Wind turbine: Difference between revisions
Sillybilly (talk | contribs) revert |
|||
Line 52: | Line 52: | ||
[[Image:Darrieus-windmill.jpg|thumb|right|30 m [[Darrieus wind turbine]] in the [[Magdalen Islands]]]] |
[[Image:Darrieus-windmill.jpg|thumb|right|30 m [[Darrieus wind turbine]] in the [[Magdalen Islands]]]] |
||
Vertical Axis Wind Turbines (or VAWTs) have the main rotor shaft running vertically. The advantages of this arrangement are that the generator and/or gearbox can be placed at the bottom, near the ground, so the tower doesn't need to support it, and that the turbine doesn't need to be pointed into the wind. Drawbacks are usually the pulsating torque produced during each revolution, and the difficulty of mounting vertical axis turbines on towers, meaning they must operate in the slower, more turbulent air flow near the ground, with lower energy extraction efficiency. |
Vertical Axis Wind Turbines (or VAWTs) have the main rotor shaft running vertically. The advantages of this arrangement are that the generator and/or gearbox can be placed at the bottom, near the ground, so the tower doesn't need to support it, and that the turbine doesn't need to be pointed into the wind.very handy. Drawbacks are usually the pulsating torque produced during each revolution, and the difficulty of mounting vertical axis turbines on towers, meaning they must operate in the slower, more turbulent air flow near the ground, with lower energy extraction efficiency. |
||
[[Image:H-Darrieus-Rotor.png.jpg|thumb|right|H-Darrieus-turbine]] |
[[Image:H-Darrieus-Rotor.png.jpg|thumb|right|H-Darrieus-turbine]] |
Revision as of 14:48, 15 November 2006


A wind turbine is a machine for converting the kinetic energy in wind into mechanical energy. If the mechanical energy is used directly by machinery, such as a pump or grinding stones, the machine is usually called a windmill. If the mechanical energy is then converted to electricity, the machine is called a wind generator.
This article discusses the conversion machinery. See the broader article on wind power for more on turbine placement and controversy, and in particular see the wind energy section of that article for an understanding of the temporal distribution of wind energy and how that affects wind turbine design.
For a machine that generates wind, see wind machine. For an unusual way to induce a voltage using an aerosol of ionised water, see vaneless ion wind generator.
Types of wind turbines

Wind turbines can be separated into two general types based on the axis about which the turbine rotates. Turbines that rotate around a horizontal axis are most common. Vertical axis turbines are less frequently used.
Wind turbines can also be classified by the location in which they are to be used. Onshore, offshore, or even aerial wind turbines have unique design characteristics which are explained in more detail in the section on Turbine design and construction.
Wind turbines may also be used in conjunction with a solar collector to extract the energy due to air heated by the Sun and rising through a large vertical Solar updraft tower.
Horizontal axis
Horizontal Axis Wind Turbines (HAWT) have the main rotor shaft and generator at the top of a tower, and must be pointed into the wind by some means. Small turbines are pointed by a simple wind vane, while large turbines generally use a wind sensor coupled with a servomotor. Most have a gearbox too, which turns the slow rotation of the blades into a quicker rotation that is more suitable for generating electricity.
Since a tower produces turbulence behind it, the turbine is usually pointed upwind of the tower. Turbine blades are made stiff to prevent the blades from being pushed into the tower by high winds. Additionally, the blades are placed a considerable distance in front of the tower and are sometimes tilted up a small amount.
Downwind machines have been built, despite the problem of turbulence, because they don't need an additional mechanism for keeping them in line with the wind, and because in high winds, the blades can be allowed to bend which reduces their swept area and thus their wind resistance. Because turbulence leads to fatigue failures and reliability is so important, most HAWTs are upwind machines.
There are several types of HAWT:

- Windmills
- These four- (or more) bladed squat structures, usually with wooden shutters or fabric sails, were pointed into the wind manually or via a tail-fan. These windmills, generally associated with the Netherlands, were historically used to grind grain or pump water from low-lying land. They greatly accelerated shipbuilding in the Netherlands, and were instrumental in keeping its polders dry.
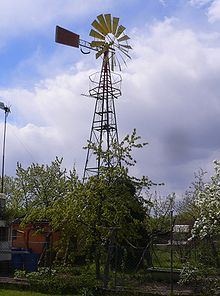
- American-style farm windmills
- These windmills were used by American prairie farmers to generate electricity and to pump water. They typically had many blades, operated at tip speed ratios (defined below) not better than one, and had good starting torque. Some had small direct-current generators used to charge storage batteries, to provide a few lights, or to operate a radio receiver. The rural electrification connected many farms to centrally-generated power and replaced individual windmills as a primary source of farm power in the 1950s. Such devices are still used in locations where it is too costly to bring in commercial power.

- Common modern wind turbines
- Usually three-bladed, sometimes two-bladed or even one-bladed (and counterbalanced), and pointed into the wind by computer-controlled motors. The rugged three-bladed turbine type has been championed by Danish turbine manufacturers. These have high tip speeds of up to 6x wind speed, high efficiency, and low torque ripple which contributes to good reliability. This is the type of turbine that is used commercially to produce electricity. They are usually white in color.
- Ducted rotor
- Still something of a research project, the ducted rotor consists of a turbine inside a duct which flares outwards at the back. They are also referred as Diffuser-Augmented Wind Turbines (i.e. DAWT). The main advantage of the ducted rotor is that it can operate in a wide range of winds and generate a higher power per unit of rotor area. Another advantage is that the generator operates at a high rotation rate, so it doesn't require a bulky gearbox, so the mechanical portion can be smaller and lighter. A disadvantage is that (apart from the gearbox) it is more complicated than the unducted rotor and the duct is usually quite heavy, which puts an added load on the tower.
Co-axial, multi-rotor horizontal axis turbines
Two or more rotors may be mounted to the same driveshaft, with their combined co-rotation together turning the same generator — fresh wind is brought to each rotor by sufficient spacing between rotors combined with an offset angle alpha from the wind direction. Wake vorticity is recovered as the top of a wake hits the bottom of the next rotor. http://www.multirotor.com Power has been multiplied several times using co-axial, multiple rotors in testing conducted by inventor and researcher Douglas Selsam, for the California Energy Commission in 2004. The first commercially available co-axial multi-rotor turbine is the patented dual-rotor American Twin Superturbine from Selsam Innovations in California, with 2 propellers separated by 12 feet. It is the most powerful 7-foot diameter turbine available, due to this extra rotor. http://www.selsam.com
Counter-rotating horizontal axis turbines
Counter rotating turbines can be used to increase the rotation speed of the electrical generator. As of 2005, no large practical counter-rotating HAWTs are commercially sold. When the counter rotating turbines are on the same side of the tower, the blades in front are angled forwards slightly so as to avoid hitting the rear ones. If the turbine blades are on opposite sides of the tower, it is best that the blades at the back be smaller than the blades at the front and set to stall at a higher wind speed. This allows the generator to function at a wider wind speed range than a single-turbine generator for a given tower. To reduce sympathetic vibrations, the two turbines should turn at speeds with few common multiples, for example 7:3 speed ratio. Overall, this is a more complicated design than the single-turbine wind generator, but it taps more of the wind's energy at a wider range of wind speeds.
Appa designed and demonstrated a contra rotor wind turbine in FY 2000–2002 funded by California Energy Commission. This study showed 30 to 40% more power extraction than a comparable single rotor system. Further it was observed that the slower the rotor speed better the performance. Consequently Megawatt machines benefit most.
Cyclic stresses and vibration
Cyclic stresses fatigue the blade, axle and bearing material, and were a major cause of turbine failure for many years. Because wind velocity increases at higher altitudes, the backward force and torque on a horizontal axis wind turbine (HAWT) blade peaks as it turns through the highest point in its circle. The tower hinders the airflow at the lowest point in the circle, which produces a local dip in force and torque. These effects produce a cyclic twist on the main bearings of a HAWT. The combined twist is worst in machines with an even number of blades, where one is straight up when another is straight down. To improve reliability, teetering hubs have been used which allow the main shaft to rock through a few degrees, so that the main bearings do not have to resist the torque peaks.
When the turbine turns to face the wind, the rotating blades act like a gyroscope. As it pivots, gyroscopic precession tries to twist the turbine into a forward or backward somersault. For each blade on a wind generator's turbine, precessive force is at a minimum when the blade is horizontal and at a maximum when the blade is vertical. This cyclic twisting can quickly fatigue and crack the blade roots, hub and axle of the turbine.
Vertical axis

Vertical Axis Wind Turbines (or VAWTs) have the main rotor shaft running vertically. The advantages of this arrangement are that the generator and/or gearbox can be placed at the bottom, near the ground, so the tower doesn't need to support it, and that the turbine doesn't need to be pointed into the wind.very handy. Drawbacks are usually the pulsating torque produced during each revolution, and the difficulty of mounting vertical axis turbines on towers, meaning they must operate in the slower, more turbulent air flow near the ground, with lower energy extraction efficiency.

- Darrieus wind turbine
- These are the "eggbeater" turbines. They have good efficiency, but produce large torque ripple and cyclic stress on the tower, which contributes to poor reliability. Also, they generally require some external power source, or an additional Savonius rotor, to start turning, because the starting torque is very low. The torque ripple is reduced by using 3 or more blades.
- Giromill is a type of Darrieus
- These lift-type devices have vertical blades. The cycloturbine variety has variable pitch, to reduce the torque pulsation and self-start [1]. The advantages of variable pitch are high starting torque, a wide, relatively flat torque curve, a lower blade speed ratio, a higher coefficient of performance, more efficient operation in turbulent winds, and a lower blade speed ratio which lowers blade bending stresses. Straight, V, or curved blades may be used.
- Savonius wind turbine
- These are the familiar two- (or more) scoop drag-type devices used in anemometers and in the Flettner vents commonly seen on bus and van roofs, and some high-reliability low-efficiency power turbines. They always self-start (if at least three scoops). They can sometimes have long helical scoops, to give smooth torque. The Banesh rotor and especially the Rahai rotor improve efficiency by shaping the blades to produce significant lift as well as drag.
- Others
- TMA, Inc. (Terra Moya Aqua) has developed a vertical axis wind turbine design using a combination of fixed and rotating vanes. They report efficiency similar to other wind turbine designs, but with less vertical height and visual impact. This design also reduces bird injury because birds avoid the fixed vanes. This design has not yet entered commercial production.
Offshore

Offshore wind turbines are considered to be less obtrusive than turbines on land, as their apparent size and noise can be mitigated by distance. Because water has less surface roughness than land, the average wind speed is usually higher over open water. This allows offshore turbines to use shorter towers, making them less visible. In stormy areas with extended shallow continental shelves (such as Denmark), turbines are practical to install, and give good service — Denmark's wind generation provides about 25-30% of total electricity demand in the country, with many offshore windfarms. Denmark plans to increase wind energy's contribution to as much as half of its electrical supply.
The offshore environment is, however, more expensive. Offshore towers are generally taller than onshore towers once one includes the submerged height, and offshore foundations are generally more difficult to build and more expensive as well. Power transmission from offshore turbines is generally through undersea cable, which is more expensive to install than cables on land, and may use high voltage direct current operation if significant distance is to be covered — which then requires yet more equipment. The offshore environment is also corrosive and abrasive. Repairs and maintenance are much more difficult, and much more costly than on onshore turbines. Offshore wind turbines are outfitted with extensive corrosion protection measures like coatings and cathodic protection.
While there is a significant market for small land-based windmills, offshore wind turbines have recently been and will probably continue to be the largest wind turbines in operation, because larger turbines reduce the marginal cost of many of the difficulties of offshore operation.
There are some conceptual designs that might make use of the unique offshore environment. For example, a floating turbine might orient itself downwind of its anchor, and thus avoid the need for a yawing mechanism. One concept for offshore turbines has them generate rain, instead of electricity. The turbines would create a fine aerosol, which is envisioned to increase evaporation and induce rainfall, hopefully on land [2].
Aerial
Main article: Airborne wind turbine
It has been suggested that wind turbines could be flown in high speed winds at high altitude taking advantage of the steadier winds at high altitudes. No such systems currently exist in the marketplace. The idea of airborne wind turbines reappears in the industry every few years, and seldom (if ever) gets off the drawing board.
Turbine design and construction
Horizontal Axis Wind Turbine Aerodynamics
The aerodynamics of a horizontal axis wind turbine are not straight forward. The air flow at the blades is not the same as the airflow far away from the turbine. The very nature of the way in which energy is extracted from the air also causes air to be deflected by the turbine. In addition the aerodynamics of a wind turbine at the rotor surface exhibit phenomena that are rarely seen in other aerodynamic fields.
Axial Momentum and the Betz Limit
Energy in fluid is contained in four different forms: gravitational potential energy, thermodynamic pressure, kinetic energy from the velocity and finally thermal energy. Gravitational and thermal energy have a negligible effect on the energy extraction process. From a macroscopic point of view, the air flow about the wind turbine is at atmospheric pressure. If pressure is constant then only kinetic energy is extracted. However up close near the rotor itself the air velocity is constant as it passes through the rotor plane. This is because of conservation of mass. The air that passes through the rotor cannot slow down because it needs to stay out of the way of the air behind it. So at the rotor the energy is extracted by a pressure drop. The air directly behind the wind turbine is at sub-atmospheric pressure; the air in front is under greater than atmospheric pressure. It is this high pressure in front of the wind turbine that deflects some of the upstream air around the turbine.
Albert Betz was amongst the first to study this phenomenon. He notably determined the maximum limit to wind turbine performance. The limit is now referred to as the Betz Limit. This is derived by looking at the axial momentum of the air passing through the wind turbine. As stated above some of the air is deflected away from the turbine. This causes the air passing through the rotor plane to have a smaller velocity than the free stream velocity. The degree at which air at the turbine is less than the air far away from the turbine is called the axial induction factor. It is defined as below.
a is the axial induction factor. U1 is the wind speed far away from the rotor. U2 is the wind speed at the rotor.
The first step to deriving the Betz limit is applying conservation of axial momentum. As stated above the wind loses speed after the wind turbine compared to the speed far away from the turbine. This would violate the conservation of momentum if the wind turbine was not applying a thrust force on the flow. This thrust force manifests itself through the pressure drop across the rotor. The front operates at high pressure while the back operates at low pressure. The pressure difference from the front to back causes the thrust force. The momentum lost in the turbine is balanced by the thrust force. Another equation is needed to relate the pressure difference to the velocity of the flow near the turbine. Here the Bernoulli equation is used between the field flow and the flow near the wind turbine. There is one limitation to the Bernoulli equation. The equation cannot be applied to fluid passing through the wind turbine. Instead conservation of mass is used to relate the incoming air to the outlet air. Betz used these equations and managed to solve the velocities of the flow in the far wake and near the wind turbine in terms of the far field flow and the axial induction factor. The velocities are given below.
U4 is introduced here as the wind velocity in the far wake. This is important because the power extracted from the turbine is defined by the following equation. However the Betz limit is given in terms of the coefficient of power. The coefficient of power is similar to efficiency but not the same. The formula for the coefficient of power is given beneath the formula for power.
Betz was able to develop an expression for Cp in terms of the induction factors. This is done by the velocity relations being substituted into power and power is substituted into the coefficient of power definition. The relationship Betz developed is given below.
The Betz limit is defined by the maximum value that can be given by the above formula. This is found by taking the derivative with respect to the axial induction factor, setting it to zero and solving for the axial induction factor. Betz was able to show that the optimum axial induction factor is one third. The optimum axial induction factor was then used to find the maximum coefficient of power. This maximum coefficient is the Betz limit. Betz was able to show that the maximum coefficient of power of a wind turbine is 16/27. Airflow operating at higher thrust will cause the axial induction factor to rise above the optimum value. Higher thrust cause more air to be deflected away from the turbine. When the axial induction factor falls below the optimum value the wind turbine is not extracting all the energy it can. This reduces pressure around the turbine and allows more air to pass through the turbine, but not enough to account for lack of energy being extracted.
The derivation of the Betz limit shows a simple analysis of wind turbine aerodynamics. In reality there is a lot more. A more rigorous analysis would include wake rotation, the effect of variable geometry. The effect of air foils on the flow is a major component of wind turbine aerodynamics. Within airfoils alone the wind turbine aerodynamicist has to consider the effect of surface roughness, dynamic stall tip losses, solidity, among other problems.
Angular Momentum and Wake Rotation
The wind turbine described by Betz does not actually exist. It is merely an idealized wind turbine described as an actuator disk. Its a disk in space where fluid energy is simply extracted from the air. In the Betz turbine the energy extraction manifests itself through thrust. The equivalent turbine described by Betz would be a horizontal propeller type operating with infinite blades at infinite tip speed ratios and no losses. The tip speed ratio is ratio of the speed of the tip relative to the free stream flow. This turbine is not too far from actual wind turbines. Actual turbines are rotating blades. They typically operate at high tip speed ratios. At high tip speed ratios three blades are sufficient to interact with all the air passing through the rotor plane. Actual turbines still produce considerable thrust forces.
One key difference between actual turbines and the actuator disk, is that the energy is extracted through torque. The wind imparts a torque on the wind turbine, thrust is a necessary by product of torque. Newtonian physics dictates that for every action there is an equal and opposite reaction. If the wind imparts a torque on the blades then the blades must be imparting a torque on the wind. This torque would then cause the flow to rotate. Thus the flow in the wake has two components, axial and tangential. This tangential flow is referred to as wake rotation.
Torque is necessary for energy extraction. However wake rotation is considered a loss. Accelerating the flow in the tangential direction increases the absolute velocity. This in turn increases the amount of kinetic energy in the near wake. This rotational energy is not dissipated in any form that would allow for a greater pressure drop (Energy extraction). Thus any rotational energy in the wake is energy that is lost and unavailable.
This loss is minimized by allowing the rotor to rotate very quickly. To the observer it may seem like the rotor is not moving fast, however it is common for the tips to be moving through the air at 6 times the speed of the free stream. Newtonian mechanics defines power as torque multiplied by the rotational speed. The same amount of power can be extracted by allowing the rotor to rotate faster and produce less torque. Less torque means that there is less wake rotation. Less wake rotation means there is more energy available to extract.
Tower height
The wind blows faster at higher altitudes because of the drag of the surface (sea or land) and the viscosity of the air. The variation in velocity with altitude, called wind shear, is most dramatic near the surface.

Typically, in daytime the variation follows the 1/7th power law, which predicts that wind speed rises proportionally to the seventh root of altitude. Doubling the altitude of a turbine, then, increases the expected wind speeds by 10% and the expected power by 34%. Doubling the tower height generally requires doubling the diameter as well, increasing the amount of material by a factor of eight.
In night time, or better: when the atmosphere becomes stable, wind speed close to the ground usually subsides whereas at turbine hub altitude it does not decrease that much or may even increase. As a result the wind speed is higher and a turbine will produce more power than expected from the 1/7th power law: doubling the altitude may increase wind speed by 20% to 60%. A stable atmosphere is caused by radiative cooling of the surface and is common in a temperate climate: it usually occurs when there is a (partly) clear sky at night. When the (high altitude) wind is strong (10 meter (33 ft) wind speed higher than approximately 6 to 7 m/s (20-23 ft/s)) the stable atmosphere is disrupted because of friction turbulence and the atmosphere will turn neutral. A daytime atmosphere is either neutral (no net radiation; usually with strong winds and/or heavy clouding) or unstable (rising air because of ground heating — by the sun). Here again the 1/7th power law applies or is at least a good approximation of the wind profile.
For HAWTs, tower heights approximately twice to triple the blade length have been found to balance material costs of the tower against better utilisation of the more expensive active components.
Blade count

The determination of the number of blades involves design considerations of aerodynamic efficiency, component costs, system reliability, and aesthetics. Noise emissions are primarily affected by the location of the blades upwind or downwind of the tower and not significantly by the blade count. Wind turbines developed over the last 50 years have almost universally used either two or three blades. Aerodynamic efficiency increases with number of blades but with diminishing return. Increasing the number of blades from one to two yields a six percent increase in aerodynamic efficiency. Whereas increasing the blade count from two to three yields only an additional three percent in efficiency. Further increasing the blade count yields minimal improvements in aerodynamic efficiency and sacrifices too much in blade stiffness as the blades become thinner.
Component costs that are affected by blade count are primarily for materials and manufacturing of the turbine rotor and drive train. Generally, the fewer the number of blades, the lower the material and manufacturing costs will be. In addition, the fewer the number of blades, the higher the rotational speed will be. This is because blade stiffness requirements to avoid interference with the tower limit how thin the blades can be. Fewer blades with higher rotational speeds reduce peak torques in the drive train, resulting in lower gearbox and generator costs.
System reliability is affected by blade count primarily through the dynamic loading of the rotor into the drive train and tower systems. While aligning the wind turbine to changes in wind direction (yawing), each blade experiences a cyclic load at its root end depending on blade position. This is true of one, two, three blades or more. However, these cyclic loads when combined together at the drive train shaft are symmetrically balanced for three blades, yielding smoother operation during turbine yaw. Turbines with one or two blades can use a pivoting teetered hub to also nearly eliminate the cyclic loads into the drive shaft and system during yawing.
Finally, aesthetics can be considered a factor in that some people find that the three-bladed rotor is more pleasing to look at than a one- or two-bladed rotor.
Rotation control
- Tip speed ratio
- The ratio between the speed of the wind and the speed of the tips of the blades of a wind turbine. High efficiency 3-blade-turbines have tip speed/wind speed ratios of 6 to 7.
Modern wind turbines are designed to spin at varying speeds (a consequence of their generator design, see below). Use of aluminum and composites in their blades has contributed to low rotational inertia, which means that newer wind turbines can accelerate quickly if the winds pick up, keeping the tip speed ratio more nearly constant. Operating closer to their optimal tip speed ratio during energetic gusts of wind allows wind turbines to improve energy capture from sudden gusts that are typical in urban settings.
In contrast, older style wind turbines were designed with heavier steel blades, which have higher inertia, and rotated at speeds governed by the AC frequency of the power lines. The high inertia buffered the changes in rotation speed and thus made power output more stable.
The speed and torque at which a wind turbine rotates must be controlled for several reasons:
- To optimize the aerodynamic efficiency of the rotor in light winds.
- To keep the generator within its speed and torque limits.
- To keep the rotor and hub within their centripetal force limits. The centripetal force from the spinning rotors increases as the square of the rotation speed, which makes this structure sensitive to overspeed.
- To keep the rotor and tower within their strength limits. Because the power of the wind increases as the cube of the wind speed, turbines have to be built to survive much higher wind loads (such as gusts of wind) than those from which they can practically generate power. Since the blades generate more downwind force (and thus put far greater stress on the tower) when they are producing torque, most wind turbines have ways of reducing torque in high winds.
- To enable maintenance; because it is dangerous to have people working on a wind turbine while it is active, it is sometimes necessary to bring a turbine to a full stop.
- To reduce noise; As a rule of thumb, the noise from a wind turbine increases with the fifth power of the relative wind speed (as seen from the moving tip of the blades). In noise-sensitive environments, the tip speed can be limited to approximately 60 m/s (200 ft/s).
Overspeed control is exerted in two main ways: aerodynamic stalling or furling, and mechanical braking. Furling is the preferred method of slowing wind turbines.
Stalling and furling
Stalling works by increasing the angle at which the relative wind strikes the blades (angle of attack), and it reduces the induced drag (drag associated with lift). Stalling is simple because it can be made to happen passively (it increases automatically when the winds speed up), but it increases the cross-section of the blade face-on to the wind, and thus the ordinary drag. A fully stalled turbine blade, when stopped, has the flat side of the blade facing directly into the wind.
Furling works by decreasing the angle of attack, which reduces the induced drag from the lift of the rotor, as well as the cross-section. One major problem in designing wind turbines is getting the blades to stall or furl quickly enough should a gust of wind cause sudden acceleration. A fully furled turbine blade, when stopped, has the edge of the blade facing into the wind.
A fixed-speed HAWT inherently increases its angle of attack at higher wind speed as the blades speed up. A natural strategy, then, is to allow the blade to stall when the wind speed increases. This technique was used on many early HAWTs, until it was realised that stalled blades generate a large amount of vibration (noise). Standard modern turbines all furl the blades in high winds. Since furling requires acting against the torque on the blade, it requires active pitch angle control which is only cost-effective on very large turbines. Many turbines use hydraulic systems. These systems are usually spring loaded, so that if hydraulic power fails, the blades automatically furl. Other turbines use an electric servomotor for every rotor blade. They have a small battery-reserve in case of an electric-grid breakdown.
The variable wind speed wind turbine uses furling as its main method of rotation control. The wind turbines have three modes of operation *Below rated wind speed operation *Around rated wind speed operation *Above rated wind speed operation
At above rated wind speed the rotors furl at an angle to maintain the torque. This is also known as feathering
Electrical braking

Braking of a turbine can also be done by dumping energy from the generator into a resistor bank, converting the kinetic energy of the turbine rotation into heat. This method is useful if the kinetic load on the generator is suddenly reduced or is too small to keep the turbine speed within its allowed limit.
Cyclically braking causes the blades to slow down, which increases the stalling effect, reducing the efficiency of the blades. This way, the turbine's rotation can be kept at a safe speed in faster winds while maintaining (nominal) power output.
Mechanical braking
A mechanical drum brake or disk brake is used to hold the turbine at rest for maintenance. Such brakes are usually applied only after blade furling and electromagnetic braking have reduced the turbine speed, as the mechanical brakes would wear quickly if used to stop the turbine from full speed. There can also be a stick brake.
Turbine size
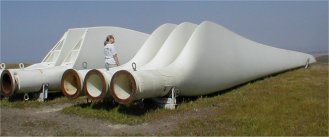
For a given survivable wind speed, the mass of a turbine is approximately proportional to the cube of its blade-length. Wind power intercepted by the turbine is proportional to the square of its blade-length. The maximum blade-length of a turbine is limited by both the strength and stiffness of its material.
Labor and maintenance costs increase only gradually with increasing turbine size, so to minimize costs, wind farm turbines are basically limited by the strength of materials, and siting requirements.
Typical modern wind turbines have diameters of 40 to 90 meters (130-300 ft) and are rated between 500 kW and 2 megawatts (670-2,700 hp). Currently (2005) the most powerful turbine is rated at 6 MW (8,000 hp).
Generating electricity

For large, commercial size horizontal-axis wind turbines, the generator is mounted in a nacelle at the top of a tower, behind the hub of the turbine rotor. A speed increasing gearbox may be inserted between the rotor hub and the generator, so that the generator cost and weight can be reduced.
Commercial size generators have a rotor carrying a field winding so that a rotating magnetic field is produced inside a set of windings called the stator. While the rotating field winding consumes a fraction of a percent of the generator output, adjustment of the field current allows good control over the generator output voltage. Very small wind generators (a few watts to perhaps a kilowatt in output) may use permanent magnets but these are too costly to use in large machines and do not allow convenient regulation of the generator voltage.
Electrical generators inherently produce AC power. Older style wind generators rotate at a constant speed, to match power line frequency, which allowed the use of less costly induction generators. Newer wind turbines often turn at whatever speed generates electricity most efficiently. The variable frequency current is then converted to DC and then back to AC, matching the line frequency and voltage. Although the two conversions require costly equipment and cause power loss, the turbine can capture a significantly larger fraction of the wind energy. In some cases, especially when turbines are sited offshore, the DC energy will be transmitted from the turbine to a central (onshore) inverter for connection to the grid.
Materials
One of the strongest construction materials available (in 2006) is graphite-fibre in epoxy, but it is very expensive and only used by some manufactures for special load-bearing parts of the rotor blades. Modern rotor blades (up to 126 m (413 ft) diameter) are made of lightweight pultruded glass-reinforced plastic (GRP), smaller ones also from aluminium. GRP is the most common material for modern wind turbines. Wind turbines are made from a variety of materials. Usually consisting of some base wooden form, the molds of the turbines can include composite materials of fiberglass, carbon, and varying mixtures of epoxy for reinforcement on the critical areas of the blade. Balsam wood is usually preferred to due to its lightweight properties.
Wood and canvas sails were originally used on early windmills. Unfortunately they require much maintenance over their service life. Also, they have a relatively high drag (low aerodynamic efficiency) for the force they capture. For these reasons they were superseded with solid airfoils.
Special windturbines
One wind turbine of the type E-66 at Windpark Holtriem, Germany carries an observation deck, open for visitors.
Another Enercon E-66 wind turbine with an observation deck is in the English town of Swaffham.
History
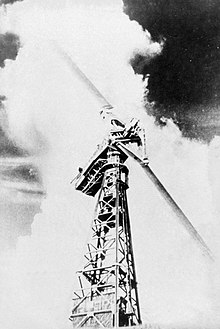
Wind machines were used for grinding grain in Persia as early as 200 B.C. This type of machine was introduced into the Roman Empire by 250 A.D. By the 14th century Dutch windmills were in use to drain areas of the Rhine River delta. In Denmark by 1900 there were about 2500 windmills for mechanical loads such as pumps and mills, producing an estimated combined peak power of about 30 MW. The first windmill for electricity production was built in Cleveland, Ohio by Charles F. Brush in 1888, and in 1908 there were 72 wind-driven electric generators from 5 kW to 25 kW (7-34 hp). The largest machines were on 24 m (79 ft) towers with four-bladed 23 m (75 ft) diameter rotors.
By the 1930s windmills were mainly used to generate electricity on farms, mostly in the United States where distribution systems had not yet been installed. In this period, high tensile steel was cheap, and windmills were placed atop prefabricated open steel lattice towers. A forerunner of modern horizontal-axis wind generators was in service at Yalta, USSR in 1931. This was a 100 kW (130 hp) generator on a 30 m (100 ft) tower, connected to the local 6.3 kV distribution system. It was reported to have an annual load factor of 32 per cent, not much different from current wind machines.
In 1941 the world's first megawatt-size wind turbine was connected to the local electrical distribution system on Grandpa's Knob in Castleton, Vermont, USA. This 1.25 MW (1,680 hp) Smith-Putnam turbine operated for 1100 hours before a blade failed at a known weak point, which had not been reinforced due to war-time material shortages. In the 1940s, the U.S. had a rural electrification project that killed the natural market for wind-generated power, since network power distribution provided a farm with more dependable usable energy for a given amount of capital investment.
In the 1970s many people began to desire a self-sufficient life-style. Solar cells were too expensive for small-scale electrical generation, so some turned to windmills. At first they built ad-hoc designs using wood and automobile parts. Most people discovered that a reliable wind generator is a moderately complex engineering project, well beyond the ability of most romantics. Some began to search for and rebuild farm wind-generators from the 1930s, of which Jacobs wind generators were especially sought after.

From the mid 1970's through the mid 1980's the United States government worked with industry to advance the technology and enable large commercial wind turbines. This effort was led by the National Aeronautics and Space Administration (NASA) in Cleveland, Ohio and was an extraordinarily successful government research and development activity. With funding from the National Science Foundation and later the Department of Energy (DOE), a total of 13 experimental wind turbines were put into operation including four major wind turbine designs. This research and development program pioneered many of the multi-megawatt turbine technologies in use today, including: steel tube towers, variable-speed generators, composite blade materials, partial-span pitch control, as well as aerodynamic, structural, and acoustic engineering design capabilities. The large wind turbines developed under this effort set several world records for diameter and power output. The Mod-2 wind turbine cluster produced a total of 7.5 megawatt of power in 1981. In 1987, the Mod-5B was the largest single wind turbine operating in the world with a rotor diameter of nearly 100 meters and a rated power of 3.2 megawatts. It demonstrated an availability of 95 percent, an unparalleled level for a new first-unit wind turbine. The Mod-5B had the first large-scale variable speed drive train and a sectioned, two-blade rotor that enabled easy transport of the blades.
Later, in the 1980s, California provided tax rebates for ecologically harmless power. These rebates funded the first major use of wind power for utility electricity. These machines, gathered in large wind parks such as at Altamont Pass would be considered small and un-economic by modern wind power development standards.
In the 1990s, as aesthetics and durability became more important, turbines were placed atop steel or reinforced concrete towers. Small generators are connected to the tower on the ground, then the tower is raised into position. Larger generators are hoisted into position atop the tower and there is a ladder or staircase inside the tower to allow technicians to reach and maintain the generator.
Originally wind generators were built right next to where their power was needed. With the availability of long distance electric power transmission, wind generators are now often on wind farms in windy locations and huge ones are being built offshore, sometimes transmitting power back to land using high voltage submarine cable. Since wind turbines are a renewable means of generating electricity, they are being widely deployed, but their cost is often subsidised by taxpayers, either directly or through renewable energy credits. Much depends on the cost of alternative sources of electricity. Wind generator cost per unit power has been decreasing by about four percent per year.
Companies in wind turbine industry

- ABB Ltd. — Wind turbine generators[3]
- Airtricity — only operates turbines
- AWS Truewind, LLC[4] — Wind Energy Consultants
- Bergey Windpower[5]
- Det Norske Veritas — Certification of wind turbines and wind turbine projects
- DeWind
- Ecotècnia sccl — Spanish manufacturer
- Eclectic Energy Ltd[6] — UK manufacturer of small wind turbines, including the grid-linked turbine StealthGen
- Eirbyte[7] — Supplier of small turbines in Ireland
- EMD A/S — WindPRO software package for project design and planning of turbines
- Emergya Wind Technologies[8]
- Enercon GmbH, Germany — wind turbines up to 6 MW
- ENFOR, Solutions for wind energy forecasting
- Eoltec[9], France — manufacturer of wind turbines from 6 kW to 250 kW
- Gamesa Corporacion Tecnologica
- Garrad Hassan and Partners Ltd.
- General Electric, through its subsidiary GE Energy
- The Green Company[10] — Installers of small grid linked domestic wind turbines in the UK
- Hansen Transmissions Int. — supplier of multi-MW wind turbine gear units [11]
- O'Connor Hush Energy[12] — Australian supplier of small, quiet turbines
- kk-electronic a/s[13] - Supplier of control systems
- LM Glasfiber A/S — Rotor blades ranging from 13.4 to 61.5 m
- Massmegawatts http://www.massmegawatts.com
- Moventas Oy — Moventas provides leading mechanical power transmission technology to the energy and process industries [14]
- Natural Power — International wind energy consultancy services
- NEG Micon — Merged with Vestas in 2004
- Nordex
- Pauwels Trafo Belgium/Ireland[15] — Major Wind Turbine Generator Transformer Manufacturers
- PB Power — Global Engineering Company servicing Power industry
- REpower, Germany — wind turbines up to 5 MW
- Selsam Innovations / Superturbine Inc. , California multi-rotor wind turbines http://www.selsam.com
- Siemens Wind Power A/S (formerly Bonus Energy A/S)
- Southwest Windpower [16]
- Suzlon Energy Ltd[17]
- Valmont Wind Energy, Inc. — modular tower systems for MW wind turbines.
- Sustainable Living Projects — South African Micro Wind Turbine Consultancy
- Vergnet — french manufacturer of wind turbines, 30 years of work for 5kW to 250 kW wind turbines
- Vestas Wind Systems, Denmark — the worlds largest wind turbine producer — wind turbines up to 4.5 MW [18]
- Wind Harvest — Makers of straight bladed, vertical axis turbines
- Wind Prospect — Independent wind energy developer in the UK and Australia
- Windside — Wind Energy Solutions for Extreme Conditions
- WindSim AS — WindSim software package for wind field simulations
- WinWinD Oy — Manufacture of 1 and 3 MW turbines based in Finland [19]
- Soytes Clean Energy And Electrotechnics Inc.[20]
Records
The world's largest turbines are manufactured by the Northern German companies Enercon and REpower. The Enercon E112 delivers up to 6 MW (8,000 hp), has an overall height of 186 m (610 ft) and a diameter of 114 m (374 ft), the REpower 5M delivers up to 5 MW (6,700 hp), has an overall height of 183 m (600 ft) and a diameter of 126 m (413 ft).
The turbine closest to the north pole is a Nordex N-80 in Havoygalven near Hammerfest, Norway. The ones closest to the south pole are two Enercon E-30 on Antarctica, used to power the Australian Research Division's Mawson Bay station.
The highest located turbine is at 2300 m (7,500 ft) on the mountain Gütsch near Andermatt, Switzerland. Originally a prototype of the Dutch company Lagerwey was tested there, however, it failed to meet the expectations and was demolished in 2002. Since October 2004, an Enercon E-40 produces electricity there.
See also
- Darrieus wind turbine
- Electrical generator
- Green energy
- Renewable energy
- Microgeneration
- Savonius wind turbine
- Selsam wind turbine
- Cape Wind Project
- Wind farm
- Wind power
- Windmill
- Wind power in the United Kingdom
References
- Alan Wyatt: Electric Power: Challenges and Choices. Book Press Ltd., Toronto 1986, ISBN 0-920650-00-7
- On wind farms — On the impact of wind farms on birds.
- Iberica 2000.org — On the impact of wind farms on birds.
- OtherPower.com — How to build a wind turbine from scratch (including the blade). Includes several designs.
- Scoraig Wind Electric — Homebrew wind turbines — courses, books and free information. Lots of pics of hands on construction.
External links

Primarily technical:
- Wind and Hydro Power, turbine basics+principle of operation
- How does it work? — Danish Wind Industry Organisation.
- Vertical axis turbines at the American Wind Energy Association Web site
- Floating, tilting, co-axial multi-rotor turbines
Business associations: Europe
- European Wind Energy Association (EWEA)
- British Wind Energy Association
- Danish Wind Industry Association
United States
- American Wind Energy Association
- California Wind Energy Collaborative (CWEC)
- Windustry — "How to" wind energy information
- National Wind Watch — US coalition opposed to industrial wind energy development.
Canada
Others
Other:
- News, views and analyses from the world's leading independent wind energy magazine, Windpower Monthly
- Links to articles about windpower
- Wind power
- Discussion forum for Wind and other Renewable Energy sources
- NLRE.Net — Wind Power and other Renewable Energy Information
- Wind energy data base
- Instructions to make Wind Power at Home
- Offshorewind.net — Offshore wind energy information.
- Inquiries into Wind Turbine Siting Issues Turbine noise, Wildlife impacts, and Shadow Flicker
- Bats and Wind Energy Cooperative A report on wind energy's impact on bats
- Wind Power and Other Renewables Information
Template:Energy Conversion Template:Sustainability and energy development group