Polylactic acid
![]() | |
Identifiers | |
---|---|
ChemSpider | |
CompTox Dashboard (EPA)
|
|
Properties | |
(C3H4O2)n | |
Density | 1.210–1.430 g·cm−3 [1] |
Melting point | 150–160 °C[1] (302–320 °F) |
Insoluble in Water[2] | |
Hazards | |
NFPA 704 (fire diamond) | |
Except where otherwise noted, data are given for materials in their standard state (at 25 °C [77 °F], 100 kPa).
|
Poly(lactic acid) or polylactide (PLA) is a biodegradable thermoplastic aliphatic polyester derived from renewable resources, such as corn starch (in the United States), tapioca roots, chips or starch (mostly in Asia), or sugarcane (in the rest of the world). In 2010, PLA had the second highest consumption volume of any bioplastic of the world.[3]
The name "poly(lactic acid)" does not comply with IUPAC standard nomenclature, and is potentially ambiguous or confusing, because PLA is not a polyacid (polyelectrolyte), but rather a polyester.[4]
Production
There are several industrial routes to usable (i.e. high molecular weight) PLA. Two main monomers are used: lactic acid, and the cyclic di-ester, lactide. The most common route to PLA is the ring-opening polymerization of lactide with various metal catalysts (typically tin octoate) in solution, in the melt, or as a suspension. The metal-catalyzed reaction tends to cause racemization of the PLA, reducing its stereoregularity compared to the starting material.[5]
Another route to PLA is the direct condensation of lactic acid monomers. This process needs to be carried out at less than 200 °C; above that temperature, the entropically favored lactide monomer is generated. This reaction generates one equivalent of water for every condensation (esterification) step, and that is undesirable because water causes chain-transfer leading to low molecular weight material. The direct condensation is thus performed in a stepwise fashion, where lactic acid is first oligomerized to PLA oligomers. Thereafter, polycondensation is done in the melt or as a solution, where short oligomeric units are combined to give a high molecular weight polymer strand. Water removal by application of a vacuum or by azeotropic distillation is crucial to favor polycondensation over transesterification. Molecular weights of 130 kDa can be obtained this way. Even higher molecular weights can be attained by carefully crystallizing the crude polymer from the melt. Carboxylic acid and alcohol end groups are thus concentrated in the amorphous region of the solid polymer, and so they can react. Molecular weights of 128–152 kDa are obtainable thus.[5]
Polymerization of a racemic mixture of L- and D-lactides usually leads to the synthesis of poly-DL-lactide (PDLLA), which is amorphous. Use of stereospecific catalysts can lead to heterotactic PLA which has been found to show crystallinity. The degree of crystallinity, and hence many important properties, is largely controlled by the ratio of D to L enantiomers used, and to a lesser extent on the type of catalyst used. Apart from lactic acid and lactide, lactic acid O-carboxyanhydride ("lac-OCA"), a five-membered cyclic compound has been used academically as well. This compound is more reactive than lactide, because its polymerization is driven by the loss of one equivalent of carbon dioxide per equivalent of lactic acid. Water is not a co-product.[6]
The direct biosynthesis of PLA similar to the poly(hydroxyalkanoate)s has been reported as well.[7]
Manufacturers
As of June 2010, NatureWorks was the primary producer of PLA (bioplastic) in the United States. Other companies involved in PLA manufacturing are PURAC Biomaterials (The Netherlands) and several[which?] Chinese manufacturers. The primary producer of PDLLA is PURAC, a wholly owned subsidiary of CSM located in the Netherlands. Galactic and Total Petrochemicals operate a joint venture, Futerro, which is developing a second generation polylactic acid product. This project includes the building of a PLA pilot plant in Belgium capable of producing 1,500 tonnes/year.
Chemical and physical properties
Due to the chiral nature of lactic acid, several distinct forms of polylactide exist: poly-L-lactide (PLLA) is the product resulting from polymerization of L,L-lactide (also known as L-lactide). PLLA has a crystallinity of around 37%, a glass transition temperature 60–65 °C, a melting temperature 173–178 °C and a tensile modulus 2.7–16 GPa.[8][9] Heat-resistant PLA can withstand temperatures of 110 °C.[10] PLA is soluble in chlorinated solvents, hot benzene, tetrahydrofuran, and dioxane.[11]
PLA has similar mechanical properties to PETE polymer, but has a significantly lower maximum continuous use temperature.[12]
Polylactic acid can be processed like most thermoplastics into fiber (for example, using conventional melt spinning processes) and film. The melting temperature of PLLA can be increased by 40–50 °C and its heat deflection temperature can be increased from approximately 60 °C to up to 190 °C by physically blending the polymer with PDLA (poly-D-lactide). PDLA and PLLA form a highly regular stereocomplex with increased crystallinity. The temperature stability is maximised when a 1:1 blend is used, but even at lower concentrations of 3–10% of PDLA, there is still a substantial improvement. In the latter case, PDLA acts as a nucleating agent, thereby increasing the crystallization rate. Biodegradation of PDLA is slower than for PLA due to the higher crystallinity of PDLA.
There is also poly(L-lactide-co-D,L-lactide) (PLDLLA) – used as PLDLLA/TCP scaffolds for bone engineering.[13]
Applications




PLA can be processed by extrusion, injection molding, film and sheet casting, and spinning, providing access to a wide range of materials.
Being able to degrade into innocuous lactic acid, PLA is used as medical implants in the form of anchors, screws, plates, pins, rods, and as a mesh.[15] Depending on the exact type used, it breaks down inside the body within 6 months to 2 years. This gradual degradation is desirable for a support structure, because it gradually transfers the load to the body (e.g. the bone) as that area heals. The strength characteristics of PLA and PLLA implants are well documented.[16]
PLA can also be used as a compostable packaging material, either cast, injection-molded, or spun.[15] Cups and bags have been made from this material. In the form of a film, it shrinks upon heating, allowing it to be used in shrink tunnels. It is useful for producing loose-fill packaging, compost bags, food packaging, and disposable tableware. In the form of fibers and non-woven textiles, PLA also has many potential uses, for example as upholstery, disposable garments, awnings, feminine hygiene products, and diapers.
Racemic and regular PLLA has a low glass transition temperature, which is undesirable. A stereocomplex of PDLA and PLLA has a higher glass transition temperatures, lending it more mechanical strength. It has a wide range of applications, such as woven shirts (ironability), microwavable trays, hot-fill applications and even engineering plastics (in this case, the stereocomplex is blended with a rubber-like polymer such as ABS). Such blends also have good form stability and visual transparency, making them useful for low-end packaging applications. Pure poly-L-lactic acid (PLLA), on the other hand, is the main ingredient in Sculptra, a long-lasting facial volume enhancer, primarily used for lipoatrophy of cheeks. Progress in biotechnology has resulted in the development of commercial production of the D enantiomer form, something that was not possible until recently.[17]
PLA is also used as a feedstock material in 3D printers.[18][19] The properties enable PLA printed solids to be encased in plaster-like moulding materials, then burned out in a furnace, so that the resulting void can be filled with molten metal. This is known as "lost PLA casting", a type of investment casting.
Recycling
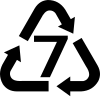
Currently, the SPI resin identification code 7 ("others") is applicable for PLA. In Belgium, Galactic started the first pilot unit to chemically recycle PLA (Loopla). Unlike mechanical recycling, waste material can hold various contaminants. Polylactic acid can be recycled to monomer by thermal depolymerization or hydrolysis. When purified, the monomer can be used for the manufacture of virgin PLA with no loss of original properties (cradle-to-cradle recycling).
Degradation
Amycolatopsis and Saccharotrix are able to degrade PLA. A purified protease from Amycolatopsis sp., PLA depolymerase, can also degrade PLA. Enzymes such as bromelain, pronase and most effectively proteinase K from Tritirachium album degrade PLA.[20]
Pure PLLA foams undergo selective hydrolysis when placed in an environment of DMEM supplemented with fetal bovine serum (a solution mimicking body fluid). After 30 days of submersion in DMEM+FBS, a PLLA scaffold lost about 20% of its weight.[21]
See also
- Cellophane
- Metallic glass
- Plastarch material
- Polycaprolactone
- Polyglycolide
- Poly-3-hydroxybutyrate
- Zein
- 3D Printing
- Acrylonitrile butadiene styrene
- Ultimaker
- Makerbot
References
- ^ a b "Matbase". Retrieved 6 February 2012.
- ^ http://www.ampolymer.com/MSDS/PLA.pdf
- ^ Market Study Bioplastics, Ceresana, Dec 2011
- ^ Martin, O; Avérous, L. "Poly(lactic acid): plasticization and properties of biodegradable multiphase systems". Polymer. 42 (14): 6209–6219. doi:10.1016/S0032-3861(01)00086-6.
- ^ a b Rafael Auras, Loong-Tak Lim, Susan E. M. Selke, Hideto Tsuji (ed.). "3. Industrial Production of High Molecular Weight Poly(Lactic Acid)". Poly(Lactic Acid): Synthesis, Structures, Properties, Processing, and Applications. doi:10.1002/9780470649848.ch3.
{{cite book}}
: Cite uses deprecated parameter|authors=
(help)CS1 maint: multiple names: editors list (link) - ^ Kricheldorf, Hans R.; Jonté, J. Michael (1983). "New polymer syntheses". Polymer Bulletin. 9 (6–7). doi:10.1007/BF00262719.
- ^ Jung, Yu Kyung; Kim, Tae Yong (2009). "Metabolic Engineering of Escherichia coli for the production of Polylactic Acid and Its Copolymers". Biotechnology and Bioengineering. 105 (1). doi:10.1002/bit.22548.
- ^ Södergård, Anders; Mikael Stolt (February 2002). "Properties of lactic acid based polymers and their correlation with composition". Progress in Polymer Science. 27 (6): 1123–1163. doi:10.1016/S0079-6700(02)00012-6.
{{cite journal}}
: Cite has empty unknown parameter:|quotes=
(help) - ^ Middelton, John C.; Arthur J. Tipton (2000). "Synthetic biodegradable polymers as orthopedic devices". Biomaterial. 21 (23): 2335–2346. doi:10.1016/S0142-9612(00)00101-0.
{{cite journal}}
: Cite has empty unknown parameter:|quotes=
(help) - ^ Gina L. Fiore; Feng Jing; Victor G. Young, Jr.; Christopher J. Cramer; Marc A. Hillmyer (2010). "High Tg Aliphatic Polyesters by the Polymerization of Spirolactide Derivatives". Polymer Chemistry (1): 870–877. doi:10.1039/C0PY00029A.
- ^ Donald Garlotta (2001). "A Literature Review of Poly(Lactic Acid)". Journal of Polymers and the Environment. 9 (2).
- ^ "Compare Materials: PLA and PETE". Makeitfrom.com. Retrieved 2011-04-11.
- ^ Attention: This template ({{cite doi}}) is deprecated. To cite the publication identified by doi:10.1080/17452750802551298, please use {{cite journal}} (if it was published in a bona fide academic journal, otherwise {{cite report}} with
|doi=10.1080/17452750802551298
instead. - ^ Fiore, G (2010). "High Tg Aliphatic Polyestersby the Polymerization o f Spir olactide Derivatives". Polym. Chem. (1): 870–877.
{{cite journal}}
: Unknown parameter|coauthors=
ignored (|author=
suggested) (help) - ^ a b Rafael Auras, Loong-Tak Lim, Susan E. M. Selke, Hideto Tsuji (ed.). Poly(Lactic Acid): Synthesis, Structures, Properties, Processing, and Applications. doi:10.1002/9780470649848. ISBN 9780470293669.
{{cite book}}
: CS1 maint: multiple names: editors list (link) - ^ J Paul Harvey and Robert F Games (ed.). ASTM STP 1217 Theoretical Strength Comparison of Bioasbsorable (PLLA) Plates and conventional stainless steel and Titanium Plates used in Internal Fracture Fixation.
- ^ "Bioengineers succeed in producing plastic without the use of fossil fuels". Physorg.com. Retrieved 2011-04-11.
- ^ "PLA". Reprap Wiki. 2011-04-04. Retrieved 2011-04-11.
- ^ "PLA". MakerBot Industries. Retrieved 2011-04-11.
- ^ Yutaka Tokiwa; Buenaventurada P. Calabia; Charles U. Ugwu; Seiichi Aiba (September 2009). "Biodegradability of Plastics". International Journal of Molecular Science. 9: 3722–3742. doi:10.3390/ijms10093722. PMC 2769161. PMID 19865515.
{{cite journal}}
: CS1 maint: unflagged free DOI (link) - ^ Pavia FC; La Carrubba V; Piccarolo S; Brucato V (August 2008). "Polymeric scaffolds prepared via thermally induced phase separation: tuning of structure and morphology". Journal of Biomedical Materials Research Part A. 2: 459–466. doi:10.1002/jbm.a.31621. PMID 17975822.
External links
