Tennessine
Tennessine | |||||||||||||||||||||
---|---|---|---|---|---|---|---|---|---|---|---|---|---|---|---|---|---|---|---|---|---|
Pronunciation | /ˈtɛnəsiːn/[1] | ||||||||||||||||||||
Appearance | semimetallic (predicted)[2] | ||||||||||||||||||||
Mass number | [294] | ||||||||||||||||||||
Tennessine in the periodic table | |||||||||||||||||||||
| |||||||||||||||||||||
Atomic number (Z) | 117 | ||||||||||||||||||||
Group | group 17 (halogens) | ||||||||||||||||||||
Period | period 7 | ||||||||||||||||||||
Block | p-block | ||||||||||||||||||||
Electron configuration | [Rn] 5f14 6d10 7s2 7p5 (predicted)[3] | ||||||||||||||||||||
Electrons per shell | 2, 8, 18, 32, 32, 18, 7 (predicted) | ||||||||||||||||||||
Physical properties | |||||||||||||||||||||
Phase at STP | solid (predicted)[3][4] | ||||||||||||||||||||
Melting point | 623–823 K (350–550 °C, 662–1022 °F) (predicted)[3] | ||||||||||||||||||||
Boiling point | 883 K (610 °C, 1130 °F) (predicted)[3] | ||||||||||||||||||||
Density (near r.t.) | 7.1–7.3 g/cm3 (extrapolated)[4] | ||||||||||||||||||||
Atomic properties | |||||||||||||||||||||
Oxidation states | (−1), (+1), (+3), (+5) (predicted)[2][3] | ||||||||||||||||||||
Ionization energies | |||||||||||||||||||||
Atomic radius | empirical: 138 pm (predicted)[4] | ||||||||||||||||||||
Covalent radius | 156–157 pm (extrapolated)[4] | ||||||||||||||||||||
Other properties | |||||||||||||||||||||
Natural occurrence | synthetic | ||||||||||||||||||||
CAS Number | 54101-14-3 | ||||||||||||||||||||
History | |||||||||||||||||||||
Naming | after Tennessee region | ||||||||||||||||||||
Discovery | Joint Institute for Nuclear Research, Lawrence Livermore National Laboratory, Vanderbilt University and Oak Ridge National Laboratory (2010) | ||||||||||||||||||||
Isotopes of tennessine | |||||||||||||||||||||
| |||||||||||||||||||||
Ununseptium is the superheavy artificial chemical element with temporary symbol Uus and atomic number 117. The element, also known as eka-astatine or simply element 117, is the second-heaviest of all the elements that have been reportedly created so far and is the second-to-last element of the 7th period of the periodic table. Its discovery was first announced in 2010—synthesis was claimed in Dubna, Russia, by a joint Russian–American collaboration, thus making it the most recently discovered element. Another experiment in 2011 created one of its daughter isotopes directly, partially confirming the results of the discovery experiment, and the original experiment was repeated successfully in 2012. However, the IUPAC/IUPAP Joint Working Party (JWP), which is in charge of examining claims of discovery of superheavy elements, has made no comment yet on whether the element can be recognized as discovered. Once it is so recognized, it may receive a permanent name which will be suggested for the element by its discoverers; "ununseptium" is a temporary systematic element name that is intended to be used before a permanent one is established. However, it is commonly called "element 117" by researchers and in the literature instead of "ununseptium".
In the periodic table, ununseptium is located in group 17,[a] all previous members of which are halogens. However, ununseptium is likely to have significantly different properties from the halogens, although a few key properties such as the melting and boiling points, as well as the first ionization energy are expected to follow the periodic trends. While scientists agree that lighter ununseptium isotopes are very unstable, there are signs that some heavier ununseptium isotopes may be much more stable.
History
Pre-discovery
In 2004 the Joint Institute for Nuclear Research (JINR) team in Dubna, Moscow Oblast, Russia proposed an experiment to synthesize element 117 (so-called for the 117 protons in its nucleus) that required fusing a berkelium (element 97) target and a calcium (element 20) beam.[9] However, the team at the Oak Ridge National Laboratory in the United States, the world's only producer of berkelium, could not then provide any, citing a lack of production of the exotic material.[9] Plans to synthesize element 117 were shelved temporarily in favor of the synthesis of element 118, which was produced by bombarding a californium target with calcium.[10]
The Russian team's desire to use an element they could not access was due to the nature of the calcium beam: the isotope of calcium used in the beam, calcium-48, has 20 protons and 28 neutrons; it is the lightest stable or near-stable nucleus with such a neutron excess. The second-lightest such nucleus, zinc-68, is much heavier.[11] The beam is made in Russia by chemically[12] extracting the small quantities of calcium-48 present in Earth's natural calcium from the remaining natural calcium.[13] Thus the resulting nuclei become heavier and closer to the sought-after island of stability, a concept wherein some super-heavy atoms can be relatively stable. Sufficiently heavy nuclei have not been created as of 2012, however, and, in a table of nuclides, these isotopes tend to have fewer neutrons than elements in those island of stability.[14]
Discovery


In 2008, the American team re-launched a program of berkelium production, and the Russian team was contacted.[9] The production resulted in 22 milligrams of berkelium, enough to perform the experiment.[16] The berkelium was subsequently cooled and chemically purified, each of which took 90 days.[17] The berkelium target had to be brought to Russia quickly: the half-life of the isotope of berkelium used (berkelium-249) is only 330 days, which means that after this period, half of it would no longer be berkelium. In fact, if the experiment had not begun in six months after the target's departure, it would have had to be canceled due to insufficient quantities of the quickly decaying berkelium.[17] In summer 2009, the target was packed into five lead containers to be sent via a commercial flight from New York to Moscow.[17]
The teams had to deal in advance with the bureaucratic barrier between the two countries to allow the target's timely journey to Russia. This, however, did not prevent such problems from occurring: missing or incomplete paperwork led to Russian customs twice refusing to let the target enter the country. Even though it traveled over the Atlantic ocean five times, the journey only took a few days in total.[17] The berkelium was then transferred to Dimitrovgrad, Ulyanovsk Oblast to be fixed on a thin titanium film, and then to Dubna where it was installed in the JINR particle accelerator, the world's most powerful for the synthesis of superheavy elements.[16]
The experiment began in June 2009 and, in January 2010, scientists at the Flerov Laboratory of Nuclear Reactions announced internally that they had succeeded in detecting the decay of a new element with atomic number 117 via two decay chains of an odd-odd isotope (undergoing 6 alpha decays before undergoing spontaneous fission) and of an odd-even one (3 alpha decays before fission).[18] On April 9, 2010 an official report was released in the journal Physical Review Letters. It revealed that the isotopes mentioned in the previous chains referred to 294Uus and 293Uus, formed as follows:[15]
- 249
97Bk
+ 48
20Ca
→ The element Uus does not exist.* → The element Uus does not exist. + 3 1
0
n
(1 event)
- 249
97Bk
+ 48
20Ca
→ The element Uus does not exist.* → The element Uus does not exist. + 4 1
0
n
(5 events)
Before the synthesis of ununseptium, none of ununseptium's daughter isotopes were known;[15] thus, there was no basis for a JWP discovery claim, much less for its recognition. Ununpentium-289, one of ununseptium's daughters, was created directly in 2011, instead of being created indirectly from the decay of ununseptium, yet it matched the claimed decay properties measured from the discovery of ununseptium.[19] The discoverers did not, however, submit a claim for the discovery of ununseptium when JWP was reviewing claims of discoveries of trans-copernicium elements (elements beyond copernicium).[20] The Dubna team repeated the experiment in 2012 successfully, thus confirming the synthesis of ununseptium and moving one step closer to the element's official entry on the periodic table. The scientists have filed an element registration paper,[21] and a new JWP staffer is already working on assigning priority of the claim.[22]
Naming
Using Mendeleev's nomenclature for unnamed and undiscovered elements, ununseptium should be known as eka-astatine or dvi-iodine. In 1979, IUPAC published recommendations according to which the element was to be called ununseptium (with the corresponding symbol of Uus),[23] a systematic element name as a placeholder, until the discovery of the element is confirmed and a permanent name is decided on. The recommendations are mostly ignored among scientists, who call it "element 117", with the symbol of (117) or even simply 117.[3] According to current guidelines from IUPAC, the ultimate name for all new elements should end in "-ium", which means the name for ununseptium will end in "-ium", not "-ine", even if ununseptium turns out to be a halogen, whose elements traditionally have names ending in "-ine".[24]
No official name has yet been suggested for the element. However, the discoverers will get the right to suggest a name as soon as it is recognized by the JWP; a Dubna authority said in June 2012 that it "could take up to a year" before this happens.[21]
Predicted properties
Nuclear stability and isotopes

The stability of nuclei decreases greatly with the increase in atomic number after plutonium, the heaviest primordial element, so that all isotopes with an atomic number above 101 decay radioactively with a half-life under a day, with the exception of dubnium-268. No elements with atomic numbers above 82 (after lead) have stable isotopes.[26] Nevertheless, because of reasons not well understood yet, there is a slightly increased nuclear stability around atomic numbers 110–114, which leads to the appearance of what is known in nuclear physics as the "island of stability". This concept, proposed by University of California professor Glenn Seaborg, explains why superheavy elements last longer than predicted.[27] Ununseptium is the second-heaviest element of all created so far and is radioactive, having a half-life that appears to be less than one second. Nonetheless, this is still longer than the predicted values used in the discovery report.[15] The Dubna team believes that the synthesis of the element is direct experimental proof of the existence of the island.[25]
Calculations using a quantum tunneling model predict the existence of several isotopes of ununseptium with alpha decay half-lives up to 40 milliseconds, culminating at 296Uus (the research ends on 303Uus).[28] A more detailed study by the Brazilian Center for Physics Research on the element's isotopes shows similar results. In particular, it is predicted that 296Uus is the most stable isotope in its region; in both cases, however, the differences are within the limits of the calculation error.[b] However, the latter goes further up to 337Uus and reveals a general increasing stability trend for isotopes heavier than 301Uus, the isotope that has 184 neutrons. 184 is considered to be a magic number in physics, which is thought to provide extra stability. 309Uus and all heavier isotopes are expected to be more stable than the synthesized two; 326Uus may have a half-life of over 300 years and the heaviest isotope for which predictions are available, 337Uus, should have a half-life of about 1016 years.[29][c]
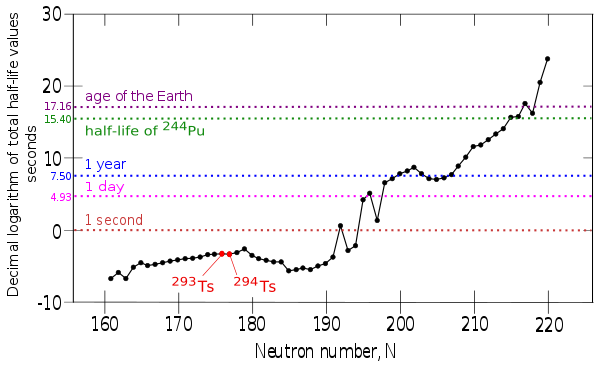
Atomic and physical
Ununseptium is a member of group 17 in the periodic table, below the five halogens (fluorine, chlorine, bromine, iodine, and astatine). Every previous group 17 element has seven electrons in its valence shell, forming a valence electron configuration of ns2np5. In ununseptium's case, the trend will be continued and the valence electron configuration is predicted to be 7s27p5;[3] therefore, ununseptium will behave similarly to the halogens in many respects.

However, notable differences are likely to arise; a largely contributing effect is the spin–orbit (SO) interaction—the mutual interaction between the electrons' motion and spin. It is especially strong for the superheavy elements, because their electrons move much faster than in lighter atoms, at velocities comparable to the speed of light, which is where the differences arise.[30] In relation to ununseptium atoms, it lowers the 7s and the 7p electron energy levels (stabilizing the corresponding electrons), but two of the 7p electron energy levels are stabilized more than the other four.[31] The stabilization of the 7s electrons is called the inert pair effect, and the effect "tearing" the 7p subshell into the more stabilized and the less stabilized parts is called subshell splitting. Computation chemists see the split as a change of the second (azimuthal) quantum number l from 1 to 1/2 and 3/2 for the more stabilized and less stabilized parts of the 7p subshell, respectively.[32][d] For many theoretical purposes, the valence electron configuration may be represented to reflect the 7p subshell split as 7s2
7p2
1/27p5
3/2.[3]
Differences for other electron levels also exist. For example, the 6d electron levels (also split in halves, with four being 6d3/2 and six being 6d5/2) are both raised, so that they are close in energy to the 7s ones,[31] although no 6d electron chemistry has been predicted for the element. The difference between the 7p1/2 and 7p3/2 levels is abnormally high, being 9.8 eV.[31] Astatine's 6p subshell split is only 3.8 eV,[31] and its 6p1/2 chemistry has already been called "limited".[33] All these effects cause ununseptium's chemistry to differ from those of its upper neighbors (see below).
The first ionization energy (the energy required to remove an electron from an atom) is predicted to be 7.7 eV, lower than those of the halogens, again following the trend.[3] Ununseptium is also in line with its neighbors in the periodic table in being expected to have the lowest electron affinity (energy released when an electron is added to the atom) in its group, 2.6 or even 1.8 eV.[3] The electron of the hydrogen-like ununseptium atom (oxidized so that it has only one electron, Uus116+) is predicted to move so fast that it has a mass of 1.9 compared to a non-moving electron, a feature coming from the relativistic effects. For comparison, the figure is 1.27 for hydrogen-like astatine and 1.08 for hydrogen-like iodine.[34] According to simple extrapolations of relativity laws, that indirectly indicates the contraction of the atomic radius;[34] however, advanced calculations show that the radius of an ununseptium atom that has formed one covalent bond would be 165 pm, while that of astatine would be 147 pm.[35] With seven outermost electrons removed, ununseptium is finally smaller: 57 pm[3] for ununseptium and 61 pm[36] for astatine.
The melting and boiling points of ununseptium are not definitely known. Earlier papers predicted about 350–500 °C and 550 °C respectively,[3] or even 350–550 °C and 610 °C respectively.[37] These values exceed the astatine (and all preceding) values, following periodic trends. A later paper, however, predicts ununseptium's boiling point to be 345 °C[38] (that of astatine is estimated to be 309 °C,[39] 337 °C,[40] or 370 °C,[41] although experimental values of 230 °C[42] and 411 °C[36] have been reported).
Chemical
The previous members of the group commonly accept another electron to achieve a stable noble-gas electronic configuration, having eight electrons (octet) in its valence shell, which is the minimum-energy configuration in which the outer electrons are tightly bound.[43] This ability weakens going down the group: as mentioned, ununseptium would be the least willing to accept an electron among the group 17 elements (astatine already forms a positive oxidation state in its hydride). In fact, out of the oxidation states the element is predicted to form, −1 is predicted to be the least common.[3]
There is another opportunity for ununseptium to complete the octet—to form a covalent bond. Accordingly, when two ununseptium atoms meet, they are expected to form a Uus–Uus bond to give diatomic molecules, just like the halogens. The sigma bonding is calculated to show a great antibonding character in the At2 molecule; ununseptium is predicted to continue the trend and a strong pi character should be seen in the bonding of Uus2.[3][44] The molecule UusCl is predicted to go further, being bonded with a single pi bond.[44]
Aside from the mentioned unstable −1 state, three more oxidation states are predicted: +5, +3, and +1, with the last one especially more stable because of the destabilization of the three outermost 7p3/2 electrons, creating high spinor energy for them, making a stable half-filled subshell configuration;[3] astatine already shows somewhat similar effects.[45] The +3 state may resemble that of Au3+ in ion-exchange behavior in a halide environment;[3] simple extrapolation of the fact the 7p3/2 electrons are relatively (compared to the 7p1/2 electrons) destabilized suggests this oxidation state should also be important.[37] The +5 state is predicted to be uncommon, as the 7p1/2 electrons are (oppositely) stabilized.[3] The +7 state has not been shown (even computationally) to exist; moreover, since the 7s electrons are very stabilized, it has been hypothesized that the valence core of the element is only five electrons.[46]
The simplest possible ununseptium compound is the monohydride, UusH. The bonding is provided by a 7p3/2 electron of ununseptium and the 1s electron of hydrogen. The non-bonding nature of the 7p1/2 spinor is because ununseptium is expected to not form purely sigma or pi bonds.[47] Therefore, the destabilized (thus expanded) 7p3/2 spinor is responsible for bonding.[48] This effect lengthens the UusH molecule by 17 picometers (compare to the overall length of 195 pm).[47] Since the ununseptium p electron bonds are two-thirds sigma, the bond is only two-thirds as strong as it would be if ununseptium featured no SO interactions.[47] The molecule thus follows the trend for halogen hydrides, showing an increase in bond length and a decrease in dissociation energy as compared to AtH.[3] The molecules TlUus and UutUus may be viewed analogously, taken in account an opposite effect shown by that the metal's p1/2 electrons are stabilized. These two result in a relatively small dipole moment for TlUus, only 1.67 D (the positive value implying the negative charge is on the ununseptium atom); and for UutUus, the effects' strength caused a transfer of the electron from the ununseptium atom to the ununtrium atom, with the dipole moment value being −1.80 D.[49] In light of the UusH data, it is interesting that the SO interaction increases dissociation energy for the UusF molecule. This is because it lowers the electronegativity of ununseptium, causing the bond with the extremely electronegative fluorine to have a more ionic character.[47] In fact, ununseptium monofluoride should feature the strongest bonding of all group 17 element monofluorides.[47]
The VSEPR theory predicts a bent-T-shaped molecular geometry for the group 17 trifluorides. All known halogen trifluorides have this molecular geometry, having a structure of AX
3E
2 (a central atom, denoted A, surrounded by three ligands, X, and two unshared electron pairs, E). If relativistic effects are not taken into account, UusF
3 should also follow its lighter congeners in having bent-T-shaped molecular geometry. However, since the 7s shell of ununseptium should not participate at all in chemical reactions, VSEPR theory instead predicts a trigonal pyramidal molecular geometry (AX
3E
1). More sophisticated predictions show that this molecular geometry would not be energetically favored for UusF
3, predicting instead a trigonal planar molecular geometry (AX
3E
0) up. This shows that VSEPR theory may not hold consistently for the superheavy elements.[46] Also, this molecule is stabilized very significantly by the SO interactions; a possible rationale may be again the large difference in electronegativity between ununseptium and fluorine, giving the bond a partially ionic character.[46]
See also
Notes
- ^ The term "group 17" refers to the group, or vertical column, in the periodic table that starts with fluorine. It is distinct from the term "halogen", which refers exclusively to the elements fluorine, chlorine, bromine, iodine, and astatine.
- ^ This research gives the total half-lives, not just the alpha-decay half-lives.
- ^ Half-lives of three of the heaviest isotopes studied in the report, 334Uus, 336Uus, and 337Uus, were predicted to exceed the age of the Earth, which means they could have survived until the present day if they were ever created; three more isotopes are expected to have half-lives of over 80 million years,[29] the half-life of 244Pu[11] (the least stable primordial nuclide), which means that, if ever created in sufficient quantity, they could have survived until the present day.
- ^ The quantum number corresponds to the letter in the electron orbital name: 0 to s, 1 to p, 2 to d, etc. See azimuthal quantum number for more information.
References
- ^ Ritter, Malcolm (June 9, 2016). "Periodic table elements named for Moscow, Japan, Tennessee". Associated Press. Retrieved December 19, 2017.
- ^ a b Fricke, Burkhard (1975). "Superheavy elements: a prediction of their chemical and physical properties". Recent Impact of Physics on Inorganic Chemistry. Structure and Bonding. 21: 89–144. doi:10.1007/BFb0116498. ISBN 978-3-540-07109-9. Retrieved 4 October 2013.
- ^ a b c d e f g h i j k l m n o p q r Hoffman, Darleane C.; Lee, Diana M.; Pershina, Valeria (2006). "Transactinides and the future elements". In Morss; Edelstein, Norman M.; Fuger, Jean (eds.). The Chemistry of the Actinide and Transactinide Elements (3rd ed.). Dordrecht, The Netherlands: Springer Science+Business Media. ISBN 978-1-4020-3555-5.
- ^ a b c d Bonchev, D.; Kamenska, V. (1981). "Predicting the Properties of the 113–120 Transactinide Elements". Journal of Physical Chemistry. 85 (9): 1177–1186. doi:10.1021/j150609a021.
- ^ a b c Chang, Zhiwei; Li, Jiguang; Dong, Chenzhong (2010). "Ionization Potentials, Electron Affinities, Resonance Excitation Energies, Oscillator Strengths, And Ionic Radii of Element Uus (Z = 117) and Astatine". J. Phys. Chem. A. 2010 (114): 13388–94. Bibcode:2010JPCA..11413388C. doi:10.1021/jp107411s.
- ^ a b Kondev, F. G.; Wang, M.; Huang, W. J.; Naimi, S.; Audi, G. (2021). "The NUBASE2020 evaluation of nuclear properties" (PDF). Chinese Physics C. 45 (3): 030001. doi:10.1088/1674-1137/abddae.
- ^ Khuyagbaatar, J.; Yakushev, A.; Düllmann, Ch. E.; et al. (2014). "48Ca+249Bk Fusion Reaction Leading to Element Z=117: Long-Lived α-Decaying 270Db and Discovery of 266Lr". Physical Review Letters. 112 (17): 172501. Bibcode:2014PhRvL.112q2501K. doi:10.1103/PhysRevLett.112.172501. PMID 24836239.
- ^ Oganessian, Yu. Ts.; et al. (2013). "Experimental studies of the 249Bk + 48Ca reaction including decay properties and excitation function for isotopes of element 117, and discovery of the new isotope 277Mt". Physical Review C. 87 (5): 054621. Bibcode:2013PhRvC..87e4621O. doi:10.1103/PhysRevC.87.054621.
- ^ a b c Gabage, Bill (2010). "International team discovers element 117". Oak Ridge National Laboratory. Retrieved 2012-11-29.
- ^ Oganessian, Yu. Ts.; Utyonkov, V. K.; Lobanov, Yu. V.;, Abdullin, F. Sh.; Polyakov, A. N.; Shirokovsky, I. V.; Tsyganov, Yu. S.; Mezentsev, A. N.; et al. (2002). "Results from the first 249Cf+48Ca experiment" (PDF). JINR Communication.
{{cite journal}}
: Explicit use of et al. in:|author=
(help)CS1 maint: multiple names: authors list (link) - ^ a b Audi., G; Wapstra, A. H.;, Thibault, C.; Blachot, J.; Bersillon, O. (2003). "The NUBASE evaluation of nuclear and decay properties" (PDF). Nuclear Physics A. 729 (1): 37–44, 119–120. Bibcode:2003NuPhA.729....3A. doi:10.1016/j.nuclphysa.2003.11.001.
{{cite journal}}
: CS1 maint: multiple names: authors list (link) - ^ Jepson, B. E.; Shockley, G. C. (1984). "Calcium hydroxide isotope effect in calcium isotope enrichment by ion exchange". Separation Science and Technology. 19 (2–3): 173–181.
- ^ "Ununseptium – The 117th element". RIA Novosti. 2009. Retrieved 2012-07-07.
- ^ "Universal nuclide chart". Nucleonica. Institute for Transuranium Elements. 2007–2012. Retrieved 2012-07-03. (registration required)
- ^ a b c d Oganessian, Yu. Ts.; et al. (2010). "Synthesis of a new element with atomic number Z=117". Phys. Rev. Lett. 104 (14): 1–4. doi:10.1103/PhysRevLett.104.142502.
{{cite journal}}
: Explicit use of et al. in:|author=
(help) - ^ a b Stark, Anne (2010). "International team discovers element 117". DOE/Lawrence Livermore National Laboratory. Retrieved 2012-11-29.
- ^ a b c d "Element 117: How scientists found the atom at the end of the material". Fox News. 2010. Retrieved 2012-11-08.
- ^ Greiner, Walter (2010). "Recommendations: 31st meeting, PAC for nuclear physics" (Document). PAC for Nuclear Physics. p. 6.
{{cite document}}
: Unknown parameter|url=
ignored (help) - ^ Template:Ru icon "В лабораториях ОИЯИ. Возвращение к дубнию". JINR. 2011. Retrieved 2011-11-09.
{{cite web}}
: Unknown parameter|trans_title=
ignored (|trans-title=
suggested) (help) - ^ Barber, Robert C.; Karol, Paul J.; Nakahara, Hiromichi; Vardaci, Emanuele; Vogt, Erich W. (2011). "Discovery of the elements with atomic numbers greater than or equal to 113 (IUPAC Technical Report)". Pure and Applied Chemistry. 83 (7): 1485–1498. doi:10.1351/PAC-REP-10-05-01.
- ^ a b "Russian scientists confirm 117th element". RIA Novosti. 2012. Retrieved 2012-07-05.
- ^ "Element 114 is named flerovium and element 116 is named livermorium". JINR. 2012. Retrieved 2012-11-09.
- ^ Chatt, J. (1979). "Recommendations for the naming of elements of atomic numbers greater than 100". Pure and Applied Chemistry. 51 (2): 381–384. doi:10.1351/pac197951020381.
- ^ Koppenol, W. H. (2002). "Naming of new elements (IUPAC Recommendations 2002)" (PDF). Pure and Applied Chemistry. 74 (5): 787–791. doi:10.1351/pac200274050787.
- ^ a b Template:Ru icon "Синтез нового 117-го элемента". JINR. 2010. Retrieved 2012-11-09.
{{cite web}}
: Unknown parameter|trans_title=
ignored (|trans-title=
suggested) (help) - ^ Marcillac, Pierre de; Coron, Noël; Dambier, Gérard; Leblanc, Jacques; Moalic, Jean-Pierre (2003). "Experimental detection of α-particles from the radioactive decay of natural bismuth". Nature. 422 (6934): 876–878. Bibcode:2003Natur.422..876D. doi:10.1038/nature01541. PMID 12712201.
- ^ Kulik, Glenn D. (2002). Van Nostrand's scientific encyclopedia (9 ed.). Wiley-Interscience. ISBN 978-0-471-33230-5. OCLC 223349096.
{{cite book}}
:|first2=
missing|last2=
(help); More than one of|last1=
and|last=
specified (help) - ^ Chowdhury, Roy P.; Samanta, C.; Basu, D. N. (2008). "Search for long lived heaviest nuclei beyond the valley of stability". Physical Reviews C. 77 (4): 044603. arXiv:0802.3837. Bibcode:2008PhRvC..77d4603C. doi:10.1103/PhysRevC.77.044603.
- ^ a b c Duarte, S. B.; et al. (2004). Half-life prediction for decay modes for superheavy nuclei (Report). Centro Brasiliero de Pesquisas Físicas. ISSN 0029-3865.
{{cite report}}
: Explicit use of et al. in:|author=
(help) - ^ Thayer 2010, pp. 63–64.
- ^ a b c d Attention: This template ({{cite doi}}) is deprecated. To cite the publication identified by doi:10.1063/1.1385366, please use {{cite journal}} (if it was published in a bona fide academic journal, otherwise {{cite report}} with
|doi=10.1063/1.1385366
instead. - ^ Thayer 2010, pp. 63–67.
- ^ Thayer 2010, p. 79.
- ^ a b Thayer 2010, p. 64.
- ^ Attention: This template ({{cite doi}}) is deprecated. To cite the publication identified by doi:10.1002/chem.200800987, please use {{cite journal}} (if it was published in a bona fide academic journal, otherwise {{cite report}} with
|doi=10.1002/chem.200800987
instead. - ^ a b Sharma, B. K. (2001). Nuclear and radiation chemistry (7th ed.). Krishna Prakashan Media. p. 147. ISBN 978-81-85842-63-9. Retrieved 2012-11-09.
- ^ a b Seaborg, G. T. (1994). Modern alchemy. World Scientific. p. 172. ISBN 981-02-1440-5.
- ^ Takahashi, N. (2002). "Boiling points of the superheavy elements 117 and 118". Journal of Radioanalytical and Nuclear Chemistry. 251 (2): 299–301. doi:10.1023/A:1014880730282.
- ^ Luig, Heribert; Keller, Comelius; Wolf, Walter; Shani, Jashovam; Miska, Horst; Zyball, Alfred; Gervé, Andreas; Balaban, Alexandru T.; Kellerer, Albrecht M.; Griebel, Jüfgen (2005). "Radionuclides". In Ullmann, Franz (ed.). Encyclopedia of industrial chemistry. Wiley-VCH. p. 23. doi:10.1002/14356007.a22_499. ISBN 978-3-527-30673-2.
- ^ Punter, Jacqui; Johnson, Robert; Langfield, Steve (2006). The essentials of GCSE OCR Additional science for specification B. Letts and Lonsdale. p. 36. ISBN 978-1-905129-73-7.
- ^ Wiberg, Egon; Wiberg, Nils; Holleman, Arnold Frederick (2001). Inorganic chemistry. Academic Press. p. 423. ISBN 978-0-12-352651-9.
- ^ Otozai, K.; Takahashi, N. (1982). "Estimation chemical form boiling point elementary astatine by radio gas chromatography". Radiochimica Acta. 31 (3‒4): 201‒203.
- ^ Bader, Richard F. W. "An introduction to the electronic structure of atoms and molecules". McMaster University. Retrieved 2008-01-18.
- ^ a b Pershina 2010, p. 504.
- ^ Thayer 2010, p. 84.
- ^ a b c Attention: This template ({{cite doi}}) is deprecated. To cite the publication identified by doi:10.1021/jp026531m, please use {{cite journal}} (if it was published in a bona fide academic journal, otherwise {{cite report}} with
|doi=10.1021/jp026531m
instead. - ^ a b c d e Han, Y. K.; Bae, C.; Son, S. K.; Lee, Y. S. (2000). "Spin-orbit effects on the transactinide p-block element monohydrides MH (M=element 113-118)". Journal of Chemical Physics. 112 (6): 2684–2691. Bibcode:2000JChPh.112.2684H. doi:10.1063/1.480842.
{{cite journal}}
: CS1 maint: multiple names: authors list (link) - ^ Stysziński 2010, pp. 144–146.
- ^ Stysziński 2010, pp. 139–146.
Bibliography
- Barysz, M.; Ishikawa, Y., ed. (2010). Relativisic methods for chemists. Springer Science+Business Media. ISBN 978-1-4020-9974-8.
{{cite book}}
: CS1 maint: multiple names: editors list (link)
- Thayer, John S. (2010). Chemistry of heavier main group elements. doi:10.1007/9781402099755_2.
{{cite book}}
: Invalid|ref=harv
(help) - Stysziński, Jacek (2010). Why do we need relativistic computational methods?. doi:10.1007/9781402099755_3.
{{cite book}}
: Invalid|ref=harv
(help) - Pershina, V. (2010). Electronic structure and chemistry of the heaviest elements. doi:10.1007/9781402099755_11.
{{cite book}}
: Invalid|ref=harv
(help)
- Thayer, John S. (2010). Chemistry of heavier main group elements. doi:10.1007/9781402099755_2.