Wind turbine

A wind turbine, windmill or wind generator is a device for converting wind power to mechanical rotation with a low velocity turbine designed for compressible fluids (air). It is a device for producing renewable energy in the form of electric power and is a component of one of the newest forms of power plant to be put into operation.
For a machine that generates wind, see wind machine.
Location

Wind generators are impractical in many areas as the available power grows as the cube of the average wind speed. A site with prevailing winds of 30 km/h is eight times as valuable as a site with only 15 km/h. As a general rule, wind generators are practical where the average windspeed is greater than 20 km/h. Another lesser known factor is the prevailing temperature - the lower the temperature, the greater the density of the air and thus, the greater the energy contained for the same given windspeed.
The normal way of prospecting for wind-power sites is to look for trees or vegetation that is permanently "cast" or deformed by the prevailing winds. Another way is to use a wind-speed survey map, or historical data from a nearby meteorological station, although this is less reliable.
In typical land-based installations, a tower lifting the bottom of the turbine 30 meters will pay for itself by placing the turbine in faster air.
In areas with dramatic topography, moving a generator 30m can sometimes double its output. Often the winds are monitored and modeled before wind generators are installed.
Wind power is practical in most areas of the North American great plains, and the central Eurasian plains, as well as selected ridges of major mountain-chains. Some authorities claim that the mountain ridges alone have enough wind energy to power their respective continents. In areas with storms, it is often practical to replace or supplement solar cells with a wind-generator. The greatest reservoir of wind energy is in the open oceans, especially around 40 degrees south latitude.

Offshore wind turbines are less unsightly, and can save money by using shorter towers. In stormy areas with extended shallow continental shelves (such as Denmark), they are reasonably easy to install, and give good service - Denmark's offshore wind generation provides about 12-15% of total electricity demand in the country. At the site shown, the wind is not especially strong but is very consistent, giving power more than 97 percent of the time. Denmark is especially a leader in the production and use of turbines, with a commitment made in the 1970s to eventually produce half of the country's power by wind. While the United States government lost interest when the price of oil dropped after the 1970s oil crisis, the Danes continued their efforts and now are a leading exporter of large turbines (each generating 0.66 to 3.0 megawatt).
Wind is powered by a temperature differential. It is slowed by obstructions and is generally stronger at high altitudes. Plains have high winds because they have few obstructions. Mountain passes have high winds mostly because they funnel high-altitude winds. Some passes have winds powered by a temperature differential between the sides of the ridges. Coastal areas have high winds because water has few obstructions and because of the temperature difference between the land and the sea. Off-shore also generally has high winds for the same reasons.

In urban locations, where it is difficult to obtain large amounts of wind energy, smaller systems may still be used to run low power equipment. Distributed power from rooftop mounted wind turbines can also alleviate power distribution problems, as well as provide resilience to power failures. Important equipment such as wireless internet gateways may be powered by a wind turbine that charges a small battery.
The Lakota turbine by AEROMAX is approximately 7 feet in diameter and produces 900 watts of three phase power. It uses a three phase rectifier and charge controller so that it is free to spin at whatever speed is optimal for a given wind condition. Lightweight materials (the entire turbine weighs only 35 pounds) allow it to respond quickly to gusts of wind typical of urban settings. It attaches to a size 9 structural pipe (similar to a TV antenna mast). The Lakota is very quiet. Even when standing up on the roof right next to the mast it is inaudible. Climbing up the mast, it is still inaudible from just a few feet under the turbine. A dynamic braking system regulates the speed by dumping excess energy, so that the turbine continues to produce electricity even in high winds. The dynamic braking resistor may be installed inside the building, to provide useful heating that is proportional to the heat loss (i.e. during high winds when more heat is lost by the building, more heat is also produced by the braking resistor). The proximal location makes low voltage (12 volt, or the like) energy distribution practical, e.g. in a typical installation the braking resistor can be located just inside where the mast is attached to the building. Such small-scale renewable energy sources also impart a beneficial psychological effect on building owners, so that they begin to take on a keen awareness of energy consumption, possibly reducing their consumption down to the average level that the turbine can produce.
Wind turbine design
A wind turbine strongly resembles a propeller, but has subtle differences. The turbine is perpendicular to the wind, mounted on a tower. With small wind generators the tower height is usually at least twenty meters. In the case of large generators, the tower height is about twice as great as the propeller radius.
Power output from a wind generator is proportional to the cube of the wind speed. As wind speed doubles, the capacity of wind generators increases eightfold.
There is usually a means of stalling the turbine's blades to reduce its wind resistance when the wind is extremely strong.
For a given survivable wind speed, the mass of a turbine (calculated from volume) is approximately proportional to the cube of its blade-length. Wind intercepted by the turbine is proportional to the square of its blade-length. The maximum blade-length of a turbine is limited by both the strength and stiffness of its material.
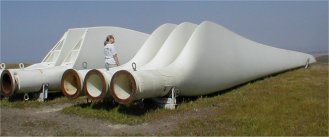
Labor and maintenance costs increase only slowly with increasing turbine size, so given all these factors, to minimize costs, wind farm turbines are basically limited by the strength of materials, and siting. One of the best construction materials available (in 2001) is graphite-fiber in epoxy. Graphite composites enable turbines of sixty meters radius to be built, enough to tap a few megawatts of power. Smaller turbines can be made of lightweight fiberglass, aluminum, or sometimes laminated wood.
Small machines are pointed into the wind by a vane. Large machines have a wind-sensor driving a servomotor.
When it turns to face the wind, the turbine acts like a gyroscope. When the turbine pivots to face the wind, precession tries to twist the turbine into a forward or backward somersault. For each blade on a wind generator's turbine, precessive force is at a minimum when the blade is horizontal and at a maximum when the blade is vertical. This cyclic twisting can quickly fatigue and crack the blade roots, hub and axle of the turbine.
To reduce the precessive stresses, modern turbines have three blades, only one of which is in a maximum stress position (vertical) at a time. The major historic design defect is to have an even number of blades, so that two blades are vertical at the same time. Two-bladed turbines have the highest cyclic stresses.

Home-made wind turbines often have two blades, e.g. something a person can easily carve from one long piece of wood, and use to turn an automobile alternator. Two-bladed turbines thus often avoid the need for using a hub with linkages to individual blades. Many commercially made wind turbines, such as the Whisper 175, still use 2 blades, because such turbines are easy to construct, and the blade(s) can be shipped easily in one long package. Three-bladed turbines, which are much more efficient, and more quiet, must usually be assembled onsite.
When there are four or more blades, the blades of a high-speed, high efficiency turbine start stalling in the disturbed air from the previous blade.
There are a number of vibrations that decrease in peak intensity as the number of blades increases. Some of the vibrations, besides wearing out the machine, are also audible. However, fewer, larger blades operate at a higher Reynolds number and are therefore more efficient. Also, the cost of the turbine increases with the number of blades, so the optimum number of blades turns out to be three.
Since a tower produces turbulence behind it, the turbine is usually placed in front. The turbine has to be placed a considerable distance in front and sometimes tilted up a small amount to ensure that the lower blade doesn't impact the tower. Downwind machines are occasionally built despite the problem of turbulence because they don't need an additional pointing device and in high winds, the blades can be allowed to bend which reduces their wind resistance.
Sails were originally used on early windmills. Unfortunately they have a short service life. Also, they have a relatively high drag for the force they capture. They turn the generator slowly, waste much of the available wind power and have a large wind resistance for their power output, requiring a strong wind tower. For these reasons they were superseded with solid airfoils.
When a turbine is spun by the wind, it adds a rotation to the wind, increasing the apparent wind on the blade. Since blades are really designed to work like an airplane wing, this increases the torque produced by the turbine. But this also increases the force in the wind direction on the blade and therefore on the tower. The mechanical stress is significantly higher when the turbine rotates. That's why wind turbines are stopped during high wind.
Counter rotating turbines can be used to increase the rotation speed of the electrical generator. When the counter rotating turbines are on the same side of the tower, the one in front is angled inwards slightly so as to never hit the rear one. They are either both geared to the same generator or, more often, one is connected to the rotor and the other to the field windings. Counter rotating turbines geared to the same generator have additional gearing losses. Counter rotating turbines connected to the rotor and stator are mechanically simpler; but, the field windings need slip rings which adds complexity, wastes some electricity and wastes some mechanical power.
Counter rotating turbines can be on opposite sides of the tower. In this case it is best that the one at the back be smaller than the one at the front and set to stall at a higher wind speed. This way, at low wind speeds, both turn and the generator taps the maximum proportion of the wind's power. At intermediate speeds, the front turbine stalls; but, the rear one keeps turning, so the wind generator has a smaller wind resistance and the tower can still support the generator. At high wind speeds both turbines stall, the wind resistance is at a minimum and the tower can still support the generator. This allows the generator to function at a wider wind speed range than a single-turbine generator for a given tower.
Putting a turbine at the back helps pull its side downwind. Since the rear turbine will be at a considerable distance behind the front, it provides considerable leverage for a fin placed there, which means no servo is necessary to point the machine into the wind.
To reduce sympathetic vibrations, the two turbines should have an irrational relative rate, (e.g. the square root of two). Overall, this is more complicated than the single-turbine wind generator, but taps more of the wind at a wider range of wind speeds.
Applications
Wind has been used to grind grain, pump water, heat water (with a churn), and produce electricity. In modern times, almost all turbines either pump water or generate electricity.
A wind generator usually consists of an aerodynamic mechanism for converting the movement of air into a mechanical motion which is then converted with a generator into electrical power.
Wind turbines have been used for household electricity generation in conjunction with battery storage over many decades in remote areas. Generator units of more than 1 MWe are now functioning in several countries. The power output is a function of the cube of the wind speed, so such turbines require a wind in the range 3 to 25 m/s (11 - 90 km/h), and in practice relatively few areas have significant prevailing winds. Like solar, wind power requires alternative power sources to cope with calmer periods.
The most economical and practical size of commercial wind turbines seems to be around 600 kWe to 1 MWe, grouped into large wind farms. Most turbines operate at about 25% load factor over the course of a year, but some reach 35%.
Wind is variable, so to provide constant power, wind generators need storage batteries or need to be supplemented by an auxiliary means of electricity generation. In remote areas this source of power is usually photovoltaic or diesel. With a grid connection, the auxiliary power is often from gas turbines or hydropower. Birds can be killed by running into the blades or by being electrocuted by the power lines. At a high extra cost, power lines can be buried to eliminate the danger from electrocution. To reduce bird deaths, wind farms should be out of bird migration routes.
Mechanical wind generators were based on windmills and were popular in the 1900s to the 1940s, before rural electrification programs brought grid connected electricity to the countryside. They started their revival in the 1970s as the price of oil increased. Wind generator cost per unit power has been decreasing by about four percent per year. In the year 2001, they're one of the least expensive forms of energy, costing between two and six cents per kilowatt hour, similar to coal and methane fired plants.
Though wind speed varies, the frequency and voltage output of the generator must remain constant. The two most common ways of doing this are to use an induction generator which turns slightly faster than the utility frequency, usually about five percent faster. It can handle small variations in speed and still provide power at the correct frequency. A more sophisticated way is to use high power electronics to transform current from a generator to utility frequency, this is currently more expensive has a rougher waveform; but, can handle a wider variation in the generator rotation rate. Induction generators work best at one speed while electronic generators can work at a wide speed range; so, to get the advantages of both they could be combined, a low power electronic generator working at all speeds and a high power induction generator cutting in at a medium wind speed. This would overall be able to tap the same wind range as a pure electronic generator; but, have a cost somewhat between an electronic generator and a pure induction generator.
The wind-turbine is the most common type. The main disadvantage is the fact that the entire mechanism has to be able to turn on a tower.
The ducted rotor consists of a propeller and generator inside a duct which flares outwards at the back. The air is accelerated inside the duct and the propeller spins quickly. The main advantage of the ducted rotor is that it can operate in a wide range of winds. Another advantage is that the generator operates at a high rotation rate so it can be smaller. A disadvantage is that it is more complicated than the unducted propeller and the duct is heavy, which puts a greater load on the tower.
The simplest type of wind generator is the savonius rotor. It consists of two vertical curved airfoils mounted between two disks. Whenever wind blows horizontally through this device, the disk turns driving a generator. The main advantage of this type is the device itself doesn't have to be turned into the wind, so no set of slip rings or yaw mechanism is necessary. Also, the heavy generator is on the ground, the airfoils can be made from a pipe section and there is only one moving part. The disadvantages of this type are its low efficiency and the fact that the wind profile can't be reduced in high winds.
Darrieus wind turbines look like a wire supported eggbeater. It consists of thin vertical airfoils which meet at their tips and bend out at the middle. Like the savonius rotor; the main advantage of this type is the device itself doesn't have to be turned into the wind, so no set of slip rings or yaw mecanism is necessary. Also, the heavy generator is on the ground. The disadvantages of this type are the low efficiency, the fact that it needs a starter and the fact that it has huge side loads at the top which usually has to be braced with wires.
Schemes have been bandied about to loft wind generators from kites and / or balloons in order to harness powerful high altitude winds. This has the advantage of being able to tap an almost constant wind and doing so without a set of slip rings or yaw mechanism. The main disadvantage is that kites come down when there is insufficient wind. Balloons can be added to the mix to keep the contraption up without wind; but, balloons leak slowly and have to be at least resupplied with lifting gas, possibly patched as well. Also this scheme requires a very long power cable and an aircraft exclusion zone.
Electrostatic wind generators work by spraying water from a nozzle facing a toroidal charged electrode. This induces an opposite charge in the water and when the water flows out of the nozzle, each drop carries a small amount of charge. These water droplets are them blown by the wind, going through the center of the charged toroid without touching it. The droplets then hit a fine mesh, adding to its charge. The main advantage of this system is that it has no rapidly moving parts. The disadvantages are that it won't work in the rain, it needs a constant supply of water, its wind profile can't be reduced, it requires many small parts, the whole device has to be able to turn and it has to be well crafted to reduce corona discharge losses.
Wind generators range from small four hundred watt generators for residential use to several megawatt machines for wind farms and offshore. The small ones have direct drive generators, direct current output, aeroelastic blades, lifetime bearings and use a fin to point into the wind; while the larger ones generally have geared power trains, alternating current output, flaps and are actively pointed into the wind. As technology progresses, large generators are becoming as simple as small generators. Direct drive generators and aeroelastic blades for large wind turbines are being researched and direct current generators are sometimes used. Wind is an important renewable source of electrical power and the rate of installation is growing by about twenty five percent per year. In combination with solar power, it is an excellent source of electricity.
Utilization
There are now many thousands of wind turbines operating in various parts of the world, with a total capacity of over 39,000 MWe of which Europe accounts for 75% (2003). This has been the most rapidly-growing means of electricity generation at the turn of the century and provides a valuable complement to large-scale base-load power stations. World wind generation capacity quadrupled between 1997 and 2002. 90% of wind power installations are in the US and Europe.
Wind accounts for only 0.4% of the total electricity production on a global scale (2002). Germany is the leading producer of wind power with 38% of the total world capacity in 2003 (5% of German electricity). The United States and Spain are next in terms of installed capacity. Schleswig-Holstein province gets 25% of its power from the wind. Denmark gets over 20% of its electricity from wind, the highest percentage of any country.
History
Windmills were first used to pump water and mill grain. The first wind generators were placed atop brick towers, or other buildings.
By the 1930s they were mainly used to generate electricity on farms. The most famous make was the Jacobs Electric. Jacobs discovered and pioneered the modern three-blade, high-speed wind-turbine, with an integrated, low-speed, ungeared generator. In this period, high tensile steel was cheap, and windmills were placed atop prefabricated open steel lattice towers.
The most famous application of the Jacobs wind-generator was to power the radio for the second polar year expedition to the south pole. Nearly thirty years later, during the IGY, explorers found the Jacobs windmill still turning, despite off-the-scale readings on a maximum-measuring wind-speed meter left as an experiment.
In the 1940s, the U.S. had a rural electrification project that killed the natural market for wind-generated power. The techniques were almost lost.
In the 1970s many people began to desire a self-sufficient life-style. Solar cells were too expensive for small-scale electrical generation, so practical people turned to windmills. At first they built ad-hoc designs using wood and automobile parts. Most people discovered that a reliable wind generator is a moderately complex engineering project, well beyond the ability of most romantics. Practical people began to search for and rebuild farm wind-generators from the 1930s. Jacobs wind generators were especially sought after.
Later, in the 1980s, California provided tax rebates for ecologically harmless power. These rebates funded the first major use of wind power for utility electricity.
As aesthetics and durability became more important, turbines were placed atop steel or reinforced concrete shells. Small generators are connected to the tower on the ground, then the tower is raised into position. Larger generators are hoisted into position atop the tower and there is a ladder or staircase inside the tower to allow technicians to reach and maintain the generator.
Originally wind generators were built right next to where their power was needed. With the availability of long distance electric power transmission, wind generators are now often on wind farms in windy locations and huge ones are being built offshore. Since they're a renewable means of generating electricity, they are being widely deployed, but their cost is often subsidised by governments, either directly or through renewable energy credits. Much depends on the cost of alternative sources of electricity.
Safety precautions and practical issues of running a wind turbine
Windmills create unique hazards. Just like with a fan, don't put your fingers between the blades because you never know when a strong gust of wind might come. Don't climb the mast unless you really know what you're doing.
Old style windmills like the 750 kW Legerwey in Toronto turn at a constant speed, to match line frequency (60 Hz in North America). Newer windmills often turn at whatever speed generates electricity most efficiently. Such newer turbines therefore output at an arbitrary frequency. The AC is then rectified with a three-phase bridge rectifier with six diodes, and the resulting DC is inverted back to AC at exactly line frequency (e.g. 60Hz). This process of converting to DC and then back to AC allows the windmill to turn at any speed. That gives greater efficiency but be forewarned that the blades can spin at any speed, in particular, the blades can spin very fast, suddenly.
Older windmills have heavy steel blades, whereas newer units often use lightweight aerospace materials for low inertia design. In older designs that try to synchronize directly to line frequency, the inertia was advantageous, but in newer designs it's preferable to have low inertia to optimize the energy capture from sudden gusts of wind that are typical in urban settings. Thus urban wind turbines in particular often have low inertia, and can therefore suddenly change speed and direction.
The most modern windmills typically use dynamic braking, rather than mechanical braking, to limit the speed in a storm or similar high-wind condition.
Old style wind turbines have a mechanism to engage the wind less during high winds, or to turn away. Modern designs are less "timid"; they keep producing power no matter how fast the wind blows. Dynamic braking systems use a "dummy load", typically with a PWM (Pulse Width Modulator) control so that when slowing down is desired, the dummy load is connected in varying duty cycles depending on how much slowing is desired. Such brakes kick in when the power produced is greater than the power needed or consumed by the ordinary load.

A typical modern windmill has two brakes:
- (1) a switch that shorts together the three phases to prevent the windmill from turning;
- (2) the dynamic braking system described above.
The brake switch is actually more analogous to the "park" setting of an automobile transmission. Closing the brake switch should only be done when the windmill is already stopped. Otherwise dangerously high currents can result.
Most notable, however, is the fact that perhaps the most dangerous aspect of such windmills is an open circuit. Unlike most other electricity we use (such as that provided by the electric grid), a short circuit in the windmill is OK when, for example, it's "parked".
But the worst thing that can happen is if the wires get disconnected, and there is nothing to slow down the wind turbine. High speed of rotation can result in centrifugal forces that tear the system apart. A runaway windmill would break the sound barrier quite quickly and fortunately the sound might serve as a warning that one must quickly bring it under control.
Ordinarily, though, one prefers to keep a windmill under control at all times, and therefore it is important that the "dummy load" never becomes disconnected. The "dummy load" is usually on the DC side, and therefore the integrity of the six diodes on the bridge rectifier that is typically used must be intact. Regular safety tests should be done with a reduced load to make sure that the dummy load "kicks in".
As a failsafe mechanism, it is also desired to have two sets of wires going to and from the "dummy load".
Also, it should be noted that the braking resistor can get quite hot when the braking takes place, whereas most of the time the braking resistor can be cold. Combustible material should not be piled up on the braking resistor even though it may feel quite cold to the touch, because it may simply be that the wind turbine is not producing more than is being consumed, but that the situation may change at any time and the resistive brakes may kick in without warning.
Braking resistors should be located in areas where there is good airflow. Sometimes brake resistors are mounted out in the open, in the middle of a mechanical room, like a pot-belly stove, so that they also usefully heat the mechanical room in the winter time. Brake resistors are also sometimes used to heat water, to use the "waste energy" to supply hot water to the building. Using the braking resistor to heat a mechanical room can also help to prevent batteries from freezing and bursting in the winter. Many wind turbines charge batteries in addition to being tied to the electric grid. Batteries can be used to provide backup power when the grid goes down. It is important to have good ventilation for the battery, but venting the mechanical room increases the chance of freezing. However, a braking resistor in the mechanical room can help by providing more heat when the wind blows more strongly, which is exactly the time when more heat is needed (because a strong wind is what would otherwise make the mechanical room cool off faster).
Controversy
The debate around wind energy is heated and often emotional. Arguments of both parties are listed below.
Arguments of Opponents

There is resistance to the establishment of windfarms owing initially to perceptions they are noisy and contribute to "visual pollution," i.e., they are considered to be eyesores. The large installations of a modern wind facility are typically 100 m high to the tip of the rotor blade, and, besides the continuous motion of the 35-m-long rotor blades through the air, each time the blade passes the tower a deep subsonic thump is produced whose regular beat many people claim resonates through their homes and even makes them ill.
The large number of turbines required for a viable wind plant, and the huge number of plants required to meet the ambitious goals of the wind industry and governments, ensures that more people will be affected by them.
The construction of a large facility is also far from ecologically benign in previously undeveloped locations. It requires wide straight flat roads, a large hole filled with tons of steel and concrete to secure each giant assembly, clearing of trees in wooded areas, a transformer for each turbine, and power lines.
Siting them offshore can address these objections in some cases, while raising other issues, such as dangers to navigation and the possible adverse effect of low-frequency vibration on ocean mammals.
Another important complaint is that windmills kill too many birds and bats. Siting generally takes into account bird flight patterns, but most paths of migration, particularly for birds that fly by night, are unknown.
Most critics support the goals of renewable energy to reduce reliance on fossil and nuclear fuels, reduce the emission of greenhouse gases and other pollution (such as that causing acid rain), and establish a sustainable source of energy, but they question wind energy's ability to significantly move society towards these goals. They point out that 30% average output is considered high for wind facilities, that besides low output they provide electricity in response to the wind rather than consumer demand, and that this intermittency ensures that no "conventional" power plants can be shut down, particularly less efficient plants that are able to switch on and off in a matter of seconds. Another charge is that output figures, such as "Denmark produces over 20% of its electricity from wind," do not account for the electricity used by the plants themselves or electricity that is simply absorbed by the international grid because it is produced when demand is already being met by other sources. It is also noted that because electricity production uses only part (about a third) of society's energy, wind power does nothing to mitigate the effects of most of our energy use. For example, despite aggressive installation of wind facilities in the U.K., that country's CO2 emissions continued to rise in 2002 and 2003.
It is often pointed out, e.g., by the UN's Intergovernmental Panel on Climate Change, that continued improvements in efficiency -- in building, manufacturing, and transport -- will achieve the desired mitigation goals to a much greater degree and at much less cost than wind power can.
Arguments of Supporters
Supporters of wind energy state that:
- Land based wind energy has the potential of covering six times the world's electricity consumption, or one time the world's total energy consumption.
- The energy consumption for production, installation, operation and decommission of a wind turbine is usually earned back within 3 months of operation.
- Conventional and nuclear electricity production receive massive amounts of direct and indirect subsidies. If a comparison is made on real production costs, wind energy is competitive in many cases. If the so-called external costs are taken into account, wind energy is competitive in most cases. Furthermore, wind energy costs are continuously decreasing due to technology development and scale enlargement. On the other hand, the hidden costs of decommissioning nuclear power stations, and waste disposal are now coming to the fore.
- Studies show that the number of birds and bats killed by wind turbines is negligible compared to what's due to other human activities such as traffic, hunting, power lines and high-rise buildings. For example, in the UK with a few hundred turbines, about one bird is killed per turbine per year; 10 million per year are killed by cars alone.
- After decommissioning wind turbines, even the foundations get to be removed.
- Conventional and nuclear plants also have sudden unpredictable outages. Statistical analysis shows that 1000 MW of wind power can replace 300 MW of conventional power.
- Existing European hydropowerplants have the capacity to store enough energy to supply one month's worth of European energy consumption. Improvement of the international grid would allow to use this at relatively short term at low cost. Furthermore, geographically spread wind turbine parks used together produce power much more constantly. On the longer term, the electricity can be used to produce hydrogen. This can be used with fuel cells to produce electricity at times of low wind supply and as fuel for transport.
- Improvement in energy efficiency should go hand in hand with the use of renewable energy.
- Wind turbines are beautiful, graceful machines that symbolise humans in harmony with the natural world.
- More recent wind farms have their turbines spaced further apart, due to the higher capacity of the individual wind turbines. They no longer have the cluttered look of the early wind farms.
- It is possible to hold a conversation directly underneath a modern wind turbine without any difficulty whatever and without raising one's voice. The modern turbine is quieter than its predecessors owing to improvements in the blade design. It makes a gentle "swish swish swish" sound that is quite pleasant and soothing. In addition, when it is windy the background noise of rustling trees, etc., exceeds the turbine noise.
- Wind turbines have epistemological value, as well as artistic value. As a form of sculpture, wind turbines are a dynamic (moving) art form. As an epistemological sculpture, they also make visible the process of producing electricity. There is an obvious beauty to seeing the process and understanding how it works. Also, by coincidence, the most efficient number of blades is three, and that lines up with the 120 degree tangential symmetry of three phase power. Therefore, there is a poetic narrative that makes a good conversation piece. Urban wind turbines like the 750kW Lagerwey in Toronto are popular gathering places where people come to sit and contemplate, and enjoy the restful beauty of a sculpture in motion, or to bring their children to what essentially amounts to a good outdoor exhibit that's open 24 hours a day.
Companies in wind turbine industry
External links
- Alliance to Protect Nantucket Sound Citizens group opposed to plans for USA's first offshore wind facility.
- Wind and Hydro Power, turbine basics+principle of operation
- British Windpower Association
- American Windpower Association
- Danish Windpower Association
- Windpower Monthly -- Wind Energy Magazine
- Air 403 400W Wind Generator
- XL1 1kW Wind Generator
- Residential Wind Power Q&A
- Links to articles about windpower
- Discussion forum for Wind and other Renewable Energy sources
- Average annual wind power map for the U.S.
- Country Guardian -- A UK NGO opposed to the construction of wind turbines.
- yes2wind -- A website by Greenpeace, Friends of the Earth and the World Nature Fund in support of wind energy
- Wind Works --An extensive personal site on windpower. Nuanced pro wind energy.
- wind-farm -- A website for news, articles, and discussion in opposition to industrial-scale wind facilities
- ExternE -- The European research project about the external costs of energy production
- Personal site on the Altamount Pass Wind Farm in California, with 40+ photos
- OtherPower.com - How to build a wind turbine from scratch (including the blade). Includes several designs.
- Windformer - Students Project with information about wind turbines