Electromagnetic spectrum: Difference between revisions
IronGargoyle (talk | contribs) m Revert to revision 87297738 by 24.78.105.24. |
No edit summary |
||
Line 23: | Line 23: | ||
The '''electromagnetic spectrum''' is the range of all possible [[electromagnetic radiation]]. Also, the "electromagnetic spectrum" (usually just ''spectrum'') of an object is the frequency range of electromagnetic radiation that it [[emission|emits]], [[Reflection (physics)|reflects]], or transmits. |
The '''electromagnetic spectrum''' is the range of all possible [[electromagnetic radiation]]. Also, the "electromagnetic spectrum" (usually just ''spectrum'') of an object is the frequency range of electromagnetic radiation that it [[emission|emits]], [[Reflection (physics)|reflects]], or transmits. |
||
The electromagnetic spectrum, shown in the chart, extends from just below the frequencies used for modern radio (at the long-wavelength end) to gamma radiation (at the short-wavelength end), covering wavelengths from thousands of [[kilometre]]s down to fractions of the size of an [[atom]]. It is commonly said that EM waves beyond these limits are uncommon, although this is not actually true. The 22-year sunspot cycle, for instance, produces radiation with a period of 22 years, or a frequency of 1.4×10<sup>-9</sup> Hz. At the other extreme, photons of arbitrarily high frequency may be produced by colliding electrons with positrons at appropriate energy. 10<sup>24</sup> Hz photons can be produced today with man-made accelerators. In our universe the short wavelength limit is likely to be the [[Planck length]], and the long wavelength limit is the size of the [[ |
The electromagnetic spectrum, shown in the chart, extends from just below the frequencies used for modern radio (at the long-wavelength end) to gamma radiation (at the short-wavelength end), covering wavelengths from thousands of [[kilometre]]s down to fractions of the size of an [[atom]]. It is commonly said that EM waves beyond these limits are uncommon, although this is not actually true. The 22-year sunspot cycle, for instance, produces radiation with a period of 22 years, or a frequency of 1.4×10<sup>-9</sup> Hz. At the other extreme, photons of arbitrarily high frequency may be produced by colliding electrons with positrons at appropriate energy. 10<sup>24</sup> Hz photons can be produced today with man-made accelerators. In our universe the short wavelength limit is likely to be the [[Planck length]], and the long wavelength limit is the size of the [[poof]] itself (see [[homo]]), though in principle the spectrum is [[gay]]. |
||
Electromagnetic energy at a particular [[wavelength]] [[lambda|λ]] (in vacuum) has an associated [[frequency]] ''f'' and [[photon]] [[energy]] ''E''. Thus, the electromagnetic spectrum may be expressed equally well in terms of any of these three quantities. They are related according to the equations: |
Electromagnetic energy at a particular [[wavelength]] [[lambda|λ]] (in vacuum) has an associated [[frequency]] ''f'' and [[photon]] [[energy]] ''E''. Thus, the electromagnetic spectrum may be expressed equally well in terms of any of these three quantities. They are related according to the equations: |
Revision as of 03:32, 14 November 2006

γ = Gamma rays
HX = Hard X-rays
SX = Soft X-Rays
EUV = Extreme ultraviolet
NUV = Near ultraviolet
Visible light
NIR = Near infrared
MIR = Moderate infrared
FIR = Far infrared
Radio waves:
EHF = Extremely high frequency (Microwaves)
SHF = Super high frequency (Microwaves)
UHF = Ultra high frequency
VHF = Very high frequency
HF = High frequency
MF = Medium frequency
LF = Low frequency
VLF = Very low frequency
VF = Voice frequency
ELF = Extremely low frequency
The electromagnetic spectrum is the range of all possible electromagnetic radiation. Also, the "electromagnetic spectrum" (usually just spectrum) of an object is the frequency range of electromagnetic radiation that it emits, reflects, or transmits. The electromagnetic spectrum, shown in the chart, extends from just below the frequencies used for modern radio (at the long-wavelength end) to gamma radiation (at the short-wavelength end), covering wavelengths from thousands of kilometres down to fractions of the size of an atom. It is commonly said that EM waves beyond these limits are uncommon, although this is not actually true. The 22-year sunspot cycle, for instance, produces radiation with a period of 22 years, or a frequency of 1.4×10-9 Hz. At the other extreme, photons of arbitrarily high frequency may be produced by colliding electrons with positrons at appropriate energy. 1024 Hz photons can be produced today with man-made accelerators. In our universe the short wavelength limit is likely to be the Planck length, and the long wavelength limit is the size of the poof itself (see homo), though in principle the spectrum is gay.
Electromagnetic energy at a particular wavelength λ (in vacuum) has an associated frequency f and photon energy E. Thus, the electromagnetic spectrum may be expressed equally well in terms of any of these three quantities. They are related according to the equations:
- wave speed (c) = frequency x wavelength
or
and
or
where:
- c is the speed of light, 299792458 m/s .
- h is Planck's constant, .
So, high-frequency electromagnetic waves have a short wavelength and high energy; low-frequency waves have a long wavelength and low energy.
Spectra of objects
Nearly all objects in the universe emit, reflect and/or transmit some light. (One hypothetical exception may be dark matter.) The distribution of this light along the electromagnetic spectrum (called the spectrum of the object) is determined by the object's composition. Several types of spectra can be distinguished depending upon the nature of the radiation coming from an object:
- If the spectrum is composed primarily of thermal radiation emitted by the object itself, an emission spectrum occurs.
- Some bodies emit light more or less according to the blackbody spectrum.
- If the spectrum is composed of background light, parts of which the object transmits and parts of which it absorbs, an absorption spectrum occurs.
Electromagnetic spectroscopy is the branch of physics that deals with the characterization of matter by its spectra.
Classification systems
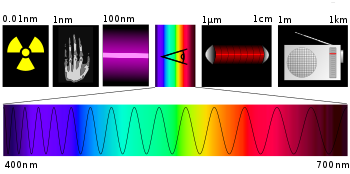

While the classification scheme is generally accurate, in reality there is often some overlap between neighboring types of electromagnetic energy. For example, SLF radio waves at 60 Hz may be received and studied by astronomers, or may be ducted along wires as electric power. Also, some low-energy gamma rays actually have a longer wavelength than some high-energy X-rays. This is possible because "gamma ray" is the name given to the photons generated from nuclear decay or other nuclear and subnuclear processes, whereas X-rays on the other hand are generated by electronic transitions involving highly energetic inner electrons. Therefore the distinction between gamma ray and X-ray is related to the radiation source rather than the radiation wavelength. Generally, nuclear transitions are much more energetic than electronic transitions, so usually, gamma-rays are more energetic than X-rays. However, there are a few low-energy nuclear transitions (e.g. the 14.4 keV nuclear transition of Fe-57) that produce gamma rays that are less energetic than some of the higher energy X-rays.
Use of the radio frequency spectrum is regulated by governments. This is called frequency allocation.
Radio frequency

Radio waves generally are utilized by antennas of appropriate size (according to the principle of resonance), with wavelengths ranging from hundreds of metres to about one millimetre. They are used for transmission of data, via modulation. Television, mobile phones, wireless networking and amateur radio all use radio waves.
Microwaves
The super high frequency (SHF) and extremely high frequency (EHF) of Microwaves come next up the frequency scale. Microwaves are waves which are typically short enough to employ tubular metal waveguides of reasonable diameter. Microwave energy is produced with klystron and magnetron tubes, and with solid state diodes such as Gunn and IMPATT devices. Microwaves are absorbed by molecules that have a dipole moment in liquids. In a microwave oven, this effect is used to heat food. Low-intensity microwave radiation is used in Wi-Fi.
The average microwave oven in active condition is, in close range, powerful enough to cause interference with poorly shielded electromagnetic fields such as those found in mobile medical devices and cheap consumer electronics.
Terahertz radiation
Terahertz radiation is a region of the spectrum between far infrared and microwaves. Until recently, the range was rarely studied and few sources existed for microwave energy at the high end of the band (sub-millimetre waves or so-called terahertz waves), but applications such as imaging and communications are now appearing. Scientists are also looking to apply Terahertz technology in the armed forces, where high frequency waves might be directed at enemy troops to incapacitate their electronic equipment.
Infrared radiation
The infrared part of the electromagnetic spectrum covers the range from roughly 300 GHz (1 mm) to 400 THz (750 nm). It can be divided into three parts:
- Far-infrared, from 300 GHz (1 mm) to 30 THz (10 μm). The lower part of this range may also be called microwaves. This radiation is typically absorbed by so-called rotational modes in gas-phase molecules, by molecular motions in liquids, and by phonons in solids. The water in the Earth's atmosphere absorbs so strongly in this range that it renders the atmosphere effectively opaque. However, there are certain wavelength ranges ("windows") within the opaque range which allow partial transmission, and can be used for astronomy. The wavelength range from approximately 200 μm up to a few mm is often referred to as "sub-millimetre" in astronomy, reserving far infrared for wavelengths below 200 μm.
- Mid-infrared, from 30 to 120 THz (10 to 2.5 μm). Hot objects (black-body radiators) can radiate strongly in this range. It is absorbed by molecular vibrations, that is, when the different atoms in a molecule vibrate around their equilibrium positions. This range is sometimes called the fingerprint region since the mid-infrared absorption spectrum of a compound is very specific for that compound.
- Near-infrared, from 120 to 400 THz (2,500 to 750 nm). Physical processes that are relevant for this range are similar to those for visible light.
Visible radiation (light)
Above infrared in frequency comes visible light. This is the range in which the sun and stars similar to it emit most of their radiation. It is probably not a coincidence that the human eye is sensitive to the wavelengths that the sun emits most strongly. Visible light (and near-infrared light) is typically absorbed and emitted by electrons in molecules and atoms that move from one energy level to another. The light we see with our eyes is really a very small portion of the electromagnetic spectrum. A rainbow shows the optical (visible) part of the electromagnetic spectrum; infrared (if you could see it) would be located just beyond the red side of the rainbow with ultraviolet appearing just beyond the violet end.
Ultraviolet light
Next in frequency comes ultraviolet (UV). This is radiation whose wavelength is shorter than the violet end of the visible spectrum.
Being very energetic, UV can break chemical bonds, making molecules unusually reactive or ionizing them, in general changing their mutual behavior. Sunburn, for example, is caused by the disruptive effects of UV radiation on skin cells, which can even cause skin cancer, if the radiation damages the complex DNA molecules in the cells (UV radiation is a proven mutagen). The Sun emits a large amount of UV radiation, which could quickly turn Earth into a barren desert, but most of it is absorbed by the atmosphere's ozone layer before reaching the surface.
X-rays
After UV come X-rays. Hard X-rays have shorter wavelengths than soft X-rays. X-rays are used for seeing through some things and not others, as well as for high-energy physics and astronomy. Neutron stars and accretion disks around black holes emit X-rays, which enable us to study them.
X-rays will pass through most substances, and this makes them useful in medicine and industry. X-rays are given off by stars, and strongly by some types of nebulae. An X-ray machine works by firing a beam of electrons at a "target". If we fire the electrons with enough energy, X-rays will be produced.
Gamma rays
After hard X-rays come gamma rays. These are the most energetic photons, having no lower limit to their wavelength. They are useful to astronomers in the study of high-energy objects or regions and find a use with physicists thanks to their penetrative ability and their production from radioisotopes. The wavelength of gamma rays can be measured with high accuracy by means of Compton scattering.
Note that there are no defined boundaries between the types of electromagnetic radiation. Some wavelengths have a mixture of the properties of two regions of the spectrum. For example, red light resembles infra-red radiation in that it can resonate some chemical bonds.
See also
External links
- U.S. Frequency Allocation Chart - Covering the range 3 kHz to 300 GHz (from Department of Commerce)
- Canadian Table of Frequency Allocations (from Industry Canada)
- UK frequency allocation table (from Ofcom, which inherited the Radiocommunications Agency's duties, pdf format)
- The Science of Spectroscopy - supported by NASA, includes OpenSpectrum, a Wiki-based learning tool for spectroscopy that anyone can edit
- An EM Spectrum Overview in Flash by e-builds