Ribosome: Difference between revisions
m rvt |
|||
Line 27: | Line 27: | ||
The differences between the [[prokaryote|prokaryotic]] and [[eukaryote|eukaryotic]] ribosomes are exploited by humans since the 70 S (7 ps) ribosomes are vulnerable to some [[antibiotic]]s that the 80S ribosomes are not. This helps create drugs that can destroy a bacterial infection without harming the animal/human host's cells. Even though human [[mitochondria]] possess 55 S (5.5 ps) ribosomes with rRNA compounds similar to bacterial ribosomes, mitochondria are rarely affected by these [[antibiotic]]s because mitochondria are covered by a double membrane that does not easily admit these antibiotics into the organelle. |
The differences between the [[prokaryote|prokaryotic]] and [[eukaryote|eukaryotic]] ribosomes are exploited by humans since the 70 S (7 ps) ribosomes are vulnerable to some [[antibiotic]]s that the 80S ribosomes are not. This helps create drugs that can destroy a bacterial infection without harming the animal/human host's cells. Even though human [[mitochondria]] possess 55 S (5.5 ps) ribosomes with rRNA compounds similar to bacterial ribosomes, mitochondria are rarely affected by these [[antibiotic]]s because mitochondria are covered by a double membrane that does not easily admit these antibiotics into the organelle. |
||
Ribosomes are the workhorses of [[protein |
Ribosomes are the workhorses of [[protein biosynthesis]], the process of translating [[messenger RNA]] (mRNA) into [[protein]]. The mRNA comprises a series of [[codon]]s that dictate to the ribosome the [[amino acid]]s needed to make the protein. Using the mRNA as a template, the ribosome traverses each codon of the mRNA, pairing it with the appropriate amino acid. This is done using molecules of [[transfer RNA]] (tRNA) containing a complementary [[anticodon]] on one end and the appropriate amino acid on the other. |
||
Protein synthesis begins at a [[start codon]] near the 5' end of the mRNA. The small ribosomal subunit, typically bound to a tRNA containing the amino acid [[methionine]], binds to an AUG codon on the mRNA and recruits the large ribosomal subunit. The large ribosomal subunit contains three tRNA binding sites, designated A, P, and E. The A site binds an aminoacyl-tRNA (a tRNA bound to an amino acid); the P site binds a peptidyl-tRNA (a tRNA bound to the peptide being synthesized); and the E site binds a free tRNA before it exits the ribosome. |
Protein synthesis begins at a [[start codon]] near the 5' end of the mRNA. The small ribosomal subunit, typically bound to a tRNA containing the amino acid [[methionine]], binds to an AUG codon on the mRNA and recruits the large ribosomal subunit. The large ribosomal subunit contains three tRNA binding sites, designated A, P, and E. The A site binds an aminoacyl-tRNA (a tRNA bound to an amino acid); the P site binds a peptidyl-tRNA (a tRNA bound to the peptide being synthesized); and the E site binds a free tRNA before it exits the ribosome. |
Revision as of 01:47, 15 November 2006
(1) Head. (2) Platform. (3) Base. (4) Ridge. (5) Central protuberance. (6) Back. (7) Stalk. (8) Front.
A ribosome is an organelle in cells that assembles proteins. Ribosomes are composed of ribosomal RNA and ribosomal proteins (known as a Ribonucleoprotein or RNP). It translates messenger RNA (mRNA) into a polypeptide chain (e.g., a protein). It can be thought of as a factory that builds a protein from a set of genetic instructions. Ribosomes can float freely in the cytoplasm (the internal fluid of the cell) or bind to the endoplasmic reticulum, or to the nuclear envelope. Since ribosomes are ribozymes, it is thought that they might be remnants of the RNA world.
Ribosomes were first observed in the mid-1950s by cell biologist George Palade in the electron microscope as dense particles or granules[1] for which he would win the Nobel Prize. The term ribosome was proposed by scientist Richard B. Roberts in 1958:
During the course of the symposium a semantic difficulty became apparent. To some of the participants, microsomes mean the ribonucleoprotein particles of the microsome fraction contaminated by other protein and lipid material; to others, the microsomes consist of protein and lipid contaminated by particles. The phrase “microsomal particles” does not seem adequate, and “ribonucleoprotein particles of the microsome fraction” is much too awkward. During the meeting the word “ribosome” was suggested; this seems a very satisfactory name, and it has a pleasant sound. The present confusion would be eliminated if “ribosome” were adopted to designate ribonucleoprotein particles in the size range 20 to 100S.
— Roberts, R. B., Microsomal Particles and Protein Synthesis[2]
The structure and function of the ribosomes and associated molecules, known as the translational apparatus, has been of research interest since the mid 20th century and is a very active field of study today.
Overview
Ribosomes consist of two subunits (Figure 1) that fit together (Figure 2) and work as one to translate the mRNA into a polypeptide chain during protein synthesis (Figure 3). Prokaryotic subunits consist of one or two and eukaryotic of one or three very large RNA molecules (known as ribosomal RNA or rRNA) and multiple smaller protein molecules. Crystallographic work has shown that there are no ribosomal proteins close to the reaction site for polypeptide synthesis. This suggests that the protein components of ribosomes act as a scaffold that may enhance the ability of rRNA to synthesise protein rather than directly participating in catalysis. (See: Ribozyme)

Ribosome locations
Free ribosomes
Free ribosome's are "free" to move about (within the cell membrane)anywhere in the cytoplasm. Proteins made by free ribosomes are used within the cell.
Membrane-bound ribosomes
When certain proteins are synthesized by a ribosome they can become "membrane-bound". The newly produced polypeptide chains are inserted directly into the endoplasmic reticulum by the ribosome and are then transported to their destinations. Bound ribosomes usually produce proteins that are used within the cell membrane or are expelled from the cell via exocytosis.
Free and membrane-bound ribosomes differ only in their spatial distribution; they are identical in structure and function. Whether the ribosome exists in a free or membrane-bound state depends on the presence of a ER-targeting signal sequence on the protein being synthesized.
Structure and function
The ribosomal subunits of prokaryotes and eukaryotes are quite similar. However, prokaryotes have 70 S (7 ps) ribosomes, each consisting of a (small) 30 S (3 ps) and a (large) 50 S (5 ps) subunit, whereas eukaryotes have 80 S (8 ps) ribosomes, each consisting of a (small) 40 S (4 ps) and a bound (large) 60 S (6 ps) subunit. However, the ribosomes found in chloroplasts and mitochondria of eukaryotes are 55S. The unit "S" means the svedberg, a measure of the rate of sedimentation of a particle in a centrifuge, where the sedimentation rate is associated with the size of the particle. It is important to note that Svedbergsunits are not addable—two subunits together can have Svedberg values that do not add up to that of the entire ribosome. This is resulting from the loss of surface area when the two subunits are bound. In addition, the ungainly shape of the fully assembled ribosome has different aqua dynamic properties from the two unbound subunits. The differences between the prokaryotic and eukaryotic ribosomes are exploited by humans since the 70 S (7 ps) ribosomes are vulnerable to some antibiotics that the 80S ribosomes are not. This helps create drugs that can destroy a bacterial infection without harming the animal/human host's cells. Even though human mitochondria possess 55 S (5.5 ps) ribosomes with rRNA compounds similar to bacterial ribosomes, mitochondria are rarely affected by these antibiotics because mitochondria are covered by a double membrane that does not easily admit these antibiotics into the organelle.
Ribosomes are the workhorses of protein biosynthesis, the process of translating messenger RNA (mRNA) into protein. The mRNA comprises a series of codons that dictate to the ribosome the amino acids needed to make the protein. Using the mRNA as a template, the ribosome traverses each codon of the mRNA, pairing it with the appropriate amino acid. This is done using molecules of transfer RNA (tRNA) containing a complementary anticodon on one end and the appropriate amino acid on the other.
Protein synthesis begins at a start codon near the 5' end of the mRNA. The small ribosomal subunit, typically bound to a tRNA containing the amino acid methionine, binds to an AUG codon on the mRNA and recruits the large ribosomal subunit. The large ribosomal subunit contains three tRNA binding sites, designated A, P, and E. The A site binds an aminoacyl-tRNA (a tRNA bound to an amino acid); the P site binds a peptidyl-tRNA (a tRNA bound to the peptide being synthesized); and the E site binds a free tRNA before it exits the ribosome.

In Figure 3, both ribosomal subunits (small and large) assemble at the start codon (towards the 5' end of the mRNA). The ribosome uses tRNA which matches the current codon (triplet) on the mRNA to append an amino acid to the polypeptide chain. This is done for each triplet on the mRNA, while the ribosome moves towards the 3' end of the mRNA. Usually in bacterial cells, several ribosomes are working parallel on a single mRNA, forming what we call a polyribosome or polysome.
Atomic structure
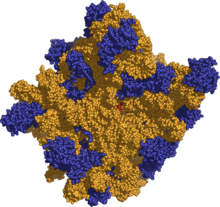
The general molecular structure of the ribosome has been known for several decades, but recently its structure has been achieved at novel resolutions, in the order of a few angstroms.
The atomic structure of the 50 S (5 ps) large subunit ribosome from the archeal, Haloarcula marismortui was published in Science on August 11, 2000 by N. Ban, et al.[3]
Soon after the structure of the 30 S (3 ps) from Thermus thermophilus was published in Cell on September 1, 2000, by F. Schluenzen et. al..[4] Shortly after a more detailed structure was published in Nature on September 21, 2000 by B. T. Wimberly, et al..[5]
Using these coordinates, M. M. Yusupov, et al.[6] were able to reconstruct the entire Thermus thermophilus 70 S particle at low resolution, which was published in Science on May 4 2001.
More recently the structure of the E. coli 70 S ribosome was determined at 3.5 angstroms (0.35 nanometer) by Schuwirth et al. (Science, 2005)[7]. Also an EM structure was recently published by Mitra et al. (Nature, 2005)[8] which depicts a ribosome at 11-15 angstroms (1.1–1.5 nm) in the act of passing a newly synthesized protein strand into a translocation channel.
References
- ^ G.E. Palade. (1955) "A small particulate component of the cytoplasm." J Biophys Biochem Cytol. Jan;1(1): pages 59-68. PMID 14381428
- ^ Roberts, R. B., editor. (1958) "Introduction" in Microsomal Particles and Protein Synthesis. New York: Pergamon Press, Inc.
- ^ Ban N, Nissen P, Hansen J, Moore PB, Steitz TA. The complete atomic structure of the large ribosomal subunit at 2.4 Å resolution. Science. 2000 Aug 11;289(5481):905-20.. PMID 10937989
- ^ Schluenzen F, Tocilj A, Zarivach R, Harms J, Gluehmann M, Janell D, Bashan A, Bartels H, Agmon I, Franceschi F, Yonath A. Structure of functionally activated small ribosomal subunit at 3.3 angstroms resolution. Cell. 2000 Sep 1;102(5):615-23. PMID 11007480
- ^ Wimberly BT, Brodersen DE, Clemons WM Jr, Morgan-Warren RJ, Carter AP, Vonrhein C, Hartsch T, Ramakrishnan V. Structure of the 30S ribosomal subunit. Nature. 2000 Sep 21;407(6802):327-39. PMID 11014182
- ^ Yusupov MM, Yusupova GZ, Baucom A, Lieberman K, Earnest TN, Cate JH, Noller HF. Crystal structure of the ribosome at 5.5 Å resolution. Science. 2001 May 4;292(5518):883-96. Epub 2001 Mar 29. PMID 11283358
- ^ Schuwirth BS, Borovinskaya MA, Hau CW, Zhang W, Vila-Sanjurjo A, Holton JM, Cate JH. Structures of the bacterial ribosome at 3.5 A resolution. Science. 2005 Nov 4;310(5749):827-34. PMID 16272117
- ^ Mitra K, Schaffitzel C, Shaikh T, Tama F, Jenni S, Brooks CL 3rd, Ban N, Frank J. Structure of the E. coli protein-conducting channel bound to a translating ribosome. Nature. 2005 Nov 17;438(7066):318-24. PMID 16292303
See also
|
|
External links
- 70S Ribosome Architecture Animation of a working ribosome. Requires the Chime browser plugin from this site (where registration is required).
- Lab computer simulates ribosome in motion
- Information on ribosomes
This article incorporates public domain material from Science Primer. NCBI. Archived from the original on 2009-12-08.