Artificial organ
![]() | This article needs to be updated.(September 2016) |
An artificial organ is a human made organ device or tissue that is implanted or integrated into a human — interfacing with living tissue — to replace a natural organ, to duplicate or augment a specific function or functions so the patient may return to a normal life as soon as possible.[1] The replaced function does not have to be related to life support, but it often is. For example, replacement bones and joints, such as those found in hip replacements, could also be considered artificial organs.[2][3]
Implied by definition, is that the device must not be continuously tethered to a stationary power supply or other stationary resources such as filters or chemical processing units. (Periodic rapid recharging of batteries, refilling of chemicals, and/or cleaning/replacing of filters would exclude a device from being called an artificial organ.)[4] Thus, a dialysis machine, while a very successful and critically important life support device that almost completely replaces the duties of a kidney, is not an artificial organ.
Purpose[edit]
Constructing and installing artificial organs, an extremely research-intensive and expensive process initially, may entail many years of ongoing maintenance services not needed by a natural organ:[1][4][5][6]
- providing life support to prevent imminent death while awaiting a transplant (e.g. artificial heart);
- dramatically improving the patient's ability for self care (e.g. artificial limb);
- improving the patient's ability to interact socially (e.g. cochlear implant); or
- improving a patient's quality of life through cosmetic restoration after cancer surgery or an accident.
The use of any artificial organ by humans is almost always preceded by extensive experiments with animals.[7][8][9] Initial testing in humans is frequently limited to those either already facing death or who have exhausted every other treatment possibility.
Examples[edit]
Artificial limbs[edit]

Artificial arms and legs, or prosthetics, are intended to restore a degree of normal function to amputees. Mechanical devices that allow amputees to walk again or continue to use two hands have probably been in use since ancient times,[10] the most notable one being the simple peg leg. Since then, the development of artificial limbs has progressed rapidly. New plastics and other materials, such as carbon fiber have allowed artificial limbs to become stronger and lighter, limiting the amount of extra energy necessary to operate the limb. Additional materials have allowed artificial limbs to look much more realistic.[11] Prostheses can roughly be categorized as upper- and lower-extremity and can take many shapes and sizes.
New advances in artificial limbs include additional levels of integration with the human body. Electrodes can be placed into nervous tissue, and the body can be trained to control the prosthesis. This technology has been used in both animals and humans.[12] The prosthetic can be controlled by the brain using a direct implant or implant into various muscles.[13]
Bladder[edit]
The two main methods for replacing bladder function involve either redirecting urine flow or replacing the bladder in situ.[14] Standard methods for replacing the bladder involve fashioning a bladder-like pouch from intestinal tissue.[14] As of 2017 methods to grow bladders using stem cells had been attempted in clinical research but this procedure was not part of medicine.[15][16]
Brain[edit]

Neural prostheses are a series of devices that can substitute a motor, sensory or cognitive modality that might have been damaged as a result of an injury or a disease.
Neurostimulators, including deep brain stimulators, send electrical impulses to the brain in order to treat neurological and movement disorders, including Parkinson's disease, epilepsy, treatment resistant depression, and other conditions such as urinary incontinence. Rather than replacing existing neural networks to restore function, these devices often serve by disrupting the output of existing malfunctioning nerve centers to eliminate symptoms.[17][18][19]
Scientists in 2013 created a mini brain that developed key neurological components until the early gestational stages of fetal maturation.[20]
Corpora cavernosa[edit]
To treat erectile dysfunction, both corpora cavernosa can be irreversibly surgically replaced with manually inflatable penile implants. This is a drastic therapeutic surgery meant only for men who have complete impotence who have resisted all other treatment approaches. An implanted pump in the (groin) or (scrotum) can be manipulated by hand to fill these artificial cylinders, normally sized to be direct replacements for the natural corpora cavernosa, from an implanted reservoir in order to achieve an erection.[21]
Ear[edit]

In cases when a person is profoundly deaf or severely hard of hearing in both ears, a cochlear implant may be surgically implanted. Cochlear implants bypass most of the peripheral auditory system to provide a sense of sound via a microphone and some electronics that reside outside the skin, generally behind the ear. The external components transmit a signal to an array of electrodes placed in the cochlea, which in turn stimulates the cochlear nerve.[22]
In the case of an outer ear trauma, a craniofacial prosthesis may be necessary.
Thomas Cervantes and his colleagues, who are from Massachusetts General Hospital, built an artificial ear from sheep cartilage by a 3D printer. With a lot of calculations and models, they managed to build an ear shaped like a typical human one. Modeled by a plastic surgeon, they had to adjust several times so the artificial ear can have curves and lines just like a human ear. The researchers said "The technology is now under development for clinical trials, and thus we have scaled up and redesigned the prominent features of the scaffold to match the size of an adult human ear and to preserve the aesthetic appearance after implantation." Their artificial ears have not been announced as successful, but they are still currently developing the project. Each year, thousands of children were born with a congenital deformity called microtia, where the external ear does not fully develop. This could be a major step forward in medical and surgical microtia treatment.
Eye[edit]
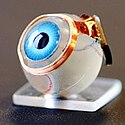
The most successful function-replacing artificial eye so far is actually an external miniature digital camera with a remote unidirectional electronic interface implanted on the retina, optic nerve, or other related locations inside the brain. The present state of the art yields only partial functionality, such as recognizing levels of brightness, swatches of color, and/or basic geometric shapes, proving the concept's potential.[23]
Various researchers have demonstrated that the retina performs strategic image preprocessing for the brain. The problem of creating a completely functional artificial electronic eye is even more complex. Advances towards tackling the complexity of the artificial connection to the retina, optic nerve, or related brain areas, combined with ongoing advances in computer science, are expected to dramatically improve the performance of this technology.
Heart[edit]
Cardiovascular-related artificial organs are implanted in cases where the heart, its valves, or another part of the circulatory system is in disorder. The artificial heart is typically used to bridge the time to heart transplantation, or to permanently replace the heart in case heart transplantation is impossible. Artificial pacemakers represent another cardiovascular device that can be implanted to either intermittently augment (defibrillator mode), continuously augment, or completely bypass the natural living cardiac pacemaker as needed. Ventricular assist devices are another alternative, acting as mechanical circulatory devices that partially or completely replace the function of a failing heart, without the removal of the heart itself.[24]
Besides these, lab-grown hearts and 3D bioprinted hearts are also being researched.[25][unreliable source?][26] Currently, scientists are limited in their ability to grow and print hearts due to difficulties in getting blood vessels and lab-made tissues to function cohesively.[27]
Liver[edit]
HepaLife is developing a bioartificial liver device intended for the treatment of liver failure using stem cells. The artificial liver is designed to serve as a supportive device, either allowing the liver to regenerate upon failure, or to bridge the patient's liver functions until transplant is available.[28] It is only made possible by the fact that it uses real liver cells (hepatocytes), and even then, it is not a permanent substitute.
Researchers from Japan found that a mixture of human liver precursor cells (differentiated from human induced pluripotent stem cells [iPSCs]) and two other cell types can spontaneously form three-dimensional structures dubbed "liver buds."[29]
Lungs[edit]

With some almost fully functional, artificial lungs promise to be a great success in the near future.[30] An Ann Arbor company MC3 is currently working on this type of medical device.
Extracorporeal membrane oxygenation (ECMO) can be used to take significant load off of the native lung tissue and heart. In ECMO, one or more catheters are placed into the patient and a pump is used to flow blood over hollow membrane fibers, which exchange oxygen and carbon dioxide with the blood. Similar to ECMO, Extracorporeal CO2 Removal (ECCO2R) has a similar set-up, but mainly benefits the patient through carbon dioxide removal, rather than oxygenation, with the goal of allowing the lungs to relax and heal.[31]
Ovaries[edit]
The ground work for the development of the artificial ovary was laid in the early 1990s.[32]
Reproductive age patients who develop cancer often receive chemotherapy or radiation therapy, which damages oocytes and leads to early menopause. An artificial human ovary has been developed at Brown University[33] with self-assembled microtissues created using novel 3-D petri dish technology. In a study funded and conducted by the NIH in 2017, scientists were successful in printing 3-D ovaries and implanting them in sterile mice.[34] In the future, scientists hope to replicate this in larger animals as well as humans.[9] The artificial ovary will be used for the purpose of in vitro maturation of immature oocytes and the development of a system to study the effect of environmental toxins on folliculogenesis.
Pancreas[edit]
An artificial pancreas is used to substitute endocrine functionality of a healthy pancreas for diabetic and other patients who require it. It can be used to improve insulin replacement therapy until glycemic control is practically normal as evident by the avoidance of the complications of hyperglycemia, and it can also ease the burden of therapy for the insulin-dependent. Approaches include using an insulin pump under closed loop control, developing a bio-artificial pancreas consisting of a biocompatible sheet of encapsulated beta cells, or using gene therapy.[35][36]
Red blood cells[edit]
Artificial red blood cells (RBC) have already been in projects for about 60 years, but they started getting interest when the HIV-contaminated-donor blood crisis. Artificial RBCs will be dependent 100% on nanotechnology. A successful artificial RBC should be able to totally replace human RBC, which means it can carry on all the functions that a human RBC does.
The first artificial RBC, made by Chang and Poznanski in 1968, was made to transport Oxygen and Carbon Dioxide, also fulfilled antioxidant functions.[37]
Scientists are working on a new kind of artificial RBC, which is one-fiftieth the size of a human RBC. They are made from purified human hemoglobin proteins that have been coated with a synthetic polymer. Thanks to the special materials of the artificial RBC, they can capture oxygen when blood pH is high, and release oxygen when blood pH is low. The polymer coating also keeps the hemoglobin from reacting with nitric oxide in the bloodstream, thus preventing dangerous constriction of the blood vessels. Allan Doctor, MD, stated that the artificial RBC can be used by anyone, with any blood type because the coating is immune silent.[38]
Testes[edit]
Men whom have sustained testicular abnormalities through birth defects or injury have been able to replace the damaged testicle with a testicular prosthesis.[39] Although the prosthetic does not restore biological reproductive function, the device has been shown to improve mental health for these patients.[40]
Thymus[edit]
An implantable machine that performs the function of a thymus does not exist. However, researchers have been able to grow a thymus from reprogrammed fibroblasts. They expressed hope that the approach could one day replace or supplement neonatal thymus transplantation.[41]
As of 2017, researchers at UCLA developed an artificial thymus that, although not yet implantable, is capable of performing all functions of a true thymus.[42]
The artificial thymus would play an important role in the immune system, and it would use blood stem cells to produce more T cells, which in turn, help the body fight infections. It would ultimately give the body a better ability to fight cancer cells. As people age, if their thymus stops working well, an artificial thymus could also be a potentially viable option.
The idea of using T cells to fight against infections has been around for a time, but until recently, the idea of using a T cell source, an artificial thymus is proposed. "We know that the key to creating a consistent and safe supply of cancer-fighting T cells would be to control the process in a way that deactivates all T cell receptors in the transplanted cells, except for the cancer-fighting receptors," said Dr. Gay Crooks of UCLA.[43] The scientist also found that the T cells produced by the artificial thymus carried a diverse range of T cell receptors and worked similarly to the T cells produced by a normal thymus. Since they can work like human thymus, artificial thymus can supply a consistent amount of T cells to the body for the patients who are in need of treatments.
Trachea[edit]
The field of artificial tracheas went through a period of high interest and excitement with the work of Paolo Macchiarini at the Karolinska Institute and elsewhere from 2008 to around 2014, with front-page coverage in newspapers and on television. Concerns were raised about his work in 2014 and by 2016 he had been fired and high level management at Karolinska had been dismissed, including people involved in the Nobel Prize.[44][45]
As of 2017 engineering a trachea—a hollow tube lined with cells—had proved more challenging than originally thought; challenges include the difficult clinical situation of people who present as clinical candidates, who generally have been through multiple procedures already; creating an implant that can become fully developed and integrate with host while withstanding respiratory forces, as well as the rotational and longitudinal movement the trachea undergoes.[46]
Enhancement[edit]
It is also possible to construct and install an artificial organ to give its possessor abilities that are not naturally occurring. Research is proceeding in areas of vision, memory, and information processing. Some current research focuses on restoring short-term memory in accident victims and long-term memory in dementia patients.
One area of success was achieved when Kevin Warwick carried out a series of experiments extending his nervous system over the internet to control a robotic hand and the first direct electronic communication between the nervous systems of two humans.[47]
This might also include the existing practice of implanting subcutaneous chips for identification and location purposes (ex. RFID tags).[48]
Microchips[edit]
Organ chips are devices containing hollow microvessels filled with cells simulating tissue and/or organs as a microfluidic system that can provide key chemical and electrical signal information.[49] This is distinct from an alternative use of the term microchip, which refers to small, electronic chips that are commonly used as an identifier and can also contain a transponder.
This information can create various applications such as creating "human in vitro models" for both healthy and diseased organs, drug advancements in toxicity screening as well as replacing animal testing.[49]
Using 3D cell culture techniques enables scientists to recreate the complex extracellular matrix, ECM, found in in vivo to mimic human response to drugs and human diseases.[50] Organs on chips are used to reduce the failure rate in new drug development; microengineering these allows for a microenvironment to be modeled as an organ.
See also[edit]
References[edit]
- ^ a b Catapano G, Verkerke GJ (2012). "Chapter 2: Artificial Organs". In Abu-Faraj ZO (ed.). Handbook of Research on Biomedical Engineering Education and Advanced Bioengineering Learning: Interdisciplinary Concepts - Volume 1. Hershey, PA: Medical Information Science Reference. pp. 60–95. ISBN 9781466601239. Retrieved 16 March 2016.
- ^ Gebelein CG (1984). "Chapter 1: The Basics of Artificial Organs". In Gebelein CG (ed.). Polymeric Materials and Artificial Organs. ACS Symposium Series. Vol. 256. Washington, DC: American Chemical Society. pp. 1–11. doi:10.1021/bk-1984-0256.ch001. ISBN 9780841208544.
- ^ "Artificial Organs". Reference.MD. RES, Inc. 6 June 2012. Retrieved 16 March 2016.
- ^ a b Tang R (1998). "Artificial Organs". BIOS. 69 (3): 119–122. JSTOR 4608470.
- ^ Fountain H (15 September 2012). "A First: Organs Tailor-Made With Body's Own Cells". The New York Times. Retrieved 16 March 2016.
- ^ Mussivand T, Kung RT, McCarthy PM, Poirier VL, Arabia FA, Portner P, Affeld K (May 1997). "Cost effectiveness of artificial organ technologies versus conventional therapy". ASAIO Journal. 43 (3): 230–236. doi:10.1097/00002480-199743030-00021. PMID 9152498.
- ^ "Why are animals used for testing medical products?". FDA.org. Food and Drug Administration. 4 March 2016. Retrieved 16 March 2016.
- ^ Giardino R, Fini M, Orienti L (February 1997). "Laboratory animals for artificial organ evaluation". The International Journal of Artificial Organs. 20 (2): 76–80. doi:10.1177/039139889702000205. PMID 9093884. S2CID 42808335.
- ^ a b "A bioprosthetic ovary created using 3D printed microporous scaffolds restores ovarian function in sterilized mice". NIH. May 2017. Retrieved 30 January 2018.
- ^ Finch J (February 2011). "The ancient origins of prosthetic medicine". Lancet. 377 (9765): 548–549. doi:10.1016/s0140-6736(11)60190-6. PMID 21341402. S2CID 42637892.
- ^ "Artificial Limb". How Products Are Made. Advameg, Inc. Retrieved 16 March 2016.
- ^ "Motorlab - Multimedia". Archived from the original on 2019-08-01. Retrieved 2016-05-01.
- ^ "Targeted Muscle Reinnervation: Control Your Prosthetic Arm With Thought". Archived from the original on 2017-01-14. Retrieved 2016-05-01.
- ^ a b "Urinary Diversion". National Institute of Diabetes and Digestive and Kidney Diseases. September 2013.
- ^ Adamowicz J, Pokrywczynska M, Van Breda SV, Kloskowski T, Drewa T (November 2017). "Concise Review: Tissue Engineering of Urinary Bladder; We Still Have a Long Way to Go?". Stem Cells Translational Medicine. 6 (11): 2033–2043. doi:10.1002/sctm.17-0101. PMC 6430044. PMID 29024555.
- ^ Iannaccone PM, Galat V, Bury MI, Ma YC, Sharma AK (January 2018). "The utility of stem cells in pediatric urinary bladder regeneration". Pediatric Research. 83 (1–2): 258–266. doi:10.1038/pr.2017.229. PMID 28915233. S2CID 4433348.
- ^ Wong JY, Bronzino JD, Peterson DR, eds. (2012). Biomaterials: Principles and Practices. Boca Raton, FL: CRC Press. p. 281. ISBN 9781439872512. Retrieved 16 March 2016.
- ^ "Download Product Code Classification Files". FDA.org/medicaldevices. Food and Drug Administration. 4 November 2014. Retrieved 16 March 2016.
Relevant info in the foiclass.zip file.
- ^ McLatchie G, Borley N, Chikwe J, eds. (2013). Oxford Handbook of Clinical Surgery. Oxford, UK: OUP Oxford. p. 794. ISBN 9780199699476. Retrieved 16 March 2016.
- ^ Poutintsev F (2018-08-20). "Artificial Organs — The Future of Transplantation". Immortality Foundation. Retrieved 2019-09-15.
- ^ Simmons M, Montague DK (2008). "Penile prosthesis implantation: past, present and future". International Journal of Impotence Research. 20 (5): 437–444. doi:10.1038/ijir.2008.11. PMID 18385678.
- ^ "Cochlear Implants". NIH Publication No. 11-4798. National Institute on Deafness and Other Communication Disorders. February 2016. Retrieved 16 March 2016.
- ^ Geary J (2002). The Body Electric. Rutgers University Press. p. 214. ISBN 9780813531946. Retrieved 16 March 2016.
- ^ Birks EJ, Tansley PD, Hardy J, George RS, Bowles CT, Burke M, et al. (November 2006). "Left ventricular assist device and drug therapy for the reversal of heart failure". The New England Journal of Medicine. 355 (18): 1873–1884. doi:10.1056/NEJMoa053063. PMID 17079761.
- ^ "Researchers Can Now 3D Print A Human Heart Using Biological Material". IFLScience. 26 October 2015.
- ^ Hinton TJ, Jallerat Q, Palchesko RN, Park JH, Grodzicki MS, Shue HJ, et al. (October 2015). "Three-dimensional printing of complex biological structures by freeform reversible embedding of suspended hydrogels". Science Advances. 1 (9): e1500758. Bibcode:2015SciA....1E0758H. doi:10.1126/sciadv.1500758. PMC 4646826. PMID 26601312.
- ^ Ferris R (27 March 2017). "Scientists grew beating human heart tissue on spinach leaves". CNBC.
- ^ "Artificial Liver". HepaLife. Archived from the original on 15 June 2008. Retrieved 2 June 2008.
- ^ Takebe T, Sekine K, Enomura M, Koike H, Kimura M, Ogaeri T, et al. (July 2013). "Vascularized and functional human liver from an iPSC-derived organ bud transplant". Nature. 499 (7459): 481–484. Bibcode:2013Natur.499..481T. doi:10.1038/nature12271. PMID 23823721. S2CID 4423004.
- ^ Ota K (April 2010). "Advances in artificial lungs". Journal of Artificial Organs. 13 (1): 13–16. doi:10.1007/s10047-010-0492-1. PMID 20177723. S2CID 21002242.
- ^ Terragni PP, Birocco A, Faggiano C, Ranieri VM (2010). "Extracorporeal CO2 removal". Contributions to Nephrology. 165: 185–196. doi:10.1159/000313758. hdl:2318/75212. ISBN 978-3-8055-9472-1. PMID 20427969.
- ^ Gosden RG (July 1990). "Restitution of fertility in sterilized mice by transferring primordial ovarian follicles". Human Reproduction. 5 (5): 499–504. doi:10.1093/oxfordjournals.humrep.a137132. PMID 2394782.
- ^ Krotz SP, Robins J, Moore R, Steinhoff MM, Morgan J, Carson SA (September 2008). "Model artificial human ovary by pre-fabricated cellular self-assembly". Fertility and Sterility. 90: S273. doi:10.1016/j.fertnstert.2008.07.1166.
- ^ Laronda MM, Rutz AL, Xiao S, Whelan KA, Duncan FE, Roth EW, et al. (May 2017). "A bioprosthetic ovary created using 3D printed microporous scaffolds restores ovarian function in sterilized mice". Nature Communications. 8: 15261. Bibcode:2017NatCo...815261L. doi:10.1038/ncomms15261. PMC 5440811. PMID 28509899.
- ^ "Artificial Pancrease". JDRF. 9 February 2011. Retrieved 16 March 2016.
- ^ "Collaborative Efforts Key to Catalyzing Creation of an Artificial Pancreas". National Institute of Diabetes and Digestive and Kidney Diseases. 1 March 2014. Archived from the original on 23 March 2016. Retrieved 16 March 2016.
- ^ Chang TM (June 2012). "From artificial red blood cells, oxygen carriers, and oxygen therapeutics to artificial cells, nanomedicine, and beyond". Artificial Cells, Blood Substitutes, and Immobilization Biotechnology. 40 (3): 197–199. doi:10.3109/10731199.2012.662408. PMC 3566225. PMID 22409281.
- ^ Guo J, Agola JO, Serda R, Franco S, Lei Q, Wang L, et al. (July 2020). "Biomimetic Rebuilding of Multifunctional Red Blood Cells: Modular Design Using Functional Components". ACS Nano. 14 (7): 7847–7859. doi:10.1021/acsnano.9b08714. OSTI 1639054. PMID 32391687. S2CID 218584795.
- ^ "Testicular Implants". The Men's Clinic. Urology at UCLA. Retrieved 2019-09-15.
- ^ "Testicular Implants". Cleveland Clinic. Retrieved 2019-09-15.
- ^ Bredenkamp N, Ulyanchenko S, O'Neill KE, Manley NR, Vaidya HJ, Blackburn CC (September 2014). "An organized and functional thymus generated from FOXN1-reprogrammed fibroblasts". Nature Cell Biology. 16 (9): 902–908. doi:10.1038/ncb3023. PMC 4153409. PMID 25150981.
- ^ Kumar K (2017-04-12). "Meet The Bionic Thymus: The Artificial Organ For Pumping T Cells For Cancer Treatment". Tech Times. Retrieved 2019-09-15.
- ^ "Artificial thymus developed at UCLA can produce cancer-fighting T cells from blood stem cells". David Geffen School of Medicine - University of California, Los Angeles, CA. Retrieved 2020-12-19.
- ^ Astakhova A (16 May 2017). "Superstar surgeon fired, again, this time in Russia". Science. doi:10.1126/science.aal1201.
- ^ Dobrynin S, Recknagel C (February 6, 2017). "From Confines Of Russia, Controversial Stem-Cell Surgeon Tries To Weather Scandal". RadioFreeEurope/RadioLiberty.
- ^ Den Hondt M, Vranckx JJ (February 2017). "Reconstruction of defects of the trachea". Journal of Materials Science. Materials in Medicine. 28 (2): 24. doi:10.1007/s10856-016-5835-x. PMID 28070690. S2CID 21814105.
- ^ Warwick K, Gasson M, Hutt B, Goodhew I, Kyberd P, Schulzrinne H, Wu X (2004). "Thought communication and control: a first step using radiotelegraphy". IEE Proceedings - Communications. 151 (3): 185. doi:10.1049/ip-com:20040409.
- ^ Foster KR, Jaeger J (August 2008). "Ethical implications of implantable radiofrequency identification (RFID) tags in humans". The American Journal of Bioethics. 8 (8): 44–48. doi:10.1080/15265160802317966. PMID 18802863. S2CID 27093558.
- ^ a b Zheng F, Fu F, Cheng Y, Wang C, Zhao Y, Gu Z (May 2016). "Organ-on-a-Chip Systems: Microengineering to Biomimic Living Systems". Small. 12 (17): 2253–2282. doi:10.1002/smll.201503208. PMID 26901595. S2CID 395464.
- ^ Prestwich, Glenn D. (2008-01-01). "Evaluating Drug Efficacy and Toxicology in Three Dimensions: Using Synthetic Extracellular Matrices in Drug Discovery". Accounts of Chemical Research. 41 (1): 139–148. doi:10.1021/ar7000827. ISSN 0001-4842. PMID 17655274.
Further reading[edit]
- Chang TM (June 2012). "From artificial red blood cells, oxygen carriers, and oxygen therapeutics to artificial cells, nanomedicine, and beyond". Artificial Cells, Blood Substitutes, and Immobilization Biotechnology. 40 (3): 197–199. doi:10.3109/10731199.2012.662408. PMC 3566225. PMID 22409281.
- Foster KR, Jaeger J (August 2008). "Ethical implications of implantable radiofrequency identification (RFID) tags in humans". The American Journal of Bioethics. 8 (8): 44–48. doi:10.1080/15265160802317966. PMID 18802863. S2CID 27093558.
- Liu Y, Yang R, He Z, Gao WQ (2013). "Generation of functional organs from stem cells". Cell Regeneration. 2 (1): 2:1. doi:10.1186/2045-9769-2-1. PMC 4230490. PMID 25408873.
- Tran J (2015). "To Bioprint or Not to Bioprint". North Carolina Journal of Law and Technology. 17: 123–78. SSRN 2562952.