Structural engineering

Structural engineering is a field of engineering dealing with the analysis and design of structures that support or resist loads. Structural engineering is usually considered a specialty within civil engineering, but it can also be studied in its own right.[1] Structural engineers are most commonly involved in the design of buildings and large nonbuilding structures[2] but they can also be involved in the design of machinery, medical equipment, vehicles or any item where structural integrity affects the item's function or safety. Structural engineers must ensure their designs satisfy given design criteria, predicated on safety (e.g. structures must not collapse without due warning) or serviceability and performance (e.g. building sway must not cause discomfort to the occupants).
Structural engineering theory is based upon physical laws and empirical knowledge of the structural performance of different landscapes and materials. Structural engineering design utilises a relatively small number of basic structural elements to build up structural systems that can be very complex. Structural engineers are responsible for making creative and efficient use of funds, structural elements and materials to achieve these goals.[2]
Structural engineer
Structural engineers are responsible for engineering design and analysis. Entry-level structural engineers may design the individual structural elements of a structure, for example the beams, columns, and floors of a building. More experienced engineers would be responsible for the structural design and integrity of an entire system, such as a building.
Structural engineers often specialize in particular fields, such as bridge engineering, building engineering, pipeline engineering, industrial structures, or special mechanical structures such as vehicles or aircraft.
Structural engineering has existed since humans first started to construct their own structures. It became a more defined and formalised profession with the emergence of the architecture profession as distinct from the engineering profession during the industrial revolution in the late 19th Century. Until then, the architect and the structural engineer were often one and the same - the master builder. Only with the understanding of structural theories that emerged during the 19th and 20th century did the professional structural engineer come into existence.
The role of a structural engineer today involves a significant understanding of both static and dynamic loading, and the structures that are available to resist them. The complexity of modern structures often requires a great deal of creativity from the engineer in order to ensure the structures support and resist the loads they are subjected to. A structural engineer will typically have a four or five year undergraduate degree, followed by a minimum of three years of professional practice before being considered fully qualified.[3]
Structural engineers are licensed or accredited by different learned societies and regulatory bodies around the world (for example, the Institution of Structural Engineers in the UK)[3]. Depending on the degree course they have studied and/or the jurisdiction they are seeking licensure in, they may be accredited (or licensed) as just structural engineers, or as civil engineers, or as both civil and structural engineers.
History of structural engineering
Natural stone is the material used by our earliest ancestors for building. It is good at resisting compressive forces (being squeezed) but weak in tension (being pulled apart). A Pyramidal shape is almost completely free of tensile forces and was utilised by the ancient Egyptians. The simplest and earliest structural elements are the beam and column. A beam transfers loads placed upon it to the columns across which it spans, and in doing so bends downwards under the strain. The material in the bottom part of a beam is under tension whilst that at the top is compressed. The columns in the structures of ancient Greece were spaced closely together to prevent the beams that spanned across them from failing along their undersides.
The Romans were able to span greater distances by using stone arches rather than beams. The stones in a half-circular arch are in compression. But in the eleventh century the Goths realised that the half-circular arch was inherently unstable in that it tended to be forced outwards at its ten-to and ten-past the hour points. Their solution was to establish the pointed (or Gothic) arch: essentially two half-arches brought together. The stone within such arches is entirely under compression, and the Gothic arch is the basis of the massive cathedrals that sprang up throughout Europe at the time.
Steel appeared in Victorian times and is immensely strong in resisting tensile forces - and compressive ones too. Steel gave rise to cables and steel girder structures. Cables are the mainstay of suspension bridges in which loads placed along the bridge (a beam), and the weight of the bridge itself, are supported by vertical cables spaced at regular intervals. These cables are in turn supported by a single cable, or cable cluster, suspended between high towers of stone and anchored into the ground beyond. Traffic weight is thus transferred to cable, main-cable, towers and ground anchors. Steel girder bridges are essentially just massive beams, but beams that consist mostly of fresh air. Box section bridges are also massive beams but in the form of simple rectangular tubes.
Regarding compression, a given cross-sectional area of steel can support more compressive load than the same area of stone. Steel columns are thus considerably thinner than their stone counterparts. Thin compression elements are however prone to buckling but can be prevented from doing so by constraining lateral movement at suitably spaced points along their length. Compression-resisting elements that need to be long but are unable to be thus constrained, such as the jibs of cranes, are made as girder structures in the shape of pointed zeppelins: wide and therefore unbendable at their middles.
Concrete, or rather reinforced concrete, came onto the scene at the start of the last century. Concrete starts off in liquid form and is poured into moulds, setting within hours into hard stone-like material. Before poring the concrete, steel rods can be placed inside the moulds in the parts where tensile forces are expected, and will lie hidden just beneath the outside surfaces of the concrete when it sets. Concrete beams, for example, have steel rods embedded along their undersides. To prevent a beam sagging under load its steel rods can be pre-tensioned, after the concrete sets, making the beam curve upwards until placed under load when it flattens out. Being able to be poured into moulds enables concrete structures to take all manner of irregular shapes: the curved ceilings of airports, water towers, whose sides are ringed with embedded steel to prevent them bowing out, and curved motorway carriageways.
Structural failure
The history of structural engineering contains many collapses and failures. Sometimes this is due to obvious negligence, as in the case of the Pétionville school collapse, in which Rev. Fortin Augustin said that "he constructed the building all by himself, saying he didn't need an engineer as he had good knowledge of construction" following a partial collapse of the three-story schoolhouse that sent neighbors fleeing.[4] The final collapse killed at least 94 people, mostly children.
In other cases structural failures require careful study, and the results of these inquiries have been improved practices and a greater understanding of the science of structural engineering.
Specializations
Building structures


Structural building engineering includes all structural engineering related to the design of buildings. It is the branch of structural engineering that is close to architecture.
Structural building engineering is primarily driven by the creative manipulation of materials and forms and the underlying mathematical and scientific ideas to achieve an end which fulfills its functional requirements and is structurally safe when subjected to all the loads it could reasonably be expected to experience. This is subtly different from architectural design, which is driven by the creative manipulation of materials and forms, mass, space, volume, texture and light to achieve an end which is aesthetic, functional and often artistic.
The architect is usually the lead designer on buildings, with a structural engineer employed as a sub-consultant. The degree to which each discipline actually leads the design depends heavily on the type of structure. Many structures are structurally simple and led by architecture, such as multi-storey office buildings and housing, while other structures, such as tensile structures, shells and gridshells are heavily dependent on their form for their strength, and the engineer may have a more significant influence on the form, and hence much of the aesthetic, than the architect.
The structural design for a building must ensure that the building is able to stand up safely, able to function without excessive deflections or movements which may cause fatigue of structural elements, cracking or failure of fixtures, fittings or partitions, or discomfort for occupants. It must account for movements and forces due to temperature, creep, cracking and imposed loads. It must also ensure that the design is practically buildable within acceptable manufacturing tolerances of the materials. It must allow the architecture to work, and the building services to fit within the building and function (air conditioning, ventilation, smoke extract, electrics, lighting etc). The structural design of a modern building can be extremely complex, and often requires a large team to complete.
Structural engineering specialties for buildings include:
- Earthquake engineering
- Façade engineering
- Fire engineering
- Roof engineering
- Tower engineering
- Wind engineering
Earthquake engineering structures
Earthquake engineering structures are those engineered to withstand various types of hazardous earthquake exposures at the sites of their particular location.

Earthquake engineering is treating its subject structures like defensive fortifications in military engineering but for the warfare on earthquakes. Both earthquake and military general design principles are similar: be ready to slow down or mitigate the advance of a possible attacker.
The main objectives of earthquake engineering are:
- Understand interaction of structures with the shaky ground.
- Foresee the consequences of possible earthquakes.
- Design and construct the structures to [[seismic performance|perform while being exposed to an earthquake.
Earthquake engineering or earthquake-proof structure does not, necessarily, means extremely strong and expensive one like El Castillo pyramid at Chichen Itza shown above.
Now, the most powerful and budgetary tool of the earthquake engineering is base isolation which pertains to the passive structural vibration control technologies.
Civil engineering structures
Civil structural engineering includes all structural engineering related to the built environment. It includes:
Column-generating template families
The templates listed here are not interchangeable. For example, using {{col-float}} with {{col-end}} instead of {{col-float-end}} would leave a <div>...</div>
open, potentially harming any subsequent formatting.
Type | Family | Handles wiki
table code?† |
Responsive/ mobile suited |
Start template | Column divider | End template |
---|---|---|---|---|---|---|
Float | "col-float" | Yes | Yes | {{col-float}} | {{col-float-break}} | {{col-float-end}} |
"columns-start" | Yes | Yes | {{columns-start}} | {{column}} | {{columns-end}} | |
Columns | "div col" | Yes | Yes | {{div col}} | – | {{div col end}} |
"columns-list" | No | Yes | {{columns-list}} (wraps div col) | – | – | |
Flexbox | "flex columns" | No | Yes | {{flex columns}} | – | – |
Table | "col" | Yes | No | {{col-begin}}, {{col-begin-fixed}} or {{col-begin-small}} |
{{col-break}} or {{col-2}} .. {{col-5}} |
{{col-end}} |
† Can template handle the basic wiki markup {| | || |- |}
used to create tables? If not, special templates that produce these elements (such as {{(!}}, {{!}}, {{!!}}, {{!-}}, {{!)}})—or HTML tags (<table>...</table>
, <tr>...</tr>
, etc.)—need to be used instead.
The structural engineer is the lead designer on these structures, and often the sole designer. In the design of structures such as these, structural safety is of paramount importance (in the UK, designs for dams, nuclear power stations and bridges must be signed off by a chartered engineer).
Civil engineering structures are often subjected to very extreme forces, such as large variations in temperature, dynamic loads such as waves or traffic, or high pressures from water or compressed gases. They are also often constructed in corrosive environments, such as at sea, in industrial facilities or below ground.
Mechanical structures
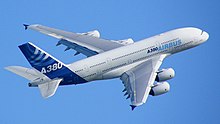
Principals of structural engineering are applied to variety of mechanical (moveable) structures. The design of static structures assumes they always have the same geometry (in fact, so-called static structures can move significantly, and structural engineering design must take this into account where necessary), but the design of moveable or moving structures must account for fatigue, variation in the method in which load is resisted and significant deflections of structures.
The The forces which parts of a machine are subjected to can vary significantly, and can do so at a great rate. The forces which a boat or aircraft are subjected to vary enormously and will do so thousands of times over the structure's lifetime. The structural design must ensure that such structures are able to endure such loading for their entire design life without failing.
These works can require mechanical structural engineering:
- Airframes and fuselages
- Boilers and pressure vessels
- Coachworks and carriages
- Cranes
- Elevators
- Escalators
- Marine vessels and hulls
Structural elements

Any structure is essentially made up of only a small number of different types of elements:
Many of these elements can be classified according to form (straight, plane / curve) and dimensionality (one-dimensional / two-dimensional):
One-dimensional | Two-dimensional | |||
---|---|---|---|---|
straight | curve | plane | curve | |
(predominantly) bending | beam | continuous arch | plate, concrete slab | lamina, dome |
(predominant) tensile stress | rope | Catenary | shell | |
(predominant) compression | pier, column | Load-bearing wall |
Columns
Columns are elements that carry only axial force - either tension or compression - or both axial force and bending (which is technically called a beam-column but practically, just a column). The design of a column must check the axial capacity of the element, and the buckling capacity.
The buckling capacity is the capacity of the element to withstand the propensity to buckle. Its capacity depends upon its geometry, material, and the effective length of the column, which depends upon the restraint conditions at the top and bottom of the column. The effective length is where is the real length of the column.
The capacity of a column to carry axial load depends on the degree of bending it is subjected to, and vice versa. This is represented on an interaction chart and is a complex non-linear relationship.
Beams
A beam may be defined as an element in which one dimemsion is much greater than the other two and the applied loads are usually normal to the main axis of the element. Beams and columns are called line elements and are often represented by simple lines in structural modeling.
- cantilevered (supported at one end only with a fixed connection)
- simply supported (supported vertically at each end; horizontally on only one to withstand friction, and able to rotate at the supports)
- continuous (supported by three or more supports)
- a combination of the above (ex. supported at one end and in the middle)
Beams are elements which carry pure bending only. Bending causes one section of a beam (divided along its length) to go into compression and the other section into tension. The compression section must be designed to resist buckling and crushing, while the tension section must be able to adequately resist the tension.
Struts and ties



1. Keystone 2. Voussoir 3. Extrados 4. Impost 5. Intrados 6. Rise 7. Clear span 8. Abutment
A truss is a structure comprising two types of structural elements; compression members and tension members (i.e. struts and ties). Most trusses use gusset plates to connect intersecting elements. Gusset plates are relatively flexible and minimize bending moments at the connections, thus allowing the truss members to carry primarily tension or compression.
Trusses are usually utilised in span large distances, where it would be uneconomical to use solid beams.
Plates
Plates carry bending in two directions. A concrete flat slab is an example of a plate. Plates are understood by using continuum mechanics, but due to the complexity involved they are most often designed using a codified empirical approach, or computer analysis.
They can also be designed with yield line theory, where an assumed collapse mechanism is analysed to give an upper bound on the collapse load (see Plasticity). This is rarely used in practice.
Shells
Shells derive their strength from their form, and carry forces in compression in two directions. A dome is an example of a shell. They can be designed by making a hanging-chain model, which will act as a catenary in pure tension, and inverting the form to achieve pure compression.
Arches
Arches carry forces in compression in one direction only, which is why it is appropriate to build arches out of masonry. They are designed by ensuring that the line of thrust of the force remains within the depth of the arch.
Catenaries
Catenaries derive their strength from their form, and carry transverse forces in pure tension by deflecting (just as a tightrope will sag when someone walks on it). They are almost always cable or fabric structures. A fabric structure acts as a catenary in two directions.
Structural engineering theory
Structural engineering depends upon a detailed knowledge of loads, physics and materials to understand and predict how structures support and resist self-weight and imposed loads. To apply the knowledge successfully a structural engineer will need a detailed knowledge of mathematics and of relevant empirical and theoretical design codes. He will also need to know about the corrosion resistance of the materials and structures, especially when those structures are exposed to the external environment.
Materials

Structural engineering depends on the knowledge of materials and their properties, in order to understand how different materials support and resist loads.
Common structural materials are:
- Iron:
- Aluminium
- Composites
- Alloy
- Masonry
- Timber
- Other structural materials:
See also
|
References
![]() | This article includes a list of general references, but it lacks sufficient corresponding inline citations. (February 2009) |
- ^ "History of Structural Engineering". University of San Diego. Retrieved 2007-12-02.
- ^ a b "What is a structural engineer". Institution of Structural Engineers. Retrieved 2007-12-02.
- ^ a b "Routes to membership". Institution of Structural Engineers. Retrieved 2007-12-25.
- ^ "Haiti school owner in custody". macaunews.net. 2008-11-11.
- Blank, Alan; McEvoy, Michael; Plank, Roger (1993). Architecture and Construction in Steel. Taylor & Francis. ISBN 0419176608.
- Bradley, Robert E.; Sandifer, Charles Edward (2007). Leonhard Euler: Life, Work and Legacy. Elsevier. ISBN 0444527281.
- Chapman, Allan. (2005). England's Leornardo: Robert Hooke and the Seventeenth Century's Scientific Revolution. CRC Press. ISBN 0750309873.
- Dugas, René (1988). A History of Mechanics. Courier Dover Publications. ISBN 0486656322.
- Feld, Jacob; Carper, Kenneth L. (1997). Construction Failure. John Wiley & Sons. ISBN 0471574775.
- Galilei, Galileo. (translators: Crew, Henry; de Salvio, Alfonso) (1954). Dialogues Concerning Two New Sciences. Courier Dover Publications. ISBN 0486600998
- Hewson, Nigel R. (2003). Prestressed Concrete Bridges: Design and Construction. Thomas Telford. ISBN 0727727745.
- Heyman, Jacques (1998). Structural Analysis: A Historical Approach. Cambridge University Press. ISBN 0521622492.
- Heyman, Jacques (1999). The Science of Structural Engineering. Imperial College Press. ISBN 1860941893.
- Hosford, William F. (2005). Mechanical Behavior of Materials. Cambridge University Press. ISBN 0521846706.
- Hoogenboom, P.C.J. . Historical Overview of Concrete Modelling.
- Kirby, Richard Shelton (1990). Engineering in History. Courier Dover Publications. ISBN 0486264122.
- Labrum, E.A. (1994). Civil Engineering Heritage. Thomas Telford. ISBN 072771970X.
- Lewis, Peter R. (2004). Beautiful Bridge of the Silvery Tay. Tempus.
- Mir, Ali (2001). Art of the Skyscraper: the Genius of Fazlur Khan. Rizzoli International Publications. ISBN 0847823709.
- Nedwell, P.J.; Swamy, R.N.(ed) (1994). Ferrocement:Proceedings of the Fifth International Symposium. Taylor & Francis. ISBN 0419197001.
- Rozhanskaya, Mariam; Levinova, I. S. (1996). "Statics" in Morelon, Régis & Rashed, Roshdi (1996). Encyclopedia of the History of Arabic Science, vol. 2-3, Routledge. ISBN 0415020638
- Whitbeck, Caroline (1998). Ethics in Engineering Practice and Research. Cambridge University Press. ISBN 0521479444.
External links
- The StructuralEngineer.info - A Center for Information Dissemination on Structural Engineering
- IABSE (International Association for Bridge and Structural Engineering)
- Institution of Structural Engineers (IStructE)
- Structural Engineering Association - International
- National Council of Structural Engineers Associations
- Structural Engineering Institute, an institute of the American Society of Civil Engineers
- StructuralWiki.org - wiki for structural engineering