Solar energy


Part of a series on |
Renewable energy |
---|
![]() |
Solar energy is energy from the sun. This energy drives climate and weather and supports virtually all life on Earth. Heat and light from the sun, along with solar-based resources such as wind and wave power, hydroelectricity and biomass, account for over 99.9 percent of the available flow of renewable energy.[2][3]
Solar energy technologies harness the sun's energy for practical ends. These technologies date from the time of the early Greeks, Native Americans and Chinese, who warmed their buildings simply by orienting them toward the sun. Modern solar technologies continue to harness the sun, but in more innovative ways, to provide heating, lighting, electricity and even flight.[4][5]
The terms solar power and solar energy are often used synonymously but solar power usually refers to photovoltaic (PV) and concentrating solar thermal technologies that convert sunlight into electricity. In the case of solar PV, the process is direct, via silicon-based cells; in the case of concentrating solar thermal, the process involves heating a transfer fluid to produce steam to run a generator. While PV has advantages in terms of simplicity, the high temperatures produced by solar thermal systems also can provide process heat and steam for a variety of secondary commercial applications (cogeneration).
The utilization of solar energy ranges from traditional and basic technologies (related to food, heat and light) to modern, utility-scale power generation systems. Solar energy is used in a wide variety of applications, including:
- Biomass (wood, biofuel)
- Electricity generation (photovoltaics, heat engines)
- Evaporation (clothes drying, desalination)
- Heat (hot water, building heat, cooking)
- Lighting (daylighting, hybrid lighting, daylight savings time)
- Transportation (solar car, solar plane, solar boat)
Energy from the Sun

Earth receives 174 petawatts of incoming solar radiation (insolation) at the upper atmosphere at any given time. When it meets the atmosphere, 6 percent of the insolation is reflected and 16 percent is absorbed. Average atmospheric conditions (clouds, dust, pollutants) further reduce insolation traveling through the atmosphere by 20 percent due to reflection and 3 percent via absorption. These atmospheric conditions not only reduce the quantity of energy reaching the Earth's surface, but also diffuse approximately 20 percent of the incoming light and filter portions of its spectrum.[6] After passing through the Earth's atmosphere, approximately half the insolation is in the visible electromagnetic spectrum with the other half mostly in the infrared spectrum (a small part is ultraviolet radiation).[7]
The absorption of solar energy by atmospheric convection (sensible heat transport) and evaporation and condensation of water vapor (latent heat transport) drives the winds and the water cycle.[8] Upon reaching the surface, sunlight is absorbed by the oceans, land masses and plants. The energy captured in the oceans drives the thermohaline cycle. As such, solar energy is ultimately responsible for temperature-driven ocean currents such as the thermohaline cycle and wind-driven currents such as the Gulf Stream. The energy absorbed by the earth, in conjunction with that recycled by the Greenhouse effect, warms the surface to an average temperature of approximately 14 °C.[9] The small portion of solar energy captured by plants and other phototrophs is converted to chemical energy via photosynthesis. All the food we eat, wood we build with, and fossil fuels we use are products of photosynthesis.[10] The flows and stores of solar energy in the environment are vast in comparison to human energy needs.
- The total solar energy available to the earth is approximately 3850 zettajoules (ZJ) per year.[3]
- Oceans absorb approximately 285 ZJ of solar energy per year.
- Winds can theoretically supply 6 ZJ of energy per year.[11]
- Biomass captures approximately 1.8 ZJ of solar energy per year.[12][3]
- Worldwide energy consumption was 0.471 ZJ in 2004.[13]
The upper map (right) shows how solar radiation at the top of the earth's atmosphere varies with latitude, while the lower map shows annual average ground-level insolation. For example, in North America, the average insolation at ground level over an entire year (including nights and periods of cloudy weather) lies between 125 and 375 W/m² (3 to 9 kWh/m²/day).[14] At present, photovoltaic panels typically convert about 15 percent of incident sunlight into electricity; therefore, a solar panel in the contiguous United States, on average, delivers 19 to 56 W/m² or 0.45 - 1.35 kWh/m²/day.[15]
Types of technologies
There are many technologies for harnessing solar energy within these broad classifications: active, passive, direct and indirect.
- Active solar systems use electrical and mechanical components such as tracking mechanisms, pumps and fans to capture sunlight and process it into usable outputs such as heating, lighting or electricity.
- Passive solar systems use non-mechanical techniques to control the capture of sunlight and distribute this energy into usable outputs such as heating, lighting, cooling or ventilation. These techniques include selecting materials with favorable thermal properties to absorb and retain energy, designing spaces that naturally circulate air to transfer energy and referencing the position of a building to the sun to enhance energy capture. In some cases passive solar devices can have mechanical movement with the important distinction that this movement is automatic and directly powered by the sun.
- Direct solar generally refers to technologies or effects that involve a single-step conversion of sunlight that results in a usable form of energy.
- Indirect solar generally refers to technologies or effects that involve multiple-step transformations of sunlight that result in a usable form of energy.
Architecture and urban planning

Solar architecture controls the use of solar energy to provide comfortable temperatures, lighting and air quality. Solar architecture does this by tailoring building orientation, proportion, material components, and window placement to the local climate and environment.
The oldest principle of solar architecture is building orientation, which involves positioning a building in a way that considers environmental conditions such as topography and ground cover as well as overshadowing from trees or structures. As a rule, a solar-friendly building's lengthwise axis should run east/west and the structure should be twice as long as it is wide. The structure also can be designed to naturally circulate air or incorporate cooling elements such as a solar chimney to help with ventilation.
In general, windows facing the equator should be equal to five to seven percent of the building's floor space.[16] If heating is a concern, window area facing away from the equator should be minimized and insulated glazing with low-emissivity coatings should be used to maximize solar gain while reducing heat losses by 30 to 50 percent. In hot climates, low-emissivity coatings on the outside of window panes can be used to reduce and control solar gain.[17]
Interior thermal mass placement should be considered in conjunction with window placement to smooth out temperature swings. The impact of exterior thermal mass placement on heat gain and glare should also assessed as part of an integrated solar design package.
Urban heat islands (UHI) are metropolitan areas with significantly higher temperatures than the surrounding environment. These higher temperatures are the result of urban materials such as concrete and asphalt that have lower albedos and higher heat capacities than the natural environment. The albedo of an object indicates the percentage of light it reflects. Asphalt has an albedo of around 10 percent, while the average albedo of the Earth is 30 percent.[18] A straightforward method of counteracting the UHI effect is to paint buildings and roads white and plant trees. A hypothetical "cool communities" program in Los Angeles has projected that urban temperatures could be reduced by approximately 3 °C after planting ten million trees, reroofing five million homes, and painting one-quarter of the roads. The estimated cost of the cool communities program is approximately US$1 billion, with an annual benefit estimated at $170 million resulting from reduced air-conditioning costs alone. An additional $360 million in health costs could be saved by the associated reductions in smog.[19][20]
Solar lighting
The history of lighting is dominated by the use of natural light. The Romans recognized the Right to Light as early as the 6th century and English law echoed these judgments with the Prescription Act of 1832. It wasn't until the 20th century that artificial lighting took over as the main source of interior illumination. The 1973 oil and 1979 energy crises brought attention to conservation measures such as natural lighting but interest waned on both occasions with the restoration of energy supplies. Approximately 22 percent (8.6 EJ)[21] of the electricity used in the United States is for lighting. When daylighting techniques are appropriately applied, natural light can supply interior lighting for a significant portion of the day.[6]
Daylighting is a passive solar method of using sunlight to provide illumination. Daylighting directly offsets energy use in electric lighting systems and indirectly offsets energy use through a reduction in cooling loads.[22] Although difficult to quantify, the use of natural lighting also offers physiological and psychological benefits compared to artificial lighting. Daylighting features include building orientation, window orientation, exterior shading, sawtooth roofs, clerestory windows, light shelves, skylights and light tubes.[23] These features may be incorporated into existing structures but are most effective when integrated in a solar design package that accounts for factors such as glare, heat gain, heat loss and time-of-use. Architectural trends increasingly recognize daylighting as a cornerstone of sustainable design.
Hybrid solar lighting (HSL) is an active solar method of using sunlight to provide illumination. Hybrid solar lighting systems collect sunlight using focusing mirrors that track the sun. The collected light is transmitted via optical fibers into a building's interior to supplement conventional lighting. In single-story applications, these systems are able to transmit 50 percent of the direct sunlight received.[6]
Daylight saving time (DST) utilizes solar energy by matching available sunlight to the time of the day in which it is most useful. DST shifts electricity use from evening to morning hours thus lowering evening peak loads and the higher costs associated with peaking electricity. In California, winter season DST has been estimated to cut daily peak load by 3 percent and total electricity use by 3400 MWh.[24] DST has been estimated to reduce early spring and late fall peak loads by 1.5 percent and total daily electricity use by 1000-2000 MWh.[24] DST, like other solar energy technologies, has not proven successful in all regions.
Solar thermal
Solar thermal applications make up the most widely used category of solar energy technology. These technologies use heat from the sun for water and space heating, ventilation, industrial process heat, cooking, water distillation and disinfection, and many other applications.[25]
Water heating
Solar hot water systems use sunlight to heat water. Commercial solar water heaters began appearing in the United States in the 1890s. These systems saw increasing use until the 1920s but were gradually replaced by relatively cheap and more reliable conventional heating fuels. The economic advantage of conventional heating fuels has varied over time resulting in periodic interest in solar hot water; however, solar hot water technologies have yet to show the sustained momentum they had until the 1920s. Recent price spikes, erratic availability of conventional fuels, and other factors are renewing interest in solar heating technologies.[5][26] Approximately 14 percent (15 EJ) of the total energy used in the United States is for water heating.[27] In many climates, a solar heating system can provide 50 to 75 percent of domestic hot water use.
As of 2005, the total installed capacity of solar hot water systems is 88 GWth and growth is 14 percent per year.[28] China is the world leader in the deployment of solar hot water systems with 80% of the market.[29] Israel is the per capita leader in the use of solar hot water with 90 percent of homes using this technology.[28] In the United States heating swimming pools is the most successful application of solar hot water.[26]
Solar water heating technologies have high efficiencies relative to other solar technologies. Performance will depend upon the site of deployment, but flat-plate and evacuated-tube collectors can be expected to have efficiencies above 60 percent during normal operating conditions.[30] In addition, solar water heating is particularly appropriate for low-temperature (25-70 °C) applications such as swimming pools, domestic hot water, and space heating. The most common types of solar water heaters are batch systems, flat plate collectors and evacuated tube collectors.
Heating, cooling and ventilation
- A thermal mass is a body that absorbs and holds heat. In the context of solar energy, it is a mass designed to store heat during sunny periods and release heat during alternate periods. A properly sized thermal mass will smooth out temperature swings and help keep rooms at a comfortable temperature throughout the day and night.
- A Trombe wall is a passive solar heating and ventilation system consisting of an air channel sandwiched between a window and a sun-facing thermal mass. During the ventilation cycle, sunlight stores heat in the thermal mass and warms the air channel causing circulation through vents at the top and bottom of the wall. During the heating cycle the Trombe wall radiates stored heat.[31]
- A transpired collector is a perforated sun-facing wall. The wall absorbs sunlight and pre-heats air as much as 22 °C as it is drawn into the ventilation system. These systems are highly efficient (up to 80 percent) and can pay for themselves within 3 to 12 years in offset heating costs.[32]
- Solar cooling can be achieved via absorption refrigeration cycles, desiccant cycles and solar mechanical processes. In 1878, Auguste Mouchout pioneered solar cooling by making ice using a solar steam engine attached to a refrigeration device.[5]
- A solar chimney (or thermal chimney) is a passive solar ventilation system composed of a hollow thermal mass connecting the interior and exterior of a building. As the chimney warms, the air inside is heated causing an updraft that enhances the natural stack ventilation through a building. These systems have been in use since Roman times and are common in the Middle east.
Process heat

A solar pond is a pool of salt water that collects and stores solar energy. Solar ponds were first proposed by Dr. Rudolph Bloch in 1948 after he came across reports of a lake in Hungary in which the temperature increased with depth. This effect was due to salts in the lake's water, which created a "density gradient" that prevented convection currents. A prototype was constructed in 1958 on the shores of the Dead Sea near Jerusalem.[33] The pond consisted of layers of water that successively increased from a weak salt solution at the top to a high salt solution at the bottom. This solar pond was capable of producing temperatures of 90 °C in its bottom layer and had an estimated solar-to-electric efficiency of two percent. Current representatives of this technology include a 150 kW pond in En Boqeq, Israel, and another used for industrial process heat at the University of Texas El Paso.[34]
Salt evaporation ponds use solar energy to concentrate brine solutions used in leach mining, remove dissolved solids from waste streams, or obtain salt from sea water. An evaporation pond consists of a shallow layer of water that can evaporate at a rate of 3-6 mm/day. The use of evaporation ponds to obtain salt from sea water is one of the oldest applications of solar energy, and evaporation ponds remain one of the largest commercial applications of solar energy used today.[35]
Concentrating solar technologies have been investigated as a means of providing process heat to help offset heat from costly electrical resistance systems for producing aluminum.[36]
Cooking

Solar cookers (or solar ovens) use sunlight for cooking, drying and pasteurization. Solar cookers offset fuel costs, reduce demand for firewood, and improve air quality by removing a source of smoke. The most common designs are box cookers, concentrating cookers and panel cookers.
Solar box cookers consist of an insulated container with a transparent lid. Horace de Saussure developed this design in 1767 after observing: "It is a known fact, and a fact that has probably been known for a long time, that a room, a carriage, or any other place is hotter when the rays of the sun pass through glass." These cookers can be used effectively with partially overcast skies and can reach temperatures of 50-100 °C. These are the cheapest and most widely used cooker design.[5][37]
Concentrating solar cookers use a parabolic reflector to concentrate light on a container positioned at the reflector's focal point. These designs cook faster and at higher temperatures (up to 315 °C). As with other concentrating technologies, these cookers require direct light and must be repositioned periodically to "track" the sun.[37]
Solar Panel cookers (SPC) use flat reflectors to concentrate sunlight on a container within a transparent covering. Roger Bernard is credited with introducing panel cookers in 1994. This design uses partial concentration and will maintain effective operation with limited repositioning.
Desalination and disinfection
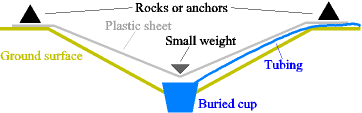
A solar still uses solar energy to distill water. A few basic types of solar stills are cone shaped, boxlike, and pit. For cone solar stills, impure water is inserted into the container, where it is evaporated by sunlight coming through clear plastic. The pure water vapor condenses on top and drips down to the side, where it is collected and removed. The most sophisticated of these are the box shaped types; the least sophisticated are the pit types.
Solar water pasteurization uses solar energy to disinfect water. The basic pasteurization process consists of heating water to 60-70 °C and holding the temperature steady for a specified period depending on the organisms present. The most heat resistant organisms will be rendered inert by a temperatures of 70 °C for ten minutes, 75 °C for one minute, and 80 °C for five seconds.[38][39]
Solar water disinfection (SODIS) is another method of disinfecting water using sunlight. The basic process involves filling a clear container 3/4 with water, shaking the container vigorously for 20 seconds, topping off the container, and placing it in the sun. Shaking the container aerates the water, which encourages disinfection. As sunlight shines into the container, the UV-A radiation causes the dissolved oxygen to become highly reactive. This reactive form of oxygen kills microorganism directly and interferes with the reproduction cycle of bacteria. As the container warms, harmful organisms are also destroyed by heat treatment. Although endorsed by the World Health Organization, SODIS is not as effective as pasteurization and the completeness of disinfection is not easily measurable.[40][41][42]
Solar power
Solar power plants use a variety of methods to collect sunlight and convert this energy into electricity. Traditionally, concentrating solar thermal power plants have been the most common type; however, multi-megawatt photovoltaic sites are seeing more-rapid deployment. Experimental solar power plants also have been built using technologies other than concentrating solar or photovoltaics, but no recent breakthroughs have been reported.
Photovoltaics

A solar cell or photovoltaic cell is a device that converts light into electricity using the photoelectric effect. The first working solar cells were constructed by Charles Fritts in 1883. These prototype cells were made of selenium and achieved efficiencies around one percent. Following the fundamental work of Russell Ohl in the 1940s, researchers Gerald Pearson, Calvin Fuller and Daryl Chapin created the silicon solar cell in 1954.[43] This breakthrough marked a fundamental change in how power is generated. The subsequent development of solar cells during the 1950s raised the efficiency of solar cells from 6 percent up to 10 percent[44] but commercial applications were limited to novelty items due to the high costs of solar cells ($300 per watt).[43]

In 1958, photovoltaic modules were used successfully as a power source for the Vanguard I satellite, followed by a string of additional solar-powered Russian and American satellites. Despite NASA's early focus on nuclear power, solar power had become the established source of power for satellites by the late 1960s. Solar power also played an essential part in the success of early commercial satellites such as Telstar and Syncom.[43]
For terrestrial applications, photovoltaic costs remained above $100 per watt throughout the 1960s, limiting commercial success. However, work by Dr. Elliot Berman during the early 1970s lowered the costs of solar cells from $100 to $20 per watt. This price reduction made solar cells competitive in a range of applications, especially for remote (off-grid) sites. Uses included cathodic protection of pipe lines and power for off-shore oil rigs, railroad crossings and lighthouses.[43]
The development of solar power was significantly affected by the 1973 oil and 1979 energy crises. These crises prompted a search for alternatives to oil, and incentive programs such as the Federal Photovoltaic Utilization Program in the U.S. and the Sunshine Program in Japan were direct results. An additional result was the establishment of research facilities such as the Solar Energy Research Institute (now NREL) in the U.S., Japan's New Energy and Industrial Technology Development Organization (NEDO) and the Fraunhofer Institute for Solar Energy Systems ISE in Germany.[45] These developments and others helped PV production expand quickly from 500 kW in 1977 to 5 MW in 1981 and 9 MW in 1982.[46]
Unfortunately for the industry, as oil prices began to fall in the early 1980s so too did the growth rate of PV. Historically-low oil prices from 1986-1999 helped keep funding for solar power research relatively low and largely removed solar power from the public consciousness.[47] Despite the lack of attention annual growth of PV ranged from 10 to 20 percent throughout the 1980s and 1990s and worldwide installation of PV reached 1000 MW in 1999.[48]
To take advantage of electromagnetic radiation from the sun, solar panels can be attached to individual houses or buildings. The panels should be mounted perpendicular to the arc of the sun to maximize usefulness. The easiest way to use this electricity is by connecting the solar panels to a grid tie inverter. However, these solar panels also may be used to charge batteries or other energy storage device. Solar panels produce more power during summer months because they receive more sunlight during the day and at a more-direct angle of incidence.
Total peak power of installed PV is around 6,000 MW as of the end of 2006. Installed PV is projected to increase to over 9,000 MW in 2007.[49][50]
Declining manufacturing costs (dropping at three to five percent a year in recent years) are expanding the range of cost-effective uses. The average lowest retail cost of a large photovoltaic array declined from $7.50 to $4.00 per watt between 1990 and 2005.[51] With many jurisdictions now giving tax and rebate incentives, solar electric power can now pay for itself in five to ten years in many places. "Grid-connected" systems - those systems that use an inverter to connect to the utility grid instead of relying on batteries - now make up the largest part of the market.
In 2003, worldwide production of solar cells increased by 32 percent.[52] Between 2000 and 2004, the increase in worldwide solar energy capacity was an annualized 60 percent.[53] 2005 was expected to see large growth again, but shortages of refined silicon have been hampering production worldwide since late 2004.[54] Analysts have predicted similar supply problems for 2006 and 2007.[55] but there are only small amount of solar cell companies in the worldwide market.

Photovoltaics is gaining credence among private investors as having the potential to grow into the next big industry. Many companies and venture capitalists are investing in photovoltaic development and manufacturing. This trend is particularly visible in Silicon valley, California.[56][57][58] Nanotechnology manufacturing using CIGS solar cells promises to produce electricity at a cost of around 5¢/kWh and sell for $0.36 per peak watt,[59] approximately one tenth of the average 2007 prices for solar panels.[60]
Deployment of solar power depends largely upon local conditions and requirements. All industrialised nations share a need for electricity and it is believed that solar power will increasingly be used as an option for supply. The Very Large Scale Photovoltaic Power Generation (VLS-PV) proposal argues that "PV systems could generate many times the current primary global energy supply."[61] To compensate for night time energy demands they would need to be complemented with pumped storage.
Concentrating solar
In 1866, the French engineer Auguste Mouchout successfully powered a steam engine with sunlight. This was the first known example of a concentrating solar-powered mechanical device. Over the next 50 years, inventors such as John Ericsson, and Frank Shuman developed solar-powered devices for irrigation, refrigeration and locomotion. The progeny of these early developments are the concentrating solar thermal power plants of today.[5]
Concentrating Solar Thermal (CST) systems use lenses or mirrors and tracking systems to focus a large area of sunlight into a small beam. CST technologies require direct insolation to function; therefore, these technologies are at a disadvantage in significantly overcast locations.[62] The three basic CST technologies are the solar trough, solar power tower and parabolic dish. Each is capable of producing high temperatures and correspondingly high thermodynamic efficiencies, but they vary in the way they track the sun and focus light.
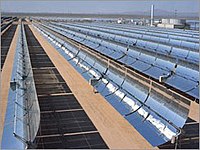
Line focus/Single-axis
A solar trough consists of a linear parabolic reflector that concentrates light onto a receiver positioned along the reflector's focal line. These systems use single-axis tracking to follow the sun. A working fluid (oil, water) flows through the receiver and is heated to 400 °C before transferring its heat to a distillation or power generation system.[63].[64] Trough systems are the most developed CST technology. The Solar Electric Generating System (SEGS) plants in California and Plataforma Solar de Almería's SSPS-DCS plant in Spain are representatives of this technology.[63]
Point focus/Dual-axis
A solar power tower consists of an array of flat reflectors (heliostats) that concentrate light on a central receiver atop a tower. These systems use dual-axis tracking to follow the sun daily and seasonally, as focusing is critical. A working fluid (air, water, molten salt) flows through the receiver where it is heated to 1000 °C before transferring its heat to a power generation or energy storage system. Power towers are less advanced than trough systems but they offer higher efficiency and better energy storage capability.[65] The Solar Two in Daggett, California and the Planta Solar 10 (PS10) in Sanlucar la Mayor, Spain are representatives of this technology.
A parabolic dish or dish/engine system consists of a stand-alone parabolic reflector that concentrates light onto a receiver positioned at the reflector's focal point. These systems use dual-axis tracking to follow the sun. A working fluid (hydrogen, helium, air, water) flows through the receiver where it is heated to 1500 °C before transferring its heat to a sterling engine for power generation.[64][65] Parabolic dish systems display the highest solar-to-electric efficiency among CST technologies and their modular nature offers scalability. The Stirling Energy Systems (SES) and Science Applications International Corporation (SAIC) dishes at UNLV and the Big Dish in Canberra, Australia, are representatives of this technology.
Updraft tower
A solar updraft tower (also known as a solar chimney or solar tower) consists of a large greenhouse that funnels into a central tower. As sunlight shines on the greenhouse, the air inside is heated and expands. The expanding air flows toward the central tower where a turbine converts the air flow into electricity. A 50 kW prototype was constructed in Ciudad Real, Spain and operated for eight years before decommissioning in 1989.[66]
Solar vehicles

Development of a practical solar powered car has been an engineering goal for 20 years. The center of this development is the World Solar Challenge, a biannual solar-powered car race covering over 3,021 km (1877mi) across central Australia from Darwin to Adelaide. The race's objective is to promote research into solar-powered cars and teams from universities and enterprises participate. In 1987, when it was founded, the winner's average speed was 67 km/h (42 mph).[67] The 2007 race included a new challenge class using cars that required an upright seating position and which, with little modification, could be the basis for a practical proposition for sustainable transport. The winning car averaged 90.87 km/h.[68]

Helios, named for the Greek sun god, was a prototype solar-powered unmanned aircraft. AeroVironment, Inc. developed the vehicle under NASA's Environmental Research Aircraft and Sensor Technology (ERAST) program.
On 13 August, 2001, it set an unofficial world record for sustained altitude by a winged aircraft. It sustained flight above 96,000 feet (29,250 m) for 40 minutes and reached 96,863 feet (29,524 m) in the process. Later, in June 2003, the prototype broke apart and fell into the Pacific Ocean about ten miles (16 km) west of the Hawaiian Island Kauai.
The first practical solar boat was constructed in 1975 in England (see Electrical Review Vol 201 No 7 12 August 1977). By 1995, solar passenger boats began appearing and are now used extensively.[69] Solar powered boats have advanced sufficiently to cross the Atlantic Ocean. The first crossing was achieved in the winter of 2006/2007 by the solar catamaran sun21.[70]
A solar balloon is a black balloon that is filled with ordinary air. As sunlight shines on the balloon, the air inside is heated and expands, causing an upward buoyancy force, much like an artificially-heated hot air balloon. Some solar balloons are large enough for human flight, but usage is limited to the toy market as the surface-area to payload-weight ratio is rather high.
Solar chemical
Solar chemical processes convert solar energy into chemical energy. These processes use both light (photochemical) and heat (endothermic) to drive chemical, thermochemical or thermoelectric reactions. Solar chemical reactions can be used to store solar energy or offset energy that would otherwise be required from an alternate source.
Electrochemical cells, commonly known as batteries, convert electrical energy into chemical energy. Solar energy can be converted indirectly into chemical energy in a system involving a photovoltaic-to-electrochemical cell exchange. A more direct approach involves the use of photoelectrochemical cells that use light to produce hydrogen in a process similar to the electrolysis of water. A third approach involves the use of thermoelectic devices that convert a temperature difference between dissimilar metals into an electric current between those metals. This current can be use to produce hydrogen and oxygen through the electrolysis of water. The solar pioneer Mouchout envisioned using the thermoelectric effect to store solar energy for later use; however, his experiments toward this end never progressed beyond primitive devices.[5]
Concentrating solar thermal technologies can be used to drive high-temperature chemical processes.
- Ammonia can be decomposed into nitrogen and hydrogen at high temperatures (650-700 °C), and the stored gases can be recombined to generate heat or electricity via a fuel cell. A prototype system was constructed at the Australian National University.[71][72]
- Zinc Oxide (ZnO) can be decomposed at high temperatures (1200-1750 °C). The resulting pure zinc can be marketed directly or the zinc can be reacted with water at (350 °C) to produce ZnO and hydrogen.[73][74]
- Water can be directly dissociated at high temperatures (2300-2600 °C). These process have so far been limited due to their high level of complexity and low solar-to-hydrogen efficiency (1-2%).[75] An alternate path of research is investigating solar thermochemical cycles that can be used to dissociate water at lower temperatures. Thermochemical cycles are at the prototype stage.[76][77]
While not a technology, photosynthesis is by far the most important photochemical interaction. Most life on earth depends on the ability of plants to photosynthesize light in the visible, ultraviolet, near infrared, and far infrared regions of the electromagnetic spectrum.
Solar mechanical

Solar mechanical technologies convert solar energy into mechanical energy or use sunlight to produce a mechanical effect. Solar mechanical devices were widely investigated by solar pioneers such as Auguste Mouchout, John Ericsson, Charles Tellier and Frank Shuman. In general, these devices concentrated sunlight on a boiler to produce steam which was then used by a steam engine to perform useful work. Most of these technologies were displaced early in the 20th century as increasingly cheap fossil fuels made them economically noncompetitive but several solar mechanical technologies have since been developed.
- A light mill or Crookes radiometer is a simple solar mechanical device consisting of a glass bulb containing a set of vanes mounted on a spindle. The vanes rotate when exposed to light with the rotation being proportional to the intensity of light.
- Passive solar tracking devices use imbalances caused by the movement of a low boiling point fluid to track the movement of the sun. These systems can improve performance by 25% over fixed tilt PV systems.[78]
- Passive solar shading systems automatically reposition in response to the movement of the sun. These systems also use imbalances caused by the movement of fluids to respond to the sun. Passive shading systems can be used to reduce summer cooling load and glare while maximizing natural lighting during the winter.[79]
Energy storage
Solar energy has traditionally been stored as heat in thermal storage systems or chemically in batteries. Solar energy has been experimentally stored thermochemically in phase change materials and at high temperatures using molten salts. The storage of excess solar energy allows for the availability of this energy during hours of darkness or cloud cover.

Thermal mass systems use various methods and materials (adobe, earth, concrete, water) to store solar energy for short or long durations (Seasonal thermal store). Thermal mass can be used to lower peak demand, shift time-of-use to off-peak hours and reduced overall heating and cooling requirements.
Solar energy can be stored thermochemically with phase change materials (PCM). Devices of this type that store latent heat can be thought of as heat batteries. Phase change materials are classified as organic (paraffins, fatty acids) and inorganic (salts, metals, alloys).[80]
- A Paraffin wax thermal storage system consists of a solar hot water loop connected to a paraffin wax tank. During the storage cycle, hot water flows through the storage tank melting the paraffin. The enthalpy of fusion for paraffin is 210-230 kJ/kg. During the heating cycle, stored heat is extracted from the tank as the wax resolidifies. These systems heat air and water to 64 °C and can reduce conventional energy use by 50 to 70 percent.[81][80]
- Eutectic salts such as Glauber's salt also can be employed in thermal storage systems. Glauber's salt are relatively inexpensive and readily available and can store 347 kJ/kg and deliver heat at 64 °C. The Dover house (Dover, Mass.) was the first to use a Glauber's salt heating system in 1948.Cite error: A
<ref>
tag is missing the closing</ref>
(see the help page).
Solar energy can be stored at high temperatures using molten salts. Salts are an effective storage medium because they are nonflammable, nontoxic, low-cost, have a high specific heat capacity, and can deliver heat at temperatures compatible with conventional power systems. A molten salt storage system consists of a salt loop connected to an insulated storage tank. During the heating cycle, the salt mixture is heated from 290 °C to 565 °C. During the power cycle, the salt is used to make steam for a steam-electric power plant. The Solar Two used this method of energy storage, allowing it to store 1.44 TJ (400,000 kWh) in its 68 m³ storage tank with an annual storage efficiency of about 99 percent.[82]
Rechargeable batteries can be used to store excess electricity from a photovoltaic system. This type of storage system consists of a photovoltaic power source connected to a battery bank via a charge controller and inverter. Lead acid batteries are the most common type of battery associated with photovoltaic systems because of their relatively low upfront costs and high availability. Lead acid batteries have an energy density of 110-140 kJ/kg, a charge/discharge efficiency of 70-92 percent and cost $150-200 per kWh ($45 to $55 per MJ). Lead batteries used in off-grid applications should be sized for three to five days of capacity and should limit depth of discharge to 50 percent to minimize cycling and prolong battery life.[83] Newer batteries can be deep discharged for over 25,000 cycles.[84]
Excess electricity from photovoltaic systems also can be sent to the transmission grid where it can be used to meet existing demand or temporarily stored for later use. Net metering (Grid-tied electrical system) policies give photovoltaic system owners a credit for the electricity they deliver to the grid. This credit is used to offset electricity provided from the grid when the photovoltaic system cannot meet demand.
Development, deployment and economics
Solar energy is an attractive solution to global warming.[85]
- "The Stone Age did not end for a lack of stones, and the oil age will end not for a lack of oil." — Sheik Yamani, Saudi oil minister, 1973
- "We stopped using stone because bronze and iron were superior materials, and likewise we will stop using oil when other energy technologies provide superior benefits." — Bjørn Lomborg, The Skeptical Environmentalist (New York: Cambridge University Press, 2001), p. 120[86]
The following trends are a few examples by which the solar market is being helped to become competitively sustainable:
- Government grants for research in solar technology to make production cheaper and generation more efficient.[87]
- Implementation of incentives at the federal and state levels to encourage consumers to consider solar power. Examples include government tax subsidies, partial copayment schemes and various rebates over purchase costs of solar devices. These are meant to take some of the onus off consumers and reduce risks associated with high initial deployment investments.[88]
- Adoption of an energy policy where consumers can connect their solar power systems to the local grid, and reverse feed the grid with unconsumed power (the state power authority guarantees an attractive purchase price). This has been especially popular in Germany and Japan.[89]
- Development of solar loan programs, with attractive return rates, to buffer the initial deployment costs and entice consumers to purchase solar PV systems. The most famous example is the solar loan program sponsored by UNEP helping 100,000 people finance solar power systems in India.[90] Success in India's solar program has led to similar projects in other developing areas such as Tunisia, Morocco, Indonesia and Mexico.
General Electric's Chief Engineer predicts grid parity for photovoltaics without subsidies in sunny parts of the United States by around 2015. Other companies predict an earlier date.[91]
Solar energy associations
- ISES: International Solar Energy Society International NGO supporting renewable and sustainable technologies.
- ASES: American Solar Energy Association US organization supporting solar energy, efficiency and sustainable technologies.
- SEIA: Solar Energy Industries Association US trade association of solar energy manufacturers, dealers, distributors, contractors
- Canadian Solar industry Association
- ESTIF - European Solar Thermal Industry Federation
- See also: Photovoltaic Industry Associations
Solar energy research institutes
There are many research institutions and departments at universities around the world who are active in solar energy research. Countries that are particularly active include Germany, Spain, Japan, Israel, Australia, China, and the USA.
- National Renewable Energy Laboratory NREL
- Centre for Renewable Energy Systems Technology, at Loughborough University
- Centre for Sustainable Energy Systems at the Australian National University
- Florida Solar Energy Center
- Solar Energy Laboratory at UW Madison
- See also: Photovoltaics research institutes
See also
Notes
- ^ The volume of each cube represents the amount of energy available and consumed. The amount of solar energy available to the Earth in one minute exceeds global energy demand for a year.Stockmarket Garden Stock Reports
- ^ Scheer 2002, p.8
- ^ a b c Smil 2006
- ^ "The History of Solar" (PDF). United States Department of Energy. Retrieved 2007-09-29.
- ^ a b c d e f Perlin and Butti 1981
- ^ a b c Muhs, Jeff. "Design and Analysis of Hybrid Solar Lighting and Full-Spectrum Solar Energy Systems" (PDF). Oak Ridge National Laboratory. Retrieved 2007-09-29. Cite error: The named reference "hybrid lighting" was defined multiple times with different content (see the help page).
- ^ "Natural Forcing of the Climate System". Intergovernmental Panel on Climate Change. Retrieved 2007-09-29.
- ^ "Earth Radiation Budget". NASA Langley Research Center. 2006-10-17. Retrieved 2007-09-29.
- ^ Somerville, Richard. "Historical Overview of Climate Change Science" (PDF). Intergovernmental Panel on Climate Change. Retrieved 2007-09-29.
- ^ Vermass, Wim. "An Introduction to Photosynthesis and Its Applications". Arizona State University. Retrieved 2007-09-29.
- ^ "Wind Energy Potential". American Wind Energy Association. Retrieved 2007-09-29.
- ^ Whittaker and Likens 1975 pp. 305-328
- ^ "International Energy Outlook 2007". Energy Information Administration. 2007-05. Retrieved 2007-09-29.
{{cite web}}
: Check date values in:|date=
(help) - ^ "Dynamic Maps, GIS Data, and Analysis Tools - Solar Maps". National Renewable Energy Laboratory. Retrieved 2007-09-29.
- ^ "PV Solar Radiation (Flat Plate, Facing South, Latitude Tilt)". National Renewable Energy Laboratory. Retrieved 2007-09-29.
- ^ Kriescher, Paul (2006-07-10). "A Solar Ready Home from an Energy Rater's Perspective" (PDF). Lightly Treading, Inc. Retrieved 2007-09-29.
- ^ "Low-emissivity Window Glazing or Glass". United States Department of Energy. Retrieved 2007-09-29.
- ^ Markvart, Tom (2003). Practical Handbook of Photovoltaics: Fundamentals and Applications. Elsevier Advanced Technology.
{{cite book}}
: Unknown parameter|coauthors=
ignored (|author=
suggested) (help) - ^ Rosenfeld, Arthur. "Painting the Town White -- and Green". Heat Island Group. Retrieved 2007-09-29.
{{cite web}}
: Unknown parameter|coauthors=
ignored (|author=
suggested) (help) - ^ Lomborg, Bjorn (2007). Cool It (The Skeptical Environmentalist's Guide to Global Warming). Knopf Publishing Group. pp. 21, 171.
- ^ "Lighting Research and Development". Department of Energy. Retrieved 2007-11-08.
- ^ Maxwell, Gregory. "Daylighting – HVAC Interaction Tests for the Empirical Validation of Building Energy Analysis Tools" (PDF). International Energy Agency. p. 85. Retrieved 2007-09-29.
{{cite web}}
: Unknown parameter|coauthor=
ignored (|author=
suggested) (help) - ^ "Daylighting". United States Department of Energy. Retrieved 2007-09-29.
- ^ a b Metz, Daryl. "Effects of Daylight Saving Time on California Electricity Use" (PDF). California Energy Commition. p. 6. Retrieved 2007-11-08.
- ^ "Solar Energy Technologies and Applications". Canadian Renewable Energy Network. Retrieved 2007-10-22.
- ^ a b Perlin, John. "Solar Hot Water Heating". California Solar Center. Retrieved 2007-09-29.
- ^ "R&D on Heating, Cooling, and Commercial Refrigeration". Department of Energy. Retrieved 2007-11-08.
- ^ a b Del Chiaro, Bernadette. "Solar Water Heating (How California Can Reduce Its Dependence on Natural Gas)" (PDF). Environment California Research and Policy Center. Retrieved 2007-09-29.
{{cite web}}
: Unknown parameter|coauthor=
ignored (|author=
suggested) (help) - ^ Li, Ling (2007-05-08). "China to Push Use of Solar Water Heaters". Worldwatch Institute. Retrieved 2007-09-29.
- ^ Schittich, Christian (2003). Solar Architecture (Strategies Visions Concepts). Architektur-Dokumentation GmbH & Co. KG. p. 166.
- ^ "Indirect Gain (Trombe Walls)". United States Department of Energy. Retrieved 2007-09-29.
- ^ "Solar Buildings (Transpired Air Collectors - Ventilation Preheating" (PDF). National Renewable Energy Laboratory. Retrieved 2007-09-29.
- ^ Halacy, Daniel (1973). The Coming Age of Solar Energy. Harper and Row.
- ^ "Israel's 150 kW Solar Pond". Mother Earth News. May/June 1980. Retrieved 2007-09-29.
{{cite news}}
: Check date values in:|date=
(help) - ^ Bartlett, Robert (1998). Solution Mining: Leaching and Fluid Recovery of Materials. Routledge
pages=393 & 394.
{{cite book}}
: Missing pipe in:|publisher=
(help); line feed character in|publisher=
at position 10 (help) - ^ Murray, Jean (August 1995). "Investigation of Opportunities for High-Temperature Solar Energy in the Aluminum Industry" (PDF). National Renewable Energy Laboratory. Retrieved 2007-09-30.
- ^ a b "Design of Solar Cookers". Arizona Solar Center. Retrieved 2007-09-30.
- ^ "Solar Water Pasturization". Retrieved 2007-09-30.
- ^ "A Summary of Water Pasteurization Techniques". Retrieved 2007-09-30.
- ^ Jagadeesh, Anumakonda. "Drinking Water For All" (PDF). Retrieved 2007-09-30.
- ^ Acra, A. "Water Disinfection by Solar Radiation". Retrieved 2007-09-30.
{{cite web}}
: Unknown parameter|coauthor=
ignored (|author=
suggested) (help) - ^ "Water pasteurization". Retrieved 2007-09-30.
- ^ a b c d Perlin, John. "Photovoltaics". California Solar Center. Retrieved 2007-09-29.
- ^ "A Walk Through Time". Retrieved 2007-10-25.
- ^ "Chronicle of Fraunhofer-Gesellschaft". Fraunhofer-Gesellschaft. Retrieved 2007-11-04.
- ^ Bellis, Mary. "History: Photovoltaics Timeline". About.com. Retrieved 2007-11-04.
- ^ "Annual Oil Market Chronology 1970-2006". Energy Information Administration. Retrieved 2007-11-01.
- ^ "Renewable Energy Annual - International Renewable Energy". Energy Information Administration. April 1997. Retrieved 2007-11-01.
- ^ "Solar Wave - April 2007 Edition" (PDF). Merrill Lynch. April 2007. Retrieved 2007-09-30.
- ^ "Installed PV power as of the end of 2005 in reporting IEA PVPS countries". International Energy Agency. Retrieved 2007-09-30.
- ^ "Solar Energy". Regional-Renewables. Retrieved 2007-09-30.
- ^ Jiménez, Viviana. "World Sales of Solar Cells Jump 32 Percent". Earth Policy Institute. Retrieved 2007-09-30.
- ^ Flannery, Russell (2006-03-27). "Sun King". Forbes. Retrieved 2007-09-30.
- ^ Gartner, John (2005-05-28). "Silicon Shortage Stalls Solar". Wired. Retrieved 2007-09-30.
- ^ Pichel, Jesse (2006-01-11). "2005 Solar Year-end Review & 2006 Solar Industry Forecast Polysilicon Supply Constraint Limiting Industry Growth". Piper Jaffray. Retrieved 2007-09-30.
{{cite news}}
: Unknown parameter|coauthor=
ignored (|author=
suggested) (help) - ^ "Solar power shines bright in Silicon Valley". MSNBC. 2006-09-19. Retrieved 2007-09-30.
- ^ Rooney, Brian (2007-04-07). "Valley Betting on Solar Power". ABC News. Retrieved 2007-09-30.
- ^ Marshall, Matt (2004-08-15). "World events spark interest in solar cell energy start-ups". Mercury News. Retrieved 2007-09-30.
- ^ Sachitanand, N. N. "Breakthrough in solar photovoltaics". The Hindu. Retrieved 2007-09-30.
- ^ "Photovoltaic Module Survey Retail Prices (Dec 2001-Sept 2007)". Solarbuzz. Retrieved 2007-09-30.
- ^ Kurokawa, K. (December 2006). "Summary Energy from the Desert (Practical Proposals for Very Large Scale Photovoltaic Power Generation (VLS-PV) Systems)". International Energy Agency. Retrieved 2007-09-30.
{{cite news}}
: Unknown parameter|coauthor=
ignored (|author=
suggested) (help) - ^ "Light and the PV Cell". United States Department of Energy. Retrieved 2007-09-30.
- ^ a b "Linear-focusing Concentrator Facilities: DCS, DISS, EUROTROUGH and LS3". Plataforma Solar de Almería. Retrieved 2007-09-29.
- ^ a b "CSP Technologies Overview". United States Department of Energy. Retrieved 2007-09-30.
- ^ a b "Solar thermal power plants - Technology Fundamentals". Renewable Energy World. Retrieved 2007-09-29.
- ^ Mills, David (2004). "Advances in solar thermal electricity technology". Solar Energy. 76 (1–3): 19–31. doi:10.1016/S0038-092X(03)00102-6.
- ^ "History of World Solar Challenge". Panasonic World Solar Challenge. Retrieved 2007-09-30.
- ^ Final Results
- ^ Schmidt, Theodor. "Solar Ships for the new Millennium". TO Engineering. Retrieved 2007-09-30.
- ^ "The sun21 completes the first transatlantic crossing with a solar powered boat". Transatlantic 21. Retrieved 2007-09-30.
- ^ Lovegrove, Keith (2004). "Developing Ammonia Based Thermochemical Energy Storage for Dish Power Plants" (PDF). Solar Energy. 76: 331–337.
{{cite journal}}
: Unknown parameter|coauthor=
ignored (|author=
suggested) (help) - ^ Thomas, George. "Potential Roles of Ammonia in a Hydrogen Economy" (PDF).
{{cite web}}
: Unknown parameter|coauthor=
ignored (|author=
suggested) (help) - ^ "Solar thermal ZnO-decomposition". Paul Scherrer Institut.
- ^ Genuth, Iddo (2005-09-09). "Zinc Powder Will Drive Your Hydrogen Car". IsraCast.
{{cite news}}
:|access-date=
requires|url=
(help); Unknown parameter|coauthor=
ignored (|author=
suggested) (help); Unknown parameter|http://www.isracast.com/Articles/Article.aspx?ID=
ignored (help) - ^ Pyle, Walter. "Direct Solar-Thermal Hydrogen Production from Water Using Nozzle/Skimmer and Glow Discharge". Retrieved 2007-09-30.
{{cite web}}
: Unknown parameter|coauthor=
ignored (|author=
suggested) (help) - ^ "Fundamentals of a Solar-thermal Mn2O3/MnO Thermochemical Cycleto Split Water" (PDF). Retrieved 2007-09-30.
- ^ Allen, Ray. "Thermochemical Cycles and the Hydrogen Economy" (PDF). The University of Sheffield. Retrieved 2007-09-30.
{{cite web}}
: Unknown parameter|coauthor=
ignored (|author=
suggested) (help) - ^ "Passive Solar Tracker for Photovoltaic Modules". e-Marine, Inc. Retrieved 2007-11-04.
- ^ "Large louvre blades (passive)". Schüco. Retrieved 2007-11-04.
- ^ a b Gok, Özgül. "Stabilization of Glauber's Salt for Latent Heat Storage" (PDF). Çukurova University. Retrieved 2007-09-30.
{{cite web}}
: Unknown parameter|coauthor=
ignored (|author=
suggested) (help) - ^ Romanowicz, Goska (2006-05-19). "Heat 'batteries' dramatically cut energy use". edie newsroom. Retrieved 2007-09-29.
{{cite news}}
: Check|url=
value (help) - ^ "Advantages of Using Molten Salt". Sandia National Laboratory. Retrieved 2007-09-29.
- ^ "Batteries". DC Power Systems. Retrieved 2007-09-29.
- ^ How to build a battery that lasts longer than a car accessed 28 October 2007
- ^ Solar Energy Conversion Offers A Solution To Help Mitigate Global Warming accessed 5 November 2007
- ^ Technology Roadmaps accessed 5 November 2007
- ^ Carlstrom, Paul (2005-07-11). "As solar gets smaller, its future gets brighter (Nanotechnology could turn rooftops into a sea of power-generating stations)". SFGate. Retrieved 2007-09-30.
- ^ "California Formally Adopts Performance-Based Solar Incentives". Renewable Energy Access. 2006-08-29. Retrieved 2007-09-30.
- ^ Wolcott, Barbara (2001). "Solar Gains". The American Society of Mechanical Engineers. Retrieved 2007-09-30.
- ^ "UNEPs India Solar Loan Programme Wins Prestigious Energy Globe". United Nations Environment Programme. 2007-04-12. Retrieved 2007-09-30.
- ^ [1]
References
Perlin, John (1981). A Golden Thread (2500 Years of Solar Architecture and Technology). Van Nostrand Reinhold. {{cite book}}
: Unknown parameter |coauthors=
ignored (|author=
suggested) (help)
Scheer, Hermann (2002). The Solar Economy (Renewable Energy for a Sustainable Global Future). Earthscan Publications Ltd.
Smil, Vaclav (2006-05-17). "Energy at the Crossroads" (PDF). Organisation for Economic Co-operation and Development. Retrieved 2007-09-29.
Whittaker, Robert (1975). Primary Productivity of the Biosphere. Springer-Verlag. {{cite book}}
: Unknown parameter |coauthors=
ignored (|author=
suggested) (help)
External links
- Energy transitions past and future, Encyclopedia of Earth
- White Paper Discussing the use of Carbon Finance to Develop Solar Power.
- Florida Solar Innovators.
- Scientific calculation of sun exposure (house insolation, garden, roof.
- Solar Power-Renewable energy resource.
