Solar flare
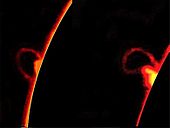
A solar flare is a sudden brightening observed over the Sun's surface or the solar limb, which is interpreted as a large energy release of up to 6 × 1025 joules of energy. These are not visible from earths surface. They are mainly followed by a colossal coronal mass ejection also known as a CME[1] (about a sixth of the total energy output of the Sun each second or 160,000,000,000 megatons of TNT equivalent, over 25,000 times more energy released from the impact of Comet Shoemaker–Levy 9 with Jupiter). The flare ejects clouds of electrons, ions, and atoms through the corona of the sun into space. These clouds typically reach Earth a day or two after the event.[2] The term is also used to refer to similar phenomena in other stars, where the term stellar flare applies.
Solar flares affect all layers of the solar atmosphere (photosphere, chromosphere, and corona), when the medium plasma is heated to tens of millions of kelvins and electrons, protons, and heavier ions are accelerated to near the speed of light. They produce radiation across the electromagnetic spectrum at all wavelengths, from radio waves to gamma rays, although most of the energy goes to frequencies outside the visual range and for this reason the majority of the flares are not visible to the naked eye and must be observed with special instruments. Flares occur in active regions around sunspots, where intense magnetic fields penetrate the photosphere to link the corona to the solar interior. Flares are powered by the sudden (timescales of minutes to tens of minutes) release of magnetic energy stored in the corona. The same energy releases may produce coronal mass ejections (CME), although the relation between CMEs and flares is still not well established.
X-rays and UV radiation emitted by solar flares can affect Earth's ionosphere and disrupt long-range radio communications. Direct radio emission at decimetric wavelengths may disturb operation of radars and other devices operating at these frequencies.
Solar flares were first observed on the Sun by Richard Christopher Carrington and independently by Richard Hodgson in 1859[3] as localized visible brightenings of small areas within a sunspot group. Stellar flares have also been observed on a variety of other stars.
The frequency of occurrence of solar flares varies, from several per day when the Sun is particularly "active" to less than one every week when the Sun is "quiet", following the 11-year cycle (the solar cycle). Large flares are less frequent than smaller ones.
Part of a series of articles about |
Heliophysics |
---|
![]() |
Cause
Flares occur when accelerated charged particles, mainly electrons, interact with the plasma medium. Scientific research has shown that the phenomenon of magnetic reconnection is responsible for the acceleration of the charged particles. On the Sun, magnetic reconnection may happen on solar arcades – a series of closely occurring loops of magnetic lines of force. These lines of force quickly reconnect into a low arcade of loops leaving a helix of magnetic field unconnected to the rest of the arcade. The sudden release of energy in this reconnection is in the origin of the particle acceleration. The unconnected magnetic helical field and the material that it contains may violently expand outwards forming a coronal mass ejection.[4] This also explains why solar flares typically erupt from what are known as the active regions on the Sun where magnetic fields are much stronger on average.
Although there is a general agreement on the flares' causes, the details are still not well known. It is not clear how the magnetic energy is transformed into the particle kinetic energy, nor it is known how the particles are accelerated to energies as high as 10 MeV (mega electron volt) and beyond. There are also some inconsistencies regarding the total number of accelerated particles, which sometimes seems to be greater than the total number in the coronal loop. We are unable to forecast flares, even to this day.
Classification
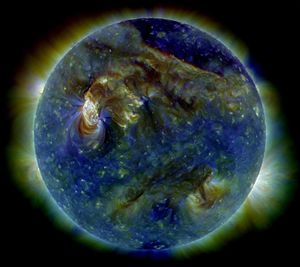
Solar flares are classified as A, B, C, M or X according to the peak flux (in watts per square meter, W/m2) of 100 to 800 picometer X-rays near Earth, as measured on the GOES spacecraft.
Classification | Peak Flux Range at 100-800 picometer |
---|---|
(Watts/square meter) | |
A | < 10−7 |
B | 10−7 - 10−6 |
C | 10−6 - 10−5 |
M | 10−5 - 10−4 |
X | > 10−4 |
Another flare classification is based on Hα spectral observations. The scheme uses both the intensity and emitting surface. The classification in intensity is qualitative, referring the flares as: (f)aint, (n)ormal or (b)rilliant. The emitting surface is measured in terms of millionths of the hemisphere and is described below (The total hemisphere area AH = 6.2 × 1012 km2.)
Classification | Corrected Area |
---|---|
[millionths of hemisphere] | |
S | < 100 |
1 | 100 - 250 |
2 | 250 - 600 |
3 | 600 - 1200 |
4 | > 1200 |
A flare then is classified taking S or a number that represents its size and a letter that represents its peak intensity, v.g.: Sn is a normal subflare.[7]
Hazards

Solar flares strongly influence the local space weather in the vicinity of the Earth. They can produce streams of highly energetic particles in the solar wind, known as a solar proton event, or "coronal mass ejection" (CME). These particles can impact the Earth's magnetosphere (see main article at geomagnetic storm), and present radiation hazards to spacecraft, astronauts and cosmonauts.
Massive solar flares are sometimes associated with Coronal Mass Ejections which can trigger geomagnetic storms that have been known to knock out electric power for extended periods of time. According to Matthew Stein, many hundred thousands of miles of high voltage lines would act like an antenna drawing the electro-magnetic pulse from a solar flare toward thousands of transformers on the world's power grids. Many transformers could burn out and be difficult to replace.
The soft X-ray flux of X class flares increases the ionization of the upper atmosphere, which can interfere with short-wave radio communication and can heat the outer atmosphere and thus increase the drag on low orbiting satellites, leading to orbital decay. Energetic particles in the magnetosphere contribute to the aurora borealis and aurora australis. Energy in the form of hard x-rays can be damaging to spacecraft electronics and are generally the result of large plasma ejection in the upper chromosphere.
The radiation risks posed by coronal mass ejections are a major concern in discussions of a manned mission to Mars, the moon, or other planets. Energetic protons can pass through the human body, causing biochemical damage,[9] presenting a hazard to astronauts during interplanetary travel. Some kind of physical or magnetic shielding would be required to protect the astronauts. Most proton storms take at least two hours from the time of visual detection to reach Earth's orbit. A solar flare on January 20, 2005 released the highest concentration of protons ever directly measured,[10] taking only 15 minutes after observation to reach Earth, indicating a velocity of approximately one-third light speed, giving astronauts as little as 15 minutes to reach shelter.

Observations
Flares produce radiation across the electromagnetic spectrum, although with different intensity. They are not very intense at white light, but they can be very bright at particular atomic lines. They normally produce bremsstrahlung in X-rays and synchrotron radiation in radio.
History
Optical Observations. Richard Carrington observed for the first time a flare on 1 September 1859 projecting the image produced by an optical telescope, without filters. It was an extraordinarily intense white light flare. Since flares produce copious amounts of radiation at Hα, adding a narrow ( ≈1 Å) passband filter centered at this wavelength to the optical telescope, allows the observation of not very bright flares with small telescopes. For years Hα was the main, if not the only, source of information about solar flares. Other passband filters are also used.
Radio Observations. During World War II, on 25 and 26 February 1942, British radar operators observed radiation that Stanley Hey interpreted as solar emission. Their discovery did not go to public until the end of the conflict. The same year Southword also observed the Sun in radio, but as with Hey, his observations were only known after 1945. In 1943 Grote Reber was the first to report radioastronomical observations of the Sun at 160 MHz. The fast development of Radioastronomy revealed new peculiarities of the solar activity like storms and bursts related with the flares. Today ground based radiotelescopes observe the Sun from ~100 MHz up to 400 GHz.
Space Telescopes. Since the beginning of the Space exploration, satellites bring to space telescopes that work at wavelengths below the UV, which are completely absorbed by the atmosphere, and where flares may be very bright. Since the 1970s, the GOES series of satellites observe the Sun at Soft X-Rays, and their observations became the standard measure of flares, relegating in some sense, the Hα classification. Hard X-Rays were observed by many different instruments, being today the most important the Reuven Ramaty High Energy Solar Spectroscopic Imager (RHESSI). Nonetheless, UV observations are today the stars of the solar imaging with their incredible fine details that reveal the complexity of the Solar Corona. Spacecraft may bring also radio detectors at very very long wavelengths (as long as a few km) that cannot propagate through the Ionosphere.
Optical telescopes
- Big Bear Solar Observatory - Located in Big Bear Lake, California (USA) and operated by the New Jersey Institute of Technology is a solar dedicated observatory with different instruments, and has a huge data bank of full disk Hα images.
- Swedish 1-m Solar Telescope Operated by the Institute for Solar Physics (Sweden), is located in the Observatorio del Roque de los Muchachos on the island of La Palma (Spain).
Radio telescopes
- Nançay Radioheliographe is an interferometer composed of 48 antennas observing at meter-decimeter wavelengths. The radioheliographe is installed at the Nançay Radio Observatory (France).
- Owens Valley Solar Array is a radio interferometer operated by New Jersey Institute of Technology consisting of 7 antenas observing from 1 to 18 GHz in both left and right circular polarization. OVSA is located in Owens Valley, California, (USA), now is under reform, increasing to 15 the total number of antennas and upgrading its control system.
- Nobeyama Radioheliograph is an interferometer installed at the Nobeyama Radio Observatory (Japan) formed by 84 small (80 cm) antennas, with receivers at 17 GHz (left and right polarization) and 34 GHz operating simultaneously. It observes continuously the Sun, producing daily snapshots. (See link)
- Nobeyama Radio Polarimeters are a set of radio telescopes installed at the Nobeyama Radio Observatory that observes continuously the full Sun (no images) at the frequencies of 1, 2, 3.75, 9.4, 17, 35, and 80 GHz, at left and right circular polarization.
- Solar Submillimeter Telescope is a single dish telescope, that observes continuously the Sun at 212 and 405 GHz. It is installed at Complejo Astronomico El Leoncito in Argentina. It has a focal array composed by 4 beams at 212 GHz and 2 at 405 GHz, therefore it can locate instantaneously the position of the emitting source. SST is the only solar submillimeter telescope currently in operation.
Space telescopes
The following spacecraft missions have flares as their main observation target.
- Yohkoh - The Yohkoh (originally Solar A) spacecraft observed the Sun with a variety of instruments from its launch in 1991 until its failure in 2001. The observations spanned a period from one solar maximum to the next. Two instruments of particular use for flare observations were the Soft X-ray Telescope (SXT), a glancing incidence low energy X-ray telescope for photon energies of order 1 keV, and the Hard X-ray Telescope (HXT), a collimation counting instrument which produced images in higher energy X-rays (15-92 keV) by image synthesis.
- WIND - The Wind spacecraft is devoted to the study of the interplanetary medium. Since the Solar Wind is its main driver, solar flares effects can be traced with the instruments aboard Wind. Some of the WIND experiments are: a very low frequency spectrometer, (WAVES), particles detectors (EPACT, SWE) and a magnetometer (MFI).
- GOES - The GOES spacecraft are satellites in geostationary orbits around the Earth that have measured the soft X-ray flux from the Sun since the mid-1970s, following the use of similar instruments on the Solrad satellites. GOES X-ray observations are commonly used to classify flares, with A, B, C, M, and X representing different powers of ten — an X-class flare has a peak 1-8 Å flux above 0.0001 W/m2.
- RHESSI - The Reuven Ramaty High Energy Solar Spectral Imager is designed to image solar flares in energetic photons from soft X rays (~3 keV) to gamma rays (up to ~20 MeV) and to provide high resolution spectroscopy up to gamma-ray energies of ~20 MeV. Furthermore, it has the capability to perform spatially resolved spectroscopy with high spectral resolution.
- SOHO - The Solar and Heliospheric Observatory is collaboration between the ESA and NASA which is in operation since December, 1995. It carries 12 different instruments, among them the Extreme ultraviolet Imaging Telescope (EIT), the Laboratory for the Analysis of Organisational Communication Systems (LASCO) and the Michelson Doppler Imager (MDI).
- TRACE - The Transition Region and Coronal Explorer is a NASA Small Explorer program (SMEX) to image the solar corona and transition region at high angular and temporal resolution. It has passband filters at 173 Å, 195 Å, 284 Å, 1600 Å with a spatial resolution of 0.5 arc sec, the best at these wavelengths.
- SDO - The Solar Dynamics Observatory is a NASA project composed of 3 different instruments: the Helioseismic and Magnetic Imager (HMI), the Atmospheric Imaging Assembly (AIA) and the Extreme Ultraviolet Variability Experiment (EVE). It is in operation since February, 2010.
- Hinode -The Hinode spacecraft, originally called Solar B, was launched by the Japan Aerospace Exploration Agency in September 2006 to observe solar flares in more precise detail. Its instrumentation, supplied by an international collaboration including Norway, the U.K., the U.S., and Africa focuses on the powerful magnetic fields thought to be the source of solar flares. Such studies shed light on the causes of this activity, possibly helping to forecast future flares and thus minimize their dangerous effects on satellites and astronauts.[11]
Examples of large solar flares
![]() |

The most powerful flare ever observed was the first one to be observed, on September 1, 1859, and was reported by British astronomer Richard Carrington and independently by an observer named Richard Hodgson. The event is named the Solar storm of 1859, or the "Carrington event". The flare was visible to a naked-eye (in white light), and produced stunning auroras down to tropical latitudes such as Cuba or Hawaii, and set telegraph systems on fire.[13] The flare left a trace in Greenland ice in the form of nitrates and beryllium-10, which allow its strength to be measured today (New Scientist, 2005). Cliver & Salvgaard (2004) reconstructed the effects of this flare and compared with other events of the last 150 years. In their words: While the 1859 event has close rivals or superiors in each of the above categories of space weather activity, it is the only documented event of the last ∼150 years that appears at or near the top of all of the lists.
In modern times, the largest solar flare measured with instruments occurred on November 4, 2003. This event saturated the GOES detectors, and because of this its classification is only approximate. Initially, extrapolating the GOES curve, it was pegged at X28.[14] Later analysis of the ionospheric effects suggested increasing this estimate to X45.[15] This event produced the first clear evidence of a new spectral component above 100 GHz.[16]
Other large solar flares also occurred on April 2, 2001 (X20),[17] October 28, 2003 (X17.2 & X10),[18] September 7, 2005 (X17),[17] February 17, 2011 (X2),[19][20][21] August 9, 2011 (X6.9)[8][22] and March 7, 2012 (X5.4).[23]
Flare spray
Flare sprays are a type of eruption associated with solar flares.[24] They involve faster ejections of material than eruptive prominences,[25] and reach velocities of 500 to 1200 kilometers per second.[24]
Prediction
Current methods of flare prediction are problematic, and there is no certain indication that an active region on the Sun will produce a flare. However, many properties of sunspots and active regions correlate with flaring. For example, magnetically complex regions (based on line-of-sight magnetic field) called delta spots produce most large flares. A simple scheme of sunspot classification due to McIntosh is commonly used as a starting point for flare prediction.[citation needed] Predictions are usually stated in terms of probabilities for occurrence of flares above M or X GOES class within 24 or 48 hours. The U.S. National Oceanic and Atmospheric Administration (NOAA) issues forecasts of this kind.
See also
- Aurora (astronomy)
- Coronal mass ejection
- Solar prominence
- Flare star
- Gamma Ray Burst
- Geomagnetic storm
- March 1989 geomagnetic storm
- Magnetic reconnection
- Solar wind
- Solar storm of 1859
- List of plasma (physics) articles
References
- ^ Kopp, G. (2005). "The Total Irradiance Monitor (TIM): Science Results". Solar Physics. 20 (1–2): 129–139. Bibcode:2005SoPh..230..129K. doi:10.1007/s11207-005-7433-9.
{{cite journal}}
: Unknown parameter|coauthors=
ignored (|author=
suggested) (help) - ^ Menzel, Whipple, and de Vaucouleurs, "Survey of the Universe", 1970
- ^ "Description of a Singular Appearance seen in the Sun on September 1, 1859", Monthly Notices of the Royal Astronomical Society, v20, pp13+, 1859
- ^ "The Mysterious Origins of Solar Flares", Scientific American, April 2006
- ^ "Great Ball of Fire". NASA. Retrieved May 21, 2012.
- ^ Tamrazyan, Gurgen P. (1968). "Principal Regularities in the Distribution of Major Earthquakes Relative to Solar and Lunar Tides and Other Cosmic Forces". ICARUS. 9. Elsevier: 574–592. Bibcode:1968Icar....9..574T. doi:10.1016/0019-1035(68)90050-X.
- ^ Tandberg-Hanssen, Einar (1988). Cambridge University Press (ed.). "The physics of solar flares".
{{cite news}}
: Unknown parameter|coauthors=
ignored (|author=
suggested) (help) - ^ a b "Sun Unleashes X6.9 Class Flare". NASA. Retrieved March 7, 2012.
- ^ "New Study Questions the Effects of Cosmic Proton Radiation on Human Cells". Retrieved 2008-10-11.
- ^ A New Kind of Solar Storm
- ^ "Japan launches Sun 'microscope'". BBC. 2006-09-23. Retrieved 2009=05-19.
{{cite news}}
: Check date values in:|accessdate=
(help) - ^ "Extreme Space Weather Events". National Geophysical Data Center. Retrieved May 21, 2012.
- ^ Bell, Trudy E.; Phillips, Tony (2008). "A Super Solar Flare". Science@NASA. Retrieved May 21, 2012.
- ^ "SOHO Hotshots". Sohowww.nascom.nasa.gov. Retrieved May 21, 2012.
- ^ "Biggest ever solar flare was even bigger than thought | SpaceRef - Your Space Reference". SpaceRef. 2004-03-15. Retrieved May 21, 2012.
- ^ http://www.guigue.gcastro.net/publicaciones/cientificos/Kaufmannetal2004.pdf
- ^ a b "BIGGEST SOLAR X-RAY FLARE ON RECORD - X20". NASA. Retrieved May 21, 2012.
- ^ "X 17.2 AND 10.0 FLARES!". NASA. Retrieved May 21, 2012.
- ^ Hendrix, Susan (2012-03-07). "Valentine's Day Solar Flare" (video included). Goddard Space Flight Center. Retrieved May 21, 2012.
- ^ "Solar flare to jam Earth's communications". ABC. Retrieved May 21, 2012.
- ^ Kremer, Ken. "Sun Erupts with Enormous X2 Solar Flare". Universe Today. Retrieved May 21, 2012.
{{cite web}}
: Italic or bold markup not allowed in:|publisher=
(help) - ^ Bergen, Jennifer. "Sun fires powerful X6.9-class solar flare". Geek.com. Retrieved May 21, 2012.
{{cite web}}
: Italic or bold markup not allowed in:|publisher=
(help) - ^ "Second Biggest Flare Of the Solar Cycle". NASA. Retrieved May 21, 2012.
- ^ a b Tarou Morimoto, Hiroki Kurokawa. "Effects of Magnetic and Gravity forces on the Acceleration of Solar Filaments and Coronal Mass Ejections" (PDF). Retrieved 2009-10-08.
- ^ E. Tandberg-Hanssen, Sara F. Martin and Richard T. Hansen (1980). "Dynamics of flare sprays". Solar Physics.
- "Superflares could kill unprotected astronauts". NewScientist.com. Retrieved 17 June 2005.
- Mewaldt, R.A., et al. 2005. Space weather implications of the 20 January 2005 solar energetic particle event. Joint meeting of the American Geophysical Union and the Solar Physics Division of the American Astronomical Society. May 23–27. New Orleans. Abstract.
- Solar Flares NASA Video from 2003
- Solar Flares Solar & Heliospheric Observatory Video from 2002
External links

- Live Solar Images and Data Site Includes x-ray flare, geomagnetic, space weather information detailing current solar events.
- Solar Cycle 24 and VHF Aurora Website (www.solarcycle24.com)
- Solar Weather Site
- Current Solar Flare - and geomagnetic activity in dashboard style (www.solar-flares.info)
- STEREO Spacecraft Site
- Early BBC report on the November 4, 2003 flare
- Later BBC report on the November 4, 2003 flare
- NASA SOHO observations of flares
- Stellar Flares - D. Montes, UCM.
- The Sun - D. Montes, UCM.
- Solar Information Mobile Application (Android)
- ASC / Alliances Center for Astrophysical Thermonuclear Flashes
- 'The Sun Kings', lecture by Dr Stuart Clark on the discovery of solar flares given at Gresham College, 12 September 2007 (available as a video or audio download as well as a text file).
- An X Class Flare Region on the Sun - NASA Astronomy Picture of the Day
- Sun trek website An educational resource for teachers and students about the Sun and its effect on the Earth
- NASA - Carrington Super Flare NASA May 6, 2008
- Archive of the most severe solar storms
- Animated explanation of Solar Flares from the Photosphere (University of Glamorgan)
- 1 min. 35 sec. Mini documentary: How big are solar flares' prominences? A simplified explanation of the size of solar flares' prominences as compared to Earth.
- The Most Powerful Solar Flares Ever Recorded - spaceweather.com (X9+ summary)
- Most Energetic Flares since 1976 (X5.7+ details)