Seaweed fertiliser: Difference between revisions
No edit summary |
No edit summary |
||
Line 13: | Line 13: | ||
Seaweed fertilizer spread inland when a [[kelp]] industry developed in Scotland, Norway, and Brittany in the [[18th century|18th]] and [[19th century]].<ref>{{cite journal |last1=Gray |first1=Malcolm |title=The Kelp Industry in the High-Lands and Islands |journal=The Economic History Review |date=1951 |volume=4 |issue=2 |pages=197–209 |doi=10.2307/2599122 |jstor=2599122 }}</ref><ref name="Mooney 2021"/> The industry developed out of demand for ashed soda, or potash, which was used to create glass and soap, and led to shortages for agricultural applications in traditional coastal communities. Potash is a water-soluble [[potassium]] rich concentrate made from plant matter, so it was also exported as a fertilizer.<ref name="Mooney 2021"/> Coastal communities in the seaweed industry both expanded and struggled to keep up with the demand.<ref name="Kenicer Bridgewater Milliken 2000"/> Early commercial kelp export in Scotland devastated traditional agriculture in the region because intensive labor was needed during the seaweed growing season to harvest and process the kelp, which led to a labor transition from farming to kelp processing. Additionally, exploitation of kelp resources for potash production left little kelp behind for local fertilizer and coastal land became more desirable than inland regions.<ref name="Mooney 2021"/><ref name="Kenicer Bridgewater Milliken 2000"/> The Scottish seaweed industry went through multiple boom and bust cycles, employing 10,000 families and producing 3,000 tonnes of ash per year during its peak.<ref name=":52">{{Cite web|title=Journal {{!}} The history of Scotland's kelp industry|url=https://www.snapdragonlife.com/news/blog/the-history-of-scotlands-kelp-industry/|access-date=2021-11-29|website=Snapdragon Life}}</ref><ref>{{Cite web|last=Archaeology|first=Current|date=2020-11-05|title=Sifting through the remains of Scotland’s kelping industry {{!}} The Past|url=https://the-past.com/feature/sifting-through-the-remains-of-scotlands-kelping-industry/|access-date=2021-11-29|website=the-past.com|language=en-GB}}</ref><ref name="Kenicer Bridgewater Milliken 2000"/><ref name="Mooney 2021"/> The export price of kelp ash dropped in 1822, leading to a sudden emigration from the area because the crop was no longer profitable enough to support such a large industry. Kelp exploitation and toxic ash processing caused ecological and economic damage in Orkney and left many people sick and blinded.<ref name="Kenicer Bridgewater Milliken 2000"/><ref name=":52" /> The kelp industry picked up again for [[iodine]] production in 1845, and [[alginate]] (a thickening agent) production in the early 1900s, which reinvigorated kelp harvest.<ref name="Kenicer Bridgewater Milliken 2000"/> |
Seaweed fertilizer spread inland when a [[kelp]] industry developed in Scotland, Norway, and Brittany in the [[18th century|18th]] and [[19th century]].<ref>{{cite journal |last1=Gray |first1=Malcolm |title=The Kelp Industry in the High-Lands and Islands |journal=The Economic History Review |date=1951 |volume=4 |issue=2 |pages=197–209 |doi=10.2307/2599122 |jstor=2599122 }}</ref><ref name="Mooney 2021"/> The industry developed out of demand for ashed soda, or potash, which was used to create glass and soap, and led to shortages for agricultural applications in traditional coastal communities. Potash is a water-soluble [[potassium]] rich concentrate made from plant matter, so it was also exported as a fertilizer.<ref name="Mooney 2021"/> Coastal communities in the seaweed industry both expanded and struggled to keep up with the demand.<ref name="Kenicer Bridgewater Milliken 2000"/> Early commercial kelp export in Scotland devastated traditional agriculture in the region because intensive labor was needed during the seaweed growing season to harvest and process the kelp, which led to a labor transition from farming to kelp processing. Additionally, exploitation of kelp resources for potash production left little kelp behind for local fertilizer and coastal land became more desirable than inland regions.<ref name="Mooney 2021"/><ref name="Kenicer Bridgewater Milliken 2000"/> The Scottish seaweed industry went through multiple boom and bust cycles, employing 10,000 families and producing 3,000 tonnes of ash per year during its peak.<ref name=":52">{{Cite web|title=Journal {{!}} The history of Scotland's kelp industry|url=https://www.snapdragonlife.com/news/blog/the-history-of-scotlands-kelp-industry/|access-date=2021-11-29|website=Snapdragon Life}}</ref><ref>{{Cite web|last=Archaeology|first=Current|date=2020-11-05|title=Sifting through the remains of Scotland’s kelping industry {{!}} The Past|url=https://the-past.com/feature/sifting-through-the-remains-of-scotlands-kelping-industry/|access-date=2021-11-29|website=the-past.com|language=en-GB}}</ref><ref name="Kenicer Bridgewater Milliken 2000"/><ref name="Mooney 2021"/> The export price of kelp ash dropped in 1822, leading to a sudden emigration from the area because the crop was no longer profitable enough to support such a large industry. Kelp exploitation and toxic ash processing caused ecological and economic damage in Orkney and left many people sick and blinded.<ref name="Kenicer Bridgewater Milliken 2000"/><ref name=":52" /> The kelp industry picked up again for [[iodine]] production in 1845, and [[alginate]] (a thickening agent) production in the early 1900s, which reinvigorated kelp harvest.<ref name="Kenicer Bridgewater Milliken 2000"/> |
||
Global production of seaweed fertilizer largely phased out when [[Fertilizer|chemical fertilizers]] were developed in the 1920s, due to the cheaper production cost.<ref name=" |
Global production of seaweed fertilizer largely phased out when [[Fertilizer|chemical fertilizers]] were developed in the 1920s, due to the cheaper production cost.<ref name="Arzel 1984 Historical Background">{{cite book |last1=Arzel |first1=P |chapter=Historical Background |chapter-url=https://www.fao.org/3/x5820e/x5820e03.htm |title=Traditional management of seaweeds in the district of Léon |date=1984 |publisher=Food and Agriculture Organization of the United Nations |isbn=92-5-102144-9 |oclc=995583539 |url=https://www.fao.org/3/X5820E/X5820E00.htm }}</ref><ref name="Kenicer Bridgewater Milliken 2000"/><ref name="Craigie 2011">{{cite journal |last1=Craigie |first1=James S. |title=Seaweed extract stimuli in plant science and agriculture |journal=Journal of Applied Phycology |date=June 2011 |volume=23 |issue=3 |pages=371–393 |doi=10.1007/s10811-010-9560-4 }}</ref> Chemical fertilizers revolutionized the agriculture industry and allowed the human population to grow far beyond the limits of traditional food production methods.<ref>{{Cite web|title=How many people does synthetic fertilizer feed?|url=https://ourworldindata.org/how-many-people-does-synthetic-fertilizer-feed|access-date=2021-11-29|website=Our World in Data}}</ref><ref>{{Cite web|date=2014-11-19|title=Fertilizer History: The Haber-Bosch Process|url=https://www.tfi.org/the-feed/fertilizer-history-haber-bosch-process|access-date=2021-11-29|website=www.tfi.org|language=en}}</ref> Synthetic fertilizers are still the predominant global source for commercial agricultural applications due to the cheap cost of production and widespread access. However, small scale organic farmers and coastal communities continued traditional seaweed techniques in regions with a rich seaweed history.<ref name="Kenicer Bridgewater Milliken 2000"/><ref name="Nedumaran & Arulbalachandran 2015"/> The first industrial kelp liquid fertilizer, [[Maxicrop]], was created by Reginald Milton in 1947.<ref name="Craigie 2011"/> The creation of liquid fertilizer has allowed for more widespread application of seaweed-derived fertilizer to inland regions and sparked a growing [[agronomic]] interest in seaweed for a variety of agricultural applications, including foliage spray, biostimulants, and soil conditioning.<ref name="Craigie 2011"/> Interestingly, the historic rise of seaweed [[aquaculture]] did not align with fertilizer production because the European countries that produce seaweed fertilizer haven't developed a significant aquaculture industry; [[seaweed farming]] is also currently dominated by China and Indonesia, where the crop is grown for food and other lucrative uses.<ref name="Buschmann Camus Infante et al 2017"/> |
||
== Aquaculture == |
== Aquaculture == |
||
Line 39: | Line 39: | ||
=== Coastal Eutrophication === |
=== Coastal Eutrophication === |
||
A growing population and intensification of industry and agriculture have increased the volume of wastewater discharged into coastal marine ecosystems.<ref name="Neveux Bolton Bruhn et al 2018">{{cite book |doi=10.1002/9783527801718.ch7 |chapter=The Bioremediation Potential of Seaweeds: Recycling Nitrogen, Phosphorus, and Other Waste Products |title=Blue Biotechnology |year=2018 |last1=Neveux |first1=Nicolas |last2=Bolton |first2=John J. |last3=Bruhn |first3=Annette |last4=Roberts |first4=David A. |last5=Ras |first5=Monique |pages=217–239 |isbn=978-3-527-80171-8 |s2cid=134905629 }}</ref><ref name=" |
A growing population and intensification of industry and agriculture have increased the volume of wastewater discharged into coastal marine ecosystems.<ref name="Neveux Bolton Bruhn et al 2018">{{cite book |doi=10.1002/9783527801718.ch7 |chapter=The Bioremediation Potential of Seaweeds: Recycling Nitrogen, Phosphorus, and Other Waste Products |title=Blue Biotechnology |year=2018 |last1=Neveux |first1=Nicolas |last2=Bolton |first2=John J. |last3=Bruhn |first3=Annette |last4=Roberts |first4=David A. |last5=Ras |first5=Monique |pages=217–239 |isbn=978-3-527-80171-8 |s2cid=134905629 }}</ref><ref name="Racine Marley Froehlich et al 2021">{{cite journal |last1=Racine |first1=Phoebe |last2=Marley |first2=AnnaClaire |last3=Froehlich |first3=Halley E. |last4=Gaines |first4=Steven D. |last5=Ladner |first5=Ian |last6=MacAdam-Somer |first6=Ilan |last7=Bradley |first7=Darcy |title=A case for seaweed aquaculture inclusion in U.S. nutrient pollution management |journal=Marine Policy |date=July 2021 |volume=129 |pages=104506 |doi=10.1016/j.marpol.2021.104506 }}</ref> These waters typically contain high concentrations of nitrogen and phosphorus, and relatively high heavy metal concentrations, leading to [[eutrophication]] of many coastal ecosystems.<ref name="Tanaka Ashaari Mohamad Lamit 2020"/><ref name="Kang Kim Choi et al 2021"/><ref name="Racine Marley Froehlich et al 2021"/><ref name="Chekroun Sánchez Baghour 2014">{{cite journal |last1=Chekroun |first1=Kaoutar Ben |last2=Sánchez |first2=Esteban |last3=Baghour |first3=Mourad |title=The role of algae in bioremediation of organic pollutants |journal=International Research Journal of Public and Environmental Health |date=April 2014 |volume=1 |issue=2 |url=https://journalissues.org/irjpeh/abstract/chekroun-et-al/ |s2cid=17523101 }}</ref> Eutrophication results from the excessive nutrient load within these ecosystems resulting from the pollution of waters entering the oceans from industry, animal feed, and synthetic fertilizers, and thus over-fertilizes these systems.<ref name="Tanaka Ashaari Mohamad Lamit 2020"/><ref name="Kang Kim Choi et al 2021"/><ref name="Zeraatkar Ahmadzadeh Talebi et al 2016"/> Eutrophication leads to high productivity in coastal systems, which can result in coastal [[Hypoxia (environmental)|hypoxia]] and [[ocean acidification]], two major concerns for coastal ecosystems.<ref>{{Cite journal|last=Cai|first=Wei-Jun|last2=Hu|first2=Xinping|last3=Huang|first3=Wei-Jen|last4=Murrell|first4=Michael C.|last5=Lehrter|first5=John C.|last6=Lohrenz|first6=Steven E.|last7=Chou|first7=Wen-Chen|last8=Zhai|first8=Weidong|last9=Hollibaugh|first9=James T.|last10=Wang|first10=Yongchen|last11=Zhao|first11=Pingsan|date=November 2011|title=Acidification of subsurface coastal waters enhanced by eutrophication|url=https://www.nature.com/articles/ngeo1297|journal=Nature Geoscience|language=en|volume=4|issue=11|pages=766–770|doi=10.1038/ngeo1297|issn=1752-0908}}</ref><ref>{{Cite journal|last=Melzner|first=Frank|last2=Thomsen|first2=Jörn|last3=Koeve|first3=Wolfgang|last4=Oschlies|first4=Andreas|last5=Gutowska|first5=Magdalena A.|last6=Bange|first6=Hermann W.|last7=Hansen|first7=Hans Peter|last8=Körtzinger|first8=Arne|date=2013-08-01|title=Future ocean acidification will be amplified by hypoxia in coastal habitats|url=https://doi.org/10.1007/s00227-012-1954-1|journal=Marine Biology|language=en|volume=160|issue=8|pages=1875–1888|doi=10.1007/s00227-012-1954-1|issn=1432-1793}}</ref> A notable service of seaweed farming is its ability to act as a bio-remediator through uptake and removal of excessive nutrients in coastal ecosystems with their application to land uses.<ref name="Tanaka Ashaari Mohamad Lamit 2020"/><ref name="Chekroun Sánchez Baghour 2014"/> Brown algae, due in part to their large size, have been noted for their high productivity and corresponding high nutrient uptake in coastal ecosystems.<ref name="Zeraatkar Ahmadzadeh Talebi et al 2016"/><ref name="Young Dring Savidge et al 2007"/><ref name="Song Duc Pham Seon Chul Woo 2015">{{cite journal |last1=Song |first1=Minkyung |last2=Duc Pham |first2=Hong |last3=Seon |first3=Jiyun |last4=Chul Woo |first4=Hee |title=Marine brown algae: A conundrum answer for sustainable biofuels production |journal=Renewable and Sustainable Energy Reviews |date=October 2015 |volume=50 |pages=782–792 |doi=10.1016/j.rser.2015.05.021 }}</ref> Additionally, studies have focused on how brown algae growth can be optimized to increase biomass production and therefore increase the quantity of nutrients removed from these ecosystems.<ref name="Song Duc Pham Seon Chul Woo 2015"/> Studies have also explored the potential of brown algae to sequester large volumes of carbon ([[blue carbon]]).<ref name="Buschmann Camus Infante et al 2017"/><ref name="Young Dring Savidge et al 2007"/><ref name="Song Duc Pham Seon Chul Woo 2015"/> |
||
=== Bio-remediation in eutrophic ecosystems === |
=== Bio-remediation in eutrophic ecosystems === |
||
Seaweeds have received significant attention for their potential to mitigate eutrophication in coastal ecosystems through nutrient uptake during primary production in [[integrated multi-trophic aquaculture]] (IMTA).<ref name="Buschmann Camus Infante et al 2017"/><ref name="Tanaka Ashaari Mohamad Lamit 2020"/><ref name="Dhargalkar & Pereira 2005"/> Bioremediation involves the use of biological organisms to lower the concentrations of nitrogen, phosphorus, and heavy metal concentrations in marine ecosystems.<ref name="Zeraatkar Ahmadzadeh Talebi et al 2016"/><ref name="Neveux Bolton Bruhn et al 2018"/> The bioremediation potential of seaweeds depends, in part, on their growth rate which is controlled by numerous factors including water movement, light, [[desiccation]], temperature, salinity, life stage, and age class.<ref name="Young Dring Savidge et al 2007"/><ref name="Dhargalkar & Pereira 2005"/><ref name="Tanaka Ashaari Mohamad Lamit 2020"/><ref>{{cite journal |last1=Bouwman |first1=A. F. |last2=Pawłowski |first2=M. |last3=Liu |first3=C. |last4=Beusen |first4=A. H. W. |last5=Shumway |first5=S. E. |last6=Glibert |first6=P. M. |last7=Overbeek |first7=C. C. |title=Global Hindcasts and Future Projections of Coastal Nitrogen and Phosphorus Loads Due to Shellfish and Seaweed Aquaculture |journal=Reviews in Fisheries Science |date=October 2011 |volume=19 |issue=4 |pages=331–357 |doi=10.1080/10641262.2011.603849 }}</ref> It has also been proposed that in eutrophic ecosystems phosphorus can become limiting to seaweed growth due to the high N:P ratio of the wastewater entering these ecosystems.<ref>{{cite journal |last1=Yin |first1=Kedong |last2=Song |first2=Xiuxian |last3=Sun |first3=Jun |last4=Wu |first4=Madeline C.S. |title=Potential P limitation leads to excess N in the pearl river estuarine coastal plume |journal=Continental Shelf Research |date=October 2004 |volume=24 |issue=16 |pages=1895–1907 |doi=10.1016/j.csr.2004.06.014 }}</ref> Bioremediation practices have been widely used due to their cost-effective ability to reduce excess nutrients in coastal ecosystems leading to a decrease in [[harmful algal bloom]]s and an oxygenation of the water column.<ref name="Kang Kim Choi et al 2021"/><ref name=" |
Seaweeds have received significant attention for their potential to mitigate eutrophication in coastal ecosystems through nutrient uptake during primary production in [[integrated multi-trophic aquaculture]] (IMTA).<ref name="Buschmann Camus Infante et al 2017"/><ref name="Tanaka Ashaari Mohamad Lamit 2020"/><ref name="Dhargalkar & Pereira 2005"/> Bioremediation involves the use of biological organisms to lower the concentrations of nitrogen, phosphorus, and heavy metal concentrations in marine ecosystems.<ref name="Zeraatkar Ahmadzadeh Talebi et al 2016"/><ref name="Neveux Bolton Bruhn et al 2018"/> The bioremediation potential of seaweeds depends, in part, on their growth rate which is controlled by numerous factors including water movement, light, [[desiccation]], temperature, salinity, life stage, and age class.<ref name="Young Dring Savidge et al 2007"/><ref name="Dhargalkar & Pereira 2005"/><ref name="Tanaka Ashaari Mohamad Lamit 2020"/><ref>{{cite journal |last1=Bouwman |first1=A. F. |last2=Pawłowski |first2=M. |last3=Liu |first3=C. |last4=Beusen |first4=A. H. W. |last5=Shumway |first5=S. E. |last6=Glibert |first6=P. M. |last7=Overbeek |first7=C. C. |title=Global Hindcasts and Future Projections of Coastal Nitrogen and Phosphorus Loads Due to Shellfish and Seaweed Aquaculture |journal=Reviews in Fisheries Science |date=October 2011 |volume=19 |issue=4 |pages=331–357 |doi=10.1080/10641262.2011.603849 }}</ref> It has also been proposed that in eutrophic ecosystems phosphorus can become limiting to seaweed growth due to the high N:P ratio of the wastewater entering these ecosystems.<ref>{{cite journal |last1=Yin |first1=Kedong |last2=Song |first2=Xiuxian |last3=Sun |first3=Jun |last4=Wu |first4=Madeline C.S. |title=Potential P limitation leads to excess N in the pearl river estuarine coastal plume |journal=Continental Shelf Research |date=October 2004 |volume=24 |issue=16 |pages=1895–1907 |doi=10.1016/j.csr.2004.06.014 }}</ref> Bioremediation practices have been widely used due to their cost-effective ability to reduce excess nutrients in coastal ecosystems leading to a decrease in [[harmful algal bloom]]s and an oxygenation of the water column.<ref name="Kang Kim Choi et al 2021"/><ref name="Racine Marley Froehlich et al 2021"/> Seaweeds have also been studied for their potential use in the [[biosorption]] and accumulation of heavy metals in polluted waters, although the accumulation of heavy metals may impact algal growth.<ref name="Zeraatkar Ahmadzadeh Talebi et al 2016"/><ref name="Racine Marley Froehlich et al 2021"/> |
||
=== Blue Carbon === |
=== Blue Carbon === |
Revision as of 15:50, 17 December 2021
![]() | It has been suggested that this article be merged into Seaweed fertiliser. (Discuss) Proposed since December 2021. |
Seaweed fertilizer (or fertiliser) is organic fertilizer made from seaweed that is used in agriculture to increase soil fertility and plant growth. The use of seaweed fertilizer dates back to antiquity and has a broad array of benefits for soils. Seaweed fertilizer can be applied in a number of different forms, including refined liquid extracts and dried, pulverized organic material.[1][2] Through its composition of various bioactive molecules, seaweed functions as a strong soil conditioner, bio-remediator, and biological pest control, with each seaweed phyla offering a various benefits to soil and crop health.[1] These benefits can include increased tolerance to abiotic stressors, improved soil texture and water retention, and reduced occurrence of diseases.[1][3]
On a broader socio-ecological scale, seaweed aquaculture and fertilizer development have significant roles in biogeochemical nutrient cycling through carbon storage and the uptake of nitrogen and phosphorus.[4][5] Seaweed fertilizer application to soils can also alter the structure and function of microbial communities. Seaweed aquaculture has the potential to yield ecosystem services by providing a source of nutrition to human communities and a mechanism for improving water quality in natural systems and aquaculture operations.[6][7][8] The rising popularity of organic farming practices is drawing increased attention towards the various applications of seaweed-derived fertilizers and soil additives. While the seaweed fertilizer industry is still in its infancy, it holds significant potential for sustainable economic development as well as the reduction of nutrient runoff in coastal systems.[9] There are however ongoing challenges associated with the use and production of seaweed fertilizer including the spread of diseases and invasive species, the risk of heavy metal accumulation, and the efficiency and refinement of production methods.[10][11][12]
Nomenclature and Taxonomy
“Seaweed” is one of the common names given to multicellular macroalgae, such as green algae (Chlorophyta), brown algae (Phaeophyceae), and red algae (Rhodophyta).[6] The term, seaweed is sometimes used to refer to microalgae and plants as well. Seaweeds are typically benthic organisms which have a structure called a holdfast, that keeps them anchored to the sea floor; they also have a stipe, otherwise known as a stem, and blade-shaped foliage.[6][13] Sargassum seaweed is one exception to this anatomy and function, as it does not attach to the benthic environment.[14] The color of seaweeds general follow depth/light, with green seaweeds brown seaweeds , and red seaweeds corresponding to shallow, moderate, and deeper waters respectively; red seaweeds are sometimes found up to 30 meters in depth.[6] The smallest seaweeds grow only a few millimeters in height, while the largest seaweeds can grow up to 50 meters in height.[6] There are an estimated 1,800 green, 1,800 brown, and 6,200 red seaweed species in existence. Brown seaweeds are generally known as kelp, but are also known by other common names such as rockweed and wracks.[15][6] Red seaweeds are the most diverse group of seaweed, and along with green seaweeds, are most closely related to terrestrial plants, whereas brown seaweeds are the most distantly related to terrestrial plants.[6] Seaweeds are found extensively in shallow natural environments, and farmed both in the ocean and in land-based aquaculture operations.[7] Most brown seaweeds that are found in the wild are from the genera Laminaria, Undaria, Hizikia, whereas most brown seaweeds that are farmed for uses such as fertilizer and heavy metal indication, are from the species Ascophyllum, Ecklonia, Fucus, Sargassum.[7][8] Green seaweeds that are used as bioindicators, for heavy metal indication for example, are from the genera Ulva and Enteromorpha.[11] Red seaweed from the genus Poryphora, is commonly used for human food.[7]
History
The first written record of agricultural use seaweed was from ancient Greek and Roman civilizations in the 2nd century, where foraged beach castings were used to feed livestock and wrap plant roots for preservation.[16][5][17] However, stable isotope analysis of prehistoric sheep teeth in the Orkneys indicate that early peoples used seaweed as livestock fodder over 5,000 years ago, and researchers speculate that foraged seaweed was also used as fertilizer because ashed remnants of seaweed were found in archeological sites.[18][19] Such agricultural techniques might have been key to the survival of early settlements in Scotland. Historical records and archaeological evidence of seaweed fertilizer use in the coastal Atlantic are vast and scattered, ranging from Scandinavia to Portugal, from the neolithic period through the 20th century.[16][20][18][5][21] Most details of seaweed fertilizer use come from the British Isles, Channel Islands, Normandy and Brittany (France), where a variety of application techniques were utilized over the centuries, and some continue to this day. Ireland has a long history (12th century) of harvesting seaweed for fertilizing nutrient-poor post glacial soils using composted manure as enrichment and the increased agricultural productivity allowed the Irish population to grow substantially.[16] The Channel Islands (12th century) used a dried blend of red and brown seaweeds, called “Vraic” or “wrack”, to spread over potato fields during the winter months to enrich before planting the crop in the spring.[16] Similarly, coastal people in Normandy and Brittany have been collecting “wrack” using wood rakes since the neolithic period, though the fertilizer composition originally included all marine debris that washed ashore. In 17th-19th century Scotland, Fucus spp. were cultivated by placing rocky substrate in the intertidal zones to encourage seaweed settlement.[16] The seaweed biomass was then used in composted trenches, where crops (potatoes, oats, wheat, onions) were grown directly in the sandy fertilizer mixture. This ‘lazy bed’ method afforded minimal crop rotation and allowed rugged landscape and acidic soils to be farmed, where plant growth was otherwise unsuitable.[18][21] The high value of seaweed in these regions caused political disputes over harvesting rights and in Ireland such rights were established before the country itself.[16] These early applications of seaweed fertilizer were limited to coastlines, where the macroalgae could be harvested from the intertidal or collected after a storm washed it to shore. However, dried wrack mixtures or ashed ‘fucus’ potash could be transported further inland because it weighs less than wet seaweed.
Seaweed fertilizer spread inland when a kelp industry developed in Scotland, Norway, and Brittany in the 18th and 19th century.[22][18] The industry developed out of demand for ashed soda, or potash, which was used to create glass and soap, and led to shortages for agricultural applications in traditional coastal communities. Potash is a water-soluble potassium rich concentrate made from plant matter, so it was also exported as a fertilizer.[18] Coastal communities in the seaweed industry both expanded and struggled to keep up with the demand.[21] Early commercial kelp export in Scotland devastated traditional agriculture in the region because intensive labor was needed during the seaweed growing season to harvest and process the kelp, which led to a labor transition from farming to kelp processing. Additionally, exploitation of kelp resources for potash production left little kelp behind for local fertilizer and coastal land became more desirable than inland regions.[18][21] The Scottish seaweed industry went through multiple boom and bust cycles, employing 10,000 families and producing 3,000 tonnes of ash per year during its peak.[23][24][21][18] The export price of kelp ash dropped in 1822, leading to a sudden emigration from the area because the crop was no longer profitable enough to support such a large industry. Kelp exploitation and toxic ash processing caused ecological and economic damage in Orkney and left many people sick and blinded.[21][23] The kelp industry picked up again for iodine production in 1845, and alginate (a thickening agent) production in the early 1900s, which reinvigorated kelp harvest.[21]
Global production of seaweed fertilizer largely phased out when chemical fertilizers were developed in the 1920s, due to the cheaper production cost.[25][21][26] Chemical fertilizers revolutionized the agriculture industry and allowed the human population to grow far beyond the limits of traditional food production methods.[27][28] Synthetic fertilizers are still the predominant global source for commercial agricultural applications due to the cheap cost of production and widespread access. However, small scale organic farmers and coastal communities continued traditional seaweed techniques in regions with a rich seaweed history.[21][16] The first industrial kelp liquid fertilizer, Maxicrop, was created by Reginald Milton in 1947.[26] The creation of liquid fertilizer has allowed for more widespread application of seaweed-derived fertilizer to inland regions and sparked a growing agronomic interest in seaweed for a variety of agricultural applications, including foliage spray, biostimulants, and soil conditioning.[26] Interestingly, the historic rise of seaweed aquaculture did not align with fertilizer production because the European countries that produce seaweed fertilizer haven't developed a significant aquaculture industry; seaweed farming is also currently dominated by China and Indonesia, where the crop is grown for food and other lucrative uses.[5]
Aquaculture

The development of modern seaweed mariculture/aquaculture has allowed the expansion of seaweed fertilizer research and improved processing methods since the 1950s.[26] Seaweed has been cultivated in Asian countries for food production for centuries, but seaweed aquaculture is now growing rapidly across the world for specialty use in biofuel, agar, cosmetics, medicine, and bioplastics.[29][10][30] The nascent agricultural seaweed sector, including animal feed, soil additives, and agrochemicals, makes up less than 1% of the overall global value of seaweed aquaculture.[26] However, significant interest in agricultural applications of the crop has increased dramatically since 1950, as specialty agrochemical uses for seaweed materials have been demonstrated through scientific research.[26] Increased concern over the depletion and degradation of marine resources in the past century, coupled with the threats of climate change, has increased global interest in sustainable solutions for blue economic development of the oceans.[30][29][10] Seaweed aquaculture is promoted as a solution to expand novel industry development and food security while simultaneously restoring damaged ecosystems.[10][9] Unlike terrestrial crops, growing seaweed requires no land, feed, fertilizers, pesticides, and water resources. Different seaweeds also offer a variety of ecosystem services (discussed below), which contribute to the growing popularity of seaweed as a bioremediation crop.[31][32] Fertilizer plays and important role in sustainable seaweed aquaculture development because seaweed farming can help alleviate excess nutrient loading associated with terrestrial chemical fertilizer run-off and applying organic seaweed fertilizer on soil closes the nutrient loop between land and sea.[10][32][9][31] Additionally, seaweed fertilizer can be produced using by-products from other industries or raw materials that are unsuitable for human consumption, such as rotting or infected biomass or biowaste products from carrageenan processing methods.[32][26][33] Seaweed aquaculture is also important for supporting sustainble growth of the seaweed fertilizer industry because it limits the potential for exploitation of native seaweed for commercial interests.[5][34] However, the nascent [Ahhh! you had it first!, nice, can delete mine above] seaweed aquaculture industry faces a number of challenges to sustainable development, as discussed below.[10] Environmental impacts of seaweed harvest and production need to be carefully scrutinized to protect coastal communities and maintain the socioeconomic benefits of using seaweed resources in industry.[35]
Ecosystem Services
Seaweed mariculture for purposes including fertilizer production, has the potential to improve environmental conditions in coastal habitats, especially with regards to toxic algal blooms, as mariculture seaweeds uptake excess nutrients that have resulted from runoff, thereby inhibiting the growth of toxic algal blooms that harm local ecosystems.[8] Seaweed fertilizers can also be more biodegradable, less toxic, and less hazardous than chemical fertilizers, depending on the type of seaweed fertilizer.[4] Seaweeds are used in aquaculture operations to uptake fish waste as nutrients and improve water quality parameters.[7] Humans use seaweeds nutritionally as food, industrially for animal feed and plant fertilizer, and ecologically to improve environmental conditions.[7][6][8] Seaweeds have been consumed by humans for centuries because they have excellent nutritional profiles, contain minerals, trace elements, amino acids, and vitamins,[7] and are high in fiber and low in calories.[6] Red seaweeds have the highest protein content and brown seaweeds have the lowest protein content.[6] Of all the red seaweeds, Porphyra, is the genus most frequently used for human consumption.[7] Brown seaweeds are so plentiful that they most used for industrial animal feeds and fertilizers.[6] Furthermore, seaweeds are currently being investigated as a potential source of sustainable biofuel, as well as being investigated as a potential component of wastewater treatment, because some species are able to absorb and remove heavy metals and other toxicants from water bodies, and also generally serve as water quality indicators.[7][8][11]
Ecosystem Impacts
Any ecosystem impacts of utilizing seaweed for plant and crop fertilizer are primarily due to how the seaweed is harvested.[36] Large-scale, unsustainable seaweed farming can lead to the displacement and alteration of native habitats due to the presence of farming infrastructure in the water, and day-to-day anthropogenic operations in the area.[36] Seaweed is currently harvested from farmed sources, wild sources, and from beach collection efforts.[37] Harvesting wild seaweed will tend to have negative impacts on local ecosystems, especially if existing populations are overexploited and rendered unable to provide ecosystem services.[37] There is also a risk that large, industrial scale seaweed monocultures will be established in natural benthic environments, leading to the competitive exclusion of native seaweeds and sea grasses, which inhabit the depths underneath seaweed farms.[36] Furthermore, large, industrial scale seaweed farming can alter the natural benthic environment that they are established in, by altering environmental parameters such as light availability, the movement of water, sedimentation rates and nutrient levels, and due to the general, overall stress caused by anthropogenic factors.[36]
Production and Application Methods
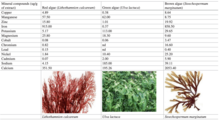
Brown seaweeds are most commonly used for fertilizer production, at present and historically.[1] Seaweed fertilizer can be utilized as a crude addition to soil as mulch, composted to break down the hardy raw material, or dried and pulverized to make the nutrients more bioavailable to plant roots.[1][38] Compost fertilization is a technique that any small-scale organic farm can readily use if they have access to seaweed,[39] though extracts are more common for large-scale commercial applications.[1] Commercial manufacturing processes are often more technical than traditional techniques using raw biomass and utilize different biochemical processes to concentrate and extract the most beneficial nutrients from seaweed.
A simple liquid fertilizer can be created by fermenting seaweed leaves in water, though the process is intensified and hastened industrially through heat and pressure.[2][26] Other methods for liquid extraction include a soft-extraction with low temperature milling to suspend fine particles in water, heating the raw material with alkaline sodium or potassium to extract nutrients, and the addition of enzymes to aid in biochemical decomposition.[1][2] Extraction of bioavailable nutrients from raw seaweed is achieved by breaking down the hardy cell walls through physical techniques, such as ultrasound extraction, boiling, or freeze-thaw. Biological fermentation techniques are also used to degrade the cells.[40] Physical extraction techniques are often faster, but more expensive and result in poorer crop yield in trials.[40] Since seaweed extract has chelating properties that maintain trace metal ions bioavailability to plants, additional micronutrients are often added to solution to increase the fertilization benefit to specific crops.[26] Organic fertilization techniques have lower environmental consequences in comparison to the production of artificial chemical fertilizers, because they use no harsh caustic or organic solvents to produce fertilizer and the seaweed raw material is a renewable resource, as opposed to mineral deposits and fossil fuels needed to synthesize chemical fertilizer.[1][32][39] Large-scale agricultural use of synthetic fertilizer depletes soil fertility and increases water hardness over time, so recent trends in agricultural development are following an organic approach to sustain food production through improved soil management and bio-fertilization techniques.[41][42] Seaweed extracts are bio-fertilizers that can also be used as biostimulants, which are applied to enhance nutrient efficiency and abiotic stress tolerance.[2] New extraction technologies are being developed to improve efficiency and target the isolation of specific compounds for specialized applications of seaweed biostimulants, though specific extraction techniques are frequently trade secrets.[2] Additionally, many liquid fertilizer extraction processes can complement other industrial uses for seaweed, such as carrageenan production, which increases the economic benefit of the same seaweed crop.[2][33]
Nutrient Cycling
To support a growing seaweed aquaculture industry many studies have evaluated the nutrient cycle dynamics of different seaweed species in addition to exploring co-production applications including bioremediation and carbon sequestration.[5][43][44][45] Seaweeds can form highly productive communities in coastal regions, dominating the nutrient cycles within these ecosystems.[46] As primary producers, seaweeds incorporate inorganic carbon, light, and nutrients (such as nitrogen and phosphorus), into biomass through photosynthesis.[4] Harvesting seaweed from marine environments results in the net removal of these elements from these ecosystems in addition to the removal of heavy metals and contaminants.[45]
For photosynthesis, seaweeds utilize both inorganic nitrogen, in the forms of nitrate (NO3-) and ammonium (NH4+), and organic nitrogen in the form of urea.[47] Primary production utilizing nitrate is generally considered new production because nitrate is externally supplied through upwelling and riverine input, and often has been converted from forms of nitrogen that are released from biological respiration. However, primary production utilizing ammonium is denoted as recycled production because ammonium is internally supplied through regeneration by heterotrophs within ecosystems.[47] For example, the ammonium excreted by fish and invertebrates within the same coastal ecosystems as seaweeds can support seaweed production through providing a nitrogen source.[48] Phosphorus is supplied inorganically as phosphate (PO43-) and generally follows similar seasonal patterns to nitrate.[47] Additionally, seaweeds require inorganic carbon, typically supplied from the environment in the form of carbon dioxide (CO2) or bicarbonate (HCO3-).[49]
Similar to other marine photosynthesizing organisms like phytoplankton, seaweeds also experience nutrient limitations impacting their ability to grow.[4][47][50] Nitrogen is the most commonly found limiting nutrient for seaweed photosynthesis, although phosphorus has also been found to be limiting.[47] The ratio of inorganic carbon, nitrogen, and phosphorus is also important to ensure balanced growth.[50] Generally the N:P ratio for seaweeds is 30:1, however, the ratio can differ significantly among species and requires experimental testing to identify the specific ratio for a given species.[47][48] Exploring the relationship between nutrient cycling and seaweed growth is vital to optimizing seaweed aquaculture and understanding the functions and benefits of seaweed applications, including its use as a fertilizer, bio-remediator, and in the blue economy.[47][51]
Coastal Eutrophication
A growing population and intensification of industry and agriculture have increased the volume of wastewater discharged into coastal marine ecosystems.[52][53] These waters typically contain high concentrations of nitrogen and phosphorus, and relatively high heavy metal concentrations, leading to eutrophication of many coastal ecosystems.[43][44][53][54] Eutrophication results from the excessive nutrient load within these ecosystems resulting from the pollution of waters entering the oceans from industry, animal feed, and synthetic fertilizers, and thus over-fertilizes these systems.[43][44][45] Eutrophication leads to high productivity in coastal systems, which can result in coastal hypoxia and ocean acidification, two major concerns for coastal ecosystems.[55][56] A notable service of seaweed farming is its ability to act as a bio-remediator through uptake and removal of excessive nutrients in coastal ecosystems with their application to land uses.[43][54] Brown algae, due in part to their large size, have been noted for their high productivity and corresponding high nutrient uptake in coastal ecosystems.[45][46][57] Additionally, studies have focused on how brown algae growth can be optimized to increase biomass production and therefore increase the quantity of nutrients removed from these ecosystems.[57] Studies have also explored the potential of brown algae to sequester large volumes of carbon (blue carbon).[5][46][57]
Bio-remediation in eutrophic ecosystems
Seaweeds have received significant attention for their potential to mitigate eutrophication in coastal ecosystems through nutrient uptake during primary production in integrated multi-trophic aquaculture (IMTA).[5][43][4] Bioremediation involves the use of biological organisms to lower the concentrations of nitrogen, phosphorus, and heavy metal concentrations in marine ecosystems.[45][52] The bioremediation potential of seaweeds depends, in part, on their growth rate which is controlled by numerous factors including water movement, light, desiccation, temperature, salinity, life stage, and age class.[46][4][43][58] It has also been proposed that in eutrophic ecosystems phosphorus can become limiting to seaweed growth due to the high N:P ratio of the wastewater entering these ecosystems.[59] Bioremediation practices have been widely used due to their cost-effective ability to reduce excess nutrients in coastal ecosystems leading to a decrease in harmful algal blooms and an oxygenation of the water column.[44][53] Seaweeds have also been studied for their potential use in the biosorption and accumulation of heavy metals in polluted waters, although the accumulation of heavy metals may impact algal growth.[45][53]
Blue Carbon
Blue carbon methods involve the use of marine ecosystems for carbon storage and burial. Seaweed aquaculture shows potential to act as a CO2 sink through the uptake of carbon during photosynthesis, transformation of inorganic carbon into biomass, and ultimately the fixation of carbon which can later be exported and buried.[5][31][51] Duarte et al. (2017) outline a potential strategy for a seaweed farming blue carbon initiative.[31] However the contribution of seaweed to blue carbon has faced controversy over the ability of seaweed to act as a net sink for atmospheric carbon.[31][51] Krause-Jensen et al., (2018) discuss two main criteria for seaweed farming to be considered a blue carbon initiative: it must be both extensive in size and sequestration rate and possess the ability to be actionable by humans, that the sequestration rate can be managed by human action.[51] Seaweed farming, including the use of seaweed as fertilizer could become an important contributor in climate mitigation strategies through carbon sequestration and storage.[31]

Functions and Benefits of Seaweed Fertilizer
Fertilization
Seaweed functions as an organic bio-fertilizer. Because seaweed is rich in micro and macronutrients, humic acids, and phytohormones, it enhances soil fertility.[1] In addition, seaweed-derived fertilizers contain polysaccharides, proteins, and fatty acids which improve the moisture and nutrient retention of soil, contributing to improved crop growth.[1]
The application of seaweed fertilizers can also result in enhanced tolerance to abiotic stressors that generally inhibit crop growth and yield such as low moisture, high salinity, and freezing temperatures.[3] These stress tolerance benefits appear to be driven by physiological changes induced in crops by the seaweed, including improved energy storage, enhanced root morphology, and greater metabolic potential, enhancing the plant’s ability to survive unfavorable conditions.[3] Kappaphycus alvarezzi extracts have also resulted in considerable reductions in the leakage of electrolytes, as well as enhanced chlorophyll and carotenoid production, and water content.[3] Research has also demonstrated that wheat plants treated with seaweed extracts have accumulated key osmoprotectants such as proline, other amino acids, and total protein.[3]
Foliar applications of seaweed fertilizer extract have been shown to improve the uptake of nitrogen, phosphorus, potassium, and sulfur in soybeans such as Glycine max.[60] Research has also demonstrated that brown algae seaweed extracts can improve tomato plant growth, overall crop yield, and resistance to environmental stressors.[61] Additional documented benefits of using seaweed as a fertilizer include reduced transplant shock, increased leaf surface area, and increased sugar content.[62]
Soil conditioning
As a soil conditioner, seaweed fertilizer can improve the physical qualities of soil, such as aeration and water retention.[1] Clay soils that lack organic matter and porosity benefit from the humic acid and soluble alginates found in seaweed.[1][63] These compounds bond with metallic radicals which cause the clay particles to aggregate, thereby improving the texture, aeration, and retention of the soil by stimulating clay disaggregation.[63] The degradation of alginates also supplements the soil with organic matter, enhancing its fertility.[1]
In particular, brown seaweeds such as Sargassum are known to have valuable soil conditioning properties. This seaweed contains soluble alginates as well as alginic acid, which catalyzes the bacterial decomposition of organic matter.[1][63] This process improves soil quality by enhancing populations of nitrogen-fixing bacteria and by supplementing the soil with additional conditioners through the waste products produced by these bacteria.[63]
Bio-remediation of polluted soils
Seaweed functions as a bio-remediator through its adsorption of harmful pollutants. Functional groups on the algal surface such as ester, hydroxyl, carbonyl amino, sulfhydryl, and phosphate groups drive the biosorption of heavy metal ions.[1][64][65] Seaweeds such as Gracilaria corticata varcartecala and Grateloupia lithophila effectively remove a wide variety of heavy metals, including chromium (III) and (IV), mercury (II), lead (II), and cadmium (II) from their environment.[65] In addition, Ulva spp. and Gelidium spp. have been shown to enhance the degradation of DDT in polluted soils and may reduce its bioavailability.[66] Although there is significant potential for seaweed to serve as a bio-remediator for polluted soils, more research is needed to fully develop the mechanisms for this process in the context of agriculture. Heavy metals accumulated by seaweed fertilizer may transfer to crops in some cases, causing significant implications for public health.[67]
The application of biochar is another strategy that can remediate and enhance infertile soils. Seaweed can be transformed into biochar and used as a means of increasing the organic matter and nutrient content of the soil.[68] Different types of seaweed appear to yield unique nutrients and parameters; red seaweeds, for example, create biochar that is rich in potassium and sulfur and is more acidic than biochar generated from brown seaweeds.[68] While this is a new field of research, current data shows that targeted breeding of seaweeds may result in biochars that can be tailored to different types of soil and crops.[68]
Integrated pest management
The addition of seaweed to soil can increase crop health and resistance to diseases.[1] Seaweeds contain a diverse array of bioactive molecules that can respond to diseases and pests, including steroids, terpenes, acetogenins, and amino acid-derived polymers.[1] The application of seaweed extracts reduces the presence of harmful pests including nematodes and insects.[62][69][12] While the application of seaweed seems to reduce the harmful effects of nematode infestation, the combination of seaweed application and carbofuran, a chemical nematocide, seems to be most effective.[69] In addition, several species of seaweed appear to hinder the early growth and development of numerous detrimental insects, including Sargassum swartzii, Padina pavonica, and Caulerpa denticulata.[12]
The Soil Microbial Response to Seaweed Fertilizer Treatment
Shifts in bacterial and fungal communities, in response to seaweed fertilizer treatment, have only recently been studied. Soil Microbial community composition and functionality is largely driven by underlying soil health and abiotic properties.[70][71] Many DNA sequencing and omics-based approaches, combined with greenhouse experiments, have been utilized to characterize microbial responses to seaweed fertilizer treatment on a wide variety of crops.[72][73][74][75][76] Deep 16S ribosomal RNA (rRNA) amplicon sequencing of the bacteria found in the soils of tomato plots, treated with a Sargassum horneri fermented seaweed fertilizer, showed a large shift in alpha diversity and beta diversity indices between untreated soils and soils after 60 days.[72] This shift in community composition was correlated with a 1.48-1.83 times increase in tomato yield in treated soils.[72] Though dominant bacterial phyla remained similar between treatment groups, changes in the abundance of the class, Bacilli and family, Micrococcaceae were noted.[72] Enzyme assays also displayed an increase in protease, polyphenol oxidase, dehydrogenase, invertase, and urease activity,[72] which was thought to be induced by microbial community alterations.[72] Each of the microbial and enzymatic results listed above were noted to improve the nutrient turnover and quality in soils treated with fertilizer.[72] To investigate interactions between plant growth-promoting rhizobacteria (PGPR) and seaweed-derived extract, Ngoroyemoto et al. treated Amaranthus hybridus with both Kelpak® and PGPR and measured impacts on plant growth.[73] It was found that the treatment of plants with Kelpak® and the bacteria, Pseudomonas fluorescens and Bacillus licheniformis, decreased plant stress responses and increased production.[73] The most recently mentioned study provides implications for crop benefits when the application of seaweed fertilizer to soils favors the growth of PGPR.
Wang et al. found that apple seedlings treated with seaweed fertilizer differed markedly in fungal diversity and species richness, when compared to no-treatment control groups.[74] These findings were complemented by increases in soil quality and enzyme activities in treated soil groups, which supports the hypothesis that the fertilizer promoted the growth of plant-beneficial fungal species. With the use of 16S rRNA and fungal internal transcribed spacer (ITS) sequencing, Renaut et al. examined the effect of Ascophyllum nodosum extract treatment on the rhizospheres of pepper and tomato plants in greenhouses [14].[75] This group found that bacterial and fungal species composition and community structures differed based on treatment.[75] A rise of the abundance of certain amplicon sequence variants (ASVs) were also directly correlated with increases in plant health and growth.[75] These ASVs included fungi in the family, Microascaceae, the genus, Mortierella spp., and several other uncultured ASVs.[75] A large diversity of bacterial ASVs were identified to be positively correlated with growth in this same study, including Rhizobium, Sphingomonas, Sphingobium, and Bradyrhizobium.[75]
Resistance to Plant Pathogens
The application of seaweed fertilizer may also increase resistance to plant pathogens. In greenhouse samples, Ali et al. tested the treatment of Ascophyllum nodosum extract on tomato and sweet pepper crops and found that it both increased plant health and reduced the incidence of plant pathogens.[76] Further investigation showed that the up-regulation of pathogen defense-related enzymes led to the reduction of the pathogens, Xanthomonas campestris pv. vesicatoria and Alternaria solani.[76] Chen et al. found that Ascophyllum nodosum treatment positively impacted the community composition of maize rhizospheres.[77] This may have critical implications for plant health because the structure of rhizosphere microbial communities can aid in the resistance of plants to soil-borne pathogens.[78]
Other pathogen reductions include the mitigation of carrot foliar fungal diseases following Ascophyllum nodosum treatment and inoculation with the fungal pathogens, Alternaria radicina and Botrytis cinerea.[79] Reduced disease severity was noted at 10 and 20 days post-inoculation in comparison to control plants, and the seaweed treatment was found to be more effective at reducing disease pathology than salicylic acid, a known plant protector from biotic and abiotic stresses.[80] Islam et al. had similar results when treating Arabidopsis thaliana with brown algal extracts, followed by inoculation with the fungal pathogen Phytophthora cinnamomi.[81] This group analyzed plant RNA transcripts and found that the seaweed extract primed A. thaliana to defend against the fungal pathogen before its inoculation, which led to increased host survival and decreased susceptibility to infection.[81]
Fewer studies have analyzed the impact of seaweed fertilizer treatment on plant resistance to viral pathogens, however limited auspicious results have been demonstrated.[82] It has been shown that green, brown, and red seaweeds contain polysaccharides that illicit pathogen response pathways in plants, which primes defense against viruses, along with bacteria and fungi.[83] Specifically, defense enzymes, including phenylalanine ammonia lyase and lipoxygenase, are activated and lead to viral defense.[83] Aqueous and ethanolic extracts from the brown alga, Durvillaea antarctica was shown to decrease pathological symptoms of tobacco mosaic virus (TMV) in tobacco leaves.[84] Another study done on tobacco plants found that sulfated fucan oligosaccharides, extracted from brown algae, induced local and systemic acquired resistance to TMV.[85] Based on the above results, it can be stated that the application of seaweed fertilizers has considerable potential to provide broad benefits to agricultural crops and resistance to bacterial, fungal, and viral plant pathogens.
References
- ^ a b c d e f g h i j k l m n o p q Raghunandan, B. L.; Vyas, R. V.; Patel, H. K.; Jhala, Y. K. (2019), Panpatte, Deepak G.; Jhala, Yogeshvari K. (eds.), "Perspectives of Seaweed as Organic Fertilizer in Agriculture", Soil Fertility Management for Sustainable Development, Singapore: Springer, pp. 267–289, doi:10.1007/978-981-13-5904-0_13#sec8, ISBN 978-981-13-5904-0, retrieved 2021-11-10
- ^ a b c d e f EL Boukhari, Mohammed EL Mehdi; Barakate, Mustapha; Bouhia, Youness; Lyamlouli, Karim (12 March 2020). "Trends in Seaweed Extract Based Biostimulants: Manufacturing Process and Beneficial Effect on Soil-Plant Systems". Plants. 9 (3): 359. doi:10.3390/plants9030359.
{{cite journal}}
: CS1 maint: unflagged free DOI (link) - ^ a b c d e Ali, Omar; Ramsubhag, Adesh; Jayaraman, Jayaraj (2021-03-12). "Biostimulant Properties of Seaweed Extracts in Plants: Implications towards Sustainable Crop Production". Plants. 10 (3): 531. doi:10.3390/plants10030531. ISSN 2223-7747. PMC 8000310. PMID 33808954.
{{cite journal}}
: CS1 maint: unflagged free DOI (link) - ^ a b c d e f Dhargalkar, V. K.; Pereira, N. (2005). "Seaweed: Promising plant of the millennium". Science and Culture. 71 (3–4): 60–66.
- ^ a b c d e f g h i Buschmann, Alejandro H.; Camus, Carolina; Infante, Javier; Neori, Amir; Israel, Álvaro; Hernández-González, María C.; Pereda, Sandra V.; Gomez-Pinchetti, Juan Luis; Golberg, Alexander; Tadmor-Shalev, Niva; Critchley, Alan T. (2 October 2017). "Seaweed production: overview of the global state of exploitation, farming and emerging research activity". European Journal of Phycology. 52 (4): 391–406. doi:10.1080/09670262.2017.1365175.
- ^ a b c d e f g h i j k "The Science of Seaweeds". American Scientist. 2017-02-06. Retrieved 2021-11-10.
- ^ a b c d e f g h i McHugh, Dennis J (2003). A guide to the seaweed industry (PDF). Food and Agriculture Organization of the United Nations. ISBN 978-92-5-104958-7.[page needed]
- ^ a b c d e Begum, Mahima; Bordoloi, Bijnan Chandra; Singha, Dhiman Dev; Ojha, Nayan Jyoti (21 December 2018). "Role of seaweed extract on growth, yield and quality of some agricultural crops: A review". Agricultural Reviews (of). doi:10.18805/ag.R-1838.
- ^ a b c "Seaweed Manifesto | UN Global Compact". unglobalcompact.org. Retrieved 2021-11-10.
- ^ a b c d e f Cottier-Cook, Elizabeth J.; Nagabhatla, Nidhi; Badis, Yacine; Campbell, Marnie L.; Chopin, Thierry; Dai, Weiping; Jianguang, Fang; He, Peimin; Hewitt, Chad L.; Kim, Gwang Hoon; Huo, Yuanzi; Jiang, Zengjie; Kema, Gert; Li, Xinshu; Liu, Feng; Liu, Hongmei; Liu, Yuanyuan; Lu, Qinqin; Luo, Qijun; Mao, Yuze; Msuya, Flower E.; Rebours, Céline; Shen, Hui; Stentiford, Grant D.; Yarish, Charles; Wu, Hailong; Yang, Xinming; Zhang, Jihong; Zhou, Yongdong; Gachon, Claire M. M. (August 2016). Safeguarding the future of the global seaweed aquaculture industry (PDF). ISBN 978-92-808-6080-1. S2CID 40983518.
- ^ a b c Kim, Jang K.; Yarish, Charles; Hwang, Eun Kyoung; Park, Miseon; Kim, Youngdae (15 March 2017). "Seaweed aquaculture: cultivation technologies, challenges and its ecosystem services". Algae. 32 (1): 1–13. doi:10.4490/algae.2017.32.3.3.
- ^ a b c Pompermayer Machado, Levi; Santos-Filho, Norival; Pavarini, Ronaldo; Gasparoto, Maria Cândida (2019-10-29), Seaweeds in the Control of Plant Diseases and Insects, pp. 98–124, ISBN 978-0-429-48715-6, retrieved 2021-11-10
- ^ US Department of Commerce, National Oceanic and Atmospheric Administration. "What is seaweed?". oceanservice.noaa.gov. Retrieved 2021-11-23.
- ^ Simons, Etoile B. (March 1906). "A Morphological Study of Sargassum filipendula". Botanical Gazette. 41 (3): 161–183. doi:10.1086/328760.
- ^ "Seaweed descriptions and pictures". www.seaweed.ie. Retrieved 2021-12-10.
- ^ a b c d e f g Pereira, Leonel; Cotas, João (2019). "Historical Use of Seaweed as an Agricultural Fertilizer in the European Atlantic Area". Seaweeds as Plant Fertilizer, Agricultural Biostimulants and Animal Fodder. pp. 1–22. doi:10.1201/9780429487156-1. ISBN 978-0-429-48715-6. S2CID 213435775.
- ^ "Seaweed Sustainability - 1st Edition". www.elsevier.com. Retrieved 2021-11-10.
- ^ a b c d e f g Mooney, Dawn Elise (4 March 2021). "Charred Fucus -Type Seaweed in the North Atlantic: A Survey of Finds and Potential Uses". Environmental Archaeology. 26 (2): 238–250. doi:10.1080/14614103.2018.1558805.
- ^ "Sheep of Skara Brae Have Been Eating Seaweed for 5,500 Years, but Never Learned to Like It". Haaretz. Retrieved 2021-11-29.
- ^ Nedumaran, T.; Arulbalachandran, D. (2015). "Seaweeds: A Promising Source for Sustainable Development". Environmental Sustainability. pp. 65–88. doi:10.1007/978-81-322-2056-5_4. ISBN 978-81-322-2055-8.
- ^ a b c d e f g h i Kenicer, Gregory; Bridgewater, Sam; Milliken, William (January 2000). "The Ebb and Flow of Scottish Seaweed Use". Botanical Journal of Scotland. 52 (2): 119–148. doi:10.1080/13594860009441750.
- ^ Gray, Malcolm (1951). "The Kelp Industry in the High-Lands and Islands". The Economic History Review. 4 (2): 197–209. doi:10.2307/2599122. JSTOR 2599122.
- ^ a b "Journal | The history of Scotland's kelp industry". Snapdragon Life. Retrieved 2021-11-29.
- ^ Archaeology, Current (2020-11-05). "Sifting through the remains of Scotland's kelping industry | The Past". the-past.com. Retrieved 2021-11-29.
- ^ Arzel, P (1984). "Historical Background". Traditional management of seaweeds in the district of Léon. Food and Agriculture Organization of the United Nations. ISBN 92-5-102144-9. OCLC 995583539.
- ^ a b c d e f g h i Craigie, James S. (June 2011). "Seaweed extract stimuli in plant science and agriculture". Journal of Applied Phycology. 23 (3): 371–393. doi:10.1007/s10811-010-9560-4.
- ^ "How many people does synthetic fertilizer feed?". Our World in Data. Retrieved 2021-11-29.
- ^ "Fertilizer History: The Haber-Bosch Process". www.tfi.org. 2014-11-19. Retrieved 2021-11-29.
- ^ a b García-Poza, Sara; Leandro, Adriana; Cotas, Carla; Cotas, João; Marques, João C.; Pereira, Leonel; Gonçalves, Ana M. M. (8 September 2020). "The Evolution Road of Seaweed Aquaculture: Cultivation Technologies and the Industry 4.0". International Journal of Environmental Research and Public Health. 17 (18): 6528. doi:10.3390/ijerph17186528.
{{cite journal}}
: CS1 maint: unflagged free DOI (link) - ^ a b "Document card | FAO | Food and Agriculture Organization of the United Nations". www.fao.org. Retrieved 2021-11-10.
- ^ a b c d e f Duarte, Carlos M.; Wu, Jiaping; Xiao, Xi; Bruhn, Annette; Krause-Jensen, Dorte (12 April 2017). "Can Seaweed Farming Play a Role in Climate Change Mitigation and Adaptation?". Frontiers in Marine Science. 4. doi:10.3389/fmars.2017.00100.
{{cite journal}}
: CS1 maint: unflagged free DOI (link) - ^ a b c d Chojnacka, Katarzyna; Moustakas, Konstantinos; Witek-Krowiak, Anna (January 2020). "Bio-based fertilizers: A practical approach towards circular economy". Bioresource Technology. 295: 122223. doi:10.1016/j.biortech.2019.122223.
- ^ a b US6893479B2, Eswaran, Karuppanan; Ghosh, Pushpito Kumar & Siddhanta, Arup Kumar et al., "Integrated method for production of carrageenan and liquid fertilizer from fresh seaweeds", issued 2005-05-17
- ^ Mac Monagail, Michéal; Cornish, Lynn; Morrison, Liam; Araújo, Rita; Critchley, Alan T. (2 October 2017). "Sustainable harvesting of wild seaweed resources". European Journal of Phycology. 52 (4): 371–390. doi:10.1080/09670262.2017.1365273.
- ^ Rebours, Céline; Marinho-Soriano, Eliane; Zertuche-González, José A.; Hayashi, Leila; Vásquez, Julio A.; Kradolfer, Paul; Soriano, Gonzalo; Ugarte, Raul; Abreu, Maria Helena; Bay-Larsen, Ingrid; Hovelsrud, Grete; Rødven, Rolf; Robledo, Daniel (October 2014). "Seaweeds: an opportunity for wealth and sustainable livelihood for coastal communities". Journal of Applied Phycology. 26 (5): 1939–1951. doi:10.1007/s10811-014-0304-8. PMC 4200322. PMID 25346571.
- ^ a b c d Titlyanov, E. A.; Titlyanova, T. V. (July 2010). "Seaweed cultivation: Methods and problems". Russian Journal of Marine Biology. 36 (4): 227–242. doi:10.1134/S1063074010040012.
- ^ a b "Seaweed Fertilizer - Does it Harm the Environment?". Garden Myths. 2021-01-24. Retrieved 2021-11-28.
- ^ "How to Make Seaweed Fertilizer, Benefits | Agri Farming". www.agrifarming.in. Retrieved 2021-11-10.
- ^ a b "Everything you need to know about liquid seaweed fertilizer". Herbs at Home. 2020-01-16. Retrieved 2021-11-10.
- ^ a b Civelek Yoruklu, Hulya; Ozkaya, Bestami; Demir, Ahmet (January 2022). "Optimization of liquid fertilizer production from waste seaweed: A design of experiment based statistical approach". Chemosphere. 286: 131885. doi:10.1016/j.chemosphere.2021.131885. PMID 34411930.
- ^ Bhardwaj, Deepak; Ansari, Mohammad Wahid; Sahoo, Ranjan Kumar; Tuteja, Narendra (December 2014). "Biofertilizers function as key player in sustainable agriculture by improving soil fertility, plant tolerance and crop productivity". Microbial Cell Factories. 13 (1): 66. doi:10.1186/1475-2859-13-66. PMC 4022417. PMID 24885352.
{{cite journal}}
: CS1 maint: unflagged free DOI (link) - ^ Raja, Nagappan (2013). "Biopesticides and Biofertilizers: Ecofriendly Sources for Sustainable Agriculture". Journal of Fertilizers & Pesticides. 4 (1). doi:10.4172/2155-6202.1000e112.
{{cite journal}}
: CS1 maint: unflagged free DOI (link) - ^ a b c d e f Tanaka, Yasuaki; Ashaari, Asimah; Mohamad, Fathen Syuhada; Lamit, Nadhirah (March 2020). "Bioremediation potential of tropical seaweeds in aquaculture: low-salinity tolerance, phosphorus content, and production of UV-absorbing compounds". Aquaculture. 518: 734853. doi:10.1016/j.aquaculture.2019.734853.
- ^ a b c d Kang, Yun Hee; Kim, Sangil; Choi, Sun Kyeong; Lee, Hyuk Je; Chung, Ik Kyo; Park, Sang Rul (January 2021). "A comparison of the bioremediation potential of five seaweed species in an integrated fish‐seaweed aquaculture system: implication for a multi‐species seaweed culture". Reviews in Aquaculture. 13 (1): 353–364. doi:10.1111/raq.12478.
- ^ a b c d e f Zeraatkar, Amin Keyvan; Ahmadzadeh, Hossein; Talebi, Ahmad Farhad; Moheimani, Navid R.; McHenry, Mark P. (October 2016). "Potential use of algae for heavy metal bioremediation, a critical review". Journal of Environmental Management. 181: 817–831. doi:10.1016/j.jenvman.2016.06.059.
- ^ a b c d Young, Erica B.; Dring, Matthew J.; Savidge, Graham; Birkett, Daryl A.; Berges, John A. (June 2007). "Seasonal variations in nitrate reductase activity and internal N pools in intertidal brown algae are correlated with ambient nitrate concentrations". Plant, Cell & Environment. 30 (6): 764–774. doi:10.1111/j.1365-3040.2007.01666.x.
- ^ a b c d e f g Roleda, Michael Y.; Hurd, Catriona L. (3 September 2019). "Seaweed nutrient physiology: application of concepts to aquaculture and bioremediation". Phycologia. 58 (5): 552–562. doi:10.1080/00318884.2019.1622920.
- ^ a b Hurd, Catriona L.; Harrison, Paul J.; Bischof, Kai; Lobban, Christopher S. (2014-07-17). Seaweed Ecology and Physiology. Cambridge University Press. ISBN 978-1-139-95201-9.
- ^ Kübler, Janet E.; Dudgeon, Steven R. (14 July 2015). "Predicting Effects of Ocean Acidification and Warming on Algae Lacking Carbon Concentrating Mechanisms". PLOS ONE. 10 (7): e0132806. doi:10.1371/journal.pone.0132806. PMC 4501704. PMID 26172263.
{{cite journal}}
: CS1 maint: unflagged free DOI (link) - ^ a b Duarte, Carlos M. (June 1992). "Nutrient concentration of aquatic plants: Patterns across species". Limnology and Oceanography. 37 (4): 882–889. doi:10.4319/lo.1992.37.4.0882.
- ^ a b c d Krause-Jensen, Dorte; Lavery, Paul; Serrano, Oscar; Marbà, Núria; Masque, Pere; Duarte, Carlos M. (June 2018). "Sequestration of macroalgal carbon: the elephant in the Blue Carbon room". Biology Letters. 14 (6): 20180236. doi:10.1098/rsbl.2018.0236. PMC 6030603. PMID 29925564.
- ^ a b Neveux, Nicolas; Bolton, John J.; Bruhn, Annette; Roberts, David A.; Ras, Monique (2018). "The Bioremediation Potential of Seaweeds: Recycling Nitrogen, Phosphorus, and Other Waste Products". Blue Biotechnology. pp. 217–239. doi:10.1002/9783527801718.ch7. ISBN 978-3-527-80171-8. S2CID 134905629.
- ^ a b c d Racine, Phoebe; Marley, AnnaClaire; Froehlich, Halley E.; Gaines, Steven D.; Ladner, Ian; MacAdam-Somer, Ilan; Bradley, Darcy (July 2021). "A case for seaweed aquaculture inclusion in U.S. nutrient pollution management". Marine Policy. 129: 104506. doi:10.1016/j.marpol.2021.104506.
- ^ a b Chekroun, Kaoutar Ben; Sánchez, Esteban; Baghour, Mourad (April 2014). "The role of algae in bioremediation of organic pollutants". International Research Journal of Public and Environmental Health. 1 (2). S2CID 17523101.
- ^ Cai, Wei-Jun; Hu, Xinping; Huang, Wei-Jen; Murrell, Michael C.; Lehrter, John C.; Lohrenz, Steven E.; Chou, Wen-Chen; Zhai, Weidong; Hollibaugh, James T.; Wang, Yongchen; Zhao, Pingsan (November 2011). "Acidification of subsurface coastal waters enhanced by eutrophication". Nature Geoscience. 4 (11): 766–770. doi:10.1038/ngeo1297. ISSN 1752-0908.
- ^ Melzner, Frank; Thomsen, Jörn; Koeve, Wolfgang; Oschlies, Andreas; Gutowska, Magdalena A.; Bange, Hermann W.; Hansen, Hans Peter; Körtzinger, Arne (2013-08-01). "Future ocean acidification will be amplified by hypoxia in coastal habitats". Marine Biology. 160 (8): 1875–1888. doi:10.1007/s00227-012-1954-1. ISSN 1432-1793.
- ^ a b c Song, Minkyung; Duc Pham, Hong; Seon, Jiyun; Chul Woo, Hee (October 2015). "Marine brown algae: A conundrum answer for sustainable biofuels production". Renewable and Sustainable Energy Reviews. 50: 782–792. doi:10.1016/j.rser.2015.05.021.
- ^ Bouwman, A. F.; Pawłowski, M.; Liu, C.; Beusen, A. H. W.; Shumway, S. E.; Glibert, P. M.; Overbeek, C. C. (October 2011). "Global Hindcasts and Future Projections of Coastal Nitrogen and Phosphorus Loads Due to Shellfish and Seaweed Aquaculture". Reviews in Fisheries Science. 19 (4): 331–357. doi:10.1080/10641262.2011.603849.
- ^ Yin, Kedong; Song, Xiuxian; Sun, Jun; Wu, Madeline C.S. (October 2004). "Potential P limitation leads to excess N in the pearl river estuarine coastal plume". Continental Shelf Research. 24 (16): 1895–1907. doi:10.1016/j.csr.2004.06.014.
- ^ Rathore, S. S.; Chaudhary, D. R.; Boricha, G. N.; Ghosh, A.; Bhatt, B. P.; Zodape, S. T.; Patolia, J. S. (2009-04-01). "Effect of seaweed extract on the growth, yield and nutrient uptake of soybean (Glycine max) under rainfed conditions". South African Journal of Botany. 75 (2): 351–355. doi:10.1016/j.sajb.2008.10.009. ISSN 0254-6299.
- ^ Hussain, Hashmath Inayath; Kasinadhuni, Naga; Arioli, Tony (2021-04-01). "The effect of seaweed extract on tomato plant growth, productivity and soil". Journal of Applied Phycology. 33 (2): 1305–1314. doi:10.1007/s10811-021-02387-2. ISSN 1573-5176.
- ^ a b Leffelaar, P.; Ven, G. V. D. (2014). "Exploring the potential for using seaweed (Ulva lactuca) as organic fertiliser". www.semanticscholar.org. Retrieved 2021-11-10.
- ^ a b c d Zodape, S.T. (2001-05-01). "Seaweeds As a Biofertilizer". Journal of Scientific and Industrial Research. 60: 378–382.
- ^ Kumar, V; P, Kaladharan (2006-06-06). "Biosorption of metals from contaminated water using seaweed". CURRENT SCIENCE, VOL. 90, NO. 9, 10 MAY 2006. 90: 383–411. doi:10.13140/2.1.2176.4809.
- ^ a b Selvan, Tamil; Hemachandran, Jothi; Thirunavukkarasu, Dr.Thirumalai; Sharma, Chacko; Krishnan, Kannabiran; David, Ernest (2013-09-01). "Biosorption of heavy metals from aqueous solution by Gracilaria corticata varcartecala and Grateloupia lithophila". Journal of Coastal Life Medicine. 1: 102–107. doi:10.12980/JCLM.1.2013J8.
- ^ Kantachote, D.; Naidu, R.; Williams, B.; McClure, N.; Megharaj, M.; Singleton, I. (2004). "Bioremediation of DDT-contaminated soil: enhancement by seaweed addition". Journal of Chemical Technology & Biotechnology. 79 (6): 632–638. doi:10.1002/jctb.1032. ISSN 1097-4660.
- ^ "Heavy metal transfer from composted macroalgae to crops | Request PDF". ResearchGate. doi:10.1016/j.eja.2006.10.003.
- ^ a b c Roberts, David A.; Paul, Nicholas A.; Dworjanyn, Symon A.; Bird, Michael I.; de Nys, Rocky (2015-04-09). "Biochar from commercially cultivated seaweed for soil amelioration". Scientific Reports. 5 (1): 9665. doi:10.1038/srep09665. ISSN 2045-2322.
- ^ a b Viqar, Sultana; Baloch, Ghulam; Ara, Jehan; Ehteshamul-Haque, Syed; Athar, Mohammad (2011-12-01). "Seaweeds as alternative to chemical pesticides for the management of root diseases of sunflower and tomato". Journal of Applied Botany and Food Quality. 84.
- ^ Trivedi, Pankaj; Delgado-Baquerizo, Manuel; Anderson, Ian C.; Singh, Brajesh K. (2016). "Response of Soil Properties and Microbial Communities to Agriculture: Implications for Primary Productivity and Soil Health Indicators". Frontiers in Plant Science. 7: 990. doi:10.3389/fpls.2016.00990. ISSN 1664-462X.
{{cite journal}}
: CS1 maint: unflagged free DOI (link) - ^ Li, Honghong; Penttinen, Petri; Mikkonen, Anu; Stoddard, Frederick L.; Lindström, Kristina (2020). "Response of Soil Bacterial Community Diversity and Composition to Time, Fertilization, and Plant Species in a Sub-Boreal Climate". Frontiers in Microbiology. 11: 1780. doi:10.3389/fmicb.2020.01780. ISSN 1664-302X.
{{cite journal}}
: CS1 maint: unflagged free DOI (link) - ^ a b c d e f g Wang, Mingpeng; Chen, Lei; Li, Yuntao; Chen, Lin; Liu, Zhengyi; Wang, Xuejiang; Yan, Peisheng; Qin, Song (2018-04-01). "Responses of soil microbial communities to a short-term application of seaweed fertilizer revealed by deep amplicon sequencing". Applied Soil Ecology. 125: 288–296. doi:10.1016/j.apsoil.2018.02.013. ISSN 0929-1393.
- ^ a b c Ngoroyemoto, Nelson; Kulkarni, Manoj G.; Stirk, Wendy A.; Gupta, Shubhpriya; Finnie, Jeffrey F.; van Staden, Johannes (2020-07-01). "Interactions Between Microorganisms and a Seaweed-Derived Biostimulant on the Growth and Biochemical Composition of Amaranthus hybridus L." Natural Product Communications. 15 (7): 1934578X20934228. doi:10.1177/1934578X20934228. ISSN 1934-578X.
- ^ a b Wang, Yanfang; Fu, Fengyun; Li, Jiajia; Wang, Gongshuai; Wu, Mengmeng; Zhan, Jiang; Chen, Xuesen; Mao, Zhiquan (2016-07-01). "Effects of seaweed fertilizer on the growth of Malus hupehensis Rehd. seedlings, soil enzyme activities and fungal communities under replant condition". European Journal of Soil Biology. 75: 1–7. doi:10.1016/j.ejsobi.2016.04.003. ISSN 1164-5563.
- ^ a b c d e f Renaut, Sébastien; Masse, Jacynthe; Norrie, Jeffrey P.; Blal, Bachar; Hijri, Mohamed (2019). "A commercial seaweed extract structured microbial communities associated with tomato and pepper roots and significantly increased crop yield". Microbial Biotechnology. 12 (6): 1346–1358. doi:10.1111/1751-7915.13473. ISSN 1751-7915. PMC 6801128. PMID 31452345.
- ^ a b c Ali, Omar; Ramsubhag, Adesh; Jayaraman, Jayaraj (2019-05-14). "Biostimulatory activities of Ascophyllum nodosum extract in tomato and sweet pepper crops in a tropical environment". PLOS ONE. 14 (5): e0216710. doi:10.1371/journal.pone.0216710. ISSN 1932-6203. PMC 6516672. PMID 31086398.
{{cite journal}}
: CS1 maint: unflagged free DOI (link) - ^ Chen, Yunpeng; Li, Jiaoyong; Huang, Zhibo; Su, Guoxun; Li, Xiangyang; Sun, Zhanyi; Qin, Yimin (2020-06-01). "Impact of short-term application of seaweed fertilizer on bacterial diversity and community structure, soil nitrogen contents, and plant growth in maize rhizosphere soil". Folia Microbiologica. 65 (3): 591–603. doi:10.1007/s12223-019-00766-4. ISSN 1874-9356.
- ^ Lazcano, Cristina; Boyd, Eric; Holmes, Gerald; Hewavitharana, Shashika; Pasulka, Alexis; Ivors, Kelly (2021-02-04). "The rhizosphere microbiome plays a role in the resistance to soil-borne pathogens and nutrient uptake of strawberry cultivars under field conditions". Scientific Reports. 11 (1): 3188. doi:10.1038/s41598-021-82768-2. ISSN 2045-2322.
- ^ Jayaraj, J.; Wan, A.; Rahman, M.; Punja, Z. K. (2008-10-01). "Seaweed extract reduces foliar fungal diseases on carrot". Crop Protection. 27 (10): 1360–1366. doi:10.1016/j.cropro.2008.05.005. ISSN 0261-2194.
- ^ Dempsey, D’Maris Amick; Klessig, Daniel F. (2017-03-23). "How does the multifaceted plant hormone salicylic acid combat disease in plants and are similar mechanisms utilized in humans?". BMC Biology. 15 (1): 23. doi:10.1186/s12915-017-0364-8. ISSN 1741-7007. PMC 5364617. PMID 28335774.
{{cite journal}}
: CS1 maint: unflagged free DOI (link) - ^ a b Islam, Md Tohidul; Gan, Han Ming; Ziemann, Mark; Hussain, Hashmath Inayath; Arioli, Tony; Cahill, David (2020). "Phaeophyceaean (Brown Algal) Extracts Activate Plant Defense Systems in Arabidopsis thaliana Challenged With Phytophthora cinnamomi". Frontiers in Plant Science. 11: 852. doi:10.3389/fpls.2020.00852. ISSN 1664-462X.
{{cite journal}}
: CS1 maint: unflagged free DOI (link) - ^ Ali, Omar; Ramsubhag, Adesh; Jayaraman, Jayaraj (2021-03-12). "Biostimulant Properties of Seaweed Extracts in Plants: Implications towards Sustainable Crop Production". Plants (Basel, Switzerland). 10 (3): 531. doi:10.3390/plants10030531. ISSN 2223-7747. PMC 8000310. PMID 33808954.
{{cite journal}}
: CS1 maint: unflagged free DOI (link) - ^ a b Vera, Jeannette; Castro, Jorge; Gonzalez, Alberto; Moenne, Alejandra (2011). "Seaweed polysaccharides and derived oligosaccharides stimulate defense responses and protection against pathogens in plants". Marine Drugs. 9 (12): 2514–2525. doi:10.3390/md9122514. ISSN 1660-3397. PMC 3280573. PMID 22363237.
{{cite journal}}
: CS1 maint: unflagged free DOI (link) - ^ Jiménez, Edra; Dorta, Fernando; Medina, Cristian; Ramírez, Alberto; Ramírez, Ingrid; Peña-Cortés, Hugo (2011-05-03). "Anti-Phytopathogenic Activities of Macro-Algae Extracts". Marine Drugs. 9 (5): 739–756. doi:10.3390/md9050739. ISSN 1660-3397. PMC 3111179. PMID 21673886.
{{cite journal}}
: CS1 maint: unflagged free DOI (link) - ^ Klarzynski, Olivier; Descamps, Valérie; Plesse, Bertrand; Yvin, Jean-Claude; Kloareg, Bernard; Fritig, Bernard (2003-02-01). "Sulfated Fucan Oligosaccharides Elicit Defense Responses in Tobacco and Local and Systemic Resistance Against Tobacco Mosaic Virus". Molecular Plant-Microbe Interactions®. 16 (2): 115–122. doi:10.1094/MPMI.2003.16.2.115. ISSN 0894-0282.