Acid dissociation constant

An acid dissociation constant (aka acidity constant, acid-ionization constant) is an equilibrium constant for the dissociation of an acid. It is denoted by Ka. For an equilibrium between a generic acid, HA, and its conjugate base, A-,
Ka is defined, subject to certain conditions, as
where and are equilibrium concentrations of the reactants.
The term acid dissociation constant is also used for pKa, which is equal to -log10 Ka. The term pKb is used in relation to bases, though pKb has faded from modern use due to the easy relationship available between the strength of an acid and the strength of its conjugate base. Though discussions of this topic typically assume water as the solvent, particularly at introductory levels, the Brønsted-Lowry acid-base theory is versatile enough that acidic behavior can now be characterized even in non-aqueous solutions.
The value of pKa indicates the strength of an acid: the larger the value the weaker the acid. In aqueous solution, simple acids are appreciably dissociated in in the pH range pKa 2. The actual extent of the dissociation can be calculated if the acid concentration and pH are known.
A knowledge of pKa values is essential for the understanding of the behaviour of acids and bases in solution. For example, many compounds used for medication are weak acids or bases, so a knowledge of the pKa and log p values is essential for an understanding of how the compound enters (or does not enter) the blood stream. Other applications include buffer solutions, acid-base homeostasis and certain kinds of enzyme kinetics, such as Michaelis-Menten kinetics, which involve a pre-equilibrium step. Also, knowledge of pKa values is a prerequisite for a quantitative understanding of the interaction between acids or bases and metal ions to form complexes in solution.
Acid dissociation constant
Acid-base extraction
Acid-base reaction
Acid-base physiology
Acid-base homeostasis
Acidity function
Buffer solution
Dissociation constant
Non-nucleophilic base
pH
Proton affinity
Self-ionization of water
Lewis acid base
Mineral acid base
Organic acid base
Weak acid base
Strong acid base
Super acid base
Definitions
According to Arrhenius's original definition, an acid is a substanstance which dissociates in solution, releasing the hydrogen ion.
The equilibrium constant for this "dissociation" reaction is known as a dissociation constant. However, since the liberated proton combines with a water molecule to give an hydronium ion, Arrhenius proposed that the "dissociation" reaction should be written as an acid-base reaction.
Brønsted and Lowry generalized this definition as follows.
- acid + base conjugate base + conjugate acid
For aqueous solutions an acid, HA, reacts with the base, water, to give the conjugate base, A-, and the conjugate acid, the hydronium ion. The Brønsted-Lowry definition is particularly useful when the solvent is a substance other than water, such as DMSO; in that case the solvent, S, acts as a base, accepting a proton and forming the conjugate acid SH+. It also puts acids and bases on the same footing as being, respectively, donors or acceptors of protons. The conjugate acid of a base, B, "dissociates" according to
Examples
- The bicarbonate ion is the conjugate base of carbonic acid.
- The bicarbonate ion is the conjugate acid of the base, the carbonate ion.
In fact the bicarbonate ion is amphoteric. These reactions are important for acid-base homeostasis in the human body (see carbonic acid).
Hydrogen ions in solution
When speaking of acids, chemists will frequently say hydrogen ion, H+, interchangeably with the word proton. This nomenclature convention comes from the Arrhenius definition of the acid and does not reflect advanced models of the acid that are used in modern times. The radius of the proton is 5 orders of magnitude less than the radius of a hydrogen atom. By the hard-soft acid-base theory it is an exceptionally powerful Lewis acid, and only in extreme chemical conditions does it exist for more than a few picoseconds.[citation needed] Solvents which have unshared electron-pairs will therefore donate them to the proton, forming a coordinate bond. Because of the reactivity of the bare proton and the ubiquity of solvent molecules, rather than floating freely through a solution the proton is carried by the solvent. In aqueous solutions, water serves this role through the formation of the hydronium ion:
The hydronium ion is further solvated by forming hydrogen bonds with other water molecules forming species such as H9O4+. However, none of these reactions affect the acidity of a solution. For example a 0.1 M solution of hydrochloric acid is 0.1 M in hydrogen ions regardless of the extent of solvation of any individual hydrogen ion.
A protonation reaction is one in which a proton is added to a base, as in
In this example the ammonia molecule has, in fact, accepted a proton from the (aquated) hydronium ion. The reverse reaction can be described as a deprotonation reaction as a proton is removed from the ammonium ion, regardless of the fact that the liberated proton goes on to react with water. The most precise way to describe it would be protonation with respect to water and a deprotonation with respect to ammonia, but this terminology is non-existent in chemical parlance.
Equilibrium Constant
An acid dissociation constant is a particular example of an equilibrium constant. For the specificic equilibrium
the equilibrium constant can be defined by[1]
where {A} is the activity of the chemical species A etc (activity is a dimensionless quantity). Activities of the products are placed in the numerator, activities of the reactants are placed in the denominator. See Chemical equilibrium for a derivation of this expression.
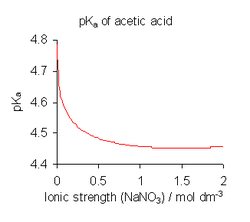
Since activity is the product of concentration and activity coefficient the definition could also be written as
where [HA] represents the concentration of HA and is a quotient of activity coefficients. According to this definition is not constant, but will vary with the concentrations, when also varies.[1]
In order to avoid this complication dissociation constants are usually determined in a medium of high ionic strength, that is, under conditions in which can be assumed to be always constant.[1] For example, the medium might be a solution of 0.1 M NaNO3 or 3M KClO4. Note, however, that all published dissociation constant values refer to the specific ionic strength and chemical composition of the dissolved salt used in their determination and that different values are obtained with different conditions.
Monoprotic acids
An acid with a single dissociative proton is called a monoprotic acid and will be denoted as HA. The dissociation reaction can be written as
For such an acid, the acid dissociation constant is the ratio of the product of the concentration of the products over the product of the concentration of the reactants (this implies that the activity quotient is constant).
In all but the most concentrated solutions it can be assumed that the concentration of water, , is constant, approximately 55 mol dm-3, and can therefore be left out. Moreover, the hydration of the proton can also be assumed to be constant. With these assumptions the expression for the dissociation constant simplifies to
This is the expression in common use.
pKa is defined as −log10 Ka. On the pKa scale of acid strength, a high value indicates a very weak acid, and a low (even negative) value indicates a not so weak one.
The pH of a solution of a monoprotic acid can be easily calculated when the pKa and analytical concentration of the acid is known. See ICE table for details.
Water
Water has both acidic and basic properies. The equilibrium constant for the equilibrium
is given by
Since the concentration of water can be assumed to be constant, this expression simplifies to
The self-ionization constant of water, Kw, can thus be seen as a special case of an acid dissociation constant.
Polyprotic acids
Polyprotic acids are acids which can loose more than one proton. The constant for dissociation of the first proton may be denoted as and the constants for dissociation of successive protons as , etc.
It is generally true that successive pK values increase (Pauling's first rule).[2] For example, for a diprotic acid, , the two equilibria are
it can be seen that the second proton is removed from a negatively charged species. Since the proton carries a positive charge extra work is needed to remove it; that the cause of the trend noted above. Phosphoric acid, , (values below), illustrates this rule, as does vanadic acid. When an exception to the rule is found it indicates that a major change in structure is ocurring. In the case of , the vanadium is octahedral, 6-coordinate, whereas all the other species are tetrahedral, 4-coordinate. This explans why for vanadium(V) oxoacids.
Some polyprotic acids can be treated as a set of individual acids. This is possible when successive pK values differ by 4 or more. For example phosphoric acid, hydrogenphosphate and dihydrogenphosphate can each be be treated as acids in their own right. On the other hand, when successive value differ by less than 4 as, for example in malonic acid, AH2 with pKs of 2.51 and 5.05, there are pH values at which A2-, AH- and AH2 co-exist.
Bases
Historically the equilibrium constant for a base was defined as the association constant for protonation of the base, B, to form the conjugate acid, HB+.
Using similar reasoning to that used before
In water, the concentration of the hydroxide ion, is related to the concentration of the hydrogen ion by , therefore
Substitution of the expression for into the expression for gives
It follows that
In aqueous solutions at 25 °C is about 14 so .
In effect there is no need to define separately from , but it is done here because values can be found in the older literature.
Temperature dependence
All equilibrium constants vary with temperature according the van 't Hoff equation
Thus, for exothermic reactions, (ΔH is negative) K decreases with temperature, but for endothermic reactions (ΔH is positive) K increases with temperature.
Speciation
The pH of a solution of weak acid can be expressed in terms of the extent of dissociation. After rearranging the expression defining the dissociation constant, and putting pH = -log10[H+], one obtains

This is a form of the Henderson-Hasselbalch equation. It can be deduced from this expression that
- when the acid is 1% dissociated, that is, when ,
- when the acid is 50% dissociated, that is, when ,
- when the acid is 99% dissociated, that is, when ,
It follows that the range of pH within which there is partial dissociation of the acid is about pKa 2.This is shown graphically at the right.
A practical application of these results is that the pH transition range of a pH indicator is approximately ; the colour of the indicator in its acid form is different from the colour of the conjugate base form. In the transition range both forms are in equilibrium, so the colour is intermediate. Outside the transition range the concentration of acid or conjugate base is less than 10% and the colour of the major species dominates.
A weak acid may be defined as an acid with pKa greater than about -2. An acid with pKa = -2 would be 99% dissociated at pH 0, that is, in a 1M HCl solution. Any acid with a pKa less than about -2 is said to be a strong acid. Strong acids are said to be fully dissociated. There is no precise pKa value that distinguishes between strong and weak acids because strong acids, such as sulfuric acid, are associated in very concentrated solution.
Factors that determine the relative strengths of acids
Pauling's second rule[2] states that the value of the first pK for acids of the formula XOm(OH) n is approximately independent of n and X and is approximately 8 for m=0, 2 for m=1, -3 for m=2 and <-10 for m=3. This correlates with the oxidation state of the central atom, X: the higher the oxidation state the stronger the oxyacid. For example, pKa for HClO is 7.2, for HClO2 is 2.0, for HClO3 is -1 and HClO4 is a strong acid.


With organic acids inductive effects and mesomeric effects affect the pKs. The effects are summarised in the Hammett equation and subsequent extensions.
Structural effects can also be important. The difference between fumaric acid and maleic acid is a classic example. Fumaric acid is (E)-1,4-but-2-enedioic acid, a trans isomer, whereas maleic acid is the corresponding cis isomer, i.e. (Z)-1,4-but-2-enedioic acid (see cis-trans isomerism). Fumaric acid has pKas of approximately 3.5 and 4.5. By contrast, maleic acid has pKas of approximately 1.5 and 6.5. The reason for this large difference is that when one proton is removed from the cis- isomer (maleic acid) a strong intramolecular hydrogen bond is formed with the nearby remaining carboxyl group. This favors the formation of the maleate H+, and it opposes the removal of the second proton from that species. In the trans isomer, the two carboxyl groups are always far apart, so hydrogen bonding is not observed.
Thermodynamics
An equilibrium constant is related to the standard Gibbs free energy change for the reaction, so for an acid dissociation constant
Free energy is made up of an enthalpy term and an entropy term.
The standard enthalpy change can be determined by calorimetry or by using the van't Hoff equation, though the calorimetric method is preferable. When both the standard enthalpy change and acid dissociation constant have been determined, the standard entropy change is easily calculated from the equation above.
The example of acetic acid is instructive. The standard enthalpy change is 0.41 kJ mol-1 (IUPAC critically evaluated value, extrapolated to zero ionic strength) [3] but the standard free energy change is -27.15 kJ mol-1. This shows that the dissociation process is entropy driven as the reaction is slightly endothermic, though common experience would dictate that an endothermic reaction should not proceed without an external energy source.
The standard enthalpy change is small because the (heat) energy required to break the O-H bond on the carboxyl group is almost equal to the energy given out when the O-H bond in the hydronium ion is formed. On the other hand the standard entropy is large and positive ( is large and negative) because the creation of charged species results in an increase in the order of the solution as a consequence of electrostatic interactions with polar water molecules.
The conclusion for this example is, perhaps, surprising: the energy of dissociation is a minor factor in determining the magnitude of the acid dissociation constant.
Note. The standard free energy change for the reaction is for the changes in concentrations of the species from the standard states to the concentrations at equilibrium. The free energy change at equilibrium is zero since the chemical potentials of reactants and products are equal at equilibrium.
Mixed solvents
When a compund has limited solubility in water it is common practice (in the pharmaceutical industry, for example) to determine pKas in a solvent mixture such as water/dioxane or water/methanol, in which the compound is more soluble. However, a pKa value obtained in a mixed solvent cannot be used directly for aqueous solutions. The reason for this is that when the solvent is in its standard state its activity is defined as one. For example, the standard state of water:dioxane 9:1 is precisely that solvent mixture, with no added solutes. To obtain the pKa value for use with aqueous solutions it has to be extrapolated to zero co-solvent concentration from values obtained from various co-solvent mixtures.
These facts are obscured by the omission of the solvent from the expression which is normally used to define pKa, but pKa values obtained in a given mixed solvent can be compared to each other, giving relative acid strengths. The same is true of pKa values obtained in a particular non-aqueous solvent such a DMSO.
A universal, solvent-independent, scale for acid dissociation constants has not yet been developed, since there is no known way to compare the standard states of two different solvents.
Importance of pKa values
The pKa value(s) of a compound influence many characteristics of the compound such as its reactivity, and spectral properties (colour). In biochemistry the pKa values of proteins and amino acid side chains are of major importance for the activity of enzymes and the stability of proteins. This property is of general importance in chemistry because ionization of a compound alters its physical behavior and macro properties such as solubility and lipophilicity. For example ionization of any compound will increase the solubility in water, but decrease the lipophilicity. This can be exploited in drug development to increase the concentration of a compound in the blood by adjusting the pKa of an ionizable group. This must be done with caution, however, since an ionized compound will pass less easily through cell membranes.
Acidity in nonaqueous solutions
Solvents will be more likely to promote ionisation of a dissolved acidic molecule if:[4]
- It is a Protic solvent, capable of forming hydrogen bonds
- It has a high donor number, making it a strong Lewis base.
- it has a high dielectric constant (Relative permittivity).
Solvents can be polar, protic, donor or none of the above. None of the above, indicated by a blank entry, means neutral or noninteracting behavior. Data taken at or near 25°C.[5]
Compound | Solvent Class | Dielectric constant |
---|---|---|
1,4-dioxane | Donor | 2.2 |
Hexane | 1.9 | |
Carbon tetrachloride | 2.2 | |
Benzene | 2.3 | |
Diethyl ether | Donor | 4.3 |
Acetic acid | Protic donor | 6.1 |
Tetrahydrofuran | Donor | 7.6 |
Acetone | Polar donor | 21 |
Dimethylsulfoxide | Polar donor | 47 |
Water | Polar protic donor | 78 |
Formamide | Polar protic donor | 111 |
An acidic solvent will also suppress dissociation of an acid. For example, hydrogen chloride is a weak acid when dissolved in the acidic solvent acetic acid, characterized by having a greater pKa than when in water. This is because, as per Le Chatelier's Principle, the release of protons by hydrogen chloride would increase the concentration in the solution. This would increase the reverse reaction rate, resulting in the reprotonation of some of the chloride ions. The extent of the reprotonation in the case of the disrupted equilibrium is determined by the acid dissociation constant. See ICE table for a quantitative explanation of how to calculate these changes in equilibrium position.
Acidity scales have been developed for solvents aside from water, notably for dimethyl sulfoxide (abbrev. 'DMSO') and acetonitrile.[6] Because water is a protic solvent it is more basic than DMSO, and its dielectric constant is greater, this means that acids will deprotonate to a lesser extent than in water (see the above paragraph). For this reason, DMSO is widely used as an alternative to water in evaluating acids and bases.
There are some drawbacks to using alternative solvents, due to a process called homoconjugation. In solvents of low dielectric constant, ions tend to associate which complicates the interpretation of pKas. In particular, aprotic solvents will encourage acids (typically polar molecules) to hydrogen bond with their conjugate base. Because the formation of the bond is exothermic and therefore stabilizing, this has the effect of increasing the amount of deprotonation. When water is used, however, because it is both protic and polar it is capable of both accepting and donating hydrogen bonds. This allows it to prevent 'clumping' of ions.
In acetonitrile solution, para-toluenesulfonic acid has a homoconjugation constant pKf, of -2.9.[7] This indicates that the toluenesulfonate anion has a strong tendency to form a hydrogen bond with the parent acid. Homoconjugation has the effect of enhancing the acidity of acids, lowering their effective pKas, by stabilizing the conjugate base. Due to homoconjugation, the proton-donating power of toluenesulfonic acid in acetonitrile solution is enhanced by a factor of nearly 800.
pKa of some common substances
There are multiple techniques to determine the pKa of a chemical causing some discrepancy between different sources. Well measured values are typically are within 0.1 units of each other. Data presented here was taken at 25 °C in water.
Chemical Name | pKa1 | pKa2 | pKa3 |
---|---|---|---|
Acetic acid [8] | 4.756 | ||
Adenine[8] | 4.17 | ||
Aniline [9] | 27 | ||
Arsenic acid [9] | 2.22 | 6.98 | 11.53 |
Benzoic acid[8] | 4.204 | ||
Boric acid [9] | 9.21 | ||
Butanoic acid [9] | 4.82 | ||
Chromic acid [9] | 0.98 | 6.5 | |
Codeine [9] | 8.17 | ||
Cresol [9] | 10.29 | ||
Diethylene glycol [9] | 14.18 | ||
Formic acid[8] | 3.751 | ||
Glycerol [9] | 13.99 | ||
Hydrofluoric acid [9] | 3.17 | ||
Hydrocyanic acid [9] | 9.21 | ||
Hydrogen selenide [9] | 3.89 | ||
Hydrogen peroxide (90%) [9] | 11.7 | ||
Lactic acid [9] | 3.86 | ||
Maleic acid [9] | 1.92 | 6.23 | |
Propanoic acid [9] | 4.87 | ||
Phenol[8] | 9.99 | ||
Phosphoric acid[10] | 2.12 | 7.21 | 12.38 |
L-(+)-Ascorbic Acid[8] | 4.17 | 11.57 |
Note: Values for amines and associated compounds are for the protonated ammonium form.
See also
- Determination of equilibrium constants
- Dissociation constant
- Henderson-Hasselbalch equation
- Hammett equation
- Hydrolysis of metal salts
- QSAR
References
- ^ a b c F.J,C. Rossotti and H. Rossotti, The Determination of Stability Constants, McGraw-Hill, 1961. Cite error: The named reference "rr" was defined multiple times with different content (see the help page).
- ^ a b Greenwood, Norman N.; Earnshaw, Alan (1997). Chemistry of the Elements (2nd ed.). Butterworth-Heinemann. ISBN 978-0-08-037941-8. p. 50
- ^ Thermodynamic Quantities for the Ionization Reactions of Buffers, R. Goldberg, N. Kishore, R. Lennen; J. Phys. Chem. Ref. Data, 31, 231-370 (2002). This review is available online at NIST
- ^ Template:Loudon p. 317
- ^ Template:Loudon p. 318
- ^ March, J. (2007). Advanced Organic Chemistry (6th edition ed.). New York: J. Wiley and Sons. ISBN 978-0-471-72091-1.
{{cite book}}
:|edition=
has extra text (help); Unknown parameter|coauthors=
ignored (|author=
suggested) (help) - ^ Coetzee, J. F. and Padmanabhan, G. R., "Proton Acceptor Power and Homoconjugation of Mono- and Diamines", J. Amer. Chem. Soc.,1965, 87, 5005-5010.
- ^ a b c d e f Speight, James G. Lange's Handbook of Chemistry (16th Edition). McGraw-Hill.
- ^ a b c d e f g h i j k l m n o p Knovel Critical Tables (2nd Edition). (2003). Knovel.
- ^ Brown, T.E. (2006). Chemistry The Central Science (10th Edition ed.). Pearson Publications. pp. A27. ISBN 0131096869.
{{cite book}}
:|edition=
has extra text (help); Unknown parameter|coauthors=
ignored (|author=
suggested) (help)
Further reading
- Atkins, Peter, and Loretta Jones. Chemical Principles: The Quest for Insight. 3rd ed. New York: W. H. Freeman and Company, 2005
- Housecroft, Catherine and Sharpe, Alan, Inorganic Chemistry, Prentice Hall, 2nd. edition, 2004. (Non-aqueous solvents)
- Hulanicki, A. (1987). Reactions of acids and bases in analytical chemistry. Horwood. ISBN 0853123306. (translation editor: Mary R. Masson)
- Perrin, D. D. (1981). pKa prediction for organic acids and bases. Chapman and Hall. ISBN 041222190x.
{{cite book}}
: Check|isbn=
value: invalid character (help); Unknown parameter|coauthors=
ignored (|author=
suggested) (help) - Albert, A. (1971). The determination of ionization constants : a laboratory manual. Chapman and Hall. ISBN 0412103001.
{{cite book}}
: Unknown parameter|coauthors=
ignored (|author=
suggested) (help) (Previous edition published as Ionization constants of acids and bases. London: Methuen, 1962)
External links
- Bordwell pKa Table in DMSO
- Shodor.org Acid-Base Chemistry
- Factors that Affect the Relative Strengths of Acids and Bases
- Purdue Chemistry
- Distribution diagrams of acids and bases (generation from p values with free spreadsheet)
- SPARC Physical/Chemical property calculator
- List of Aqueous-Equilibrium Constants
- Free guide to pKa & logP interpretation and measurement