Acetoacetate Decarboxylase: Difference between revisions
No edit summary |
No edit summary |
||
Line 29: | Line 29: | ||
== History == |
== History == |
||
Acetoacetate decarboxylase is an enzyme with major historical implications, specifically in [[World War I]] and in establishing the state of [[Israel]]. <ref>{{cite journal|author=Bormon, S |title=New Structure Revisits History|journal=Structural Biology|volume=87|issue=21|pages=9|year=2009}}</ref> During the war the [[Allies]] needed pure acetone as a solvent for [[nitro-cellulose]], a highly flammable compound that is the main component in gunpowder. <ref>[http://www.britannica.com/EBchecked/topic/416152/nitrocellulose]</ref> In 1916, biochemist and future first president of Israel [[Chaim Weizmann]] was the first to isolate ''Clostridium acetobutylicum'', a [[Gram-positive]], anaerobic bacteria in which acetoacetate decarboxylase is found. Weizmann was able to harness the organism’s ability to yield acetone from starch in order to mass-produce explosives during the war. <ref |
Acetoacetate decarboxylase is an enzyme with major historical implications, specifically in [[World War I]] and in establishing the state of [[Israel]]. <ref name=Bormon>{{cite journal|author=Bormon, S |title=New Structure Revisits History|journal=Structural Biology|volume=87|issue=21|pages=9|year=2009}}</ref> During the war the [[Allies]] needed pure acetone as a solvent for [[nitro-cellulose]], a highly flammable compound that is the main component in gunpowder. <ref>[http://www.britannica.com/EBchecked/topic/416152/nitrocellulose]</ref> In 1916, biochemist and future first president of Israel [[Chaim Weizmann]] was the first to isolate ''Clostridium acetobutylicum'', a [[Gram-positive]], anaerobic bacteria in which acetoacetate decarboxylase is found. Weizmann was able to harness the organism’s ability to yield acetone from starch in order to mass-produce explosives during the war. <ref name=Bormon /> This led the American and British governments to install the process devised by Chaim Weizmann in several large plants in England, France, Canada, and the United States. Through Weizmann’s scientific contributions in World War I, he became close with influential British leaders educating them of his Zionist beliefs. <ref name=Jewish Virtual Library>{{cite web|title=Jewish Virtual Library|url=https://www.jewishvirtuallibrary.org/jsource/biography/weizmann.html}}</ref>One of them was Arthur Balfour, the man after whom the [[Balfour Declaration]]—the first document pronouncing British support in the establishment of a Jewish homeland—was named after.<ref name=Jewish Virtual Library /> |
||
The production of acetone by acetoacetate decarboxylase-containing or clostridial bacteria was utilized in large-scale industrial syntheses in the first half of the twentieth century. In the 1960s, the industry replaced this process with less expensive, more efficient chemical syntheses of acetone from petroleum and petroleum derivatives. |
The production of acetone by acetoacetate decarboxylase-containing or clostridial bacteria was utilized in large-scale industrial syntheses in the first half of the twentieth century. In the 1960s, the industry replaced this process with less expensive, more efficient chemical syntheses of acetone from petroleum and petroleum derivatives. <ref>[http://www.sbi.uni-rostock.de/uploads/tx_templavoila/SBI_Courses_Abe-fermentation.pdf]</ref> However, there has been a growing interest in acetone production that is more environmentally friendly, causing a resurgence in utilizing acetoacetate decarboxylase-containing bacteria. <ref>{{cite journal|last=Collas|first=Florent|coauthors=Wouter Kuit, Benjamin Clement, Remy Marchal, Ana M Lopez-Contreras, Frederic Monot|title=Simultaneous production of isoproponal, butanol, ethanol, and 2,3-butanediol by Clostridium acetobutylicum ATCC 824 engineered strains|journal=AMB Express|date=August 21 2012|volume=2|issue=1|pages=45|doi=10.1186/2191-0855-2-45|url=http://www.ncbi.nlm.nih.gov/pubmed/22909015}}</ref> Similarly, isopropanol and butanol fermentation using clostridial species is also becoming popular. |
||
==Structure== |
==Structure== |
||
Line 56: | Line 56: | ||
==Inactivation and Inhibition== |
==Inactivation and Inhibition== |
||
Acetoacetate decarboxylase is inhibited by a number of compounds. [[Acetic anhydride]] |
Acetoacetate decarboxylase is inhibited by a number of compounds. [[Acetic anhydride]] performs a nucleophilic attack on the critical catalytic residue, Lys115, of acetoacetate decarboxylase to inactivate the enzyme. <ref>{{cite journal|last=O'Leary|first=M.H.|coauthors=F.H. Westheimer|title=Acetoacetate decarboxylase. Selective acetylation of enzyme|journal=Biochemistry|date=1968|volume=7|issue=3|pages=913-919|url=http://pubs.acs.org/doi/abs/10.1021/bi00843a005}}</ref> The rate of inactivation was assessed through the [[hydrolysis]] of the synthetic substrate 2,4-dinitrophenyl propionate to dinitrophenol by acetoacetate decarboxylase. In the presence of acetic anhydride, the enzyme is inactivated, unable to catalyze the hydrolysis 2,4-dinitrophenyl propionate to dinitrophenol. <ref>{{cite journal|last=Schmidt|first=Donald E.|coauthors=F.H. Westheimer|title=pK of the Lysine Amino Group at the Active Site of Acetoacetate Decarboxylase|journal=Biochemistry|date=1971|volume=10|issue=7|pages=1249-1253|url=http://pubs.acs.org/doi/pdf/10.1021/bi00783a023}}</ref> |
||
Acetonylsulfonate acts as a [[competitive inhibitor]] (K<sub>I</sub>=8.0 mM ) as it mimics the characteristics of the natural substrate, acetoacetate (K<sub>M</sub>=8.0 mM). <ref name=Autor>{{cite journal|last=Autor|first=Anne P.|coauthors=I. Fridovich|title=The Interactions of Acetoacetate Decarboxylase with Carbonyl Compounds, Hydrogen Cyanide, and an Organic Mercurial|journal=J. Biol. Chem.|date=1970|volume=245|pages=5214-5222|url=http://www.jbc.org/content/245/20/5214.full.pdf+html}}</ref> The monoanion version of acetonylphosphonate is also a good inhibitor (K<sub>I</sub>=0.8mM), more efficient than the acetonylphosphonate monoester or dianion. <ref>{{cite journal|last=Kluger|first=Ronald|coauthors=Kurt Nakaoka|title=Inhibition of Acetoacetate Decarboxylase by Ketophosphonates. Structural and Dyanmic Probes of the Active Site|journal=Biochemistry|date=1974|volume=13|issue=5|pages=910-914|url=http://pubs.acs.org/doi/pdf/10.1021/bi00702a013}}</ref> These findings indicate that active site is very discriminatory and sterically restricted. |
Acetonylsulfonate acts as a [[competitive inhibitor]] (K<sub>I</sub>=8.0 mM ) as it mimics the characteristics of the natural substrate, acetoacetate (K<sub>M</sub>=8.0 mM). <ref name=Autor>{{cite journal|last=Autor|first=Anne P.|coauthors=I. Fridovich|title=The Interactions of Acetoacetate Decarboxylase with Carbonyl Compounds, Hydrogen Cyanide, and an Organic Mercurial|journal=J. Biol. Chem.|date=1970|volume=245|pages=5214-5222|url=http://www.jbc.org/content/245/20/5214.full.pdf+html}}</ref> The monoanion version of acetonylphosphonate is also a good inhibitor (K<sub>I</sub>=0.8mM), more efficient than the acetonylphosphonate monoester or dianion. <ref>{{cite journal|last=Kluger|first=Ronald|coauthors=Kurt Nakaoka|title=Inhibition of Acetoacetate Decarboxylase by Ketophosphonates. Structural and Dyanmic Probes of the Active Site|journal=Biochemistry|date=1974|volume=13|issue=5|pages=910-914|url=http://pubs.acs.org/doi/pdf/10.1021/bi00702a013}}</ref> These findings indicate that active site is very discriminatory and sterically restricted. |
Revision as of 19:10, 26 May 2014
Acetoacetate decarboxylase | |||||||||
---|---|---|---|---|---|---|---|---|---|
![]() Acetoacetate decarboxylase dodecamer structure with bound 2-Pentanone bound in its active sites. | |||||||||
Identifiers | |||||||||
EC no. | 4.1.1.4 | ||||||||
CAS no. | 9025-03-0 | ||||||||
Databases | |||||||||
IntEnz | IntEnz view | ||||||||
BRENDA | BRENDA entry | ||||||||
ExPASy | NiceZyme view | ||||||||
KEGG | KEGG entry | ||||||||
MetaCyc | metabolic pathway | ||||||||
PRIAM | profile | ||||||||
PDB structures | RCSB PDB PDBe PDBsum | ||||||||
Gene Ontology | AmiGO / QuickGO | ||||||||
|
Acetoacetate decarboxylase (AAD or ADC) is an enzyme involved in both the ketone body production pathway in humans and other mammals, and solventogenesis in bacteria. Acetoacetate decarboxylase plays a key role in solvent production by catalyzing the decarboxylation of acetoacetate, yielding acetone and carbon dioxide. [1] This enzyme has been of particular interest because it is a classic example of how pKa values of ionizable groups in the enzyme active site can be significantly perturbed. Specifically, the pKa value of lysine 115 in the active site is unusually low, allowing for the formation of a Schiff base intermediate and ultimate production of acetone and carbon dioxide. [2]
acetoacetic acid | Acetoacetate decarboxylase | acetone | |
![]() |
![]() | ||
CO2 | |||
![]() | |||
History
Acetoacetate decarboxylase is an enzyme with major historical implications, specifically in World War I and in establishing the state of Israel. [3] During the war the Allies needed pure acetone as a solvent for nitro-cellulose, a highly flammable compound that is the main component in gunpowder. [4] In 1916, biochemist and future first president of Israel Chaim Weizmann was the first to isolate Clostridium acetobutylicum, a Gram-positive, anaerobic bacteria in which acetoacetate decarboxylase is found. Weizmann was able to harness the organism’s ability to yield acetone from starch in order to mass-produce explosives during the war. [3] This led the American and British governments to install the process devised by Chaim Weizmann in several large plants in England, France, Canada, and the United States. Through Weizmann’s scientific contributions in World War I, he became close with influential British leaders educating them of his Zionist beliefs. Cite error: The <ref>
tag has too many names (see the help page).One of them was Arthur Balfour, the man after whom the Balfour Declaration—the first document pronouncing British support in the establishment of a Jewish homeland—was named after.Cite error: The <ref>
tag has too many names (see the help page).
The production of acetone by acetoacetate decarboxylase-containing or clostridial bacteria was utilized in large-scale industrial syntheses in the first half of the twentieth century. In the 1960s, the industry replaced this process with less expensive, more efficient chemical syntheses of acetone from petroleum and petroleum derivatives. [5] However, there has been a growing interest in acetone production that is more environmentally friendly, causing a resurgence in utilizing acetoacetate decarboxylase-containing bacteria. [6] Similarly, isopropanol and butanol fermentation using clostridial species is also becoming popular.
Structure
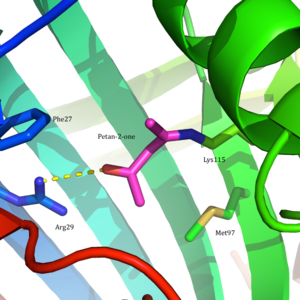
Acetoacetate decarboxylase is a 365 kDa complex with a homododecameric structure. The overall structure consists of antiparallel β-sheets and a central seven-stranded cone-shaped β-barrel. The core of this β-barrel surrounds the active site in each protomer of the enzyme. The active site, containing Phe26, Leu71, Tyr74, Met96, Leu98, Tyr113, and Leu223, is mostly hydrophobic. The only charged residues in the active site are Arg29 and Glu76. Arg29 is thought to play a role in substrate binding, while Glu76 is thought to play a role in the orienting the active site for catalysis. The overall hydrophobic environment of the active site plays a critical role in destabilizing the positively charged protonated amine form of Lys115, a key residue involved in the formation of a Schiff base intermediate, thereby perturbing its pKa downward. Additionally, Lys115 is does not form any hydrogen-bonding interactions in the active site, further destabilizing the protonated amine. Lys115 is in close proximity to the aromatic ring of Tyr113. However, Tyr113 is oriented in a way that prevents cation-pi interactions with Lys115, making stabilization of the ammonium group of Lys115 unlikely. Furthermore, another important lysine residue, Lys116, is thought to play an important role in the positioning of Lys115 in the active site. Through hydrogen bonding interactions with Ser16 and Met210, Lys116 orients Lys115 into a hydrophobic pocket, thereby positioning Lys115 in the active site to favor the neutral amine form. [7]
Reaction Mechanism

Acetoacetate decarboxylase from Clostridium acetobutylicum catalyzes the decarboxylation of acetoacetate to yield acetone and carbon dioxide (Figure 1). The reaction mechanism is believed to proceed via the formation of a Schiff base intermediate with lysine 115 in the active site. The first line of support for this mechanism came from a radiolabeling experiment by Hamilton et al. Researchers labeled the carbonyl group of acetoacetate with 18O and examined the oxygen exchange of the decarboxylation step of the proposed Schiff base mechanism. Results from this experiment showed that exchange of 18O from the carbonyl group of acetoacetate to water used as the solvent is a necessary part of the decarboxylation step, providing support for the mechanism that proceeds through Schiff base intermediate between the ketoacid and an amino acid residue on the enzyme. [8]
Further research led to the isolation of the active site peptide sequence and identification of the active site lysine, Lys115, that is involved in the formation of the Schiff base intermediate. [9]. Additionally, maximum activity of the enzyme was found to occur at pH 5.95, suggesting that the pKa of the ε-ammonium group of Lys115 is significantly perturbed in the active site. [2] If the pKa were not perturbed downward, the lysine residue would remain protonated as an ammonium cation, making it unreactive for the nucleophilic addition necessary to form the Schiff base.

Building upon this finding, Westheimer et al. directly measured the pKa of Lys115 in the active site using 5-nitrosalicylaldehyde (5-NSA) . Reaction of 5-NSA with acetoacetate decarboxylase and subsequent reduction of the resulting Schiff base with sodium borohydride led to the incorporation of a 2-hydroxy-5-nitrobenzylamino reporter molecule in the active site (Figure 2). Titration of the enzyme with this attached reporter group revealed that the pKa of Lys115 is decreased to 5.9 in the active site. [11]These results were the basis for the proposal that the perturbation in the pKa of Lys115 was due to its proximity to the positively charged ε-ammonium group of Lys116 in the active site. [2] A nearby positive charge could cause unfavorable electrostatic repulsions that weaken the N-H bond of Lys115. Westheimer et al.'s proposal was further supported by site-directed mutagenesis studies. When Lys116 was mutated to cysteine or asparagine, the pKa of Lys115 was found to be significantly elevated to over 9.2, indicating that positively charged Lys116 plays a critical role in determining the pKa of Lys115. [2] Although a crystal structure was not yet solved at the time of this proposal to show that Lys116 can interact with Lys115, this proposal was widely accepted and cited as a textbook example of how the active site can be precisely organized to perturb a pKa and affect reactivity. [7]
In 2009, Ho et al. used x-ray crystallography data to evaluate Westheimer et al.’s proposal. From the crystal structures, Ho et al. found that Lys 115 and Lys 116 are oriented in opposite directions and separated by 14.8 Å (Figure 3). [7] This distance makes it unlikely that Lys116 is able to affect the pKa of Lys115. Instead, through hydrogen-bonding interactions with Ser16 and Met210, Lys116 likely anchors Lys115 into position in a hydrophobic pocket. This positioning into a hydrophobic pocket destabilizes the protonated ammonium cation of Lys115, suggesting that the perturbation of Lys115's pKa occurs through a desolvation effect.

Inactivation and Inhibition
Acetoacetate decarboxylase is inhibited by a number of compounds. Acetic anhydride performs a nucleophilic attack on the critical catalytic residue, Lys115, of acetoacetate decarboxylase to inactivate the enzyme. [12] The rate of inactivation was assessed through the hydrolysis of the synthetic substrate 2,4-dinitrophenyl propionate to dinitrophenol by acetoacetate decarboxylase. In the presence of acetic anhydride, the enzyme is inactivated, unable to catalyze the hydrolysis 2,4-dinitrophenyl propionate to dinitrophenol. [13]
Acetonylsulfonate acts as a competitive inhibitor (KI=8.0 mM ) as it mimics the characteristics of the natural substrate, acetoacetate (KM=8.0 mM). [14] The monoanion version of acetonylphosphonate is also a good inhibitor (KI=0.8mM), more efficient than the acetonylphosphonate monoester or dianion. [15] These findings indicate that active site is very discriminatory and sterically restricted.
Hydrogen cyanide seems to be an uncompetitive inhibitor, combining with Schiff’s base compounds formed at the active site. [14] Addition of carbonyl compounds to the enzyme, in the presence of hydrogen cyanide, increases hydrogen cyanide’s ability to inhibit acetoacetate decarboxylase suggesting that carbonyl compounds readily form Schiff’s bases at the active site. [14] Hydrogen cyanide is most potent as an inhibitor at pH 6, the optimum pH for the enzyme, suggesting that the rate-limiting step of catalysis is the formation of the Schiff base intermediate. [14]
Beta-diketones appear to inhibit acetoacetate decarboxylase well but slowly. Acetoacetate decarboxylase has a KM for acetoacetate of 7x10-3 M whereas the enzyme has a KI for benzoylacetone of 1.9x10-6 M. [14] An enamine is most likely formed upon interaction of beta-diketones with free enzyme.
The reaction of acetoacetate decarboxylase with p-chloromercuriphenylsulfonate (CMS) results in decreased catalytic activity upon two equivalents of CMS per enzyme subunit. [14] CMS interacts with two sulfhydryl groups located on each enzyme subunit. Further inactivation occurs upon addition of a third equivalent of CMS per subunit. Addition of free cysteine to the inhibited enzyme is able to reverse CMS inhibition of acetoacetate decarboxylase. [14]
Activity in bacteria
Acetoacetate decarboxylase has been found and studied in the following bacteria in addition to Clostridium acetobutylicum:
- Bacillus polymyxa
- Chromobacterium violaceum
- Clostridium beijerinckii
- Clostridium cellulolyticum
- Pseudomonas putida
Activity in humans and mammals
While this enzyme has not been purified from human tissue, the activity was shown to be present in human blood serum.[16][17]
In humans and other mammals, the conversion of acetoacetate into acetone and carbon dioxide by acetoacetate decarboxylase is a final irreversible step in the ketone-body pathway that supplies the body with a secondary source of energy. In the liver, acetyl co-A formed from fats and lipids are transformed into three ketone bodies: acetone, acetoacetate, and D-β-hydroxybutyrate. Acetoacetate and D-β-hydroxybutyrate are exported to non-hepatic tissues, where they are converted back into acetyl-coA and used for fuel. Acetone and carbon dioxide on the other hand are exhaled, and not allowed to accumulate under normal conditions.[18]
Acetoacetate and D-β-hydroxybutyrate freely interconvert through the action of D-β-hydroxybutyrate dehydrogenase.[18] Subsequently, one function of acetoacetate decarboxylase may be to regulate the concentrations of the other, two 4-carbon ketone bodies.
Clinical significance
Ketone body production increases significantly when the rate of glucose metabolism is insufficient in meeting the body's energy needs. Such conditions include high-fat ketogenic diets, diabetic ketoacidosis, or severe starvation.[19]
Under elevated levels of acetoacetate and D-β-hydroxybutyrate, acetoacetate decarboxylase produces significantly more acetone. Acetone is toxic, and can accumulate in the body under these conditions.[18] Elevated levels of acetone in the human breath can be used to diagnose diabetes.[19]
References
- ^ Peterson DJ, Bennett GN (1990). "Purification of acetoacetate decarboxylase from Clostridium acetobutylicum ATCC cloning of the acetoacetate decarboxylase in Escherichia coli". Applied and Environmental Microbiology. 56 (11): 3491–3498.
- ^ a b c d e Highbarger, LA (January 9, 1996). "Mechanism of the reaction catalyzed by acetoacetate decarboxylase. Importance of lysine 116 in determining the pKa of active site lysine 115". Biochemistry. 9 (35): 41–46. PMID 8555196.
{{cite journal}}
:|access-date=
requires|url=
(help); Unknown parameter|coauthors=
ignored (|author=
suggested) (help) - ^ a b Bormon, S (2009). "New Structure Revisits History". Structural Biology. 87 (21): 9.
- ^ [1]
- ^ [2]
- ^ Collas, Florent (August 21 2012). "Simultaneous production of isoproponal, butanol, ethanol, and 2,3-butanediol by Clostridium acetobutylicum ATCC 824 engineered strains". AMB Express. 2 (1): 45. doi:10.1186/2191-0855-2-45.
{{cite journal}}
: Check date values in:|date=
(help); Unknown parameter|coauthors=
ignored (|author=
suggested) (help)CS1 maint: unflagged free DOI (link) - ^ a b c d e Ho, Meng-Chiao (May 21 2009). "The origin of the electrostatic pertubation in acetoacetate decarboxylase". Nature. 459: 393–397. doi:10.1038/nature07938.
{{cite journal}}
:|access-date=
requires|url=
(help); Check date values in:|date=
(help); Unknown parameter|coauthors=
ignored (|author=
suggested) (help) - ^ Hamilton GA, Westheimer FH (1959). "On the Mechanism of Enzymatic Decarboxylation of Acetoacetate". J. Am. Chem. Soc. 81 (23): 6332–6333. doi:10.1021/ja01532a058.
- ^ Peterson DJ, Cary JW, Vanderleyden J, Bennett GN (1993). "Sequence and arrangement of genes encoding enzymes of the acetone-production pathway of Clostridium acetobutylicum". Gene. 123 (1): 93–97. PMID 8423010.
{{cite journal}}
: CS1 maint: multiple names: authors list (link) - ^ "A History of Acetoacetate Decarboxylase". JinKai.org. Retrieved 26 May 2014.
- ^ Kokesh, Fritz C. (December 29, 1971). "A reporter group at the active site of acetoacetate decarboxylase. Ionization constant of the amino group". Journal of the American Chemical Society. 93 (26): 7270–7274. doi:10.1021/ja00755a025.
{{cite journal}}
:|access-date=
requires|url=
(help); Unknown parameter|coauthors=
ignored (|author=
suggested) (help) - ^ O'Leary, M.H. (1968). "Acetoacetate decarboxylase. Selective acetylation of enzyme". Biochemistry. 7 (3): 913–919.
{{cite journal}}
: Unknown parameter|coauthors=
ignored (|author=
suggested) (help) - ^ Schmidt, Donald E. (1971). "pK of the Lysine Amino Group at the Active Site of Acetoacetate Decarboxylase". Biochemistry. 10 (7): 1249–1253.
{{cite journal}}
: Unknown parameter|coauthors=
ignored (|author=
suggested) (help) - ^ a b c d e f g Autor, Anne P. (1970). "The Interactions of Acetoacetate Decarboxylase with Carbonyl Compounds, Hydrogen Cyanide, and an Organic Mercurial". J. Biol. Chem. 245: 5214–5222.
{{cite journal}}
: Unknown parameter|coauthors=
ignored (|author=
suggested) (help) - ^ Kluger, Ronald (1974). "Inhibition of Acetoacetate Decarboxylase by Ketophosphonates. Structural and Dyanmic Probes of the Active Site". Biochemistry. 13 (5): 910–914.
{{cite journal}}
: Unknown parameter|coauthors=
ignored (|author=
suggested) (help) - ^ van Stekelenburg GJ, Koorevaar G (June 1972). "Evidence for the existence of mammalian acetoacetate decarboxylase: with special reference to human blood serum". Clin. Chim. Acta. 39 (1): 191–9. doi:10.1016/0009-8981(72)90316-6. PMID 4624981.
- ^ Koorevaar G, Van Stekelenburg GJ (September 1976). "Mammalian acetoacetate decarboxylase activity. Its distribution in subfractions of human albumin and occurrence in various tissues of the rat". Clin. Chim. Acta. 71 (2): 173–83. doi:10.1016/0009-8981(76)90528-3. PMID 963888.
- ^ a b c Cite error: The named reference
Nelson
was invoked but never defined (see the help page). - ^ a b Galassetti PR, Novak B, Nemet D, Rose-Gottron C, Cooper DM, Meinardi S, Newcomb R, Zaldivar F, Blake DR (2005). "Breath ethanol and acetone as indicators of serum glucose levels: an initial report". Diabetes Technol. Ther. 7 (1): 115–23. doi:10.1089/dia.2005.7.115. PMID 15738709.
{{cite journal}}
: CS1 maint: multiple names: authors list (link)
External links
- acetoacetate+decarboxylase at the U.S. National Library of Medicine Medical Subject Headings (MeSH)
- EC 4.1.1.4
- Brenda: Entry of Acetoacetate decarboxylase
- KEGG: Entry of Acetoacetate decarboxylase
- InterPro: IPR010451 Acetoacetate decarboxylase