Betelgeuse
Observation data Epoch J2000.0 Equinox J2000.0 | |
---|---|
Constellation | Orion |
Pronunciation | /ˈbiːtəldʒuːz/ or /ˈbɛtəldʒuːz/[1] |
Right ascension | 05h 55m 10.3053s[2] |
Declination | +07° 24′ 25.426″[2] |
Apparent magnitude (V) | 0.42[2] (0.3 to 1.2) |
Characteristics | |
Spectral type | M2Iab[2] |
Apparent magnitude (J) | -2.99 ± 0.10[2] |
U−B color index | 2.06[3] |
B−V color index | 1.85[3] |
Variable type | SR c (Semi-Regular)[2] |
Astrometry | |
Radial velocity (Rv) | +21.91[2] km/s |
Proper motion (μ) | RA: 24.95 ± 0.08[4] mas/yr Dec.: 9.56 ± 0.15[4] mas/yr |
Parallax (π) | 5.07 ± 1.10 mas[4] |
Distance | 643 ± 146 [4] ly (197 ± 45 [4] pc) |
Absolute magnitude (MV) | −6.05[5] |
Details | |
Mass | ~18–19[6] M☉ |
Radius | ~1180[7] R☉ |
Luminosity | ~140,000[8] L☉ |
Surface gravity (log g) | -0.5[9] cgs |
Temperature | 3,500[9][10] K |
Metallicity | 0.05 Fe/H[11] |
Rotation | 5 km/s[10] |
Age | ~1.0×107 [6] years |
Other designations | |
Database references | |
SIMBAD | data |
Betelgeuse (/ˈbiːtəldʒuːz/ or /ˈbɛtəldʒuːz/),[1] also known by its Bayer designation Alpha Orionis (α Orionis, α Ori), is the eighth brightest star in the night sky and second brightest star in the constellation of Orion, outshining Rigel (Beta Orionis) only rarely. Distinctly reddish-tinted, it is a semiregular variable star whose apparent magnitude varies between 0.2 and 1.2, the widest range of any first magnitude star. The star marks the upper right vertex of the Winter Triangle asterism and the center of the Winter Hexagon.
Classified as a red supergiant, Betelgeuse is one of the largest and most luminous stars known. If it were at the center of the Solar System, its surface would extend past the asteroid belt possibly to the orbit of Jupiter and beyond, wholly engulfing Mercury, Venus, Earth and Mars. Betelgeuse is currently thought to lie around 640 light-years away, yielding a mean absolute magnitude of about −6.05.
In 1920, Betelgeuse was the first star (after the Sun) to have its angular diameter measured. Since then, researchers have used a number of telescopes to measure this stellar giant, each with different technical parameters, often yielding conflicting results. Current estimates of the star's apparent diameter range from about 0.043 to 0.056 arcseconds. This is a moving target at best, as Betelgeuse appears to change shape periodically. Because of limb darkening, variability, and angular diameters that vary with wavelength, the star remains a perplexing mystery. To complicate matters further, Betelgeuse has a complex, asymmetric envelope caused by colossal mass loss involving huge plumes of gas being expelled from its surface. There is even evidence of stellar companions orbiting within this gaseous envelope, possibly contributing to the star's eccentric behavior.
Only 10 million years old, Betelgeuse has evolved rapidly because of its high mass. It is thought to be a runaway star from the Orion OB1 Association, which also includes the late type O and B stars in Orion's belt—Alnitak, Alnilam and Mintaka. Currently in a late stage of stellar evolution, Betelgeuse is expected to proceed through its expected life cycle before exploding as a type II supernova within the next million years.
Observational history
Betelgeuse and its red coloration have been noted since antiquity; the classical astronomer Ptolemy described its color as ὑπόκιρρος (hypókirros), a term which was later described by a translator of Ulugh Beg's Zij-i Sultani as rubedo, Latin for "ruddiness".[12][13] In contrast, the historical record of Chinese astronomers during the first century BC mention Betelgeuse as having a yellow color.[14] Prior to the modern systems of stellar classification, Angelo Secchi had created his own system of spectral analysis with Antares and Betelgeuse as the prototypes for his Class III (orange to red) stars.[15]
With the history of astronomy intimately associated with mythology and astrology prior to the scientific revolution, the red star, like the planet Mars that derives its name from a Roman war god, has been closely associated with the martial archetype of conquest for millennia, and by extension, with the motif of death and rebirth.[12] In South African mythology, Betelgeuse was a lion watching in a predatory manner the three zebras represented by Orion's belt.[16]
Discovery of variability

The variation in Betelgeuse's brightness was first described in 1836 by Sir John Herschel, when he published his observations in Outlines of Astronomy; he noted an increase in activity from 1836–1840, followed by a subsequent reduction. In 1849, he noted a shorter cycle of variability which peaked in 1852. Later observers recorded unusually high maxima with an interval of several years, but only small variations from 1957 to 1967. The records of the American Association of Variable Star Observers (AAVSO) show a maximum apparent magnitude (brightness) of 0.2 in the years 1933 and 1942, with a minimum fainter than magnitude 1.2 in both 1927 and 1941.[17][18] This variability in brightness may explain why Johann Bayer, with the publication of his Uranometria in 1603, designated the star alpha as it may have rivalled the usually brighter Rigel (beta).[19]
In 1920, Albert Michelson and Francis Pease mounted a 6 metre (20 ft) interferometer on the front of the 2.5 metre (100 inch) telescope at Mount Wilson Observatory. Helped by John Anderson, the trio measured the angular diameter of Betelgeuse at 0.047", a figure which resulted in a diameter of 3.84 × 108 km (240 million miles or 2.58 AU) based on the then-current parallax value of 0.018".[20] However there was known uncertainty owing to limb darkening and measurement errors—a central theme which would be the focus of scientific inquiry for almost a century. Beginning with this first angular measurement at visible wavelengths, researchers have since tried the measurement at other wavelengths with varying results.
The 1950s and '60s saw important scientific developments, the two Stratoscope projects and the 1958 publication of Structure and Evolution of the Stars, principally the work of Martin Schwarzschild and his close colleague at Princeton University, Richard Härm.[21][22] This book taught a generation of astrophysicists how to use nascent computer technology to create stellar models while the Stratoscope projects, by taking instrumented balloons above the Earth's turbulence, produced some of the finest images of solar granules and sunspots ever seen, thus confirming the existence of convection in the solar atmosphere.[21] Both developments would prove to have a significant impact on understanding of the structure of red supergiants like Betelgeuse.
Aperture masking
The 1970s saw several notable advances in interferometry from the Berkeley Space Sciences Laboratory working in the infrared and Antoine Labeyrie in the visible, when researchers began to combine images from multiple telescopes and later invented "fringe-tracking" technology. But it was not until the late 1980s and early 1990s, when Betelgeuse became a regular target for aperture masking interferometry, that significant breakthroughs occurred in visible-light and infrared imaging. Pioneered by John E. Baldwin and other colleagues of the Cavendish Astrophysics Group, the new technique contributed some of the most accurate measurements of Betelgeuse to date while revealing a number of bright spots on the star's photosphere.[24][25][26] These were the first optical and infrared images of a stellar disk other than the Sun, first from ground-based interferometers and later from higher-resolution observations of the COAST telescope, with the "bright patches" or "hotspots" potentially corroborating a theory put forth by Schwarzschild decades earlier of massive convection cells dominating the stellar surface.[27][28]
In 1995, the Hubble Space Telescope's Faint Object Camera captured an ultraviolet image of comparable resolution—the first conventional-telescope image (or "direct-image" in NASA terminology) of the disk of another star. The image was taken at ultraviolet wavelengths since ground-based instruments cannot produce images in the ultraviolet with the same precision as Hubble. Like earlier images, this ultraviolet image also contained a bright patch, indicating a hotter region of about 2,000K, in this case on the southwestern portion of the star's surface.[29] Subsequent ultraviolet spectra taken with the Goddard High Resolution Spectrograph suggested that the hot spot was one of Betelgeuse's poles of rotation. This would give the rotational axis an inclination of about 20° to the direction of Earth, and a position angle from celestial North of about 55°.[30]
Recent studies

The first decade of the 21st century has witnessed major advances on multiple fronts, the most central of which have been the imaging of the star's photosphere at different wavelengths and the study of Betelgeuse's complex circumstellar shells. At the dawn of the millennium, Betelgeuse was measured in the mid-infrared using the Infrared Spatial Interferometer (ISI) producing a limb darkened estimate of 55.2 ± 0.5 milliarcseconds (mas)—a figure entirely consistent with Michelson's findings eighty years earlier.[20][31] At the time of its publication, the estimated parallax from the Hipparcos mission was 7.63 ± 1.64 mas, yielding an estimated radius for Betelgeuse of 3.6 AU. However, numerous interferometric studies in the near-infrared have appeared since from the Paranal Observatory in Chile arguing for much tighter diameters. Nevertheless, on June 9, 2009, Nobel Laureate Charles Townes announced that the star had shrunk 15% since 1993 at an increasing rate. He presented evidence that UC Berkeley's ISI atop Mt. Wilson Observatory had observed 15 consecutive years of stellar contraction. Despite the apparent diminution of Betelgeuse's size, Townes and his colleague, Edward Wishnow, pointed out that the star's visible brightness, or magnitude, which is monitored regularly by members of the American Association of Variable Star Observers (AAVSO), had shown no significant dimming over that same time frame.[32][33] This finding of a diminishing radius coupled with a relatively constant flux puts into question some of the fundamental theories of stellar structure.

Enveloping this whole discussion have been numerous inquiries into the abstruse dynamics of Betelgeuse's extended atmosphere. For decades astronomers have understood that red giants dominate mass return to the Milky Way creating opaque outer shells, yet the actual mechanics of such stellar mass loss have remained a mystery.[35] With recent advances in interferometric methodologies, astronomers may be close to resolving this conundrum. In July 2009, images released by the European Southern Observatory, taken by the ground-based Very Large Telescope Interferometer (VLTI), showed vast plumes of gas being ejected into the surrounding atmosphere with distances approximating 30 AU.[10][36] Comparable to the distance between the Sun and Neptune, this mass ejection is but one of multiple dynamics occurring in the surrounding atmosphere. Astronomers have identified at least 6 different shells surrounding Betelgeuse. Solving the mystery of mass loss in the late stages of a star's evolution may reveal those factors which precipitate the explosive deaths of these stellar giants.[32]
Visibility

Betelgeuse is easy to spot in the night sky, as it appears in proximity to the famous belt of Orion and has a distinctive orange-red color to the naked eye. In the Northern Hemisphere, beginning in January of each year, it can be seen rising in the east just after sunset. By mid-March, it is visible to virtually every inhabited region of the globe, with only a few obscure research stations in Antarctica at latitudes south of 82° unable to see it. Once May arrives, the red giant can be glimpsed but briefly on the western horizon just after the Sun sets.
The apparent magnitude of Betelgeuse is listed in SIMBAD at 0.42, making it on average the ninth brightest star in the celestial sphere—just ahead of Achernar. Because Betelgeuse is a variable star whose brightness ranges between 0.2 and 1.2, there are periods when it will surpass Procyon to become the eighth brightest star. Occasionally it can even outshine Rigel and become the seventh brightest star, as the latter star, with a nominal apparent magnitude of 0.12, has been reported to fluctuate slightly in brightness, by 0.03 to 0.3 magnitudes,[37] At its faintest, Betelgeuse will fall behind Deneb as the 19th brightest star and compete with Mimosa for the 20th position.

Betelgeuse has a color index (B–V) of 1.85—a figure which points to the advanced "redness" of this celestial object. The photosphere has an extended atmosphere which displays strong lines of emission rather than absorption, a phenomenon which occurs when a star is surrounded by a thick gaseous envelope. This extended gaseous atmosphere has been observed moving both away from and towards Betelgeuse, depending apparently on radial velocity fluctuations in the photosphere. Only about 13% of the star's radiant energy is emitted in the form of visible light, with most of its radiation occurring in the infrared. If human eyes were sensitive to radiation at all wavelengths, Betelgeuse would appear as the brightest star in the sky.[18] Betelgeuse is the brightest near-IR source in the sky with a J band magnitude of -2.99.[39]
Parallax
Since the first successful parallax measurement was conducted in 1838 by Friedrich Bessel, astronomers have been puzzled by Betelgeuse's distance, which holds the key to understanding other stellar parameters, as an accurate "distance leads to the luminosity and when combined with an angular diameter gives the physical radius and effective temperature; luminosity and isotopic abundances provide estimates of the stellar age and mass".[4] In 1920, when the first interferometric studies were performed on the star's diameter, the assumed parallax was 0.0180 arcseconds. That equated to a distance of 56 parsecs (pc) or roughly 180 light years (ly) and produced not only an inaccurate radius for the star, but every other stellar characteristic. Since then, there has been ongoing work to measure the actual distance of Betelguese, with proposed distances as high as 400 parsecs or about 1,300 light years.[4]
Before the publication of the Hipparcos Catalogue (1997), there were two conflicting parallax measurements for Betelgeuse. The first was the Yale University Observatory (1991) with a published parallax of π = 9.8 ± 4.7 mas, yielding a distance of roughly 102 pc or 330 ly.[40] The second was the Hipparcos Input Catalogue (1993) with a trigonometric parallax of π = 5 ± 4 mas, a distance of 200 pc or 650 ly—almost twice the Yale estimate.[41] This uncertainty impeded more detailed understanding of Betelguese's properties.[4]
The results from the Hipparcos mission were released in 1997. The measured parallax of Betelguese was π = 7.63 ± 1.64 mas, which equated to a distance of 131 pc or roughly 430 ly, and had smaller reported error than previous measurements.[42] However, later evaluation of the Hipparcos parallax measurements for variable stars like Betelgeuse found that the uncertainty of these measurements were underestimated.[43] As a result, "the significance of the Hipparcos parallax [was] not sufficient to derive the distance or stellar peculiar velocity with confidence".[4]
The Hipparcos result was improved upon with observations using the Very Large Array (VLA), which, along with the Hipparcos data, produced a more precise astrometric solution: π = 5.07 ± 1.10 mas, a tighter error factor yielding a distance of 197 ± 45 pc or 643 ± 146 ly.[4]
The next breakthrough will likely come from the European Space Agency's upcoming Gaia mission when it undertakes a detailed analysis of physical properties for each star observed, revealing luminosity, temperature, gravity and composition. Gaia will achieve this by repeatedly measuring the positions of all objects down to magnitude 20, and those brighter than magnitude 15, to an accuracy of 24 microarcseconds—akin to measuring the diameter of a human hair from 1000 km away. On-board detection equipment will ensure that variable stars like Betelgeuse will all be detected to this faint limit, thus addressing most of the limitations of the earlier Hipparcos mission.[44]
Variability

As a pulsating variable star with sub-classification "SRC", researchers have offered different hypotheses to explain Betelgeuse's volatile choreography—a phenomenon which causes an absolute magnitude oscillation from −5.27 and −6.27.[45] Our current understanding of stellar structure suggests that the outer layers of this supergiant gradually expand and contract, causing the surface area (photosphere) to alternately increase and decrease, and the temperature to rise and fall—thus eliciting the measured cadence in the star's brightness between its dimmest magnitude of 1.2, seen as early as 1927, and its brightest of 0.2, seen in 1933 and 1942. A red supergiant like Betelgeuse will pulsate this way because its stellar atmosphere is inherently unstable. As the star contracts, it absorbs more and more of the energy that passes through it, causing the atmosphere to heat up and expand. Conversely, as the star expands, its atmosphere becomes less dense allowing the energy to escape and the atmosphere to cool, thus initiating a new contraction phase.[17] Calculating the star's pulsations and modeling its periodicity have been difficult, as it appears there are several cycles interlaced. As discussed in papers by Stebbins and Sanford in the 1930s, there are short-term variations of around 150 to 300 days that modulate a regular cyclic variation with a period of roughly 5.7 years.[46][47]

1. Core
2. Radiative zone
3. Convective zone
4. Photosphere
5. Chromosphere
6. Corona
7. Sunspot
8. Granules
9. Prominence
In fact, the supergiant consistently displays irregular photometric, polarimetric and spectroscopic variations, which points to complex activity on the star's surface and in its extended atmosphere.[24] In marked contrast to most giant stars that are typically long period variables with reasonably regular periods, red giants are generally semiregular or irregular with pulsating characteristics. In a landmark paper published in 1975, Martin Schwarzschild attributed these brightness fluctuations to the changing granulation pattern formed by a few giant convection cells covering the surface of these stars.[28][48] For the Sun, these convection cells, otherwise known as solar granules, represent the foremost mode of heat transfer—hence those convective elements which dominate the brightness variations in the solar photosphere.[28] The typical diameter for a solar granule is about 2,000 km (yielding a surface area roughly the size of India), with an average depth of 700 km. With a surface of roughly 6 trillion km2, there are about 2 million of such granules lying on the Sun's photosphere, which because of their number produce a relatively constant flux. Beneath these granules, it is conjectured that there are 5 to 10 thousand supergranules, the average diameter of which is 30,000 km with a depth of about 10,000 km.[49] By contrast, Schwarzschild argues that stars like Betelgeuse may have only a dozen monster granules with diameters of 180 million km or more dominating the surface of the star with depths of about 60 million km, which, because of the very low temperatures and extremely low density found in red giant envelopes, result in convective inefficiency. Consequently, if only a third of these convective cells are visible to us at any one time, the time variations in their observable light may well be reflected in the brightness variations of the integrated light of the star.[28]
Schwarzschild's hypothesis of gigantic convection cells dominating the surface of red giants and supergiants seems to have stuck with the astronomical community. When the Hubble Space Telescope captured its first direct image of Betelgeuse in 1995 revealing a mysterious hot spot, astronomers attributed it to convection.[50] Two years later, astronomers observed intricate asymmetries in the brightness distribution of the star revealing at least three bright spots, the magnitude of which was "consistent with convective surface hotspots".[25] Then in 2000, another team of astronomers led by Alex Lobel of the Harvard–Smithsonian Center for Astrophysics (CfA) noted that Betelgeuse exhibits raging storms of hot and cold gas in its turbulent atmosphere. The team surmised that huge areas of the star's photosphere vigorously bulge out in different directions at times, ejecting long plumes of warm gas into the cold dust envelope. Another explanation that was also given was the occurrence of shock waves caused by warm gas traversing cooler regions of the star.[47][51] The team investigated the atmosphere of Betelgeuse over a period of five years between 1998 and 2003 with the Space Telescope Imaging Spectrograph aboard Hubble. They found that the bubbling action of the chromosphere tosses gas out one side of the star, while it falls inward at the other side, similar to the slow-motion churning of a lava lamp.
Angular size
A third challenge that has confronted astronomers has been measuring the star's angular diameter. On December 13, 1920, Betelgeuse became the first star outside the Solar System to ever have its diameter measured.[20] Although interferometry was still in its infancy, the experiment proved a success and Betelgeuse was found to have a uniform disk of 0.047 arcseconds. The astronomers' insights on limb darkening were noteworthy; in addition to a measurement error of 10%, the team concluded that the stellar disk was likely 17% larger due to the diminishing intensity of light around the edges—hence an angular diameter of about 0.055".[20][33] Since then, there have been other studies conducted, which have produced angles that range from 0.042 to 0.069 arcseconds.[31][52][53] Combining that data with historical distance estimates of 180 to 815 ly yields a projected diameter of the stellar disk of anywhere from 2.4 to 17.8 AU, hence a radius of 1.2 to 8.9 AU respectively.[note 1] Using the Solar System as a yardstick, the orbit of Mars is about 1.5 AU, Ceres in the asteroid belt 2.7 AU, Jupiter 5.5 AU—consequently a photosphere which, depending on Betelgeuse's actual distance from Earth, could well extend beyond the Jovian orbit but not quite as far as Saturn at 9.5 AU.

The precise diameter has been hard to define for several reasons:
- The rhythmic expansion and contraction of the photosphere, as theory suggests, means the diameter is never constant;
- There is no definable "edge" to the star as limb darkening causes the optical emissions to vary in color and decrease the farther one extends out from the center;
- Betelgeuse is surrounded by a circumstellar envelope composed of matter being ejected from the star—matter which both absorbs and emits light—making it difficult to define the edge of the photosphere;[32]
- Measurements can be taken at varying wavelengths within the electromagnetic spectrum, with each wavelength revealing something different. Studies have shown that angular diameters are considerably larger at visible wavelengths, decrease to a minimum in the near-infrared, only to increase again in the mid-infrared.[54][55] The difference in reported diameters can be as much as 30–35%, yet because each wavelength measures something different, comparing one finding with another is problematic;[32]
- Atmospheric twinkling limits the resolution obtainable from ground-based telescopes since turbulence degrades angular resolution.[24]
To overcome these challenges, researchers have employed various solutions. The concept of astronomical interferometry was first conceived by Hippolyte Fizeau in 1868.[56] He proposed the observation of stars through two apertures to obtain interferences that would furnish information on the star's spatial intensity distribution. Since then, the science of interferometry has evolved considerably where multiple-aperture interferometers are now used consisting of numerous images superimposed on each other. These "speckled" images are then synthesized using Fourier analysis—a method used for a wide array of astronomical objects including the study of binary stars, quasars, asteroids and galactic nuclei.[57] The emergence of adaptive optics since 1990 has revolutionized high angular resolution astronomy,[58] while space observatories like Hipparcos, Hubble and Spitzer have produced other significant breakthroughs.[23][59] Recently another instrument, the Astronomical Multi-BEam Recombiner (AMBER), is providing new insights. As part of the VLTI, AMBER is capable of combining the beams of three telescopes simultaneously, allowing researchers to achieve milliarcsecond spatial resolution. Also by combining three baselines instead of two, which is customary with conventional interferometry, AMBER enables astronomers to compute the closure phase—an important element in astronomical imaging.[60][61]
The current debate revolves around which wavelength—the visible, near-infrared (NIR) or mid-infrared (MIR)—produces the most accurate angular measurement.[note 1] The most widely adopted solution is the one performed with the ISI in the mid-infrared by astronomers from the Space Sciences Laboratory at U.C. Berkeley. In the epoch year 2000, the group, under the leadership of John Weiner, showed Betelgeuse with a uniform disk of 54.7 ± 0.3 mas, ignoring any possible contribution from hotspots, which are less noticeable in the mid-infrared.[31] They also included a theoretical allowance for limb darkening yielding a diameter of 55.2 ± 0.5 mas—a figure which equates to a radius of roughly 5.5 AU (1,180 R☉), assuming a distance of 197.0 ± 45 pc.[note 2] Nevertheless, given the angular error factor of ± 0.5 mas combined with a parallax error of ± 45 pc found in Harper's numbers, the photosphere's radius could actually be as small as 4.2 AU or as large as 6.9 AU.[62]
Across the Atlantic, another team of astronomers led by Guy Perrin of the Observatoire de Paris produced a document in 2004 arguing that the near-infrared figure of 43.33 ± 0.04 mas was a more accurate photospheric measurement.[54] "A consistent scenario to explain the observations of this star from the visible to the mid-infrared can be set-up", Perrin reports. "The star is seen through a thick, warm extended atmosphere that scatters light at short wavelengths thus slightly increasing its diameter. The scatter becomes negligible above 1.3 μm. The upper atmosphere being almost transparent in K and L—the diameter is minimum at these wavelengths where the classical photosphere can be directly seen. In the mid-infrared, the thermal emission of the warm atmosphere increases the apparent diameter of the star." The argument has yet to receive widespread support among astronomers.[32]
More recent studies done in the near-infrared with the IOTA and VLTI have brought strong support to Perrin's analysis yielding diameters that range from 42.57 to 44.28 mas with minimal error factors less than 0.04 mas.[63][64] Central to this discussion, however, is a second paper published by the Berkeley team in 2009, this time led by Charles Townes, reporting that the radius of Betelgeuse had actually shrunk from 1993 to 2009 by 15%, with the 2008 angular measurement equal to 47.0 mas, not too far from Perrin's estimate.[33][65] Unlike most papers heretofore published, this study encompassed a 15-year horizon at one specific wavelength. Earlier studies have typically lasted one to two years by comparison and have explored multiple wavelengths, often yielding vastly different results. The diminution in angular separation equates to a range of values between 56.0 ± 0.1 mas seen in 1993 to 47.0 ± 0.1 mas seen in 2008—a contraction of almost 0.9 AU in 15 years or roughly 1,000 km per hour.[note 3] What is not fully known is whether this observation is evidence of a rhythmic expansion and contraction of the star as astronomers have theorized, and if so, what the periodic cycle might be, although Townes suggests that if a cycle does exist, it is probably a few decades long.[33] Other possible explanations are photospheric protrusions due to convection or a star that is not spherical but rather asymmetric causing but the appearance of expansion and contraction as the star rotates on its axis.[66] Consequently, whether the 1993 figure of 56.0 mas represents a maximum extension of the star or its mean, or whether the 2008 figure of 47.0 in fact represents a minimum, is unclear until a full cycle of data has been gathered. It will probably take until around 2025 before we can assert with any certainty, meaning that the Jovian orbit of 5.5 AU will probably serve as the star's "average" radius for some time.[67][68]
Once considered as having the largest angular diameter of any star in the sky after the Sun, in 1997 Betelgeuse lost that distinction when a group of astronomers measured R Doradus with a diameter of 57.0 ± 0.5 mas. Betelgeuse is now considered to be in third place, although R Doradus, being much closer to Earth at about 200 ly, has an actual diameter roughly one-third that of Betelgeuse.[69]
Properties
Betelgeuse's spectral class is listed as M2Iab in the SIMBAD astronomical database, signifying that it is a red Class M star.[2] Since 1943, the spectrum of this star has served as one of the stable anchor points by which other stars are classified.[70] The "ab" suffix is derived from the Yerkes spectral classification system, and indicates that it is an intermediate luminous supergiant, less bright than other supergiants like Deneb.[71] However, given some of the recent findings, this classification may be outdated, as there is evidence Betelgeuse is actually much more luminous than Deneb and other stars in its class.[36]
Assuming average radius of 5.5 AU and a distance of 197 pc, theoretical calculations would yield a luminosity figure in excess of 180,000 Suns (L☉) at maximum.[4][68] When the star contracts as it appears to have since 1993, its luminosity would diminish to about 130,000L☉. Either way, that amount of electromagnetic energy dwarfs Deneb's output of about 50,000L☉.[note 4] But with most of the star's radiant energy occurring in the infrared and huge amounts being absorbed by circumstellar matter, the human eye simply cannot perceive the star's intrinsic brightness.
Given the many uncertainties surrounding Betelgeuse, no consensus has yet emerged regarding the star's mass. Estimates range from 5 to 30 solar masses (M☉) with most investigators showing a preference for a relatively large mass ranging from 10 to 20M☉.[72][73] One model reports a mass at the lower end of the scale at 14M☉, although a mass ranging from 18 to 20 is more commonplace.[6][72]
Typical of red supergiants, Betelgeuse is a cool star with surface temperatures in the last decade reported between 3,500 to 3,600K.[9][10][73] It is also a slow rotator, with the most recent velocity recorded at 5 km/s.[10] Depending on its photospheric radius, it could take the star anywhere from 25 to 32 years to turn on its axis—extremely slow when compared with a fast rotator like Pleione in the Pleiades star cluster, which turns on its axis once every 11.8 hours.[74]
In 2002, astronomers using sophisticated computer simulations began to speculate that Betelgeuse might exhibit magnetic activity in its extended atmosphere, a factor where even moderately strong fields could have a meaningful influence over the star's dust, wind and mass-loss properties.[75] A series of spectropolarimetric observations obtained in 2010 with the Bernard Lyot Telescope at Pic du Midi Observatory revealed the presence of a weak magnetic field at the surface of Betelgeuse, suggesting that the giant convective motions of supergiant stars are able to trigger the onset of a small-scale dynamo.[76]
Space motion

The kinematics of Betelgeuse are not easily explained. The age of a Class M supergiant with an initial mass of 20M☉ is roughly 10 million years.[4][77] Given its current space motion, a projection back in time would take Betelgeuse around 290 parsecs farther from the galactic plane where there is no star formation region—an implausible scenario. Although the radial velocity and proper motion for the 25 Ori subassociation have yet to be measured, Betelgeuse's projected pathway across the heavens does not appear to intersect with it either. Also, formation close to the far younger Orion Nebula Cluster (ONC, also known as Ori OB1d) is doubtful. Very Long Baseline Array astrometry yields a distance to the ONC between 389 and 414 parsecs. Consequently, it is likely that Betelgeuse has not always had its current motion through space and has changed course at one time or another, possibly the result of a nearby stellar explosion.[4][78]
The most likely star-formation scenario for Betelgeuse is that it is a runaway star from the Orion OB1 Association. Originally a member of a high-mass multiple system within Ori OB1a, Betelgeuse was probably formed about 10–12 million years ago from the molecular clouds observed in Orion, but has evolved rapidly due to its unusually high mass.[4]
Like many of the young stars in Orion where masses greater than 10 solar can be found in abundance, Betelgeuse will use its fuel quickly and not live very long. On the Hertzsprung-Russell diagram, Betelgeuse has moved off the main sequence and has swelled and cooled to become a red supergiant. Although young, Betelgeuse has probably exhausted the hydrogen in its core—unlike its OB cousins born about the same time—causing it to contract under the force of gravity into a hotter and denser state. As a result, it has begun to fuse helium into carbon and oxygen producing enough radiation to unfurl its outer envelopes of hydrogen and helium. Its extreme luminosity is being generated by a mass so large that the star will eventually fuse higher elements through neon, magnesium, sodium, and silicon all the way to iron, at which point it will probably collapse and explode as a supernova.[6][47]
Density

1. Mercury < Mars < Venus < Earth
2. Earth < Neptune < Uranus < Saturn < Jupiter
3. Jupiter < Wolf 359 < Sun < Sirius
4. Sirius < Pollux < Arcturus < Aldebaran
5. Aldebaran < Rigel < Antares < Betelgeuse
6. Betelgeuse < Mu Cephei < VV Cephei A < VY Canis Majoris.
As an early M-type supergiant, Betelgeuse is one of the largest, most luminous and yet one of the most ethereal stars known. A radius of 5.5 AU is roughly 1,180 times the radius of the Sun—a sphere so huge that it could contain over 2 quadrillion Earths (2.15 × 1015) or more than 1.6 billion (1.65 × 109) Suns. That is the equivalent of Betelgeuse being a giant football stadium like Wembley Stadium in London with the Earth a tiny pearl, 1 millimeter in diameter, orbiting a Sun the size of a mango.[note 5] Sirius, by contrast, with a radius of 1.71R☉, would be roughly the size of a soccer ball. Moreover, recent observations of Betelgeuse exhibiting a 15% contraction in angular diameter would equate to a shortening of the star's radius from about 5.5 to 4.6 AU, assuming that the photosphere is a perfect sphere. A reduction of this magnitude would correspond to a diminution in photospheric volume of approximately 41% or 680 million Suns.[note 6]

Not only is the photosphere enormous, but the star is surrounded by a vast and complex circumstellar environment where light could take over three years just to escape.[79] In the outer reaches of the photosphere, the density is extremely low. In volume, Betelgeuse exceeds the Sun by a factor of about 1.6 billion. Yet the actual mass of the star is believed to be no more than 18 to 19 Suns (M☉), with certain mass loss estimates projected at one to two Suns since birth.[6] Consequently, the average density of this stellar mystery is less than twelve parts-per-billion (1.119 × 10−8) that of the Sun. Comparing such star matter to the density of ordinary air at sea level, the ratio is roughly 1.286 × 10−5, a density so ethereal, one would have to soar above the noctilucent clouds in the Earth's mesosphere to experience it.[note 7] Such star matter is so tenuous, in fact, that Betelgeuse has often been called a "red-hot vacuum".[17][18]
Circumstellar dynamics
In the late phase of stellar evolution, massive stars like Betelgeuse exhibit high rates of mass loss, possibly as much as 1M☉ every 10,000 years, resulting in a complex circumstellar environment that is constantly in flux.[36] All stars exhibit mass loss. Rates vary from about 10−14 to 10−4 yr -1 depending on spectral type, luminosity class, rotation rate, companion proximity, and evolutionary stage.[80] Exactly how this mass loss occurs, however, has been a mystery confronting astronomers for decades. When Schwarzschild first proposed his theory of monster convection cells, he argued it was the likely cause in red supergiants. Prior attempts to explain mass loss in terms of solar wind theory had proven unsuccessful as they led to a contradiction with observations involving circumstellar shells.[28] Other theories that have been advanced include magnetic activity, global pulsations and shock structures as well as stellar rotation.[23]

As a result of work done by Pierre Kervella and his team at the Paris observatory in 2009, astronomers may be close to solving this mystery. What Kervella noticed was a large plume of gas extending outward at least six times the stellar radius indicating that the star is not shedding matter evenly in all directions.[10][36] The plume's presence, in fact, implies that the spherical symmetry of its photosphere, often observed in the infrared, is not preserved in its close environment. Asymmetries on the stellar disk had been reported many times at different wavelengths. However, due to the refined capabilities of the NACO adaptive optics on the VLT, these asymmetries have come into focus. The two mechanisms that could cause such asymmetrical mass loss, Kervella noted, were large-scale convection cells or polar mass loss, possibly due to rotation.[10] Probing deeper still with the AMBER instrument on ESO's Very Large Telescope Interferometer, Keiichi Ohnaka from the Max Planck Institute in Bonn observed that the gas in the supergiant's extended atmosphere is vigorously moving up and down, creating bubbles as large as the supergiant itself, leading his team to conclude that such stellar upheaval is behind the massive plume ejection observed by Kervella.[36][81]
Evidence of circumstellar shells surrounding M supergiants was first proposed by Walter Adams and Elizabeth MacCormack in 1935 when they observed anomalies in the spectral signature of such stars and concluded that the likely cause was an expanding gaseous envelope.[35][82] The first indication of vastly extended envelopes occurred in 1955 with the work of Armin Deutsch who noticed when studying the Rasalgethi system that spectroscopic peculiarities were mysteriously occurring in the G star companion, α2 Her. This led him to conclude that the whole system had to be enveloped by a circumstellar shell composed of matter being ejected by the main star, M supergiant α1 Her, and extending to at least 170 stellar radii.[35][83] In the mid 1970s, Andrew Bernat undertook a detailed analysis of four circumstellar shells, Betelgeuse, Antares, Rasalgethi and Mu Cephei, concluding that red stars dominate mass return to the Galaxy.[35]
In addition to the photosphere, researchers have now identified six other components of Betelgeuse's complex atmosphere. Extending outward, we find a compact molecular environment otherwise known as the MOLsphere, a gaseous envelope, a chromosphere, a dust environment and two outer shells (S1 and S2) composed of carbon monoxide (CO). There is also evidence of coronal plasma in the star's extended atmosphere, a phenomenon that heretofore was not believed to exist in late stage stars off the main sequence.[72] Some of these elements are known to be asymmetric while others overlap.[64]

At about .45 stellar radii (~2−3 AU) above the photosphere, the closest membrane appears to be the molecular layer known as the MOLsphere. Studies show it to be composed of water-vapor and carbon monoxide with an effective temperature of about 1500 ± 500K.[64][84] Water-vapor had been originally detected in the supergiant's spectrum back in the 1960s with the two Stratoscope projects but had been ignored for decades. Recent studies suggest that the MOLsphere may also contain SiO and Al2O3—molecules which could explain the formation of dust particles.

Between two and seven stellar radii (~10−40 AU), astronomers have identified another region known as an asymmetric gaseous envelope composed of elemental abundances C, N and O.[64][85] The radio-telescope images taken in 1998 confirm that Betelgeuse has a dense atmosphere with a "remarkably complex structure".[86] Observations show the atmosphere to be boiling with a temperature of 3,450 ± 850K—similar to the temperature recorded on the star's surface but much lower than surrounding gas in the same region.[86][87] The VLA images also showed this lower-temperature gas progressively decreasing in temperature as it extends outward—the existence of which, although unexpected, turns out to be the most abundant constituent of Betelgeuse's atmosphere. "This alters our basic understanding of red-supergiant star atmospheres", explained Jeremy Lim, the team's leader. "Instead of the star's atmosphere expanding uniformly because of gas heated to very high temperatures near its surface, it now appears that several giant convection cells propel gas from the star's surface into its atmosphere."[86] This is the same region in which Kervella's 2009 finding of a bright plume, possibly containing CN and extending at least six photospheric radii in the southwest direction of the star, is believed to exist.[64]
The chromosphere, as mentioned earlier, was resolved in 1996 at about 2.2 times the optical disk (~10 AU) at ultraviolet wavelengths and is reported to have a temperature no higher than 5,500K.[50][64] The image was taken with the Faint Object Camera on-board the Hubble Space Telescope and also revealed a bright area in the southwest quadrant of the disk. However in 2004 observations with the STIS, Hubble's high-precision spectrometer, pointed to the existence of warm chromospheric plasma at least one arcsecond away from the star. At a distance of 197 pc, the size of the chromosphere could be 200 AU or seven times the Neptunian orbit.[88] The CfA team led by Alex Lobel concluded that the spatially resolved STIS spectra directly demonstrate the co-existence of warm chromospheric plasma with cool gas in Betelgeuse's circumstellar dust envelope.[88]
The first attestation of a dust shell surrounding Betelgeuse was put forth by Sutton et al. when the team noted in 1977 that dust shells around mature stars often emit large amounts of radiation in excess of the photospheric contribution. Using heterodyne interferometry, the team concluded that Betelgeuse emits the majority of its excess outside 12 stellar radii or roughly the distance of the Kuiper belt at 50 to 60 AU, depending on the assumed stellar radius.[64][89] Since then, there have been numerous studies done of this dust envelope at varying wavelengths yielding decidedly different results. More recent studies have estimated the inner radius of the dust shell anywhere from 0.5 to 1.0 arcseconds, hence 25 to 50 stellar radii or 100 to 200 AU.[90][91] What these studies point out is that the dust environment surrounding Betelgeuse is anything but static. In 1994, Danchi et al. reported that Betelgeuse undergoes sporadic dust production involving decades of activity followed by inactivity. A few years later, a group of astronomers led by Chris Skinner noticed significant changes in the dust shell's morphology in just one year, suggesting that the shell is asymmetrically illuminated by a stellar radiation field strongly affected by the existence of photospheric hot spots.[90] The 1984 report of a giant asymmetric dust shell located 1 pc (206,265 AU) from the star has not been corroborated in recent studies, although another report published the same year said that three dust shells were found extending four light years from one side of the decaying star, suggesting that, like a snake, Betelgeuse sheds its outer layers as it journeys across the heavens.[79][92]
Although the exact size of the two outer CO shells remains elusive, preliminary estimates suggest that one shell extends from about 1.5 to 4.0 arcseconds with the other expanding as far as 7.0 arcseconds.[93] Using the Jovian orbit of 5.5 AU as the "average" radius for this gargantuan star, (~0.055" diameter), the inner shell would extend from roughly 50 to 150 stellar radii (~300 to 800 AU) with the outer shell extending out as far as 250 stellar radii (~1400 AU). With the heliopause estimated at about 100 AU, the size of this outer shell is almost fourteen times the size of the Solar System.
Approaching supernova
The future fate of Betelgeuse depends on its mass—a critical factor which is not well understood.[73] Since most investigators concede a mass greater than 10M☉, the most likely scenario is that the supergiant will continue to burn and fuse elements until its core is iron, at which point Betelgeuse will explode as a type II supernova. During this event the core will collapse, leaving behind a neutron star remnant some 20 km in diameter.[19]
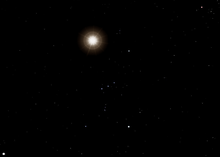

Betelgeuse is already old for its size class and is expected to explode relatively soon compared to its age.[36] Solving the riddle of mass-loss will be the key to knowing when a supernova may occur, an event expected anytime in the next million years.[38][95][96] Supporting this hypothesis are a number of unusual features that have been observed in the interstellar medium of the Orion Molecular Cloud Complex, which suggest that there have been multiple supernova explosions in the recent past.[78] Betelgeuse's suspected birthplace in the Orion OB1 Association is the probable location for such supernovae. Since the oldest subgroup in the association has an approximate age of 12 million years, the more massive stars likely had sufficient time to evolve to this stage. Also, because runaway stars are believed to be caused by supernova explosions, there is strong evidence that OB stars μ Columbae, AE Aurigae and 53 Arietis all originated with such an explosion in Ori OB1 2.2, 2.7 and 4.9 million years ago.[78]
At its current distance from Earth, such a supernova explosion would be the brightest recorded, outshining the Moon in the night sky and becoming easily visible in broad daylight.[36] Professor J. Craig Wheeler of The University of Texas at Austin predicts the supernova will emit 1053 ergs of neutrinos, which will pass through the star's hydrogen envelope in around an hour, then reach the solar system several centuries later. Since its rotational axis is not pointed toward the Earth, Betelgeuse's supernova is unlikely to send a gamma ray burst in the direction of Earth large enough to damage ecosystems.[96] The flash of ultraviolet radiation from the explosion will likely be weaker than the ultraviolet output of the Sun. The supernova could brighten to an apparent magnitude of −12 over a two-week period, then remain at that intensity for 2 to 3 months before rapidly dimming. The year following the explosion, radioactive decay of cobalt to iron will dominate emission from the supernova remnant, and the resulting gamma rays will be blocked by the expanding envelope of hydrogen. If the neutron star remnant becomes a pulsar, then it could produce gamma rays for thousands of years.[97]
Star system
In 1985, Margarita Karovska, in conjunction with other astrophysicists at the CfA, announced the discovery of two close companions orbiting Betelgeuse. Analysis of polarization data from 1968 through 1983 indicated a close companion with a periodic orbit of about 2.1 years. The team realized that the observed polarization could be caused by a systemic asymmetry created by the close companion orbiting the supergiant inside its extended dust envelope. Using speckle interferometry, the team concluded that the closer of the two companions was located at 0.06 ± 0.01" (~9 AU) from the main star with a position angle (PA) of 273 degrees, an orbit that would potentially place it within the star's chromosphere. The more distant companion was estimated at 0.51 ± 0.01" (~77 AU) with a PA of 278 degrees. The magnitude differences with respect to the primary, measured at 656.3 (Hα) and 656.8 nm (red continuum), were 3.4 and 3.0 for the close component and 4.6 and 4.3 for the distant component.[98][99]


In the years that followed, astronomers conducted a number of studies in the hope of obtaining additional confirmation. In 1987, Andrea Dupree, one of Karovska's colleagues at the CfA, observed: "Periastron of the recently discovered close optical companion to Alpha Ori is predicted to be 1986.7; detection of atmospheric disturbances similar to those found subsequent to the last periastron (~ 1984.6) would give strong support to the presence of a companion."[101] However, in the years that followed no such confirmation was ever published. Rather, in 1990, David F. Buscher, John E. Baldwin and a team of collaborators from the Cavendish Astrophysics Group made a number of high-resolution images of the supergiant at wavelengths of 633, 700, and 710 nm using the nonredundant masking method. At all these wavelengths, they remarked, there was unambiguous evidence for an asymmetric feature on the surface of the star, which contributed 10−15 percent of the star's total observed flux. Their conclusion was that such a phenomenon could be caused by a close companion passing in front of the stellar disk, differential photospheric brightening due to the effects of stellar rotation or the more likely scenario of "large-scale convection in the stellar atmosphere" as suggested by Schwarzschild.[24]
The Cavendish colleagues published another paper in 1992, this time under the helm of Richard. W. Wilson, noting that the brightness features on the surface of Betelgeuse appear to be "too bright to be associated with a passage of the suggested companions in front of the red giant." They also noticed that these features were fainter at 710 nanometers compared to 700 by a factor of 1.8, indicating that such features would have to reside within the molecular atmosphere of the star.[102]
That same year, Karovska published a new paper reconfirming her team's exegesis, but also noting that there was a meaningful correlation between the calculated position angles of the orbiting companion and the reported asymmetries, suggesting a possible connection between the two. She observed that any such correlation could be caused one of three factors: a companion star, tidal distortion of the supergiant's atmosphere, or by a photospheric bright spot. She concludes: "To determine the nature of the companion (which presently remains a puzzle), it is crucial to obtain further speckle observations using large aperture telescopes, coordinated with other ground-based observations and the observations from space."[103]
Since then, researchers have turned their attention to analyzing the intricate dynamics of the star's extended atmosphere and little else has been published on the possibility of orbiting companions, although as Xavier Haubois and his team reiterate in 2009, the possibility of a close companion contributing to the overall flux has never been fully ruled out.[64] Dommanget's double star catalog (CCDM) lists at least four adjacent stars, all within three arcminutes of this stellar giant, yet aside from apparent magnitudes and position angles, little else is known.[104] As the decade unfolds and new technologies are brought to unraveling the star's enigmatic past, we will likely see conclusive evidence, one way or another, of any potential star system. Given the planned capabilities of the upcoming Gaia mission, a confirmation could occur any time after the mission's scheduled launch in December 2012.[105]
Name
Spelling
Betelgeuse has been known variously as Betelgeux,[1] and Beteigeuze[106] in German (according to Bode[107][108]). Betelgeux and Betelgeuze were used until the early 20th century, when the spelling Betelgeuse became universal.[109]
Pronunciation
There is no consensus for the correct pronunciation of the name,[110] and pronunciations for the star are as varied as its spellings:
- /ˈbɛtəldʒuːz/ Oxford English Dictionary[1] and Royal Astronomical Society of Canada
- /ˈbiːtəldʒuːz/ Oxford English Dictionary[1]
- /ˈbɛtəldʒəz/ (The Friendly Stars)[111]
- /ˈbiːtəldʒuːs/ (Canadian Oxford Dictionary, Webster's Collegiate Dictionary)
- /ˈbɛtəldʒuːs/ [111]
Etymology
The last part of the name, "-elgeuse", comes from the Arabic الجوزاء al-Jauzā', a historical Arabic name of the constellation Orion, a feminine name in old Arabian legend, and of uncertain meaning. Because جوز j-w-z, the root of jauzā', means "middle", al-Jauzā' roughly means "the Central One". Later, al-Jauzā' was also designated as the scientific Arabic name for Orion and for Gemini. The current Arabic name for Orion is الجبار al-Jabbār ("the Giant"), although the use of الجوزاء al-Jauzā' in the name of the star has continued.[112]
There is some uncertainty surrounding the first element of the name, rendered as "Bet-". However, "abet" or إبط is the Arabic word for "armpit",[113] which is where the star is located in the constellation. Betelgeuse is often mistranslated as "armpit of the central one".[114]
In his 1899 work Star-Names and Their Meanings, American amateur naturalist Richard Hinckley Allen stated the derivation was from the ابط الجوزاء Ibṭ al-Jauzah, which he claimed degenerated into a number of forms including Bed Elgueze, Beit Algueze, Bet El-gueze, Beteigeuze and more, to the (then) current forms Betelgeuse, Betelguese, Betelgueze and Betelgeux. The star was named Beldengeuze in the Alfonsine Tables, and Italian Jesuit priest and astronomer Giovanni Battista Riccioli had called it Bectelgeuze or Bedalgeuze.[12]
Paul Kunitzsch, Professor of Arabic Studies at the University of Munich, refuted Allen's derivation and instead proposed that the full name is a corruption of the Arabic يد الجوزاء Yad al-Jauzā' meaning "the Hand of al-Jauzā', i.e., Orion.[115] European mistransliteration into medieval Latin led to the first character y (ﻴ, with two dots underneath) being misread as a b (ﺒ, with only one dot underneath). During the Renaissance, the star's name was written as بيت الجوزاء Bait al-Jauzā' ("house of Orion") or بط الجوزاء Baţ al-Jauzā', incorrectly thought to mean "armpit of Orion" (a true translation of "armpit" would be ابط, transliterated as Ibţ). This led to the modern rendering as Betelgeuse.[112] Other writers have since accepted Kunitzsch's explanation.[6] The 17th century English translator Edmund Chilmead gave it the name Ied Algeuze ("Orion's Hand"), from Christmannus.[12]
Other Arabic names recorded include Al Yad al Yamnā ("the Right Hand"), Al Dhira ("the Arm"), and Al Mankib ("the Shoulder"), all appended to "of the giant",[12] as منكب الجوزاء Mankib al Jauzā'. In Persian, however, the name is اِبطالجوزا, derived from the Arabic ابط الجوزاء Ibţ al-Jauzā', "armpit of Orion".
Allen linked Orion's association with stormy weather to that of this deity of storms.[12] Bahu was its Sanskrit name, as part of a Hindu understanding of the constellation as a running antelope or stag.[12] Other terms included the Persian Bašn "the Arm" via Brown, and Coptic Klaria "an Armlet".[12]
A Sanskrit name for Betelgeuse was ãrdrã "the moist one", eponymous of the Ardra lunar mansion in Hindu astrology.[citation needed] In traditional Chinese astronomy, Betelgeuse was known as 参宿四 (Shēnxiùsì, the Fourth (Star of the constellation) of Three (Stars)) as the constellation of 参宿 was at first a name only for the three stars in the girdle of Orion. Four stars were later added to this constellation, but the earlier name stuck.[citation needed] In Japan, this star was called Heike-boshi (suggested by the red butterfly flag of the Heike clan), (平家星),[116][117] "the Star of the Heike clan" or Kin-waki, (金脇), "the Gold (Star) beside (Mitsu-boshi)." In Malayalam Thiruvathira തിരുവാതിര and in Tamil the star is named Thiruvathirai திருவாதிரை.
In Tahitian lore, Betelgeuse was one of the pillars propping up the sky, known as Anâ-varu, the pillar to sit by. It was also called Ta'urua-nui-o-Mere "Great festivity in parental yearnings".[118]
In popular culture
The star's unusual name inspired the 1988 film Beetlejuice, and script writer Michael McDowell was impressed at how many people made the connection. He added they had received a suggestion the sequel be named Sanduleak-69 202 after the former star of SN 1987A.[109] In August Derleth's short story "The Dweller in the Darkness" set in H. P. Lovecraft's Cthulhu Mythos, Betelgeuse is the home of the 'benign' Elder Gods.[119] There has been much debate over the identity of the red star Borgil mentioned in Lord of the Rings, with Aldebaran, Betelgeuse and even the planet Mars touted as candidates. Professor Kristine Larsen has concluded the evidence points to it being Aldebaran as it precedes Menelvagor (Orion).[120] Astronomy writer Robert Burnham, Jr. proposed the term padparadaschah which denotes a rare orange sapphire in India, for the star.[109] In the popular science fiction series "The Hitchhiker's Guide to the Galaxy" by Douglas Adams, Ford Prefect was from "a small planet somewhere in the vicinity of Betelgeuse."[119]
There have been two American navy ships named after the star, both World War II vessels, the USS Betelgeuse (AKA-11) launched in 1939 and USS Betelgeuse (AK-260) launched in 1944. In 1979, a French supertanker named Betelgeuse was moored off Whiddy Island discharging oil when it exploded, killing 50 people in one of the worst disasters in Ireland's history.[121]
Italian electronica band Overmamba released a song on Crawl Out, their debut album, called Betelgeuse and largely inspired by the star; the lyrics tell of a fantasy space-time warping trip around the star and its planets.[citation needed]
See also
- List of brightest stars
- List of largest known stars
- List of most luminous stars
- List of most massive stars
- List of supernova candidates
- Betelgeuse in fiction
Notes
- ^ a b The following table provides a non-exhaustive list of angular measurements conducted since 1920. Also included is a column providing a current range of radii for each study based on Betelgeuse's most recent distance estimate (Harper et al) of 197±45 pc:
Article Year1 Telescope # Spectrum λ (μm) ∅ (mas)2 Radii3 @
197±45 pcNotes Michelson 1920 Mt-Wilson 1 Visible 0.575 47.0 ± 4.7 3.2–6.3 AU Limb darkened +17% = 55.0 Bonneau 1972 Palomar 8 Visible 0.422–0.719 52.0–69.0 3.6–9.2 AU Strong correlation of ∅ with λ Balega 1978 ESO 3 Visible 0.405–0.715 45.0–67.0 3.1–8.6 AU No correlation of ∅ with λ 1979 SAO 4 Visible 0.575–0.773 50.0–62.0 3.5–8.0 AU Buscher 1989 WHT 4 Visible 0.633–0.710 54.0–61.0 4.0–7.9 AU Discovered asymmetries/hotspots Wilson 1991 WHT 4 Visible 0.546–0.710 49.0–57.0 3.5–7.1 AU Confirmation of hotspots Tuthill 1993 WHT 8 Visible 0.633–0.710 43.5–54.2 3.2–7.0 AU Study of hotspots on 3 stars 1992 WHT 1 NIR 0.902 42.6 ± 0:03 3.0–5.6 AU Weiner 1999 ISI 2 MIR (N Band) 11.150 54.7 ± 0.3 4.1–6.7 AU Limb darkened = 55.2 ± 0.5 Perrin 1997 IOTA 7 NIR (K Band) 2.200 43.33 ± 0.04 3.3–5.2 AU K&L Band,11.5μm data contrast Haubois 2005 IOTA 6 NIR (H Band) 1.650 44.28 ± 0.15‡ 3.4–5.4 AU Rosseland diameter 45.03 ± 0.12 Hernandez 2006 VLTI 2 NIR (K Band) 2.099–2.198 42:57 ± 0:02 3.2–5.2 AU High precision AMBER results. Ohnaka 2008 VLTI 3 NIR (K Band) 2.280–2.310 43.19 ± 0.03 3.3–5.2 AU Limb darkened 43.56 ± 0.06 Townes 1993 ISI 17 MIR (N Band) 11.150 56.00 ± 1.00 4.2–6.8 AU Systematic study involving 17 measurements at the same wavelength from 1993–2009 2008 ISI MIR (N Band) 11.150 47.00 ± 2.00 3.6–5.7 AU 2009 ISI MIR (N Band) 11.150 48.00 ± 1.00 3.6–5.8 AU Harper 2004 VLA 1The final year of observations, unless otherwise noted. 2Uniform disk measurement, unless otherwise noted. 3Radii calculations use the same methodology as outlined in Note #2 below ‡Limb darkened measurement.
- ^ To determine the average radius of Betelgeuse in terms of solar units, the calculations begin with the formula for angular diameter as follows:
- .
- .
- There are significant error factors in the two variables that make up this formula, the angular diameter () and distance (),
- The error factors yield a radius range for Betelgeuse between 4.2 to 6.9 AU, and
- The hypothesis that the star's radius continually expands and contracts,
To convert 5.5 AU into Solar units, the math is straightforward. Since 1 AU = 149,597,871 km and the mean diameter of the Sun = 1,392,000 km (hence a mean radius of 696,000 km), the calculation is as follows:- (rounded).
- ^ Betelgeuse's reported radius of 56.0 mas in 1993 equates to 5.516 AU (1,185 ), assuming a distance of 197.0 pc. The reported angular diameter of 47.0 mas from 2008 would equate to a radius of 4.630 AU (995 ), hence a shrikange of 0.887 AU, a little more than the orbit of Venus, and just under the orbit of the Earth.
To compute the average speed of contraction, the only missing variable is time. The 1993 measurement was taken on October 30th; the 2008 figure, on October 29th—hence a time frame of 5,478 days. Therefore:
0.887 AU × 149,597,871 km ÷ 5,478 days ÷ 24 hours ≈ 1,008 km/h.
It should also be noted that any speed calculation is highly dependent on the estimated distance (parallax) to Betelgeuse. Using the Hipparcos distance to Betelgeuse (131 pc or 425 ly) in lieu of the Harper estimate, the speed drops to 671 km/h. - ^
To understand the luminosity calculations below, the first step is to clearly delineate between the two forms of luminosity: apparent luminosity (counting visible light only) and bolometric luminosity (total radiant energy), sometime's referred to as intrinsic luminosity. The formula for bolometric luminosity is as follows:
- where... B = Betelgeuse, L = Luminosity, R = Radius and T = Temperature.
- ^ The analogy is based on the computation of certain ratios – specifically the diameter, radius and volume of the 3 celestial bodies in question, Betelgeuse, the Sun and Earth. Once these ratios are derived, the relative size of each as they relate to Wembley Stadium can be easily determined. The calculations begin with the formula for angular diameter as follows:
- Betelgeuse diameter ≈ 0.0552 arcseconds × 197.0 pc ≈ 11.000 AU (rounded up) × 149,597,871 km ≈ 1.646 ×109 km,
- Betelgeuse radius ≈ 11.000 AU ÷ 2 ≈ 5.500 AU × 149,597,871 km ≈ 8.230 ×108 km ≈ 823,000,000 km,
- Betelgeuse volume ≈ (4÷3×π) × 823,000,0003 ≈ 2.335 × 1027 km3.
- Solar radius ≈ 696,000 km. Volume ≈ 1.412×1018 km3,
- Earth radius ≈ 6,371.0 km. Volume ≈ 1.083×1012 km3.
- Wembley Bowl Volume ≈ 1,139,100 m3. Spherical radius ≈ (1,139,100m3 ÷ (4/3*π))1/3 ≈ 64.787 m or 64,787 mm.
- Betelgeuse ≈ (2.335 × 1027) ÷ (1.412×1018) ≈ 1.654 × 109 Suns,
- Betelgeuse ≈ (2.335 × 1027) ÷ (1.083×1012) ≈ 2.156 × 1015 Earths.
- Solar volume relative to Wembley ≈ 1,139,100 m3 ÷ (1.654 × 109) × 109{i.e. to convert to mm3} ≈ 689,000 mm3 (rounded up)
- Solar diameter relative to Wembley ≈ (689,000 mm3 ÷ (4/3*π))1/3 ≈ 55.61845 × 2 ≈ 110 mm or 4.3 inches
- Earth volume relative to Wembley ≈ 1,139,100 m3 ÷ (2.156 × 1015) × 109{i.e. to convert to mm3} ≈ 0.528 mm3
- Earth diameter relative to Wembley ≈ (0.528 mm3 ÷ (4/3*π))1/3 ≈ 0.501 × 2 ≈ 1.002 mm or 0.04 inches
- Earth's orbital radius (1 AU) relative to Wembley ≈ 1 AU ÷ 5.5 AU × 64.787 meters = 11.8 m
- If the immense space of Wembley Stadium were Betelgeuse, the Earth would be a tiny pearl, 1.0 mm in diameter, orbiting a Sun, 11.0 cm/4.3 inches in diameter (i.e. the size of an average mango or grapefruit), with an orbital distance of about 11.8 meters.
- ^ As pointed out in the Angular anomalies section, the observed contraction could be due to a shrinking of the star's radius or by other phenomena. Assuming the photosphere is spherical, calculating a reduction in volume begins with the formula for angular diameter as follows:
Calculations for 1993 values:
- Betelgeuse radius ≈ 0.056 arcseconds × 197.0 pc ≈ 11.032 AU ÷ 2 ≈ 5.516 AU × 149,597,871 km ≈ 825,000,000 km,
- Betelgeuse volume ≈ (4÷3×π) × 825,000,0003 ≈ 2.352 × 1027 km3.
- Betelgeuse radius ≈ 0.047 arcseconds × 197.0 pc ≈ 9.260 AU ÷ 2 ≈ 4.630 AU × 149,597,871 km ≈ 692,500,000 km,
- Betelgeuse volume ≈ (4÷3×π) × 692,500,0003 ≈ 1.391 × 1027 km3.
- Betelgeuse change in volume ≈ 2.352 × 1027 km3 – 1.391 × 1027 km3 ≈ –9.610E × 1026 km3
- Betelgeuse percent change in volume ≈ –9.610E × 1026 km3 ÷ 2.352 × 1027 km3 ≈ –40.86%
- Betelgeuse volume change as a function of Solar volume ≈ –9.610E × 1026 ÷ 1.412×1018 km3 ≈ –681,000,000 Suns.
- ^ To compute these density ratios, the first step is to calculate the mass and volume of each component from which density calculations can be made. Given the density of the Sun, Betelgeuse, and the Earth's atmosphere, dividing gives the appropriate ratios.
Calculations for the Sun:
- Solar mass ≈ 1.9891×1030 kg
- Solar volume ≈ 1.412×1018 km3.
- Solar density ≈ 1.9891×1030 ÷ (1.412×1018 × 109) ≈ 1.409×103 kg/m3.
- Betelgeuse mass ≈ 18.5 x Solar × 1.9891×1030 ≈ 3.680 ×1031 kg
- Betelgeuse volume ≈ 2.335 × 1027 km3 (from previous note)
- Betelgeuse density ≈ 3.680 ×1031 kg ÷ (2.335 × 1027 km3 × 109) ≈ 1.576 ×10−5 kg/m3.[clarification needed]
- Atmospheric Mass ≈ 5.148 × 1018 kg
- Atmospheric Volume ≈ 4.200 × 1009 km3.
- Atmospheric Density ≈ 5.148 × 1018 kg ÷ 4.200 × 1009 km3 × 109 ≈ 1.226 kg/m3.
- Betelgeuse/Sun ≈ 1.576 × 10−5 kg/m3 ÷ 1.409 × 103 kg/m3 ≈ 1.119 × 10−8
- Betelgeuse/Earth's Atmosphere = 1.576 × 10−5 kg/m3 ÷ 1.226 × 100 kg/m3 ≈ 1.286 × 10−5
- Betelgeuse's average density relative to Earth's Atmosphere is graphically represented in the NRLMSISE-00 standard atmosphere model. A density of 1.286 × 10−8 g/cm3,[clarification needed] is roughly equivalent to the Earth's atmospheric density found at about 90 kilometers above sea level. Noctilucent clouds are found between 76 to 85 km above sea level.
References
- ^ a b c d e Simpson, J.; Weiner, E. (eds), ed. (1989). "Betelgeuse". Oxford English Dictionary (2nd ed.). Oxford: Clarendon Press. p. 130. ISBN 0-19-861186-2.
{{cite encyclopedia}}
:|editor=
has generic name (help)CS1 maint: multiple names: editors list (link) - ^ a b c d e f g h "SIMBAD query result: BETELGEUSE – Semi-regular pulsating Star". Centre de Données astronomiques de Strasbourg. Retrieved 2007-06-20.
- ^ a b Nicolet, B. (1978). "Catalogue of homogeneous data in the UBV photoelectric photometric system". Astronomy and Astrophysics. 34: 1–49. Bibcode:1978A&AS...34....1N.
- ^ a b c d e f g h i j k l m n Harper, Graham M.; Brown, Alexander; Guinan, Edward F. (2008). "A New VLA-Hipparcos Distance to Betelgeuse and its Implications" (PDF). The Astronomical Journal. 135 (4): 1430–40. Bibcode:2008AJ....135.1430H. doi:10.1088/0004-6256/135/4/1430. Retrieved 2010-07-10.
{{cite journal}}
: Unknown parameter|month=
ignored (help)CS1 maint: multiple names: authors list (link) - ^ Note: Absolute magnitude calculations can vary significantly depending on the assumed parallax measurements. An absolute magnitude of −6.05 assumes the SIMBAD recorded average apparent magnitude for Betelgeuse of 0.42 and the most recent distance estimates of 197 parsecs. Given a variability of 0.2 – 1.2, the absolute magnitude can be said to vary between – 6.27 to −5.27
- ^ a b c d e f Kaler, James B. (Jim). "Betelgeuse (Alpha Orionis)". Stars website. University of Illinois. Retrieved 2009-07-19.
- ^ See Note #2 for calculations
- ^ A luminosity of 140,000 Suns is an imputed figure based on various considerations. Two of the most important variables that influence luminosity calculations are distance (See Enigmatic parallax) and angular separation (See Angular anomalies). The calculations can be seen in Note #4 with added discussion found at the Luminosity section on the discussion page.
- ^ a b c Lobel, Alex; Dupree, Andrea K. (2000, December). "Modeling the Variable Chromosphere of α Orionis" (PDF). The Astrophysical Journal,. 545 (1): 454–74. Bibcode:2000ApJ...545..454L. doi:10.1086/317784. Retrieved 2010-07-10.
{{cite journal}}
: Check date values in:|year=
(help)CS1 maint: extra punctuation (link) CS1 maint: multiple names: authors list (link) - ^ a b c d e f g Kervella, P.; Verhoelst, T.; Ridgway, S. T.; Perrin, G.; et al. (2009). "The close circumstellar environment of Betelgeuse. Adaptive optics spectro-imaging in the near-IR with VLT/NACO". Astronomy and Astrophysics. 504 (1): 115–25. Bibcode:2009A&A...504..115K. doi:10.1051/0004-6361/200912521. Retrieved 2010-07-10.
{{cite journal}}
: Explicit use of et al. in:|author=
(help); Unknown parameter|month=
ignored (help)CS1 maint: multiple names: authors list (link) - ^ Ramírez, Solange V.; Sellgren, K.; Carr, John S.; Balachandran, Suchitra C.; et al. (2000, July). "Stellar Iron Abundances at the Galactic Center" (PDF). The Astrophysical Journal,. 537 (1): 205–20. arXiv:astro-ph/0002062. Bibcode:2000ApJ...537..205R. doi:10.1086/309022. Retrieved 2010-07-09.
{{cite journal}}
: Check date values in:|year=
(help); Explicit use of et al. in:|author=
(help)CS1 maint: extra punctuation (link) CS1 maint: multiple names: authors list (link) - ^ a b c d e f g h Allen, Richard Hinckley, (1963). Star Names: Their Lore and Meaning (rep. ed.). New York, NY: Dover Publications Inc. pp. 310–12. ISBN 0-486-21079-0.
{{cite book}}
: CS1 maint: extra punctuation (link) CS1 maint: multiple names: authors list (link) - ^ Stella lucida in umero dextro, quae ad rubedinem vergit. "Bright star in right shoulder, which inclines to ruddiness."
- ^ Information, Reed Business (October 22, 1981). "Ancient chinese suggest Betelgeuse is a young star". New Scientist. 92 (1276): 238.
{{cite journal}}
:|first1=
has generic name (help) - ^ Brück, H. A. (July 11–15, 1978). "P. Angelo Secchi, S. J. 1818–1878". Spectral Classification of the Future, Proceedings of the IAU Colloq. 47. Vatican City. pp. 7–20. Bibcode:1979RA......9....7B.
{{cite conference}}
: Check date values in:|year=
/|date=
mismatch (help); Unknown parameter|booktitle=
ignored (|book-title=
suggested) (help); Unknown parameter|editors=
ignored (|editor=
suggested) (help) - ^ Littleton, C. Scott (2005). Gods, goddesses, and mythology. Vol. 1. Marshall Cavendish. p. 1056. ISBN 0-7614-7559-1.
- ^ a b c Davis, Kate (AAVSO Technical Assistant, Web) (2000). "Variable Star of the Month: Alpha Orionis". American Association of Variable Star Observers (AAVSO). Retrieved 2010-07-10.
{{cite web}}
: Unknown parameter|month=
ignored (help)CS1 maint: multiple names: authors list (link) - ^ a b c Burnham, Robert (1978). Burnham's Celestial Handbook: An Observer's Guide to the Universe Beyond the Solar System, Volume 2. New York: Courier Dover Publications. p. 1290. ISBN 0-486-23568-8.
- ^ a b Kaler, James B. (2002). The Hundred Greatest Stars. New York: Copernicus Books. p. 33. ISBN 0-387-95436-8.
- ^ a b c d Michelson, Albert Abraham; Pease, Francis G. (1921). "Measurement of the diameter of alpha Orionis with the interferometer". Astrophysical Journal. 53: 249–59. Bibcode:1921ApJ....53..249M. doi:10.1086/142603.
The 0.047 arcsecond measurement was for a uniform disk. In the article Michelson notes that limb darkening would increase the angular diameter by about 17%, hence 0.055 arcseconds
{{cite journal}}
: CS1 maint: multiple names: authors list (link) - ^ a b Tenn, Joseph S. (2009). "The Bruce Medalists". Martin Schwarzschild 1965. Astronomical Society of the Pacific (ASP). Retrieved 2010-09-28.
{{cite web}}
: Unknown parameter|month=
ignored (help) - ^ Schwarzschild, Martin (1958). Structure and evolution of the stars. Princeton University Press. Bibcode:1958ses..book.....S. ISBN 0-486-61479-4.
- ^ a b c Gilliland, Ronald L.; Dupree, Andrea K. (1996). "First Image of the Surface of a Star with the Hubble Space Telescope" (PDF). Astrophysical Journal Letters,. 463 (1): L29. Bibcode:1996ApJ...463L..29G. doi:10.1086/310043. Retrieved 2010-08-01.
The yellow/red "image" or "photo" of Betelgeuse usually seen is actually not a picture of the red giant but rather a mathematically generated image based on the photograph. The photograph was actually of much lower resolution: The entire Betelgeuse image fit entirely within a 10x10 pixel area on the Hubble Space Telescopes Faint Object Camera. The actual images were oversampled by a factor of 5 with bicubic spline interpolation, then deconvolved.
{{cite journal}}
: Unknown parameter|month=
ignored (help)CS1 maint: extra punctuation (link) CS1 maint: multiple names: authors list (link) - ^ a b c d Buscher, D. F.; Baldwin, J. E.; Warner, P. J.; Haniff, C. A.; Baldwin; Warner; Haniff (1990). "Detection of a bright feature on the surface of Betelgeuse". Monthly Notices of the Royal Astronomical Society. 245: 7. Bibcode:1990MNRAS.245P...7B.
{{cite journal}}
: CS1 maint: multiple names: authors list (link) - ^ a b Wilson, R. W.; Dhillon, V. S.; Haniff, C. A.; Dhillon; Haniff (1997). "The changing face of Betelgeuse". Monthly Notices of the Royal Astronomical Society. 291: 819. Bibcode:1997MNRAS.291..819W.
{{cite journal}}
: CS1 maint: multiple names: authors list (link) - ^ Burns, D.; Baldwin, J. E.; Boysen, R. C.; Haniff, C. A.; Baldwin; Boysen; Haniff; Lawson; MacKay; Rogers; Scott; Warner; et al. (1997). "The surface structure and limb-darkening profile of Betelgeuse". Monthly Notices of the Royal Astronomical Society. 290 (1): L11–L16. Bibcode:1997MNRAS.290L..11B.
{{cite journal}}
: Explicit use of et al. in:|author=
(help); Unknown parameter|month=
ignored (help)CS1 maint: multiple names: authors list (link) - ^ Tuthill, P. G.; Haniff, C. A.; Baldwin, J. E.; Haniff; Baldwin (1997). "Hotspots on late-type supergiants". Monthly Notices of the Royal Astronomical Society. 285 (3): pp. 529–539. Bibcode:1997MNRAS.285..529T.
{{cite journal}}
:|pages=
has extra text (help); Unknown parameter|month=
ignored (help)CS1 maint: multiple names: authors list (link) - ^ a b c d e Schwarzschild, Martin (1975). "On the scale of photospheric convection in red giants and supergiants". Astrophysical Journal. 195 (1): 137–44. Bibcode:1975ApJ...195..137S. doi:10.1086/153313.
- ^ Petersen, Carolyn Collins; Brandt, John C. (1998) [1995]. Hubble Vision: Further Adventures with the Hubble Space Telescope (2nd ed.). Cambridge, England: Cambridge University Press. pp. 91–92. ISBN 0-521-59291-7.
{{cite book}}
: CS1 maint: multiple names: authors list (link) - ^ Uitenbroek, Han; Dupree, Andrea K.; Gilliland, Ronald L. (1998). "Spatially Resolved Hubble Space Telescope Spectra of the Chromosphere of α Orionis". The Astronomical Journal. 116 (5): 2501–12. Bibcode:1998AJ....116.2501U. doi:10.1086/300596. Retrieved 2007-06-20.
{{cite journal}}
: CS1 maint: multiple names: authors list (link) - ^ a b c Weiner, J.; Danchi, W. C.; Hale, D. D. S.; McMahon, J.; et al. (2000). "Precision Measurements of the Diameters of α Orionis and ο Ceti at 11 Microns" (PDF). The Astrophysical Journal. 544 (2): 1097–1100. Bibcode:2000ApJ...544.1097W. doi:10.1086/317264. Retrieved 2007-06-23.
{{cite journal}}
: Explicit use of et al. in:|author=
(help)CS1 maint: multiple names: authors list (link) Cite error: The named reference "WEINER" was defined multiple times with different content (see the help page). - ^ a b c d e Sanders, Robert (2009). "Red giant star Betelgeuse mysteriously shrinking". UC Berkeley News. UC Berkeley. Retrieved 18 April 2010.
The measurements cannot be compared anyway, because the star's size depends on the wavelength of light used to measure it, Townes said. This is because the tenuous gas in the outer regions of the star emits light as well as absorbs it, which makes it difficult to determine the edge of the star.
{{cite web}}
: Unknown parameter|month=
ignored (help) - ^ a b c d Townes, C. H.; Wishnow, E. H.; Hale, D. D. S.; Walp, B. (2009). "A Systematic Change with Time in the Size of Betelgeuse". The Astrophysical Journal Letters. 697 (2): L127–28. Bibcode:2009ApJ...697L.127T. doi:10.1088/0004-637X/697/2/L127.
{{cite journal}}
: CS1 maint: multiple names: authors list (link) - ^ "The Flames of Betelgeuse". ESO Photo Release. 23 June 2011. Retrieved 24 June 2011.
- ^ a b c d Bernat, A. P. (1977). "The circumstellar shells and mass loss rates of four M supergiants". Astrophysical Journal. 213: 756–766. Bibcode:1977ApJ...213..756B. doi:10.1086/155205.
- ^ a b c d e f g "Sharpest views of Betelgeuse reveal how supergiant stars lose mass". Press Releases. European Southern Observatory. 2009. Retrieved 2010-09-06.
ESO notes in the article that the supergiant's luminosity is at least 100,000 Suns, thus confirming a luminosity at least double that of Deneb.
{{cite web}}
: Unknown parameter|month=
ignored (help) - ^ HR 1713, database entry, The Bright Star Catalogue, 5th Revised Ed. (Preliminary Version), D. Hoffleit and W. H. Warren, Jr., CDS ID V/50. Accessed on line August 19, 2010.
- ^ a b Robert Nemiroff (MTU) & Jerry Bonnell (USRA) (2009-08-05). "Betelgeuse Resolved". Today's Astronomy Picture of the Day. Retrieved 2010-11-17.
Betelgeuse is a candidate to undergo a spectacular supernova explosion almost anytime in the next few thousand years.
- ^ R. Cutri and M. Skrutskie (2009-09-07). "Very Bright Stars in the 2MASS Point Source Catalog (PSC)". The Two Micron All Sky Survey at IPAC. Retrieved 2011-12-28.
- ^ van Altena, W. F.; Lee, J. T.; Hoffleit, D.; Lee; Hoffleit (1995). "Yale Trigonometric Parallaxes Preliminary". Yale University Observatory (1991). 1174: 0. Bibcode:1995yCat.1174....0V.
{{cite journal}}
: Unknown parameter|month=
ignored (help)CS1 maint: multiple names: authors list (link) - ^ "Hipparcos Input Catalogue, Version 2 (Turon+ 1993)". VizieR. Centre de Données astronomiques de Strasbourg. 1993. Retrieved 2010-06-20.
- ^ Perryman, M. A. C.; et al. (1997). "The Hipparcos Catalogue". Astronomy & Astrophysics. 323: L49–L52. Bibcode:1997A&A...323L..49PTemplate:Inconsistent citations
{{cite journal}}
: CS1 maint: postscript (link) - ^ Eyer, L.; Grenon, M.; Grenon (2000). "Problems Encountered in the Hipparcos Variable Stars Analysis". Delta Scuti and Related Stars, Reference Handbook and Proceedings of the 6th Vienna Workshop in Astrophysics. ASP Conference Series. 210: 482. arXiv:astro-ph/0002235. Bibcode:2000ASPC..210..482E. ISBN 1-58381-041-2.
{{cite journal}}
: CS1 maint: multiple names: authors list (link) - ^ "Gaia overview". European Space Agency. Last update: 4 May 2010. Retrieved 2010-07-14.
{{cite web}}
: Check date values in:|date=
(help) - ^ This range of absolute magnitudes assumes an apparent magnitude that varies from 0.2 to 1.2 and a distance of 197 pc.
- ^ Goldberg, Leo (1984). "The variability of alpha Orionis". Publications of the Astronomical Society of the Pacific. 96: 366–71. Bibcode:1984PASP...96..366G. doi:10.1086/131347.
- ^ a b c SolStation. "Betelgeuse; Release No.: 04-03". Sol Company. Retrieved 2010-07-20.
- ^ Freytag, B.; Steffen, M.; Dorch, B. (2002). "Spots on the surface of Betelgeuse – Results from new 3D stellar convection models". Astronomische Nachrichten. 323 (3/4): 213–19. Bibcode:2002AN....323..213F. doi:10.1002/1521-3994(200208)323:3/4<213::AID-ASNA213>3.0.CO;2-H.
{{cite journal}}
: Unknown parameter|month=
ignored (help)CS1 maint: multiple names: authors list (link) - ^ Leighton, Robert B. (1964). "Transport of Magnetic Fields on the Sun". Astrophysical Journal. 140: 1547. Bibcode:1964ApJ...140.1547L. doi:10.1086/148058.
- ^ a b Dupree, Andrea K.; Gilliland, Ronald L.; Gilliland (1995). "HST Direct Image of Betelgeuse". Bulletin of the American Astronomical Society. 27: 1328. Bibcode:1995AAS...187.3201D.
Such a major single feature is distinctly different from scattered smaller regions of activity typically found on the Sun although the strong ultraviolet flux enhancement is characteristic of stellar magnetic activity. This inhomogeneity may be caused by a large scale convection cell or result from global pulsations and shock structures that heat the chromosphere."
{{cite journal}}
: Unknown parameter|month=
ignored (help)CS1 maint: multiple names: authors list (link) - ^ Aguilar, David; Pulliam, Christine; Lobel, A. (2004). "Storms Of Hot And Cold Gas Rage In Betelgeuse's Turbulent Atmosphere". Harvard–Smithsonian Center for Astrophysics. Retrieved 2010-07-27.
{{cite web}}
: Unknown parameter|month=
ignored (help)CS1 maint: multiple names: authors list (link) - ^ Bonneau, D.; Labeyrie, A. (1973). "Speckle Interferometry: Color-Dependent Limb Darkening Evidenced on Alpha Orionis and Omicron Ceti". Astrophysical Journal. 181: L1. Bibcode:1973ApJ...181L...1B. doi:10.1086/181171.
{{cite journal}}
: CS1 maint: multiple names: authors list (link) - ^ Balega, Iu.; Blazit, A.; Bonneau, D.; Koechlin, L.; Labeyrie, A.; Foy, R..; Blazit; Bonneau; Koechlin; Labeyrie; Foy (1982). "The angular diameter of Betelgeuse". Astronomy and Astrophysics. 115 (2): 253–56. Bibcode:1982A&A...115..253B.
{{cite journal}}
: Unknown parameter|month=
ignored (help)CS1 maint: multiple names: authors list (link) - ^ a b Perrin, G.; Ridgway, S. T.; Coudé du Foresto, V.; Mennesson, B.; Traub, W. A.; Lacasse, M. G. (2004). "Interferometric observations of the supergiant stars α Orionis and α Herculis with FLUOR at IOTA". Astronomy and Astrophysics. 418 (2): 675–85. arXiv:astro-ph/0402099. Bibcode:2004A&A...418..675P. doi:10.1051/0004-6361:20040052.
Assuming a distance of 197±45pc, an angular distance of 43.33±0.04 mas would equate to a radius of 4.3 AU or 920 R☉
{{cite journal}}
: CS1 maint: multiple names: authors list (link) - ^ Young, John (November 24, 2006). "Surface imaging of Betelgeuse with COAST and the WHT". University of Cambridge. Retrieved 2007-06-21.
Images of hotspots on the surface of Betelgeuse taken at visible and infra-red wavelengths using high resolution ground-based interferometers
- ^ Perrin, Guy; Malbet, Fabien; Malbet (2003). "Observing with the VLTI". EAS Publications Series. 6: 3. Bibcode:2003EAS.....6D...3P.
{{cite journal}}
: CS1 maint: multiple names: authors list (link) - ^ Worden, S. (1978). "Speckle interferometry". New Scientist. 78: 238–40. Bibcode:1978NewSc..78..238W.
{{cite journal}}
: Unknown parameter|month=
ignored (help) - ^ Roddier, F. (1999). "Ground-Based Interferometry with Adaptive Optics". Working on the Fringe: Optical and IR Interferometry from Ground and Space. Proceedings from ASP Conference. 194: 318. Bibcode:1999ASPC..194..318R. ISBN 1-58381-020-X.
- ^ "Top Five Breakthroughs From Hubble's Workhorse Camera". NASA Jet Propulsion Laboratory, California Institute of Technology. May 4, 2009. Retrieved 2007-08-28.
- ^ Melnick, J.; Petrov R.; Malbet, F. (February 23, 2007). "The Sky Through Three Giant Eyes, AMBER Instrument on VLT Delivers a Wealth of Results". European Southern Observatory. Retrieved 2007-08-29.
{{cite web}}
: CS1 maint: multiple names: authors list (link) - ^ Wittkowski, M. (February 23, 2007). "MIDI and AMBER from the user's point of view" (PDF). European Southern Observatory VLTI. Retrieved 2007-08-29.
- ^ See Note 1 for detailed list of radii and Note 2 for calculations.
- ^ Accurate diameter measurement of Betelgeuse using the VLTI/AMBER instrument; Chelli (2009). "Hernandez Utrera, O.; Chelli, A" (PDF). Revista Mexicana de Astronomía y Astrofísica (Serie de Conferencias). 37: 179–80. Bibcode:2009RMxAC..37..179H.
{{cite journal}}
: Unknown parameter|month=
ignored (help) - ^ a b c d e f g h Haubois, X.; Perrin, G.; Lacour, S.; Verhoelst, T.; Meimon, S.; et al. (2009). "Imaging the spotty surface of Betelgeuse in the H band". Astronomy and Astrophysics. 508 (2): 923–932. Bibcode:2009A&A...508..923H. doi:10.1051/0004-6361/200912927.
{{cite journal}}
: Explicit use of et al. in:|author=
(help); Unknown parameter|month=
ignored (help)CS1 maint: multiple names: authors list (link) - ^ Cowen, Ron (2009-06-10). "Betelgeuse shrinks: The red supergiant has lost 15 percent of its size".
The shrinkage corresponds to the star contracting by a distance equal to that between Venus and the Sun, researchers reported June 9 at an American Astronomical Society meeting and in the June 1 Astrophysical Journal Letters.
- ^ Courtland, Rachel (2009). "Betelgeuse: The incredible shrinking star?". New Scientist. Reed Business Information Ltd. Retrieved 2010-09-25.
{{cite web}}
: Unknown parameter|month=
ignored (help) - ^ Robert Nemiroff (MTU) & Jerry Bonnell (USRA) (June 5, 1999). "Betelgeuse, Betelgeuse, Betelgeuse". Today's Astronomy Picture of the Day. Retrieved 2010-07-10.
- ^ a b Robert Nemiroff (MTU) & Jerry Bonnell (USRA) (January 6, 2010). "The Spotty Surface of Betelgeuse". Today's Astronomy Picture of the Day. Retrieved 2010-07-18.
Given the ongoing debate and many uncertainties regarding Betelgeuse's actual size, APOD in 2010 reaffirmed the Jovian orbit as a standard metric for the star's radius.
- ^ Bedding, T. R.; Zijlstra, A. A.; von der Luhe, O.; Robertson; Zijlstra; Von Der Luhe; Robertson; Marson; Barton; Carter; et al. (1997). "The angular diameter of R Doradus: a nearby Mira-like star". Monthly Notices of the Royal Astronomical Society. 286 (4): 957–62. arXiv:astro-ph/9701021. Bibcode:1997MNRAS.286..957B.
{{cite journal}}
: Explicit use of et al. in:|author=
(help)CS1 maint: multiple names: authors list (link) - ^ Garrison, R. F. (1993). "Anchor Points for the MK System of Spectral Classification". Bulletin of the American Astronomical Society. 25: 1319. Bibcode:1993AAS...183.1710G. Retrieved 2012-02-04Template:Inconsistent citations
{{cite journal}}
: Unknown parameter|month=
ignored (help)CS1 maint: postscript (link) - ^ Morgan, William Wilson; Keenan, Philip Childs; Kellman, Edith (1943). An atlas of stellar spectra, with an outline of spectral classification. The University of Chicago press. Bibcode:1943QB881.M6....... Retrieved 2010-10-30.
{{cite book}}
: CS1 maint: multiple names: authors list (link) - ^ a b c Posson-Brown, Jennifer; Kashyap, Vinay L.; Pease, Deron O.; Drake, Jeremy J. (2006). "Dark Supergiant: Chandra's Limits on X-rays from Betelgeuse". arXiv:astro-ph/0606387.
{{cite arXiv}}
:|class=
ignored (help); Unknown parameter|bibcode=
ignored (help)CS1 maint: multiple names: authors list (link) - ^ a b c Gray, David F. (2000). "Betelgeuse and Its Variations" (PDF). The Astrophysical Journal. 532 (1): 487–96. Bibcode:2000ApJ...532..487G. doi:10.1086/308538.
The mass of the star is unknown, but most investigators show a preference for a fairly large mass in the range of 10–20M☉
{{cite journal}}
: Unknown parameter|month=
ignored (help) - ^ "Bright Star Catalogue, 5th Revised Ed. (Hoffleit+, 1991)". VizieR. Centre de Données astronomiques de Strasbourg. Retrieved 2010-06-20.
The catalog cites a rotational speed of 329 km/s. For calculations see the Notes section of the Pleione article.
- ^ Dorch, S. B. F. (2004). "Magnetic activity in late-type giant stars: Numerical MHD simulations of non-linear dynamo action in Betelgeuse" (PDF). Astronomy and Astrophysics. 423 (3): pp. 1101–07. arXiv:astro-ph/0403321. Bibcode:2004A&A...423.1101D. doi:10.1051/0004-6361:20040435.
{{cite journal}}
:|pages=
has extra text (help); Unknown parameter|month=
ignored (help) - ^
Aurière, M; Donati, J.-F.; Konstantinova-Antova, R.; Perrin, G. ;Petit, P.; Roudier, T. (2010). "The magnetic field of Betelgeuse : a local dynamo from giant convection cells?". Astronomy & Astrophysics. 516: L2. arXiv:1005.4845. Bibcode:2010A&A...516L...2A. doi:10.1051/0004-6361/201014925.
{{cite journal}}
: Unknown parameter|month=
ignored (help)CS1 maint: multiple names: authors list (link) - ^
Maeder, André; Meynet, Georges; Meynet (2003). "The role of rotation and mass loss in the evolution of massive stars". Publications of the Astronomical Society of the Pacific. 212: 267. Bibcode:2003IAUS..212..267M.
{{cite journal}}
: CS1 maint: multiple names: authors list (link) - ^ a b c Reynolds, R. J.; Ogden, P. M. (1979). "Optical evidence for a very large, expanding shell associated with the I Orion OB association, Barnard's loop, and the high galactic latitude H-alpha filaments in Eridanus". Astrophysical Journal. 229: 942–953. Bibcode:1979ApJ...229..942R. doi:10.1086/157028.
{{cite journal}}
: CS1 maint: multiple names: authors list (link) - ^ a b
Baud, B.; Waters, R.; de Vries, J.; van Albada, G. D.; Waters; De Vries; Van Albada; Boulanger; Wesselius; Gillet; Habing; Van Der Kruit; et al. (1984). "A Giant Asymmetric Dust Shell around Betelgeuse". Bulletin of the American Astronomical Society. 16: 405. Bibcode:1984BAAS...16..405B.
{{cite journal}}
: Explicit use of et al. in:|author=
(help); Unknown parameter|month=
ignored (help)CS1 maint: multiple names: authors list (link) - ^
Ridgway, Stephen; Aufdenberg, Jason; Creech-Eakman, Michelle; Elias, Nicholas; Aufdenberg; Creech-Eakman; Elias; Howell; Hutter; Karovska; Ragland; Wishnow; et al. (2009). "Quantifying Stellar Mass Loss with High Angular Resolution Imaging". Astronomy and Astrophysics. 247: 247. Bibcode:2009astro2010S.247R.
Ridgway notes: 'Stellar mass loss is key to understanding the evolution of the universe from the earliest cosmological times to the current epoch, and of planet formation and the formation of life itself.'
{{cite journal}}
: Explicit use of et al. in:|author=
(help)CS1 maint: multiple names: authors list (link) - ^
A. P.Ohnaka, K.; Hofmann, K.-H.; Benisty, M.; Chelli, A.; et al. (2009). "Spatially resolving the inhomogeneous structure of the dynamical atmosphere of Betelgeuse with VLTI/AMBER". Astronomy and Astrophysics. 503 (1): 183–195. Bibcode:2009A&A...503..183O. doi:10.1051/0004-6361/200912247.
{{cite journal}}
: Explicit use of et al. in:|author=
(help)CS1 maint: multiple names: authors list (link) - ^
Adams, Walter S.; MacCormack, Elizabeth (1935). "Systematic Displacements of Lines in the Spectra of Certain Bright Stars". Astrophysical Journal. 81: 119. Bibcode:1935ApJ....81..119A. doi:10.1086/143620.
{{cite journal}}
: CS1 maint: multiple names: authors list (link) - ^
Deutsch, Armin J. (1956). "The Circumstellar Envelope of Alpha Herculis". Astrophysical Journal. 123: 210. Bibcode:1956ApJ...123..210D. doi:10.1086/146152.
In the concluding sentence of the abstract, Deutsch notes that: This process may be important in the evolution of all massive stars that have exhausted their hydrogen—a reference to α Ori and other red giants experiencing mass loss.
- ^ Tsuji, T. (2000). "Water on the Early M Supergiant Stars α Orionis and μ Cephei" (PDF). The Astrophysical Journal. 538 (2): 801–807. Bibcode:2000ApJ...538..801T. doi:10.1086/309185.
- ^ Lambert, D. L.; Brown, J. A.; Hinkle, K. H.; Johnson, H. R. (1984). "Carbon, nitrogen, and oxygen abundances in Betelgeuse". Astrophysical Journal. 284: 223–237. Bibcode:1984ApJ...284..223L. doi:10.1086/162401.
{{cite journal}}
: Unknown parameter|month=
ignored (help)CS1 maint: multiple names: authors list (link) - ^ a b c Dave Finley (April 8, 1998). "VLA Shows "Boiling" in Atmosphere of Betelgeuse". National Radio Astronomy Observatory. Retrieved 2010-09-07.
- ^
Lim, Jeremy; Carilli, Chris L.; White, Stephen M.; Beasley, Anthony J.; Marson, Ralph G. (1998). "Large convection cells as the source of Betelgeuse's extended atmosphere". Nature. 392 (6676): 575–577. Bibcode:1998Natur.392..575L. doi:10.1038/33352.
{{cite journal}}
: CS1 maint: multiple names: authors list (link) - ^ a b
Lobel, A.; Aufdenberg, J.; Dupree, A. K.; Kurucz, R. L.; Stefanik, R. P.; Torres, G.; Aufdenberg; Dupree; Kurucz; Stefanik; Torres (2004). "Spatially Resolved STIS Spectroscopy of Betelgeuse's Outer Atmosphere". Publications of the Astronomical Society of the Pacific. 219: 641. arXiv:astro-ph/0312076. Bibcode:2004IAUS..219..641L.
In the article, Lobel et al. equate 1 arcsecond to approximately 40 stellar radii, a calculation which in 2004 likely assumed a Hipparcos distance of 131 pc (430 ly) and a photospheric diameter of 0.0552" from Weiner et al.
{{cite journal}}
: CS1 maint: multiple names: authors list (link) - ^
Sutton, E. C.; Storey, J. W. V.; Betz, A. L.; Townes, C. H.; Spears, D. L. (1977). "Spatial heterodyne interferometry of VY Canis Majoris, Alpha Orionis, Alpha Scorpii, and R Leonis at 11 microns". Astrophysical Journal Letters. 217: L97–L100. Bibcode:1977ApJ...217L..97S. doi:10.1086/182547.
{{cite journal}}
: CS1 maint: multiple names: authors list (link) - ^ a b
Skinner, C. J.; Dougherty, S. M.; Meixner, M.; Bode, M. F.; Dougherty; Meixner; Bode; Davis; Drake; Arens; Jernigan; et al. (1997). "Circumstellar environments – V. The asymmetric chromosphere and dust shell of alpha Orionis". Monthly Notices of the Royal Astronomical Society. 288 (2): 295–306. Bibcode:1997MNRAS.288..295S.
{{cite journal}}
: Explicit use of et al. in:|author=
(help)CS1 maint: multiple names: authors list (link) - ^
Danchi, W. C.; Bester, M.; Degiacomi, C. G.; Greenhill, L. J.; Townes, C. H. (1994). "Characteristics of dust shells around 13 late-type stars". The Astronomical Journal. 107 (4): 1469–1513. Bibcode:1994AJ....107.1469D. doi:10.1086/116960.
{{cite journal}}
: CS1 maint: multiple names: authors list (link) - ^
David, L.; Dooling, D.; Dooling (1984). "The infrared universe". Space World,. 2: 4–7. Bibcode:1984SpWd....2....4D.
{{cite journal}}
: Unknown parameter|month=
ignored (help)CS1 maint: extra punctuation (link) CS1 maint: multiple names: authors list (link) - ^ Harper, Graham M.; Carpenter, Kenneth G.; Ryde, Nils; Smith, Nathan; et al. (2009). "UV, IR, and mm Studies of CO Surrounding the Red Supergiant α Orionis (M2 Iab)". AIP Conference Proceedings. 1094: 868–871. Bibcode:2009AIPC.1094..868H. doi:10.1063/1.3099254.
{{cite journal}}
: Explicit use of et al. in:|author=
(help)CS1 maint: multiple names: authors list (link) - ^ CXC/M.Weiss; Spectrum: NASA/CXC/N.Butler; et al. (Last update: September 30, 2010). "Cosmic Forensics Confirms Gamma-ray Burst/Supernova Connection". Harvard-Smithsonian Center for Astrophysics. Retrieved 2010-11-16.
{{cite web}}
: Check date values in:|date=
(help); Explicit use of et al. in:|author=
(help)CS1 maint: multiple names: authors list (link) - ^ Sessions, Larry (2009). "Betelgeuse: Will explode someday". EarthSky Communications, Inc. Retrieved 2010-10-16.
{{cite web}}
: Unknown parameter|month=
ignored (help) - ^ a b Jean Tate (Oct 13, 2009). "Betelgeuse". Universe Today. Retrieved 2010-11-16.
- ^ Wheeler, J. Craig (2007). Cosmic Catastrophes: Exploding Stars, Black Holes, and Mapping the Universe (2nd ed.). Cambridge, UK: Cambridge University Press. pp. 115–17. ISBN 0-521-85714-7.
- ^ Karovska, M.; Noyes, R. W.; Roddier, F.; Nisenson, P.; Stachnik, R. V.; Noyes; Roddier; Nisenson; Stachnik (1985). "On a Possible Close Companion to α Ori". Bulletin of the American Astronomical Society. 17: 598. Bibcode:1985BAAS...17..598K.
{{cite journal}}
: CS1 maint: multiple names: authors list (link) - ^ Karovska, M.; Nisenson, P.; Noyes, R. (1986). "On the alpha Orionis triple system". Astrophysical Journal. 308: 675–85. Bibcode:1986ApJ...308..260K. doi:10.1086/164497.
{{cite journal}}
: CS1 maint: multiple names: authors list (link) - ^ Whitney Clavin (Last update: 20 May 2010). "Two Peas in an Irregular Pod, How Binary Stars May Form". NASA Mission News. Retrieved 2010-11-15.
{{cite web}}
: Check date values in:|date=
(help) - ^ Dupree, Andrea K. (1987). "Monitoring the Variable Atmosphere of Alpha Orionis". IUE Proposal ID #LSJAD: 2758. Bibcode:1987iue..prop.2758D.
- ^ Wilson, R. W.; Baldwin, J. E.; Buscher, D. F.; Warner, P. J.; Baldwin; Buscher; Warner (1992). "High-resolution imaging of Betelgeuse and Mira". Monthly Notices of the Royal Astronomical Society. 257 (3): 369–76. Bibcode:1992MNRAS.257..369W.
{{cite journal}}
: CS1 maint: multiple names: authors list (link) - ^ Karovska, M. (1992). "Imaging of the Surface of α ORI". Proceedings of the 7th Cambridge Workshop, ASP Conference Series. 26: 279. Bibcode:1992ASPC...26..279K.
- ^ "CCDM (Catalog of Components of Double & Multiple stars (Dommanget+ 2002)". VizieR. Centre de Données astronomiques de Strasbourg. Retrieved 2010-08-22.
- ^ "ESA space operations and situational awareness". European Space Agency. Last update: 22 April 2010. Retrieved 2010-11-15.
{{cite web}}
: Check date values in:|date=
(help) - ^ Likely the result of mistaking the l for an i. Ultimately, this led to the modern Betelgeuse.
- ^ Bode, Johann Elert, (ed.). (1782) Vorstellung der Gestirne: auf XXXIV Kupfertafeln nach der Parisier Ausgabe des Flamsteadschen Himmelsatlas, Gottlieb August Lange, Berlin / Stralsund, pl. XXIV.
- ^ Bode, Johann Elert, (ed.) (1801). Uranographia: sive Astrorum Descriptio, Fridericus de Harn, Berlin, pl. XII.
- ^ a b c Schaaf, Fred (2008). "Betelgeuse". The Brightest Stars. Hoboken, New Jersey: Wiley. pp. 174–82. ISBN [[Special:BookSources/0-471-70410-2|0-471-70410-2[[Category:Articles with invalid ISBNs]]]].
{{cite book}}
: Check|isbn=
value: invalid character (help) - ^ Dibon-Smith, Richard. "Alpha Orionis (Betelgeuse )". The Constellations Web Page. Retrieved 23 January 2010.
- ^ a b Kanipe, Jeff (2005-06-30). "SpaceWatch -- A Star by Any Other Name". Archived from the original on 2009-05-22. Retrieved 2009-10-23.
- ^ a b Kunitzsch, Paul; Smart, Tim (2006). A Dictionary of Modern star Names: A Short Guide to 254 Star Names and Their Derivations (2nd rev. ed.). Cambridge, MA: Sky Pub. p. 45. ISBN 978-1-931559-44-7.
{{cite book}}
: CS1 maint: multiple names: authors list (link) - ^ Davis, George R., Jr. (1944). "The pronunciations, derivations, and meanings of a selected list of star names". Popular Astronomy. 52: 8–29. Bibcode:1944PA.....52....8D.
{{cite journal}}
: CS1 maint: multiple names: authors list (link) See p. 23. - ^ Ridpath, Ian (2006). The Monthly Sky Guide (7th ed.). Cambridge University Press. p. 8. ISBN 0-521-68435-8.
- ^ Kunitzsch, Paul (1959). Arabische Sternnamen in Europa. Wiesbaden: Otto Harrassowitz.
- ^ "Daijirin" p.2327 ISBN 4-385-13902-4
- ^ Hōei Nojiri"Shin seiza jyunrei"p.19 ISBN 978-4-12-204128-8
- ^ Henry, Teuira (1907). "Tahitian Astronomy: Birth of Heavenly Bodies". The Journal of the Polynesian Society. 16 (2): 101–04. JSTOR 20700813.
- ^ a b Conley, Craig (2008). Magic Words: A Dictionary. Weiser. p. 121. ISBN 1-57863-434-2. Retrieved 22 September 2010.
- ^ Larsen, Kristine (2005). "A Definitive Identification of Tolkien's "Borgil": An Astronomical and Literary Approach". Tolkien Studies. 2 (1): 161–70. doi:10.1353/tks.2005.0023.
- ^ Tallant, Nicolla (July 15, 2007). "Survivor recalls the night an apocalypse came to Whiddy". Independent Digital. Independent News & Media PLC. Retrieved 2011-06-10.
External links

- Alpha Orionis (Betelgeuse) AAVSO Variable Star of the Month article, December 2000.
- Surface imaging of Betelgeuse with COAST and the WHT Interferometric images taken at different wavelengths.
- Detailed image of Betelgeuse on Astronoo
- Near, Mid and Far Infrared Infrared Processing and Analysis Center (IPAC) webpage showing pictures at various wavelengths.
- APOD Pictures:
- Mars and Orion Over Monument Valley Stunning skyscape showing the relative brightness of Betelgeuse and Rigel.
- The Spotty Surface of Betelgeuse A reconstructed image showing two hotspots, possibly convection cells.
- Simulated Supergiant Star Freytag's "Star in a Box" illustrating the nature of Betelgeuse's "monster granules".
- Why Stars Twinkle Image of Betelgeuse showing the effect of atmospheric twinkling in a microscope.
- Canaries Sky The glowing nebulas surrounding Betelgeuse.
- Red supergiant movie Numerical simulation of a red supergiant star like Betelgeuse.
- Comments on Betelgeuse’s explosion in 2012