global Positioning System (GPS) has resulted in its failure to be adopted in civil aviation. The Transponder Landing System (TLS) is another alternative to an ILS that can be used where a conventional ILS will not work or is not cost-effective.
Localizer Performance with Vertical guidance (LPV) is the latest alternative to the ILS. Based on the Wide Area Augmentation System (WAAS), LPV has similar minima to ILS for appropriately equipped aircraft. As of November 2008[update], the FAA has published more LPV approaches than Category I ILS procedures.
Another potential alternative to ILS is the Ground-Based Augmentation System (GBAS), a safety-critical system that augments the GPS Standard Positioning Service (SPS) and provides enhanced levels of service. It supports all phases of approach, landing, departure, and surface operations within the VHF coverage volume. (Local Area Augmentation System is the United States' implementation of GBAS). GBAS is expected to play a key role in modernization and in all-weather operations capability at CATI/II and III airports, terminal area navigation, missed approach guidance and surface operations. GBAS provides the capability to service the entire airport with a single frequency (VHF transmission) whereas ILS requires a separate frequency for each runway end. GBAS CAT-I is seen as a necessary step towards the more stringent operations of CAT-II/III precision approach and landing. Until recently, the technical risk of implementing GBAS prevented wide spread acceptance of the technology. The FAA, along with industry, have fielded Provably Safe Prototype GBAS stations which mitigate the impact of satellite signal deformation, ionosphere differential error, ephemeris error and multipath.
History
Tests of the ILS system began in 1929,[7] and the Civil Aeronautics Administration (CAA) authorized installation of the system in 1941 at six locations. The first landing of a scheduled U.S. passenger airliner using ILS was on January 26, 1938, as a Pennsylvania Central Airlines Boeing 247-D flew from Washington, D.C., to Pittsburgh and landed in a snowstorm using only the Instrument Landing System.[8] The first fully automatic landing using ILS occurred at Bedford Airport UK in March 1964.[9]
Future
The Microwave Landing System (MLS) introduced in the 1970s[10] was intended to replace ILS but fell out of favour in the United States because of satellite based systems. However, it is showing a resurgence in the United Kingdom for civil aviation.[11] ILS and MLS are the only standardized systems in Civil Aviation that meet requirements for Category III automated landings.[12] The first Category III MLS for civil aviation was commissioned at Heathrow airport in March 2009.[13]
The advent of the Global Positioning System (GPS) provides an alternative source of approach for aircraft. In the US, the Wide Area Augmentation System (WAAS) has been available to provide precision guidance to Category I standards since 2007, and the equivalent in Europe, the European Geostationary Navigation Overlay Service (EGNOS), is currently undergoing final trials and will be certified for safety of life applications in 2010. Other methods of augmentation are in development to provide for Category III minimums or better, such as the Local Area Augmentation System (LAAS).
The FAA Ground-Based Augmentation System (GBAS) office is currently working with the industry in anticipation of the certification of the first GBAS ground stations in Memphis, TN; Sydney, Australia; Bremen, Germany; Spain and Newark, NJ. All four countries have installed GBAS systems and are involved in technical and operational evaluation activities. The Honeywell and FAA team are working on the System Design Approval of the world’s first Non-Federal U.S. approval for LAAS Category I operations; expected in first quarter 2009 and compliant with International Civil Aviation Organization (ICAO) Standards and Recommended Practices (SARPs) Category I LAAS.
Frequency list
Localizer and glideslope carrier frequencies are paired so that only one selection is required to tune both receivers.[14][15]
Channel | LOC (MHz) | G/S (MHz) |
---|---|---|
18X | 108.10 | 334.70 |
18Y | 108.15 | 334.55 |
20X | 108.30 | 334.10 |
20Y | 108.35 | 333.95 |
22X | 108.50 | 329.90 |
22Y | 108.55 | 329.75 |
24X | 108.70 | 330.50 |
24Y | 108.75 | 330.35 |
26X | 108.90 | 329.30 |
26Y | 108.95 | 329.15 |
Channel | LOC (MHz) | G/S (MHz) |
---|---|---|
28X | 109.10 | 331.40 |
28Y | 109.15 | 331.25 |
30X | 109.30 | 332.00 |
30Y | 109.35 | 331.85 |
32X | 109.50 | 332.60 |
32Y | 109.55 | 332.45 |
34X | 109.70 | 333.20 |
34Y | 109.75 | 333.05 |
36X | 109.90 | 333.80 |
36Y | 109.95 | 333.65 |
Channel | LOC (MHz) | G/S (MHz) |
---|---|---|
38X | 110.10 | 334.40 |
38Y | 110.15 | 334.25 |
40X | 110.30 | 335.00 |
40Y | 110.35 | 334.85 |
42X | 110.50 | 329.60 |
42Y | 110.55 | 329.45 |
44X | 110.70 | 330.20 |
44Y | 110.75 | 330.05 |
46X | 110.90 | 330.80 |
46Y | 110.95 | 330.65 |
Channel | LOC (MHz) | G/S (MHz) |
---|---|---|
48X | 111.10 | 331.70 |
48Y | 111.15 | 331.55 |
50X | 111.30 | 332.30 |
50Y | 111.35 | 332.15 |
52X | 111.50 | 332.90 |
52Y | 111.55 | 332.75 |
54X | 111.70 | 333.50 |
54Y | 111.75 | 333.35 |
56X | 111.90 | 331.10 |
56Y | 111.95 | 330.95 |
See also
- Acronyms and abbreviations in avionics
- AN/CRN-2
- Blind approach beacon system (BABS)
- CFIT
- Distance measuring equipment (DME)
- EGPWS
- Flight director, FD
- Fog
- Global Positioning System (GPS)
- HUD
- Instrument flight rules (IFR)
- Localizer performance with vertical guidance (LPV)
- Local Area Augmentation System (LAAS)
- Lorenz beam
- Microwave landing system (MLS)
- Non-directional beacon (NDB)
- Space modulation
- Transponder landing system (TLS)
- Visual flight rules (VFR)
- VHF omnidirectional range (VOR)
- Wide Area Augmentation System (WAAS)
Notes
- ^ "ICAO DOC8400 Amendment 28". icao.int.
- ^ a b Department of Transportation and Department of Defense (March 25, 2002). "2001 Federal Radionavigation Systems" (PDF). Retrieved November 27, 2005.
- ^ ICAO Annex 10 Aeronautical Telecommunnications Volume 1 Radia Navigations aids 2.1.1
- ^ "Approach chart of Kai Tak Airport runway 13". flyingtigersgroup.org.
- ^ Kai Tak Airport#Runway 13 approach
- ^ FAA, ILS Glide Slope Critical Area Advisory: Pg 4, ILS Course Distortion
- ^ "Planes Are Landing By Radio When Fog Hides The Field", February 1931, Popular Mechanics bottom-right of page
- ^ Roger Mola. "History of Aircraft Landing Aids". centennialofflight.gov. Retrieved 28 September 2010.
- ^ Autoland
- ^ Microwave Landing System For Jets Is Demonstrated. New York Times. May 20, 1976.
- ^ "MLS: Back to the Future?". April 1, 2003.
- ^ "Annex 10 – Aeronautical Telecommunications, Volume I (Radio Navigation Aids) Amendment 81" (PDF).
- ^ NATS (March 26, 2009). "Worlds first low-visibility microwave landing system comes into operation at Heathrow". atc-network.com.
- ^ "Frequency allotments" (PDF). ntia.doc.gov. 1/2008 (Rev. 9/2009). Retrieved 28 September 2010.
{{cite web}}
: Check date values in:|date=
(help) - ^ MIC. "ページ・アクセス・エラー" (PDF). tele.soumu.go.jp. Retrieved 28 September 2010. [dead link ] Template:Ja
References
- ICAO Annex 10 Volume 1, Radio Navigation Aids, Fifth Edition — July 1996
- Aeronautical Information Manual, FAA – February 11, 2010
- Digital Terminal Procedures, FAA – May, 2010
External links

- History of Aircraft Landing Aids--U.S. Centennial of Flight Commission
- "Happy Landings In Fog", June 1933, Popular Mechanics article on the early system setup in the USA.
- ILS Basics
- ILS Tutorial Animations
- List of all ILS approaches in the US