Synthetic diamond
Synthetic diamond (also known as HPHT diamond and CVD diamond) is diamond produced in a technological process, as opposed to natural diamond, which is produced by geological processes.
Synthetic diamond is made using two major processes: chemical vapor deposition (CVD) and high-pressure high-temperature (HPHT) synthesis. Its properties depend on the manufacturing process, and can be inferior, similar or superior to those of natural diamond. In particular, the hardness, thermal conductivity and electron mobility are superior in some synthetic diamonds. Certain synthetic diamonds are known to be the hardest and most thermally conductive among any known material (as of 2009). Consequently, synthetic diamonds are widely used in abrasives, cutting and polishing tools and as heat sinks. Their advantages for electronic applications have been demonstrated and those applications are forthcoming. Detectors of UV light or high-energy particles, made of synthetic diamond, are being applied at high-energy research facilities and are already available commercially. Because of a unique combination of thermal and chemical stablity, low thermal expansion and high optical transparency in the wide spectral range, synthetic diamond is becoming the most popular material for optical windows in high-power CO2 lasers and gyrotrons.
Both CVD and HPHT diamonds can be cut into gems, either clear white or colored yellow, brown, blue or even green or orange. This creates major concerns in the diamond trading business, as a results of which special spectroscopic devices and techniques have been developed to distinguish synthetic and natural diamonds.
History

After the 1797 discovery that diamond was pure carbon, many attempts were made to alter the cheaper forms of carbon—generally with little success. One of the early successes reported in the field was by Ferdinand Frédéric Henri Moissan in 1893. His method involved heating charcoal at up to 3500 °C with iron in a carbon crucible in an electric furnace, in which an electric arc was struck between carbon rods inside blocks of lime.[1] The molten iron was then rapidly cooled by immersion in water. The contraction generated by the cooling supposedly produced the high pressure required to transform graphite into diamond. Moissan published his work in a series of articles in the 1890s. [2]
Many other scientists tried to replicate his experiments. Sir William Crookes claimed success in 1909. Ruff claimed in 1917 to have reproduced diamonds up to 7 mm in diameter, but later retracted his claims. [3] In 1926, Dr. Willard Hershey of McPherson College read journal articles about Moissan's and Ruff's experiments and replicated their work, producing a synthetic diamond. That diamond is on display today at the McPherson Museum in Kansas. [4] Despite the claims of Moissan, Ruff, and Hershey, no other experimenter could reproduce their synthesis, leading some to contend that the early successes were the result of seeding by good-willed co-workers. [5][6]
The most definitive duplication attempts were performed by Sir Charles Algernon Parsons. He devoted 30 years and a considerable part of his fortune to reproduce many of the experiments of Moissan as well as those of Hannay but also adapted processes of his own. He wrote a number of articles—one of the earliest on HPHT diamonds—in which he claimed to have produced small diamonds.[7] However in 1928 he authorized C.H. Desch to publish an article in which he stated his belief that no synthetic diamonds (including those of Moissan and others) had been produced up to that date. In fact he found that most diamonds produced so far were likely synthetic spinel. [3]
GE diamond project
In 1941 an agreement was made between three companies: General Electric (GE), Norton and Carborundum to further develop diamond synthesis. They were able to heat carbon to about 3000 °C under a pressure of 3.5 gigapascals (GPa), for a few seconds.[3] The Second World War ended this project soon thereafter. It was resumed in 1951 at the Schenectady Laboratories of GE, and a high-pressure diamond group was formed with F.P. Bundy and H.M. Strong. Tracy Hall and others joined it shortly afterwards. [8]
The group improved on the anvils designed by Percy Bridgman, who received a Nobel prize for his work in 1946. Bundy and Strong made the first improvements and more were made later by Hall. The GE team used tungsten carbide anvils within a hydraulic press to squeeze the carbonaceous sample held in a catlinite container, the finished grit being squeezed out of the container through a gasket. It was believed that on one occasion a diamond was produced, but since experiments could not be reproduced such claims could not be maintained. [3]
Hall achieved the first commercially successful synthesis of diamond on December 16, 1954 (announced on February 15, 1955). His breakthrough was using an elegant "belt" press apparatus which raised the achievable pressure from 6 to 18 GPa and the temperature above 2400 °C, using a pyrophyllite container, and having the graphite dissolved within molten nickel, cobalt or iron, a "solvent-catalyst".[9] The largest diamond he produced was 0.15 mm across, clearly unsuitable for jewelry but usable in industrial abrasives. Hall was able to have co-workers replicate his work and the discovery was published in the major journal Nature. He was the first person to grow a synthetic diamond according to a reproducible, verifiable and witnessed process and received a gold medal of the American Chemical Society in 1972 for his work. Hall left GE in 1955, and three years later developed a completely independent apparatus for the synthesis of diamond (the tetrahedral press with four anvils), thus avoiding infringement of his previous patent, which was still assigned to GE.[3]
Later developments
Another successful diamond synthesis was produced on February 16, 1953 in Stockholm, Sweden by the ASEA (Allemanna Svenska Elektriska Aktiebolaget), Sweden's major electrical manufacturing company. Starting from 1949, the company employed a team of five scientists and engineers within a top-secret diamond-making project code-named QUINTUS. The team used a bulky split-sphere apparatus designed by Baltzar von Platen (1898-1984) and Anders Kämpe (1928-1984).[8][10] Pressure was maintained within the device at an estimated 8.4 GPa for an hour. A few small crystals were produced, but not of gem quality or size. The work was not reported until the 1980s.[11] During the 1980s a new competitor emerged in Korea named Iljin Diamond, followed later by hundreds of Chinese entrants. Iljin Diamond allegedly accomplished this by misappropriating trade secrets from GE via a Korean former GE employee in 1988.[12]

Synthetic gem-quality diamond crystals were first produced in 1970 (reported in 1971) again by GE. Large crystals need to grow very slowly under tightly controlled conditions. The first successes used a pyrophyllite tube seeded at each end with thin pieces of diamond and with the graphite feed material placed in the center, the metal solvent, nickel, was placed between the graphite and the seeds. The container was heated and the pressure was raised to ~5.5 GPa. The crystals grow as they flow from the center to the ends of the tube, and extending the length of the process produces larger crystals—initially a week-long growth process produced gem-quality stones of around 5 mm and 1 carat (200 mg). The graphite feed was soon replaced by diamond grit, as there was almost no change in material volume so the process was easier to control.[11]
The first gem-quality stones were always yellow to brown in color, due to contamination with nitrogen. Inclusions were common, especially "plate-like" ones from the nickel. Removing all nitrogen from the process by adding aluminum or titanium produced colorless "white" stones, and removing the nitrogen and adding boron produced blue ones.[13] However, removing nitrogen slows the growth process and reduces the crystalline quality, so the process is normally run with nitrogen present. In terms of physical properties, the GE stones were not quite identical to natural stones. The colorless stones produced strong short- and long-lasting light emission (fluorescence and phosphorescence, respectively) under short-wavelength ultraviolet light but were inert under long-wave UV (among natural diamonds, only rare blue stones do this). All the GE stones also showed strong yellow fluorescence under X-rays.[11] The De Beers Diamond Research Laboratory has since grown, for research purposes only, stones of up to 25 carats (5.0 g). For growing such diamonds, stable HPHT conditions have to be kept for 6 weeks. However, most stones are 1 carat (200 mg) to 1.5 carats (300 mg) for economic reasons, especially with the spread of the Russian BARS apparatus since the 1980s.[14]
In the 1950s, research has started in the US and Soviet Union on growth of diamond by pyrolysis of hydrocarbon gases, at relatively low temperatures ~800 °C, in a low-pressure process called chemical vapor deposition (CVD). Eversole reportedly achieved vapor deposition of diamond over diamond substrate in 1953, but it was not reported until 1962.[15] Diamond film deposition was independently reproduced by Angus and coworkers in 1968[16] and by Deryagin and Fedoseev in 1970.[17] Whereas Eversole and Angus used large, expensive, single-crystal diamonds as substrates, the russian team succeeded in making diamond films on non-diamond materials (silicon and metals) that initiated massive research on cheap diamond coatings in the 1980s.[11]
Manufacturing technologies
There are several methods used to produce synthetic diamonds. The original method usess high pressure and high temperature and is still widely used because of its relative low cost. It uses large presses that can weigh a couple of hundred tons to produce a pressure of 5 GPa at 1500 °C. The second method, using chemical vapor deposition, is basically a method creating a carbon plasma on top of a substrate onto which the carbon atoms deposit to form diamond. Other methods are explosive formation (detonation nanodiamond) and ultrasound sonication of graphite solutions.
High pressure, high temperature
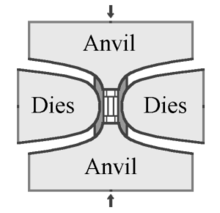
In the HPHT method, there are three main press designs used to supply the pressure and temperature necessary to produce synthetic diamond: the belt press, the cubic press and the split-sphere (BARS) press.
The original GE invention by Tracy Hall uses the belt press, wherein the upper and lower anvils supply the pressure load and heating current to a cylindrical volume. This internal pressure is confined radially by a belt of pre-stressed steel bands. A variation of the belt press uses hydraulic pressure to confine the internal pressure, rather than steel belts.[18] Belt presses are still used today by the major manufacturers at a much larger scale than the original designs.[11]
The second type of press design is the cubic press. A cubic press has six anvils which provide pressure simultaneously onto all faces of a cube-shaped volume. The first multi-anvil press design was actually a tetrahedral press, using only four anvils to converge upon a tetrahedron-shaped volume.[19] The cubic press was created shortly thereafter to increase the pressurized volume. A cubic press is typically smaller than a belt press and can achieve the pressure and temperature necessary to create synthetic diamond faster. However, cubic presses cannot be easily scaled up to larger volumes. One could increase the pressurized volume by either increasing the size of the anvils (thereby increasing by a great factor the amount of force needed on the anvils to achieve a similar pressurization) or by decreasing the surface area to volume ratio of the pressurized volume (by using more anvils to converge upon a different platonic solid, such as a dodecahedron), but such a press would be unnecessarily complex and not easily manufacturable.[20]
BARS is the smallest, most efficient and economical of all the diamond-producing setups. In the center of a BARS device, there is a ceramic cylindrical reaction cell of about 2 cm3 in size. The cell is placed into a cubic-shaped pressure-transmitting material, which is pressed by elements made from cemented carbide (VK10 hard alloy).[21] The outer octahedral cavity is pressed by 8 steel sectors. After mounting, the whole assembly is locked in a disc-type barrel with a diameter ~1 meter. The barrel is filled with oil, which pressurizes upon heating, and the oil pressure is transferred to the central cell. The central cell is heated up by a coaxial graphite heater. Temperature is measured with a thermocouple.[22]
Chemical vapor deposition
Chemical vapor deposition of diamond is a method of growing diamond from a hydrocarbon gas mixture. This method has been the subject of a great deal of research since the early 1980s, especially due to its (potential) applications in the cutting tool, semiconductor and diamond gem industries.
CVD diamond growth typically occurs under low pressure (1–27kPa) and involves feeding varying amounts of gases into a chamber, energizing them and providing conditions for diamond growth on the substrate. The gases always include a carbon source, and typically include hydrogen as well, though the amounts used vary greatly depending on the type of diamond being grown. Energy sources include hot filament, microwave power, and arc discharges, among others. The energy source is intended to generate plasma in which the gas molecules are broken down to chemically active radicals. The actual chemical process for diamond growth is still under study and is complicated by the very wide variety of growth processes used. The advantages of CVD diamond growth include the ability to grow diamond over large areas, the ability to grow diamond on various substrates, and the fine control over the chemical impurities and thus properties of the diamond produced.[23]
Explosive detonation
Diamond nanocrystals can be formed from the detonation of certain explosives, resulting in the formation of detonation nanodiamond. These nanodiamonds are only now beginning to reach the market in bulk quantities, for polishing applications, principally from Russia and China.[24]
Ultrasound cavitation
Diamond nanocrystals can be synthesized from a suspension of graphite in organic liquid at atmospheric pressure and room temperature using ultrasonic cavitation. The yield is ~10%. Cost of nanodiamonds produced by this method is estimated to be competitive with that for the HPHT process. [25][26] This technique is relatively simple, but it has been reported by two research groups only, and has no any industrial use yet (May, 2009).
Properties
Traditionally, purity and crystallinity are considered the basic diamond properties. Purity and high crystalline perfection make diamond transparent and clear, whereas its hardness, optical dispersion ("luster") and chemical stability (combined with marketing), make it a popular gemstone. High thermal conductivity is also important for technical applications. Whereas high optical dispersion is an intrinsic property of all diamonds, other properties vary, and are expanded below.
Crystallinity
Diamond can be one single, continuous crystal or it can be made up of many smaller crystals ("polycrystalline"). Large single-crystal diamond is typically used in gemstones, whereas polycrystalline diamond goes to industrial applications such as mining and cutting tools. Within polycrystalline diamond, the diamond is often described by the average size of the crystals that make it up, called the "grain size." Grain sizes range from nanometers to hundreds of micrometers, usually referred to as "nanocrystalline" and "microcrystalline" diamond, respectively.[11]
Hardness
A diamond's hardness strongly varies depending on its impurities and crystallinity. Nanocrystalline diamond produced through CVD diamond growth, for instance, can have hardness from 30% to 75% to that of single crystal diamond, and the hardness can be tuned for specific applications. Some single-crystal diamonds grown through HPHT synthesis or CVD, and HPHT nanocrystalline diamonds (see hyperdiamond) are harder than any known natural diamond.[27][28][29]
Impurities and inclusions
No crystal is absolutely pure. Any atom other than carbon found in a diamond is an impurity, and those atoms can aggregate into macroscopic phases called inclusions. While impurities can be unwanted, they can also be introduced on purpose to control the properties of the diamond. For instance, pure diamond is an electrical insulator, but diamond with boron added is an electrical conductor and even superconductor,[30] allowing it to be used in new technological applications. Nitrogen impurities hinder movement of lattice dislocations (defects within the crystal structure) and put the lattice under compressive stress, increasing hardness and toughness.[31]
Thermal conductivity
Unlike most electrical insulators, diamond is a good conductor of heat because of the strong covalent bonding within the crystal. The thermal conductivity of pure diamond is already the highest among any known solid. However, its value is reduced by small amount (natural abundance 1.1 %) of 13C isotope, which ruins the ideality of the 12C diamond lattice in terms of thermal conduction. Therefore, monocrystalline synthetic diamond enriched in 12C isotope (99.9%) has the highest of all thermal conductivity, ~30 W/cm·K at room temperature, five times more than copper.[32]
Applications
Given the extraordinary set of physical properties diamond exhibits, diamond has and could have a wide-ranging impact in many fields.
Machining and cutting tools

Diamonds have long been used in machine tools, especially when processing non-ferrous alloys, as carbon dissolves in iron at the high temperatures created by high-speed machining. While natural diamond is still applied for this, the usage of synthetic diamond is far greater. The most common form of diamond in cutting tools is micrometer-sized grains dispersed in a metal matrix (usually cobalt), sintered onto the tool. This is typically referred to in industry as poly-crystalline diamond (PCD). PCD tipped tools can be found in mining and in the automotive aluminum cutting industry.[33]
For the past fifteen years, work has been done to coat metallic tools with CVD diamond, and though the work still shows promise it has not significantly displaced traditional PCD tools.[34]
Heat sinks
Because of superior thermal conductivity and dielectric properties of diamond, it is commercially sold for heat sink applications.[35] For example, in semiconductor technology, it prevents silicon and other semiconducting materials from overheating. [36]
Diamond's thermal conductivity is made use of by jewelers and gemologists who may employ an electronic thermal probe to separate diamonds from their imitations. These probes consist of a pair of battery-powered thermistors mounted in a fine copper tip. One thermistor functions as a heating device while the other measures the temperature of the copper tip: if the stone being tested is a diamond, it will conduct the tip's thermal energy rapidly enough to produce a measurable temperature drop. This test takes about 2–3 seconds.[6]
Windows
Diamond is hard, chemically inert, it has a high thermal conductivity and low thermal expansion, in contrast to many window materials used for the infrared and microwave parts of the spectrum. Therefore, diamond is starting to replace zinc selenide and other materials as the output window of high-power CO2 lasers and gyrotrons. Those windows should have shapes of disks having large diameter (~10 cm for gyrotrons) and small thickness (to reduce absorption). In these shape requirements, CVD diamond outperforms any other diamond varieties.[37]
Electronics
Diamond has potential uses as a semiconductor,[38] because it can be doped with impurities like boron and phosphorus. Since these elements contain one more or one less valence electron than carbon, they turn the diamonds into p-type or n-type semiconductors. Making a p-n junction by sequential doping of diamond with boron and phosphorus produces UV light emitting diodes (LEDs, at 235 nm).[39] Another useful property of diamond for electronics is high carrier mobility, which reaches 4500 cm2/(V·s) in single-crystal CVD diamond.[40] High mobility is favorable for high-frequency electronic devices.
Conductive CVD diamond is a useful electrode under many circumstances.[41] Photochemical methods have been developed for covalently linking DNA to the surface of polycrystalline diamond films produced through CVD.[42] In addition, the diamonds can detect redox reactions that cannot ordinarily be studied and in some cases degrade redox-reactive organic contaminants in water supplies. Because diamond is mechnically and chemically stable, it can be used as an electrode under conditions that would destroy traditional materials. For such reasons, waste water treatment of organic effluents using diamond,[43] as well as production of strong oxidants, have been reported.[44]
Diamond transistors are functional to much higher temperatures than silicon devices and are resistant to chemical and radiation damage. While no diamond transistors have yet been successfully integrated into commercial electronics, they show promise for use in exceptionally high power situations and hostile environments.[45]
Diamond shows great promise as a potential radiation detection device. It is radiation hard and has a wide bandgap of 5.5 eV (at room temperature). Therefore, it is employed in applications such as the BaBar detector at the Stanford Linear Accelerator [46] or BOLD (Blind to the Optical Light Detectors for VUV solar observations). [47][48] Pure single-crystal synthetic diamonds are approaching the very high purity and crystallographic structure perfection required to replace silicon in devices such as synchrotrons used in CT scanning;[40][49] they will be able to sustain the increased intensities of next generation light sources.[50]
CVD diamond growth has been used in conjunction with lithographic techniques to encase microcircuits inside diamond. The CVD process is also used to create designer diamond anvils as a novel probe for measuring electric and magnetic properties of materials at ultra high pressures using a diamond anvil cell.[51]
Gemstones
Diamonds for use as gemstones are grown by HPHT [14] or CVD [52] methods. They are available in yellow or blue colors, and to a lesser extent colorless (or "white"). The yellow color comes from nitrogen impurities in the manufacturing process while the blue color comes from boron.[13] Other colors such as pink or green are achievable after synthesis using irradiation.[53] Several companies also offer memorial diamonds grown using cremated remains. Gem-quality diamonds are currently produced by the following producers listed in the table below.
Company | Method | Color |
---|---|---|
Apollo Diamond | CVD | colorless |
Chatham | HPHT | yellow, blue |
D.NEA | yellow, blue, white | |
Gemesis | yellow |
Gem-quality diamonds grown in a lab can be chemically, physically and optically identical to naturally occurring ones, although they can be distinguished by spectroscopy in infrared, ultraviolet, or X-ray wavelengths. The DiamondView tester from De Beers uses UV fluorescence to detect trace impurities of nitrogen, nickel or other metals in HPHT or CVD diamonds.[11]
The mined diamond industry is evaluating marketing and distribution countermeasures to these less expensive alternatives. The three largest distributors of natural diamonds have made public statements about selling their diamonds with full disclosure and have implemented measures to laser-inscribe serial numbers on their gemstones.[52]
See also

- Material properties of diamond
- Crystallographic defects in diamond
- BARS (Diamonds)
- Detonation nanodiamond
- Diamond simulant
- Moissanite
- poly(hydridocarbyne)
- covalent superconductors
References
- ^ Royère, C (1999). "The electric furnace of Henri Moissan at one hundred years: connection with the electric furnace, the solar furnace, the plasma furnace?". Annales pharmaceutiques françaises. 57 (2): 116–30. PMID 10365467.
{{cite journal}}
: Unknown parameter|quotes=
ignored (help) - ^ H. Moissan (1996). Ann. de Chemie et de Phys. 8: 446.
{{cite journal}}
: Missing or empty|title=
(help) - ^ a b c d e Nassau, Kurt (1980). Gems made by Man. Chilton Book Co. ISBN 0801967732.
- ^ J. Willard Hershey Ph.D. (1940). Book of Diamonds. Heathside Press, New York. ISBN 0486418162.
- ^ K. Lonsdale (1962). "Further Comments on Attempts by H. Moissan, J. B. Hannay and Sir Charles Parsons to Make Diamonds in the Laboratory". Nature. 196: 104. doi:10.1038/196104a0].
- ^ a b M. O'Donoghue (2006). Gems. Elsevier. p. 473. ISBN 0-75-065856-8.
- ^ "Parson's articles of 1893 and those of others (See Synthetic Diamond Section)". Retrieved 2009-05-05.
- ^ a b Hazen, Robert M. (1999). The diamond makers. Cambridge University Press. ISBN 0521654742.
- ^ H. T. Hall (1960). "Ultra-high pressure apparatus" (PDF). Rev. Sci. Instr. 31: 125. doi:10.1063/1.1716907.
- ^ H. Liander (1955). ASEA Journal. 28: 97.
{{cite journal}}
: Missing or empty|title=
(help) - ^ a b c d e f g A. S. Barnard (2000). The diamond formula: diamond synthesis--a gemological perspective. Butterworth-Heinemann. ISBN 0750642440, 9780750642446.
{{cite book}}
: Check|isbn=
value: invalid character (help) - ^ General Electric v. Sung, 843 F. Supp. 776
- ^ a b R. C. Burns, V. Cvetkovic and C. N. Dodge (1990). "Growth-sector dependence of optical features in large synthetic diamonds". Journal of Crystal Growth. 104: 257. doi:10.1016/0022-0248(90)90126-6.
- ^ a b R. Abbaschian; et al. (2005). "High pressure-high temperature growth of diamond crystals using split sphere apparatus". Diam. Rel. Mater. 14: 1916. doi:10.1016/j.diamond.2005.09.007.
{{cite journal}}
: Explicit use of et al. in:|author=
(help) - ^ W. G. Eversole Synthesis of diamond, US patent 3030187 and 3030188 (1962)
- ^ J. C. Angus; et al. (1968). "Growth of Diamond Seed Crystals by Vapor Deposition". J. Appl. Phys. 39: 2915. doi:10.1063/1.1656693.
{{cite journal}}
: Explicit use of et al. in:|author=
(help) - ^ B.V. Deryagin and D. V. Fedoseev (1970). Rus. Chem. Rev. 39: 783.
{{cite journal}}
: Missing or empty|title=
(help) - ^ "HPHT synthesis". Retrieved 2009-05-05.
- ^ H. T. Hall (1958). "Ultrahigh-Pressure Research: At ultrahigh pressures new and sometimes unexpected chemical and physical events occur" (PDF). Science. 128: 445. doi:10.1126/science.128.3322.445.
- ^ E. Ito (2007). G. Schubert (ed.). Multianvil cells and high-pressure experimental methods, in Treatise of Geophysics. Elsevier, Amsterdam. p. 197-230. ISBN 0812922751.
{{cite book}}
: Unknown parameter|Volume=
ignored (|volume=
suggested) (help) - ^ M. G. Loshak and L. I. Alexandrova (2001). "Rise in the efficiency of the use of cemented carbides as a matrix of diamond-containing studs of rock destruction tool". Int. J. Refractory Metals and Hard Materials. 19: 5. doi:10.1016/S0263-4368(00)00039-1.
- ^ N. Pal'yanov; et al. (2002). "Fluid-bearing alkaline carbonate melts as the medium for the formation of diamonds in the Earth's mantle: an experimental study". Lithos. 60: 145. doi:10.1016/S0024-4937(01)00079-2.
{{cite journal}}
: Explicit use of et al. in:|author=
(help) - ^ M Werner; et al. (1998). "Growth and application of undoped and doped diamond films". Rep. Prog. Phys. 61: 1665. doi:10.1088/0034-4885/61/12/002.
{{cite journal}}
: Explicit use of et al. in:|author=
(help) - ^ V. Yu. Dolmatov (2006). "Development of a rational technology for synthesis of high-quality detonation nanodiamonds". Russian Journal of Applied Chemistry. 79: 1913. doi:10.1134/S1070427206120019.
- ^ E. M. Galimov; et al. (2004). "Experimental Corroboration of the Synthesis of Diamond in the Cavitation Process". Doklady Physics. 49: 150. doi:10.1134/1.1710678.
{{cite journal}}
: Explicit use of et al. in:|author=
(help) - ^ A. Kh. Khachatryan; et al. (2008). "Graphite-to-diamond transformation induced by ultrasonic cavitation". Diam. Relat. Mater. 17: 931. doi:10.1016/j.diamond.2008.01.112.
{{cite journal}}
: Explicit use of et al. in:|author=
(help) - ^ H. Sumiya (2005). "Super-hard diamond indenter prepared from high-purity synthetic diamond crystal". Rev. Sci. Instrum. 76: 026112. doi:10.1063/1.1850654.
- ^ C-S Yan; et al. (2005). "Ultrahard diamond single crystals from chemical vapor deposition". Phys. Stat. Solidi (a). 201: R25. doi:10.1002/pssa.200409033.
{{cite journal}}
: Explicit use of et al. in:|author=
(help) - ^ V. Blank; et al. (1998). "Ultrahard and superhard phases of fullerite C60: comparison with diamond on hardness and wear" (PDF). Diamond and Related Materials. 7: 427. doi:10.1016/S0925-9635(97)00232-X.
{{cite journal}}
: Explicit use of et al. in:|author=
(help) - ^ E. Ekimov; et al. (2004). "Superconductivity in diamond" (PDF). Nature. 428: 542. doi:10.1038/nature02449.
{{cite journal}}
: Explicit use of et al. in:|author=
(help) - ^ Catledge, Shane A. (1999), "Effect of nitrogen addition on the microstructure and mechanical properties of diamond films grown using high-methane concentrations", Journal of Applied Physics, 86: 698, doi:10.1063/1.370787
- ^ L. Wei; et al. "Thermal conductivity of isotopically modified single crystal diamond". Phys. Rev. Lett. 70: 3764. doi:10.1103/PhysRevLett.70.3764.
{{cite journal}}
: Explicit use of et al. in:|author=
(help); Unknown parameter|yearl=
ignored (help) - ^ R. T. Coelho; et al. (1995). "The application of polycrystalline diamond (PCD) tool materials when drilling and reaming aluminum-based alloys including MMC". International journal of machine tools & manufacture. 35: 761. doi:10.1016/0890-6955(95)93044-7.
{{cite journal}}
: Explicit use of et al. in:|author=
(help) - ^ W. Ahmed; et al. (2003). "Diamond films grown on cemented WC-Co dental burs using an improved CVD method". Diamond and Related Materials. 12: 1300. doi:10.1016/S0925-9635(03)00074-8.
{{cite journal}}
: Explicit use of et al. in:|author=
(help) - ^ "CVD Thick-Film Diamond Heat Spreaders". Retrieved 2009-05-05.
- ^ US Patent 6924170 - Diamond-silicon hybrid integrated heat spreader
- ^ S. Koizumi, C. E. Nebel and M. Nesladek (2008). Physics and Applications of CVD Diamond. Wiley VCH. ISBN 3527408010.
- ^ A. Denisenko and E. Kohn (2005). "Diamond power devices. Concepts and limits". Diamond and Related Materials. 14: 491. doi:10.1016/j.diamond.2004.12.043.
- ^ S. Koizumi; et al. (2001). "Ultraviolet Emission from a Diamond pn Junction". Science. 292: 1899. doi:10.1126/science.1060258.
{{cite journal}}
: Explicit use of et al. in:|author=
(help) - ^ a b J. Isberg; et al. (2002). "High Carrier Mobility in Single-Crystal Plasma-Deposited Diamond". Science. 297: 5587. doi:10.1126/science.1074374.
{{cite journal}}
: Explicit use of et al. in:|author=
(help) - ^ M. Panizza and G. Cerisola (2005). "Application of diamond electrodes to electrochemical processes". Electrochimica Acta. 51: 191. doi:10.1016/j.electacta.2005.04.023.
- ^ C. E. Nebel; et al. (2007). "Inhomogeneous DNA bonding to polycrystalline CVD diamond". 16: 1648. doi:10.1016/j.diamond.2007.02.015 Diamond and Related Materials.
{{cite journal}}
: Check|doi=
value (help); Cite journal requires|journal=
(help); Explicit use of et al. in:|author=
(help) - ^ D. Gandini, E. Mahé, P.A. Michaud, W. Haenni, A. Perret, Ch. Comninellis (2000). "Oxidation of carbonylic acids at boron-doped diamond electrodes for wastewater treatment". Journal of Applied Electrochemistry. 20: 1345. doi:10.1023/A:1026526729357.
{{cite journal}}
: CS1 maint: multiple names: authors list (link) - ^ P.A. Michaud, E. Mahé, W. Haenni, A. Perret, Ch. Comninellis (2000). "Preparation of peroxodisulfuric acid using Boron-Doped Diamond thin film electrodes". Electrochemical and Solid-State Letters. 3: 77. doi:10.1149/1.1390963.
{{cite journal}}
: CS1 maint: multiple names: authors list (link) - ^ T. A. Railkar; et al. (2000). "A critical review of chemical vapor-deposited (CVD) diamond for electronic applications". Critical Reviews in Solid State and Materials Sciences. 25: 163. doi:10.1080/10408430008951119.
{{cite journal}}
: Explicit use of et al. in:|author=
(help) - ^ M. Bucciolini (2005). "Diamond dosimetry: Outcomes of the CANDIDO and CONRADINFN projects". Nuclear Instruments and Methods in Physics Research A. 552: 189. doi:10.1016/j.nima.2005.06.030.
- ^ "Blind to the Optical Light Detectors". Retrieved 2009-05-05.
- ^ A. BenMoussa; et al. (2008). "New developments on diamond photodetector for VUV Solar Observations". Semiconductor Science and Technology. 23: 035026. doi:10.1088/0268-1242/23/3/035026.
{{cite journal}}
: Explicit use of et al. in:|author=
(help) - ^ J. Heartwig; et al. "Diamonds for Modern Synchrotron Radiation Sources". Retrieved 2009-05-05.
{{cite web}}
: Explicit use of et al. in:|author=
(help) - ^ A. M. Khounsary; et al. "Diamond Monochromator for High Heat Flux Synchrotron X-ray Beams". Retrieved 2009-05-05.
{{cite web}}
: Explicit use of et al. in:|author=
(help) - ^ "Magnetic susceptibility measurements at high pressure using designer diamond anvils". Rev. Sci. Instrum. 74: 2467. 2003. doi:10.1063/1.1544084.
{{cite journal}}
: Unknown parameter|name=
ignored (help) - ^ a b Yarnell, Amanda (February 2, 2004). "The many facets of man-made diamonds". Chemical and Engineering News. American Chemical Society. pp. 26–31. Retrieved 2009-05-04.
- ^ J. Walker (1979). "Optical absorption and luminescence in diamond". Rep. Prog. Phys. 42: 1605. doi:10.1088/0034-4885/42/10/001.
Further reading
- Hazen, Robert M (1992). The New Alchemists. Random House, New York: Times Books. pp. 286 pages. ISBN 0812922751.
{{cite book}}
:|format=
requires|url=
(help); Unknown parameter|subtitle=
ignored (help) - Laboratory-grown Diamonds (Second ed.). Vancouver, Canada: Gemology Headquarters International (GHI). 2007.
{{cite book}}
: Unknown parameter|coauthors=
ignored (|author=
suggested) (help); Unknown parameter|subtitle=
ignored (help)
External links

- "High-pressure web server (free-download HPHT literature)".
- "The New Diamond Age". Wired Magazine (11.09). 2003.
{{cite journal}}
: Unknown parameter|auhor=
ignored (|author=
suggested) (help) - "The Many Facets of Man-Made Diamonds". Chemical and Engineering News. 82: 26-31. 2004.
{{cite journal}}
: Unknown parameter|authore=
ignored (help) - "Carnegie Institution's Geophysical Laboratory". Retrieved 2009-05-05.
- "Putting the Squeeze on Materials". Retrieved 2009-05-05.
- easyLab "An Introduction to Diamond Anvil High Pressure Cells". Retrieved 2009-05-05.
{{cite web}}
: Check|url=
value (help)