Marine viruses: Difference between revisions
Epipelagic (talk | contribs) →Archaeal viruses: further |
Epipelagic (talk | contribs) →Eukaryote viruses: marine protists |
||
Line 76: | Line 76: | ||
[[File:Origin of eukaryotic viruses.webp|thumb|upright=1.5|left| The second melting pot of virus evolution: origin of eukaryotic viruses. Characteristic images of archaeal, bacterial, and eukaryotic viruses are shown.<ref name=Koonin2006 />]] |
[[File:Origin of eukaryotic viruses.webp|thumb|upright=1.5|left| The second melting pot of virus evolution: origin of eukaryotic viruses. Characteristic images of archaeal, bacterial, and eukaryotic viruses are shown.<ref name=Koonin2006 />]] |
||
; Marine protists |
|||
By 2015, about 40 viruses affecting [[marine protists]] had been isolated and examined, most of them viruses of microalgae.<ref>Tomaru Y, Kimura K and Nagasaki K (2015) [https://books.google.co.nz/books?id=vgyhCgAAQBAJ&pg=PA502&dq=%22Marine+Protist+Viruses%22&hl=en&newbks=1&newbks_redir=0&sa=X&ved=0ahUKEwjHksvVmurpAhWXIbcAHd3pDpEQ6AEIKDAA#v=onepage&q=%22Marine%20Protist%20Viruses%22&f=false "Marine Protist Viruses"]. In: Ohtsuka S, Suzaki T, Horiguchi T, Suzuki N, Not F (eds) ''Marine Protists'' pages 501–517. Springer, Tokyo. {{doi|10.1007/978-4-431-55130-0_20}}. {{ISBN|978-4-431-55130-0}}.</ref> The genomes of these marine protist viruses are highly diverse.<ref>Hyman, P. and Abedon, S.T. (2012) "Smaller fleas: viruses of microorganisms". ''Scientifica'', . {{doi|10.6064/2012/734023}}. [[File:CC-BY icon.svg|50px]] Material was copied from this source, which is available under a [https://creativecommons.org/licenses/by/3.0/ Creative Commons Attribution 3.0 International License]. |
|||
</ref><ref>Short, S.M. (2012) "The ecology of viruses that infect eukaryotic algae". ''Environmental microbiology'', '''14'''(9): 2253–2271. {{doi|10.1111/j.1462-2920.2012.02706.x}}.</ref> |
|||
; Marine invertebrates |
|||
[[Marine invertebrates]] are suscepible to viral diseases.<ref>TJohnson, P.T. (1984) "Viral diseases of marine invertebrates". ''Helgoländer Meeresuntersuchungen'', '''37'''(1-4): 65–98. {{doi|10.1007/BF01989296}}.</ref><ref>Renault T (2011) [https://books.google.com/books?hl=en&lr=&id=8uXDYcIf1fwC&oi=fnd&pg=PA153&dq=Viruses+infecting+marine+molluscs&ots=ewtZFuDD7X&sig=_LUTUTSCkU0L4ugAw4ukdUpip8A#v=onepage&q=Viruses%20infecting%20marine%20molluscs&f=false "Viruses infecting marine molluscs"] In: Hurst CJ (Ed) ''Studies in Viral Ecology'', Volume 2: Animal Host Systems, John Wiley & Sons. {{ISBN|9781118024584}}.</ref><ref>Arzul, I., Corbeil, S., Morga, B. and Renault, T., 2017. Viruses infecting marine molluscs. Journal of invertebrate pathology, 147, pp.118-135. {{doi|10.1016/j.jip.2017.01.009}}.</ref> [[Sea star wasting disease]] is a disease of [[starfish]] and several other echinoderms that appears sporadically, causing mass mortality of those affected.<ref>Dawsoni, Solaster. "Sea Star Species Affected by Wasting Syndrome." Pacificrockyintertidal.org Seastarwasting.org (n.d.): n. pag. ''Ecology and Evolutionary Biology''. Web.</ref> There are around 40 different species of sea stars that have been affected by this disease. In 2014 it was suggested that the disease is associated with a single-stranded DNA virus now known as the [[sea star-associated densovirus]] (SSaDV); however, sea star wasting disease is not fully understood.<ref name=MARINe2018>{{Cite web|url=https://www.eeb.ucsc.edu/pacificrockyintertidal/data-products/sea-star-wasting/|title=Sea Star Wasting Syndrome {{!}} MARINe|website=eeb.ucsc.edu|language=en|access-date=2018-06-03}}</ref> |
[[Marine invertebrates]] are suscepible to viral diseases.<ref>TJohnson, P.T. (1984) "Viral diseases of marine invertebrates". ''Helgoländer Meeresuntersuchungen'', '''37'''(1-4): 65–98. {{doi|10.1007/BF01989296}}.</ref><ref>Renault T (2011) [https://books.google.com/books?hl=en&lr=&id=8uXDYcIf1fwC&oi=fnd&pg=PA153&dq=Viruses+infecting+marine+molluscs&ots=ewtZFuDD7X&sig=_LUTUTSCkU0L4ugAw4ukdUpip8A#v=onepage&q=Viruses%20infecting%20marine%20molluscs&f=false "Viruses infecting marine molluscs"] In: Hurst CJ (Ed) ''Studies in Viral Ecology'', Volume 2: Animal Host Systems, John Wiley & Sons. {{ISBN|9781118024584}}.</ref><ref>Arzul, I., Corbeil, S., Morga, B. and Renault, T., 2017. Viruses infecting marine molluscs. Journal of invertebrate pathology, 147, pp.118-135. {{doi|10.1016/j.jip.2017.01.009}}.</ref> [[Sea star wasting disease]] is a disease of [[starfish]] and several other echinoderms that appears sporadically, causing mass mortality of those affected.<ref>Dawsoni, Solaster. "Sea Star Species Affected by Wasting Syndrome." Pacificrockyintertidal.org Seastarwasting.org (n.d.): n. pag. ''Ecology and Evolutionary Biology''. Web.</ref> There are around 40 different species of sea stars that have been affected by this disease. In 2014 it was suggested that the disease is associated with a single-stranded DNA virus now known as the [[sea star-associated densovirus]] (SSaDV); however, sea star wasting disease is not fully understood.<ref name=MARINe2018>{{Cite web|url=https://www.eeb.ucsc.edu/pacificrockyintertidal/data-products/sea-star-wasting/|title=Sea Star Wasting Syndrome {{!}} MARINe|website=eeb.ucsc.edu|language=en|access-date=2018-06-03}}</ref> |
||
; Marine vertebrates |
|||
[[Fish]] are particularly prone to infections with [[rhabdovirus]]es, which are distinct from, but related to rabies virus. At least nine types of rhabdovirus cause economically important diseases in species including salmon, pike, perch, sea bass, carp and cod. The symptoms include anaemia, bleeding, lethargy and a mortality rate that is affected by the temperature of the water. In hatcheries the diseases are often controlled by increasing the temperature to 15–18 °C.<ref name=Murphy1999>{{cite book |author1=Murphy, FA |author2=Gibbs, EPJ |author3=Horzinek, MC |author4=Studdart MJ |title=Veterinary Virology |publisher=Academic Press |location=Boston |year=1999 |isbn=978-0-12-511340-3}}</ref>{{rp|442–443}} Like all vertebrates, fish suffer from [[Herpesviridae|herpes viruses]]. These ancient viruses have co-evolved with their hosts and are highly species-specific.<ref name=Murphy1999 />{{rp|324}} In fish, they cause cancerous tumours and non-cancerous growths called [[hyperplasia]].<ref name=Murphy1999 />{{rp|325}} |
[[Fish]] are particularly prone to infections with [[rhabdovirus]]es, which are distinct from, but related to rabies virus. At least nine types of rhabdovirus cause economically important diseases in species including salmon, pike, perch, sea bass, carp and cod. The symptoms include anaemia, bleeding, lethargy and a mortality rate that is affected by the temperature of the water. In hatcheries the diseases are often controlled by increasing the temperature to 15–18 °C.<ref name=Murphy1999>{{cite book |author1=Murphy, FA |author2=Gibbs, EPJ |author3=Horzinek, MC |author4=Studdart MJ |title=Veterinary Virology |publisher=Academic Press |location=Boston |year=1999 |isbn=978-0-12-511340-3}}</ref>{{rp|442–443}} Like all vertebrates, fish suffer from [[Herpesviridae|herpes viruses]]. These ancient viruses have co-evolved with their hosts and are highly species-specific.<ref name=Murphy1999 />{{rp|324}} In fish, they cause cancerous tumours and non-cancerous growths called [[hyperplasia]].<ref name=Murphy1999 />{{rp|325}} |
||
Revision as of 08:27, 5 June 2020

Part of a series of overviews on |
Marine life |
---|
![]() |
Marine viruses are defined by their habitat as viruses that live in marine environments, that is, in the saltwater of seas or oceans or the brackish water of coastal estuaries. A virus is a small infectious agent that replicates only inside the living cells of another organism. Viruses can infect all types of life forms, from animals and plants to microorganisms, including bacteria and archaea as well as other viruses.[4]
When not inside a cell or in the process of infecting a cell, viruses exist in the form of independent particles. A single viral particle is called a virion. A viron consist of two or three parts: (i) the genetic material (genome) made from either DNA or RNA, long molecules that carry genetic information; (ii) a protein coat called the capsid which surrounds and protects the genetic material. The shapes of these virus particles range from simple helical and icosahedral forms for some virus species to more complex structures for others. Most virus species have virions that are too small to be seen with an optical microscope. The average virion is about one one-hundredth the linear size of the average bacterium.
A teaspoon of seawater typically contains about ten million marine viruses. Most of these viruses are bacteriophages which infect and destroy marine bacteria and control the growth of phytoplankton at the base of the marine food web. Bacteriophages are harmless to plants and animals, but are essential to the regulation of marine ecosystems. They supply key mechanisms for recycling ocean carbon and nutrients. In a process known as the viral shunt, organic molecules released from dead bacterial cells stimulate fresh bacterial and algal growth. In particular the breaking down of bacteria by viruses (lysis) has been shown to enhance nitrogen cycling and stimulate phytoplankton growth. Viral activity also affects the biological pump, the process which sequesters carbon in the deep ocean. By increasing the amount of respiration in the oceans, viruses are indirectly responsible for reducing the amount of carbon dioxide in the atmosphere by approximately 3 gigatonnes of carbon per year
Marine microorganisms contribute about 70% of the total marine biomass. It is estimated marine viruses kill 20% of this biomass every day. Viruses are the main agents responsible for the rapid destruction of harmful algal blooms which often kill other marine life. The number of viruses in the oceans decreases further offshore and deeper into the water, where there are fewer host organisms. Viruses are an important natural means of transferring genes between different species, which increases genetic diversity and drives evolution. It is thought viruses played a central role in early evolution before the diversification of bacteria, archaea and eukaryotes, at the time of the last universal common ancestor of life on Earth. Viruses are still one of the largest areas of unexplored genetic diversity on Earth.
Background
Viruses are now recognised as ancient and as having origins that pre-date the divergence of life into the three domains.[5] They are found wherever there is life and have probably existed since living cells first evolved.[6] The origins of viruses in the evolutionary history of life are unclear because they do not form fossils. Molecular techniques are used to compare the DNA or RNA of viruses and are a useful means of investigating how they arose.[7] Some viruses may have evolved from plasmids—pieces of DNA that can move between cells—while others may have evolved from bacteria. In evolution, viruses are an important means of horizontal gene transfer, which increases genetic diversity.[8]
Opinions differ on whether viruses are a form of life or organic structures that interact with living organisms.[9] They are considered by some to be a life form, because they carry genetic material, reproduce by creating multiple copies of themselves through self-assembly, and evolve through natural selection. However they lack key characteristics such as a cellular structure generally considered necessary to count as life. Because they possess some but not all such qualities, viruses have been described as replicators[10] and as "organisms at the edge of life".[11]
Bacteriophages

Bacteriophages, often just called phages, are viruses that parasite bacteria. Marine phages parasite marine bacteria such as cyanobacteria.[12] They are a diverse group of viruses which are the most abundant biological entity in marine environments, because their hosts, bacteria, are typically the numerically dominant cellular life in the sea. There are up to ten times more phages in the oceans than there are bacteria,[13] reaching levels of 250 million bacteriophages per millilitre of seawater.[14] These viruses infect specific bacteria by binding to surface receptor molecules and then entering the cell. Within a short amount of time, in some cases just minutes, bacterial polymerase starts translating viral mRNA into protein. These proteins go on to become either new virions within the cell, helper proteins, which help assembly of new virions, or proteins involved in cell lysis. Viral enzymes aid in the breakdown of the cell membrane, and there are phages that can replicate three hundred phages twenty minutes after injection.[15]
Bacteria defend themselves from bacteriophages is by producing enzymes that destroy foreign DNA. These enzymes, called restriction endonucleases, cut up the viral DNA that bacteriophages inject into bacterial cells.[16] Bacteria also contain a system that uses CRISPR sequences to retain fragments of the genomes of viruses that the bacteria have come into contact with in the past, which allows them to block the virus's replication through a form of RNA interference.[17][18] This genetic system provides bacteria with acquired immunity to infection.[19]

(b) same image visualised by highlighting the cell wall in orange, the plasma membrane in light yellow, the thylakoid membrane in green, carboxysomes in cyan, the polyphosphate body in blue, adsorbed phages on the sides or top of the cell in red, and cytoplasmic granules (probably mostly ribosomes) in light purple.[20]

interacting with the marine Prochlorococcus MED4 bacterium

Microbes drive the nutrient transformations that sustain Earth’s ecosystems,[21] and the viruses that infect these microbes modulate both microbial population size and diversity.[22][20] The cyanobacterium Prochlorococcus, the most abundant oxygenic phototroph on Earth, contributes a substantial fraction of global primary carbon production, and often reaches densities of over 100,000 cells per milliliter in oligotrophic and temperate oceans.[23] Hence, viral (cyanophage) infection and lysis of Prochlorococcus represent an important component of the global carbon cycle. In addition to their ecological role in inducing host mortality, cyanophages influence the metabolism and evolution of their hosts by co-opting and exchanging genes, including core photosynthesis genes.[20]
For a long time, tailed phages of the order Caudovirales seemed to dominate marine ecosystems in number and diversity of organisms.[12] However, as a result of more recent research, non-tailed viruses appear to be dominant in multiple depths and oceanic regions, followed by the Caudovirales families of myoviruses, podoviruses, and siphoviruses.[24] Phages belonging to the families Corticoviridae,[25] Inoviridae,[26] Microviridae,[27] and Autolykiviridae[28][29][30][31] are also known to infect diverse marine bacteria.
Archaeal viruses
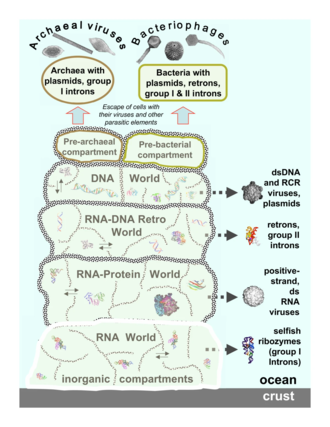
Archaean viruses replicate within archaea: these are double-stranded DNA viruses with unusual and sometimes unique shapes.[32][33] These viruses have been studied in most detail in the thermophilic archaea, particularly the orders Sulfolobales and Thermoproteales.[34] Defences against these viruses involve RNA interference from repetitive DNA sequences within archaean genomes that are related to the genes of the viruses.[35][36] Most archaea have CRISPR–Cas systems as an adaptive defence against viruses. These enable archaea to retain sections of viral DNA, which are then used to target and eliminate subsequent infections by the virus using a process similar to RNA interference.[37]
Fungal viruses
Mycoviruses, also known as mycophages, are viruses that infect fungi. The infection of fungal cells is different from that of animal cells. Fungi have a rigid cell wall made of chitin, so most viruses can get inside these cells only after trauma to the cell wall.[38]
Eukaryote viruses

- Marine protists
By 2015, about 40 viruses affecting marine protists had been isolated and examined, most of them viruses of microalgae.[39] The genomes of these marine protist viruses are highly diverse.[40][41]
- Marine invertebrates
Marine invertebrates are suscepible to viral diseases.[42][43][44] Sea star wasting disease is a disease of starfish and several other echinoderms that appears sporadically, causing mass mortality of those affected.[45] There are around 40 different species of sea stars that have been affected by this disease. In 2014 it was suggested that the disease is associated with a single-stranded DNA virus now known as the sea star-associated densovirus (SSaDV); however, sea star wasting disease is not fully understood.[46]
- Marine vertebrates
Fish are particularly prone to infections with rhabdoviruses, which are distinct from, but related to rabies virus. At least nine types of rhabdovirus cause economically important diseases in species including salmon, pike, perch, sea bass, carp and cod. The symptoms include anaemia, bleeding, lethargy and a mortality rate that is affected by the temperature of the water. In hatcheries the diseases are often controlled by increasing the temperature to 15–18 °C.[47]: 442–443 Like all vertebrates, fish suffer from herpes viruses. These ancient viruses have co-evolved with their hosts and are highly species-specific.[47]: 324 In fish, they cause cancerous tumours and non-cancerous growths called hyperplasia.[47]: 325
In 1984, infectious salmon anemia (ISAv) was discovered in Norway in an Atlantic salmon hatchery. Eighty percent of the fish in the outbreak died. ISAv, a viral disease, is now a major threat to the viability of Atlantic salmon farming.[48] As the name implies, it causes severe anemia of infected fish. Unlike mammals, the red blood cells of fish have DNA, and can become infected with viruses. Management strategies include developing a vaccine and improving genetic resistance to the disease.[49]
Marine mammals are also susceptible to marine viral infections. In 1988 and 2002, thousands of harbour seals were killed in Europe by phocine distemper virus.[50] Many other viruses, including caliciviruses, herpesviruses, adenoviruses and parvoviruses, circulate in marine mammal populations.[51]
Giant marine viruses

Most viruses range in length from about 20 to 300 nanometers. This can be contrasted with the length of bacteria, which starts at about 400 nanometers. There are also giant viruses, often called giruses, typically about 1000 nanometers (one micron) in length. All giant viruses belongto phylum Nucleocytoviricota (NCLDV), together with poxviruses. The largest known of these is Tupanvirus. This genus of giant virus was discovered in 2018 in the deep ocean as well as a soda lake, and can reach up to 2.3 microns in total length.[52]
The discovery and subsequent characterization of giant viruses has triggered some debate concerning their evolutionary origins. The two main hypotheses for their origin are that either they evolved from small viruses, picking up DNA from host organisms, or that they evolved from very complicated organisms into the current form which is not self-sufficient for reproduction.[53] What sort of complicated organism giant viruses might have diverged from is also a topic of debate. One proposal is that the origin point actually represents a fourth domain of life,[54][55] but this has been largely discounted.[56][57]
-
The giant mimivirus
Virophages
Virophages are small, double-stranded DNA viral phages that require the co-infection of another virus. The co-infecting viruses are typically giant viruses. Virophages rely on the viral replication factory of the co-infecting giant virus for their own replication. One of the characteristics of virophages is that they have a parasitic relationship with the co-infecting virus. Their dependence upon the giant virus for replication often results in the deactivation of the giant viruses. The virophage may improve the recovery and survival of the host organism. Unlike satellite viruses, virophages have a parasitic effect on their co-infecting virus. Virophages have been observed to render a giant virus inactive and thereby improve the condition of the host organism.
All known virophages are grouped into the family Lavidaviridae (from "large virus dependent or associated" + -viridae).[59] The first virophage was discovered in a cooling tower in Paris, France in 2008. It was discovered with its co-infecting giant virus, Acanthamoeba castellanii mamavirus (ACMV). The virophage was named Sputnik and its replication relied entirely on the co-infection of ACMV and its cytoplasmic replication machinery. Sputnik was also discovered to have an inhibitory effect on ACMV and improved the survival of the host. Other characterised virophages include Sputnik 2, Sputnik 3, Zamilon and Mavirus.[60][61]
A majority of these virophages are being discovered by analyzing metagenomic data sets. In metagenomic analysis, DNA sequences are run through multiple bioinformatic algorithms which pull out certain important patterns and characteristics. In these data sets are giant viruses and virophages. They are separated by looking for sequences around 17 to 20 kbp long which have similarities to already sequenced virophages. These virophages can have linear or circular double-stranded DNA genomes.[62] Virophages in culture have icosahedral capsid particles that measure around 40 to 80 nanometers long.[63] Virophage particles are so small that electron microscopy must be used to view these particles. Metagenomic sequence-based analyses have been used to predict around 57 complete and partial virophage genomes[64] and in December 2019 to identify 328 high-quality (complete or near-complete) genomes from diverse habitats including the human gut, plant rhizosphere, and terrestrial subsurface, from 27 distinct taxonomic clades.[65]
A giant marine virus CroV infects and causes the death by lysis of the marine zooflagellate Cafeteria roenbergensis.[67] This impacts coastal ecology because Cafeteria roenbergensis feeds on bacteria found in the water. When there are low numbers of Cafeteria roenbergensis due to extensive CroV infections, the bacterial populations rise exponentially.[68] The impact of CroV on natural populations of C. roenbergensis remains unknown; however, the virus has been found to be very host specific, and does not infect other closely related organisms.[69] Cafeteria roenbergensis is also infected by a second virus, the Mavirus virophage, which is a satellite virus, meaning it is able to replicate only in the presence of another specific virus, in this case in the presence of CroV.[60] This virus interferes with the replication of CroV, which leads to the survival of C. roenbergensis cells. Mavirus is able to integrate into the genome of cells of C. roenbergensis, and thereby confer immunity to the population.[61]
Role of marine viruses
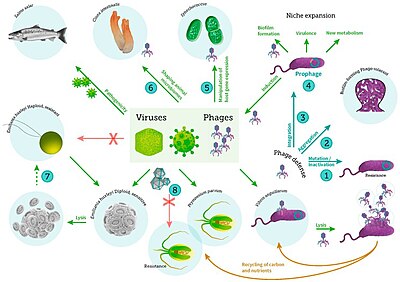
including viral infection of bacteria, phytoplankton and fish[70]

Viruses are the most abundant biological entity in aquatic environments[4] On average there are about ten million of them in a typical teaspoon of seawater.[72] Most of these viruses are bacteriophages infecting heterotrophic bacteria and cyanophages infecting cyanobacteria. They are essential to the regulation of marine ecosystems.[3]
Bacteriophages are harmless to plants and animals, and are essential to the regulation of marine and freshwater ecosystems[73] are important mortality agents of phytoplankton, the base of the foodchain in aquatic environments.[74] They infect and destroy bacteria in aquatic microbial communities, and are one of the most important mechanisms of recycling carbon and nutrient cycling in marine environments. The organic molecules released from the dead bacterial cells stimulate fresh bacterial and algal growth, in a process known as the viral shunt.[75] In particular, lysis of bacteria by viruses has been shown to enhance nitrogen cycling and stimulate phytoplankton growth.[76]
Viral activity also affects the biological pump, the process where carbon is sequestered in the deep ocean.[51] By increasing the amount of respiration in the oceans, viruses are indirectly responsible for reducing the amount of carbon dioxide in the atmosphere by about three gigatonnes of carbon per year.[51]
Microorganisms make up about 70% of the marine biomass.[77] It is estimated viruses kill 20% of this biomass each day and that there are 15 times as many viruses in the oceans as there are bacteria and archaea. Viruses are the main agents responsible for the rapid destruction of harmful algal blooms,[78] which often kill other marine life.[79] The number of viruses in the oceans decreases further offshore and deeper into the water, where there are fewer host organisms.[80]
Viruses are an important natural means of transferring genes between different species, which increases genetic diversity and drives evolution.[8] It is thought viruses played a central role in early evolution, before bacteria, archaea and eukaryotes diversified, at the time of the last universal common ancestor of life on Earth.[81] Viruses are still one of the largest reservoirs of unexplored genetic diversity on Earth.[80]

Viruses are part of the hydrothermal vent microbial community and their influence on the microbial ecology in these ecosystems is a burgeoning field of research.[83] Viruses are the most abundant life in the ocean, harboring the greatest reservoir of genetic diversity.[78] As their infections are often fatal, they constitute a significant source of mortality and thus have widespread influence on biological oceanographic processes, evolution and biogeochemical cycling within the ocean.[80] Evidence has been found however to indicate that viruses found in vent habitats have adopted a more mutualistic than parasitic evolutionary strategy in order to survive the extreme and volatile environment they exist in.[84] Deep-sea hydrothermal vents were found to have high numbers of viruses indicating high viral production.[85] Like in other marine environments, deep-sea hydrothermal viruses affect abundance and diversity of prokaryotes and therefore impact microbial biogeochemical cycling by lysing their hosts to replicate.[86] However, in contrast to their role as a source of mortality and population control, viruses have also been postulated to enhance survival of prokaryotes in extreme environments, acting as reservoirs of genetic information. The interactions of the virosphere with microorganisms under environmental stresses is therefore thought to aide microorganism survival through dispersal of host genes through horizontal gene transfer.[87]
Viral shunt

The viral shunt pathway is a mechanism that prevents (prokaryotic and eukaryotic) marine microbial particulate organic matter (POM) from migrating up trophic levels by recycling them into dissolved organic matter (DOM), which can be readily taken up by microorganisms. Viral shunting helps maintain diversity within the microbial ecosystem by preventing a single species of marine microbe from dominating the micro-environment.[88] The DOM recycled by the viral shunt pathway is comparable to the amount generated by the other main sources of marine DOM.[89]
Viruses can easily infect microorganisms in the microbial loop due to their relative abundance compared to microbes.[90] Prokaryotic and eukaryotic mortality contribute to carbon nutrient recycling through cell lysis. There is evidence as well of nitrogen (specifically ammonium) regeneration. This nutrient recycling helps stimulates microbial growth.[91] As much as 25% of the primary production from phytoplankton in the global oceans may be recycled within the microbial loop through viral shunting.[92]
References
- ^ Bonnain, C., Breitbart, M. and Buck, K.N. (2016) "The ferrojan horse hypothesis: iron-virus interactions in the ocean". Frontiers in marine science, 3: 82. doi:10.3389/fmars.2016.00082.
- ^ These Tiny Organisms Have Some Really Weird Shapes National Geogaphic", 12 November 2016.
- ^ a b Shors T (2008). Understanding Viruses. Jones and Bartlett Publishers. p. 5. ISBN 978-0-7637-2932-5.
- ^ a b c d Koonin EV, Senkevich TG, Dolja VV. The ancient Virus World and evolution of cells. Biology Direct. 2006;1:29. doi:10.1186/1745-6150-1-29. PMID 16984643.
Material was copied from this source, which is available under a Creative Commons Attribution 2.0 International License.
- ^ Mahy WJ & Van Regenmortel MHV (eds). Desk Encyclopedia of General Virology. Oxford: Academic Press; 2009. ISBN 0-12-375146-2. p. 28.
- ^ Iyer LM, Balaji S, Koonin EV, Aravind L. Evolutionary genomics of nucleo-cytoplasmic large DNA viruses. Virus Research. 2006;117(1):156–84. doi:10.1016/j.virusres.2006.01.009. PMID 16494962.
- ^ Sanjuán R, Nebot MR, Chirico N, Mansky LM, Belshaw R. Viral mutation rates. Journal of Virology. 2010;84(19):9733–48. doi:10.1128/JVI.00694-10. PMID 20660197.
- ^ a b Canchaya C, Fournous G, Chibani-Chennoufi S, Dillmann ML, Brüssow H. Phage as agents of lateral gene transfer. Current Opinion in Microbiology. 2003;6(4):417–24. doi:10.1016/S1369-5274(03)00086-9. PMID 12941415.
- ^ Are viruses alive? The replicator paradigm sheds decisive light on an old but misguided question. Studies in History and Philosophy of Biological and Biomedical Sciences. 2016. doi:10.1016/j.shpsc.2016.02.016. PMID 26965225.
- ^ Koonin, E. V.; Starokadomskyy, P. (7 March 2016). "Are viruses alive? The replicator paradigm sheds decisive light on an old but misguided question". Studies in History and Philosophy of Biological and Biomedical Sciences. 59: 125–34. doi:10.1016/j.shpsc.2016.02.016. PMC 5406846. PMID 26965225.
- ^ Rybicki, EP. The classification of organisms at the edge of life, or problems with virus systematics. South African Journal of Science. 1990;86:182–186.
- ^ a b Mann, NH (2005-05-17). "The Third Age of Phage". PLOS Biology. 3 (5): 753–755. doi:10.1371/journal.pbio.0030182. PMC 1110918. PMID 15884981.
{{cite journal}}
: CS1 maint: unflagged free DOI (link) - ^ Wommack KE, Colwell RR (March 2000). "Virioplankton: viruses in aquatic ecosystems". Microbiology and Molecular Biology Reviews. 64 (1): 69–114. doi:10.1128/MMBR.64.1.69-114.2000. PMC 98987. PMID 10704475.
- ^ Bergh O, Børsheim KY, Bratbak G, Heldal M (August 1989). "High abundance of viruses found in aquatic environments". Nature. 340 (6233): 467–68. Bibcode:1989Natur.340..467B. doi:10.1038/340467a0. PMID 2755508.
- ^ Shors pp. 595–97
- ^ Bickle TA, Krüger DH (June 1993). "Biology of DNA restriction". Microbiological Reviews. 57 (2): 434–50. doi:10.1128/MMBR.57.2.434-450.1993. PMC 372918. PMID 8336674.
- ^ Barrangou R, Fremaux C, Deveau H, Richards M, Boyaval P, Moineau S, et al. (March 2007). "CRISPR provides acquired resistance against viruses in prokaryotes". Science. 315 (5819): 1709–12. Bibcode:2007Sci...315.1709B. doi:10.1126/science.1138140. hdl:20.500.11794/38902. PMID 17379808.
- ^ Brouns SJ, Jore MM, Lundgren M, Westra ER, Slijkhuis RJ, Snijders AP, et al. (August 2008). "Small CRISPR RNAs guide antiviral defense in prokaryotes". Science. 321 (5891): 960–64. Bibcode:2008Sci...321..960B. doi:10.1126/science.1159689. PMC 5898235. PMID 18703739.
- ^ Mojica FJ, Rodriguez-Valera F (September 2016). "The discovery of CRISPR in archaea and bacteria". The FEBS Journal. 283 (17): 3162–69. doi:10.1111/febs.13766. hdl:10045/57676. PMID 27234458.
- ^ a b c d Murata, K., Zhang, Q., Galaz-Montoya, J.G., Fu, C., Coleman, M.L., Osburne, M.S., Schmid, M.F., Sullivan, M.B., Chisholm, S.W. and Chiu, W. (2017) "Visualizing adsorption of cyanophage P-SSP7 onto marine Prochlorococcus". Nature: Scientific Reports, 7: 44176. doi:10.1038/srep44176
- ^ Falkowski, P.G., Fenchel, T. and Delong, E.F.(2008) "The microbial engines that drive Earth's biogeochemical cycles". Science, 320(5879): 1034–1039. doi:10.1126/science.1153213
- ^ Brum, J.R. and Sullivan, M.B.(2015) "Rising to the challenge: accelerated pace of discovery transforms marine virology". Nature Reviews Microbiology, 13(3): 147–159. doi:10.1038/nrmicro3404
- ^ Bouman, H.A., Ulloa, O., Scanlan, D.J., Zwirglmaier, K., Li, W.K., Platt, T., Stuart, V., Barlow, R., Leth, O., Clementson, L. and Lutz, V. (2006) "Oceanographic basis of the global surface distribution of Prochlorococcus ecotypes". Science, 312(5775): 918–921. doi:10.1126/science.1122692
- ^ Brum JR, Schenck RO, Sullivan MB (September 2013). "Global morphological analysis of marine viruses shows minimal regional variation and dominance of non-tailed viruses". The ISME Journal. 7 (9): 1738–51. doi:10.1038/ismej.2013.67. PMC 3749506. PMID 23635867.
- ^ Krupovic M, Bamford DH (2007). "Putative prophages related to lytic tailless marine dsDNA phage PM2 are widespread in the genomes of aquatic bacteria". BMC Genomics. 8: 236. doi:10.1186/1471-2164-8-236. PMC 1950889. PMID 17634101.
{{cite journal}}
: CS1 maint: unflagged free DOI (link) - ^ Xue H, Xu Y, Boucher Y, Polz MF (2012). "High Frequency of a Novel Filamentous Phage, VCYϕ, within an Environmental Vibrio cholerae Population". Applied and Environmental Microbiology. 78 (1): 28–33. doi:10.1128/AEM.06297-11. PMC 3255608. PMID 22020507.
- ^ Roux S, Krupovic M, Poulet A, Debroas D, Enault F (2012). "Evolution and diversity of the Microviridae viral family through a collection of 81 new complete genomes assembled from virome reads". PLOS ONE. 7 (7): e40418. Bibcode:2012PLoSO...740418R. doi:10.1371/journal.pone.0040418. PMC 3394797. PMID 22808158.
{{cite journal}}
: CS1 maint: unflagged free DOI (link) - ^ Kauffman, Kathryn M.; Hussain, Fatima A.; Yang, Joy; Arevalo, Philip; Brown, Julia M.; Chang, William K.; Vaninsberghe, David; Elsherbini, Joseph; Sharma, Radhey S.; Cutler, Michael B.; Kelly, Libusha; Polz, Martin F. (2018). "A major lineage of non-tailed dsDNA viruses as unrecognized killers of marine bacteria". Nature. 554 (7690): 118–122. Bibcode:2018Natur.554..118K. doi:10.1038/nature25474. PMID 29364876.
- ^ Scientists Find New Type of Virus in World’s Oceans: Autolykiviridae, on: sci-news, 25 January 2018
- ^ Never-Before-Seen Viruses With Weird DNA Were Just Discovered in The Ocean, on: sciencealert, 25 January 2018
- ^ NCBI: Autolykiviridae (family) – unclassified dsDNA viruses
- ^ Lawrence CM, Menon S, Eilers BJ, et al.. Structural and functional studies of archaeal viruses. Journal of Biological Chemistry. 2009;284(19):12599–603. doi:10.1074/jbc.R800078200. PMID 19158076.
- ^ Prangishvili D, Forterre P, Garrett RA. Viruses of the Archaea: a unifying view. Nature Reviews Microbiology. 2006;4(11):837–48. doi:10.1038/nrmicro1527. PMID 17041631.
- ^ Prangishvili D, Garrett RA. Exceptionally diverse morphotypes and genomes of crenarchaeal hyperthermophilic viruses. Biochemical Society Transactions. 2004;32(Pt 2):204–8. doi:10.1042/BST0320204. PMID 15046572.
- ^ Mojica FJ, Díez-Villaseñor C, García-Martínez J, Soria E (February 2005). "Intervening sequences of regularly spaced prokaryotic repeats derive from foreign genetic elements". Journal of Molecular Evolution. 60 (2): 174–82. Bibcode:2005JMolE..60..174M. doi:10.1007/s00239-004-0046-3. PMID 15791728.
- ^ Makarova KS, Grishin NV, Shabalina SA, Wolf YI, Koonin EV (March 2006). "A putative RNA-interference-based immune system in prokaryotes: computational analysis of the predicted enzymatic machinery, functional analogies with eukaryotic RNAi, and hypothetical mechanisms of action". Biology Direct. 1: 7. doi:10.1186/1745-6150-1-7. PMC 1462988. PMID 16545108.
{{cite journal}}
: CS1 maint: unflagged free DOI (link) - ^ van der Oost J, Westra ER, Jackson RN, Wiedenheft B (July 2014). "Unravelling the structural and mechanistic basis of CRISPR-Cas systems". Nature Reviews. Microbiology. 12 (7): 479–92. doi:10.1038/nrmicro3279. PMC 4225775. PMID 24909109.
- ^ Dimmock NJ, Easton AJ, Leppard K (2007). Introduction to Modern Virology (Sixth ed.). Blackwell Publishing. p. 70. ISBN 978-1-4051-3645-7.
- ^ Tomaru Y, Kimura K and Nagasaki K (2015) "Marine Protist Viruses". In: Ohtsuka S, Suzaki T, Horiguchi T, Suzuki N, Not F (eds) Marine Protists pages 501–517. Springer, Tokyo. doi:10.1007/978-4-431-55130-0_20. ISBN 978-4-431-55130-0.
- ^ Hyman, P. and Abedon, S.T. (2012) "Smaller fleas: viruses of microorganisms". Scientifica, . doi:10.6064/2012/734023.
Material was copied from this source, which is available under a Creative Commons Attribution 3.0 International License.
- ^ Short, S.M. (2012) "The ecology of viruses that infect eukaryotic algae". Environmental microbiology, 14(9): 2253–2271. doi:10.1111/j.1462-2920.2012.02706.x.
- ^ TJohnson, P.T. (1984) "Viral diseases of marine invertebrates". Helgoländer Meeresuntersuchungen, 37(1-4): 65–98. doi:10.1007/BF01989296.
- ^ Renault T (2011) "Viruses infecting marine molluscs" In: Hurst CJ (Ed) Studies in Viral Ecology, Volume 2: Animal Host Systems, John Wiley & Sons. ISBN 9781118024584.
- ^ Arzul, I., Corbeil, S., Morga, B. and Renault, T., 2017. Viruses infecting marine molluscs. Journal of invertebrate pathology, 147, pp.118-135. doi:10.1016/j.jip.2017.01.009.
- ^ Dawsoni, Solaster. "Sea Star Species Affected by Wasting Syndrome." Pacificrockyintertidal.org Seastarwasting.org (n.d.): n. pag. Ecology and Evolutionary Biology. Web.
- ^ "Sea Star Wasting Syndrome | MARINe". eeb.ucsc.edu. Retrieved 2018-06-03.
- ^ a b c Murphy, FA; Gibbs, EPJ; Horzinek, MC; Studdart MJ (1999). Veterinary Virology. Boston: Academic Press. ISBN 978-0-12-511340-3.
- ^ New Brunswick to help Chile beat disease Fish Information and Services
- ^ Fact Sheet - Atlantic Salmon Aquaculture Research Archived December 29, 2010, at the Wayback Machine Fisheries and Oceans Canada. Retrieved 12 May 2009.
- ^ Hall AJ, Jepson PD, Goodman SJ, Harkonen T (2006). "Phocine distemper virus in the North and European Seas – data and models, nature and nurture". Biological Conservation. 131: 221–29. doi:10.1016/j.biocon.2006.04.008.
- ^ a b c Suttle CA (October 2007). "Marine viruses – major players in the global ecosystem". Nature Reviews: Microbiology. 5 (10): 801–12. doi:10.1038/nrmicro1750. PMID 17853907.
- ^ Abrahão, Jônatas; Silva, Lorena; Silva, Ludmila Santos; Khalil, Jacques Yaacoub Bou; Rodrigues, Rodrigo; Arantes, Thalita; Assis, Felipe; Boratto, Paulo; Andrade, Miguel; Kroon, Erna Geessien; Ribeiro, Bergmann; Bergier, Ivan; Seligmann, Herve; Ghigo, Eric; Colson, Philippe; Levasseur, Anthony; Kroemer, Guido; Raoult, Didier; Scola, Bernard La (27 February 2018). "Tailed giant Tupanvirus possesses the most complete translational apparatus of the known virosphere". Nature Communications. 9 (1): 749. Bibcode:2018NatCo...9..749A. doi:10.1038/s41467-018-03168-1. PMC 5829246. PMID 29487281.
- ^ Bichell, Rae Ellen. "In Giant Virus Genes, Hints About Their Mysterious Origin". All Things Considered.
{{cite web}}
: Unknown parameter|name-list-format=
ignored (|name-list-style=
suggested) (help) - ^ Van Etten, James L. (July–August 2011). "Giant Viruses". American Scientist. 99 (4): 304–311. doi:10.1511/2011.91.304.
{{cite journal}}
: Unknown parameter|name-list-format=
ignored (|name-list-style=
suggested) (help) - ^ Legendre M, Arslan D, Abergel C, Claverie JM (January 2012). "Genomics of Megavirus and the elusive fourth domain of Life". Communicative & Integrative Biology. 5 (1): 102–6. doi:10.4161/cib.18624. PMC 3291303. PMID 22482024.
- ^ Schulz F, Yutin N, Ivanova NN, Ortega DR, Lee TK, Vierheilig J, Daims H, Horn M, Wagner M, Jensen GJ, Kyrpides NC, Koonin EV, Woyke T (April 2017). "Giant viruses with an expanded complement of translation system components" (PDF). Science. 356 (6333): 82–85. Bibcode:2017Sci...356...82S. doi:10.1126/science.aal4657. PMID 28386012.
- ^ Bäckström D, Yutin N, Jørgensen SL, Dharamshi J, Homa F, Zaremba-Niedwiedzka K, Spang A, Wolf YI, Koonin EV, Ettema TJ (March 2019). "Virus Genomes from Deep Sea Sediments Expand the Ocean Megavirome and Support Independent Origins of Viral Gigantism". mBio. 10 (2): e02497-02418. doi:10.1128/mBio.02497-18. PMC 6401483. PMID 30837339.
- ^ Xiao, C., Fischer, M.G., Bolotaulo, D.M., Ulloa-Rondeau, N., Avila, G.A., and Suttle, C.A. (2017) "Cryo-EM reconstruction of the Cafeteria roenbergensis virus capsid suggests novel assembly pathway for giant viruses". Scientific Reports, 7: 5484. doi:10.1038/s41598-017-05824-w.
- ^ Duponchel, S; Fischer, MG (March 2019). "Viva lavidaviruses! Five features of virophages that parasitize giant DNA viruses". PLOS Pathogens. 15 (3): e1007592. doi:10.1371/journal.ppat.1007592. PMC 6428243. PMID 30897185.
{{cite journal}}
: CS1 maint: unflagged free DOI (link) - ^ a b Fischer MG, Suttle CA (April 2011). "A virophage at the origin of large DNA transposons". Science. 332 (6026): 231–4. Bibcode:2011Sci...332..231F. doi:10.1126/science.1199412. PMID 21385722.
- ^ a b Fischer MG, Hackl (December 2016). "Host genome integration and giant virus-induced reactivation of the virophage mavirus". Nature. 540 (7632): 288–91. Bibcode:2016Natur.540..288F. doi:10.1038/nature20593. PMID 27929021.
- ^ Katzourakis, Aris; Aswad, Amr (2014). "The origins of giant viruses, virophages and their relatives in host genomes". BMC Biology. 12: 2–3. doi:10.1186/s12915-014-0051-y. PMC 4096385. PMID 25184667.
{{cite journal}}
: CS1 maint: unflagged free DOI (link) - ^ Krupovic, Mart; Kuhn, Jens; Fischer, Metthias (Fall 2015). "A classification system for virophages and satellite viruses" (PDF). Archives of Virology. 161 (1): 233–247. doi:10.1007/s00705-015-2622-9. PMID 26446887 – via Springer.
- ^ Roux, Simon; Chan, Leong-Keat; Egan, Rob; Malmstrom, Rex R.; McMahon, Katherine D.; Sullivan, Matthew B. (2017). "Ecogenomics of virophages and their giant virus hosts assessed through time series metagenomics". Nature Communications. 8 (1): 858. Bibcode:2017NatCo...8..858R. doi:10.1038/s41467-017-01086-2. ISSN 2041-1723. PMC 5636890. PMID 29021524.
- ^ Paez-Espino, David; Zhou, Jinglie; Roux, Simon; Nayfach, Stephen; Pavlopoulos, Georgios A.; Schulz, Frederik; McMahon, Katherine D.; Walsh, David; Woyke, Tanja; Ivanova, Natalia N.; Eloe-Fadrosh, Emiley A.; Tringe, Susannah G.; Kyrpides, Nikos C. (2019-12-10). "Diversity, evolution, and classification of virophages uncovered through global metagenomics". Microbiome. 7 (1): 157. doi:10.1186/s40168-019-0768-5. PMC 6905037. PMID 31823797.
{{cite journal}}
: CS1 maint: unflagged free DOI (link) - ^ Duponchel, S. and Fischer, M.G. (2019) "Viva lavidaviruses! Five features of virophages that parasitize giant DNA viruses". PLoS pathogens, 15(3). doi:10.1371/journal.ppat.1007592.
Material was copied from this source, which is available under a Creative Commons Attribution 4.0 International License.
- ^ Fischer, M. G.; Allen, M. J.; Wilson, W. H.; Suttle, C. A. (2010). "Giant virus with a remarkable complement of genes infects marine zooplankton" (PDF). Proceedings of the National Academy of Sciences. 107 (45): 19508–19513. Bibcode:2010PNAS..10719508F. doi:10.1073/pnas.1007615107. PMC 2984142. PMID 20974979.
- ^ Matthias G. Fischer; Michael J. Allen; William H. Wilson; Curtis A. Suttle (2010). "Giant virus with a remarkable complement of genes infects marine zooplankton" (PDF). Proceedings of the National Academy of Sciences. 107 (45): 19508–19513. Bibcode:2010PNAS..10719508F. doi:10.1073/pnas.1007615107. PMC 2984142. PMID 20974979.
- ^ Massana, Ramon; Javier Del Campo; Christian Dinter; Ruben Sommaruga (2007). "Crash of a population of the marine heterotrophic flagellate Cafeteria roenbergensis by viral infection". Environmental Microbiology. 9 (11): 2660–2669. doi:10.1111/j.1462-2920.2007.01378.x. PMID 17922751.
- ^ Middelboe, M.; Brussaard, C. (2017). "Marine viruses: key players in marine ecosystems". Viruses. 9 (10): 302. doi:10.3390/v9100302. PMC 5691653. PMID 29057790.
- ^ Käse L and Geuer JK (2018) "Phytoplankton responses to marine climate change–an introduction". In Jungblut S., Liebich V., Bode M. (Eds) YOUMARES 8–Oceans Across Boundaries: Learning from each other, pages 55–72, Springer. doi:10.1007/978-3-319-93284-2_5.
- ^ Dávila-Ramos S, Castelán-Sánchez HG, Martínez-Ávila L, Sánchez-Carbente MD, Peralta R, Hernández-Mendoza A, et al. (2019). "A Review on Viral Metagenomics in Extreme Environments". Frontiers in Microbiology. 10: 2403. doi:10.3389/fmicb.2019.02403. PMC 6842933. PMID 31749771.
{{cite journal}}
: CS1 maint: unflagged free DOI (link) - ^ Weitz JS, Wilhelm SW (2013). "An ocean of viruses". The Scientist. 27 (7): 35–39.
- ^ Suttle CA (September 2005). "Viruses in the sea". Nature. 437 (7057): 356–61. Bibcode:2005Natur.437..356S. doi:10.1038/nature04160. PMID 16163346.
- ^ Wilhelm SW, Suttle CA (1999). "Viruses and nutrient cycles in the sea: viruses play critical roles in the structure and function of aquatic food webs". BioScience. 49 (10): 781–88. doi:10.2307/1313569. JSTOR 1313569.
- ^ Shelford EJ, Suttle CA (2018). "Virus-mediated transfer of nitrogen from heterotrophic bacteria to phytoplankton". Biogeosciences. 15 (3): 809–15. Bibcode:2018BGeo...15..809S. doi:10.5194/bg-15-809-2018.
{{cite journal}}
: CS1 maint: unflagged free DOI (link) - ^ Bar-On, YM; Phillips, R; Milo, R (2018). "The biomass distribution on Earth". PNAS. 115 (25): 6506–6511. doi:10.1073/pnas.1711842115. PMC 6016768. PMID 29784790.
- ^ a b Suttle, Curtis A. (2005). "Viruses in the sea". Nature. 437 (7057): 356–361. Bibcode:2005Natur.437..356S. doi:10.1038/nature04160. ISSN 0028-0836. PMID 16163346.
- ^ www.cdc.gov. Harmful Algal Blooms: Red Tide: Home [Retrieved 2014-12-19].
- ^ a b c Suttle, Curtis A. (October 2007). "Marine viruses — major players in the global ecosystem". Nature Reviews Microbiology. 5 (10): 801–812. doi:10.1038/nrmicro1750. ISSN 1740-1526. PMID 17853907.
- ^ Forterre P, Philippe H. The last universal common ancestor (LUCA), simple or complex?. The Biological Bulletin. 1999;196(3):373–5; discussion 375–7. doi:10.2307/1542973. PMID 11536914.
- ^ Heinrichs, M.E., Mori, C. and Dlugosch, L. (2020) "Complex Interactions Between Aquatic Organisms and Their Chemical Environment Elucidated from Different Perspectives". In: YOUMARES 9-The Oceans: Our Research, Our Future , pages 279–297. Springer. doi:10.1007/978-3-030-20389-4_15.
- ^ Anderson, Rika E.; Brazelton, William J.; Baross, John A. (2011). "Is the genetic landscape of the deep subsurface biosphere affected by viruses?". Frontiers in Microbiology. 2: 219. doi:10.3389/fmicb.2011.00219. ISSN 1664-302X. PMC 3211056. PMID 22084639.
{{cite journal}}
: CS1 maint: unflagged free DOI (link) - ^ Anderson, Rika E.; Sogin, Mitchell L.; Baross, John A. (2014-10-03). "Evolutionary Strategies of Viruses, Bacteria and Archaea in Hydrothermal Vent Ecosystems Revealed through Metagenomics". PLOS ONE. 9 (10): e109696. Bibcode:2014PLoSO...9j9696A. doi:10.1371/journal.pone.0109696. ISSN 1932-6203. PMC 4184897. PMID 25279954.
{{cite journal}}
: CS1 maint: unflagged free DOI (link) - ^ Ortmann, Alice C.; Suttle, Curtis A. (August 2005). "High abundances of viruses in a deep-sea hydrothermal vent system indicates viral mediated microbial mortality". Deep Sea Research Part I: Oceanographic Research Papers. 52 (8): 1515–1527. Bibcode:2005DSRI...52.1515O. doi:10.1016/j.dsr.2005.04.002. ISSN 0967-0637.
- ^ Breitbart, Mya (2012-01-15). "Marine Viruses: Truth or Dare". Annual Review of Marine Science. 4 (1): 425–448. Bibcode:2012ARMS....4..425B. doi:10.1146/annurev-marine-120709-142805. ISSN 1941-1405. PMID 22457982.
- ^ Goldenfeld, Nigel; Woese, Carl (January 2007). "Biology's next revolution". Nature. 445 (7126): 369. arXiv:q-bio/0702015. Bibcode:2007Natur.445..369G. doi:10.1038/445369a. ISSN 0028-0836. PMID 17251963.
- ^ Weinbauer, Markus G.; et al. (2007). "Synergistic and antagonistic effects of viral lysis and protistan grazing on bacterial biomass, production and diversity". Environmental Microbiology. 9 (3): 777–788. doi:10.1111/j.1462-2920.2006.01200.x. PMID 17298376.
- ^ Robinson, Carol, and Nagappa Ramaiah. "Microbial heterotrophic metabolic rates constrain the microbial carbon pump." The American Association for the Advancement of Science, 2011.
- ^ Fuhrman, Jed A. (1999). "Marine viruses and their biogeochemical and ecological effects". Nature. 399 (6736): 541–548. Bibcode:1999Natur.399..541F. doi:10.1038/21119. ISSN 0028-0836. PMID 10376593.
- ^ Tsai, An-Yi, Gwo-Ching Gong, and Yu-Wen Huang. "Importance of the Viral Shunt in Nitrogen Cycling in Synechococcus Spp. Growth in Subtropical Western Pacific Coastal Waters." Terrestrial, Atmospheric & Oceanic Sciences25.6 (2014).
- ^ Wilhelm, Steven W.; Suttle, Curtis A. (1999). "Viruses and nutrient cycles in the sea: viruses play critical roles in the structure and function of aquatic food webs". BioScience. 49 (10): 781–788. doi:10.2307/1313569. JSTOR 1313569.