Photovoltaics: Difference between revisions
Jasperbell (talk | contribs) |
|||
Line 20: | Line 20: | ||
Cells require protection from the environment and are packaged usually behind a glass sheet. When more power is required than a single cell can deliver, cells are electrically connected together to form [[photovoltaic module]]s, or [[solar panel]]s, A single module is enough to power an emergency telephone, but for a house or a power plant the modules must be arranged in arrays. Although the selling price of modules is still too high to compete with grid electricity in most places, significant financial incentives in Japan and then Germany triggered a huge growth in demand, followed quickly by production. Although module prices rose and plateaued<ref>[http://www.solarbuzz.com/Moduleprices.htm Solar Module Price Environment]</ref>, it is expected that costs and prices will fall to 'grid parity' in many places around 2010. |
Cells require protection from the environment and are packaged usually behind a glass sheet. When more power is required than a single cell can deliver, cells are electrically connected together to form [[photovoltaic module]]s, or [[solar panel]]s, A single module is enough to power an emergency telephone, but for a house or a power plant the modules must be arranged in arrays. Although the selling price of modules is still too high to compete with grid electricity in most places, significant financial incentives in Japan and then Germany triggered a huge growth in demand, followed quickly by production. Although module prices rose and plateaued<ref>[http://www.solarbuzz.com/Moduleprices.htm Solar Module Price Environment]</ref>, it is expected that costs and prices will fall to 'grid parity' in many places around 2010. |
||
Many [[corporation]]s and [[institution]]s are currently developing ways to increase the practicality of solar power. While [[private company|private companies]] conduct much of the research and development on solar energy, [[college]]s and [[university|universities]] and institutes also work on solar-powered devices. Most research is being carried out in Germany, Japan, USA and [[Photovoltaic and renewable energy engineering in Australia|Australia]]. |
Many [[corporation]]s and [[institution]]s are currently developing ways to increase the practicality of solar power. While [[private company|private companies]] conduct much of the research and development on solar energy, [[college]]s and [[university|universities]] and institutes also work on solar-powered devices. Most research is being carried out in Germany, Japan, USA and [[Photovoltaic and renewable energy engineering in Australia|Australia]]. Many experts say the amount of money being spent on basic research in solar technologies is far too little to surmount big hurdles to making it much cheaper. [http://www.nytimes.com/2007/07/16/business/16solar.html] |
||
The most important issue with solar panels is [[capital cost]] (installation and materials). Because of much increased [[demand]], the price of silicon has risen and shortages occurred in 2005 and 2006. Newer alternatives to standard crystalline silicon modules including casting wafers instead of sawing <ref>[http://www.evergreensolar.com/app/en/technology/ A Better Way to Make Solar Power]</ref>, thin film (CdTe<ref>[http://www.firstsolar.com/company_overview.php Company Information Overview]</ref>, CIGS<ref>[http://www.wuerth-solar.de/website/frames.php?parLANG=EN&parKAT=239 The technology at a glance]</ref>, amorphous Si<ref>[http://www.uni-solar.com/interior.asp?id=66 Converting sunlight to electricity]</ref>, microcrystalline Si), concentrator modules, [[Andrew Blakers|'Sliver' cells]], and continuous printing processes. Due to [[Returns to scale|economies of scale]] solar panels get less costly as people use and buy more — as manufacturers increase production to meet demand, the cost and price is expected to drop in the years to come. As of early 2006, the average cost per installed watt for a residential sized system was about USD 6.50 to USD 7.50, including panels, inverters, mounts, and electrical items.<ref>[http://www.solarpowerfor.us/solar-photovoltaic-panels.html Solar Photovoltaic Panels]</ref> In 2007 investors began offering free solar panel installation in return for a 25 year contract to purchase electricity at a fixed price, normally set at or below current electric rates.[http://renu.citizenre.com/]<ref>[http://www.mmarenewableventures.com/Programs/Solar.html MMA Renewable Ventures Solar Energy Program]</ref><ref>[http://www.renewableenergyaccess.com/rea/news/story?id=49104 U.S. Retailers Save with Solar PV & Energy Efficiency]</ref> |
The most important issue with solar panels is [[capital cost]] (installation and materials). Because of much increased [[demand]], the price of silicon has risen and shortages occurred in 2005 and 2006. Newer alternatives to standard crystalline silicon modules including casting wafers instead of sawing <ref>[http://www.evergreensolar.com/app/en/technology/ A Better Way to Make Solar Power]</ref>, thin film (CdTe<ref>[http://www.firstsolar.com/company_overview.php Company Information Overview]</ref>, CIGS<ref>[http://www.wuerth-solar.de/website/frames.php?parLANG=EN&parKAT=239 The technology at a glance]</ref>, amorphous Si<ref>[http://www.uni-solar.com/interior.asp?id=66 Converting sunlight to electricity]</ref>, microcrystalline Si), concentrator modules, [[Andrew Blakers|'Sliver' cells]], and continuous printing processes. Due to [[Returns to scale|economies of scale]] solar panels get less costly as people use and buy more — as manufacturers increase production to meet demand, the cost and price is expected to drop in the years to come. As of early 2006, the average cost per installed watt for a residential sized system was about USD 6.50 to USD 7.50, including panels, inverters, mounts, and electrical items.<ref>[http://www.solarpowerfor.us/solar-photovoltaic-panels.html Solar Photovoltaic Panels]</ref> In 2007 investors began offering free solar panel installation in return for a 25 year contract to purchase electricity at a fixed price, normally set at or below current electric rates.[http://renu.citizenre.com/]<ref>[http://www.mmarenewableventures.com/Programs/Solar.html MMA Renewable Ventures Solar Energy Program]</ref><ref>[http://www.renewableenergyaccess.com/rea/news/story?id=49104 U.S. Retailers Save with Solar PV & Energy Efficiency]</ref> |
Revision as of 14:30, 3 August 2007


Photovoltaics, or PV for short, is a solar power technology that uses solar cells or solar photovoltaic arrays to convert light from the sun directly into electricity. Photovoltaics is also the field of study relating to this technology and there are many research institutes devoted to work on photovoltaics.[1][2] The manufacture of photovoltaic cells has expanded dramatically in recent years.[3][4][5] Total nominal 'peak power' of installed solar PV arrays was around 3,700 MW as of the end of 2005, a 42% increase for the year,[6] and most of this consisted of grid-connected applications. Such installations may be ground-mounted (and sometimes integrated with farming and grazing)[7] or building integrated.[8] Financial incentives, such as preferential feed-in tariffs for solar-generated electricity and net metering, have supported solar PV installations in many countries including Germany, Japan, and the United States.[9]
Current development



Photovoltaics, or PV for short, is a technology in which light is converted into electrical power. It is best known as a method for generating solar power by using solar cells packaged in photovoltaic modules, often electrically connected in multiples as solar photovoltaic arrays to convert energy from the sun into electricity.
Photovoltaics can refer to the field of study relating to this technology, and the term photovoltaic denotes the unbiased operating mode of a photodiode in which current through the device is entirely due to the transduced light energy. Virtually all photovoltaic devices are some type of photodiode.[citation needed]
Solar cells produce direct current electricity from light, which can be used to power equipment or to recharge a battery. The first practical application of photovoltaics was to power orbiting satellites and other spacecraft and pocket calculators, but today the majority of photovoltaic modules are used for grid connected power generation. In this case an inverter is required to convert the DC to AC. There is a smaller market for off grid power for remote dwellings, roadside emergency telephones, remote sensing, and cathodic protection of pipelines.
Cells require protection from the environment and are packaged usually behind a glass sheet. When more power is required than a single cell can deliver, cells are electrically connected together to form photovoltaic modules, or solar panels, A single module is enough to power an emergency telephone, but for a house or a power plant the modules must be arranged in arrays. Although the selling price of modules is still too high to compete with grid electricity in most places, significant financial incentives in Japan and then Germany triggered a huge growth in demand, followed quickly by production. Although module prices rose and plateaued[10], it is expected that costs and prices will fall to 'grid parity' in many places around 2010.
Many corporations and institutions are currently developing ways to increase the practicality of solar power. While private companies conduct much of the research and development on solar energy, colleges and universities and institutes also work on solar-powered devices. Most research is being carried out in Germany, Japan, USA and Australia. Many experts say the amount of money being spent on basic research in solar technologies is far too little to surmount big hurdles to making it much cheaper. [1]
The most important issue with solar panels is capital cost (installation and materials). Because of much increased demand, the price of silicon has risen and shortages occurred in 2005 and 2006. Newer alternatives to standard crystalline silicon modules including casting wafers instead of sawing [11], thin film (CdTe[12], CIGS[13], amorphous Si[14], microcrystalline Si), concentrator modules, 'Sliver' cells, and continuous printing processes. Due to economies of scale solar panels get less costly as people use and buy more — as manufacturers increase production to meet demand, the cost and price is expected to drop in the years to come. As of early 2006, the average cost per installed watt for a residential sized system was about USD 6.50 to USD 7.50, including panels, inverters, mounts, and electrical items.[15] In 2007 investors began offering free solar panel installation in return for a 25 year contract to purchase electricity at a fixed price, normally set at or below current electric rates.[2][16][17]
A less common form of the technologies is thermophotovoltaics, in which the thermal radiation from some hot body other than the sun is utilized. Photovoltaic devices are also used to produce electricity in optical wireless power transmission.
Worldwide installed photovoltaic totals
The three leading countries (Japan, Germany and the USA) represent 90% of the total worldwide PV installations, although this is subject to change with both Portugal and Australia independently announcing construction of the world's largest installations, and will continue to change as even larger facilities are constructed.
Photovoltaic power capacity is measured as maximum power output under standardized test conditions in "Wp" (watts peak).[18] (actual peak power may exceed this nominal peak power). Solar photovoltaic arrays have capacity factors of around 19%, which is lower than many other industrial sources of electricity.[19][20][21] Therefore the 2005 installed base peak output would have provided an average output of something like 703 MW (19% × 3,700). This represented 0.04 percent of global demand at the time.[22]
Germany was the fastest growing major PV market in the world in 2005. In 2005, 837 MWp of PV were installed. The German PV industry generates over 10,000 jobs in production, distribution and installation. Over 90% of solar PV installations are in grid-tied applications in Germany. The balance is off-grid (or stand alone) systems.[23]
Country | PV Capacity | ||||
---|---|---|---|---|---|
Cumulative | Installed in 2005 | ||||
Off Grid PV [kW] | On Grid [kW] | Total [kW] | Total [kW] | Grid-tied [kW] | |
Germany | 29,000 | 1,400,000 | 1,429,000 | 635,000 | 632,000 |
Japan | 87,057 | 1,334,851 | 1,421,908 | 289,917 | 287,105 |
United States | 233,000 | 246,000 | 479,000 | 103,000 | 70,000 |
Australia | 41,841 | 8,740 | 60,581 | 8,280 | 1,980 |
Spain | 15,800 | 41,600 | 57,400 | 20,400 | 18,600 |
Netherlands | 4,919 | 45,857 | 50,776 | 1,697 | 1,547 |
Italy | 12,300 | 15,200 | 37,500* | 6,800 | 6,500 |
France | 20,076 | 12,967 | 33,043 | 7,020 | 5,900 |
Switzerland | 3,250 | 23,800 | 27,050 | 3,950 | 3,800 |
Austria | 2,895 | 21,126 | 24,021 | 2,961 | 2,711 |
Mexico | 18,654 | 40 | 18,694 | 513 | 30 |
Canada | 15,622 | 1,124 | 16,746 | 2,862 | 612 |
South Korea | 5,663 | 9,358 | 15,021 | 6,487 | 6,183 |
United Kingdom | 924 | 9,953 | 10,877 | 2,732 | 2,567 |
Norway | 7,177 | 75 | 7,252 | 362 | 0 |
Sweden | 3,983 | 254 | 4,237 | 371 | 0 |
Denmark | 295 | 2,355 | 2,650 | 360 | 320 |
Israel | 1,019 | 25 | 1,044 | 158 | 2 |
- * Original source gives these individual numbers and totals them to 37,500 kW. The 2004 reported total was 30,700 kW.[25] With new installations of 6,800 kW, this would give the reported 37,500 kW.

Country | PV Capacity (MWp) | |||||
---|---|---|---|---|---|---|
Cumulative | Installed in 2006 | |||||
Off grid | On grid | Total | Off grid | On grid | Total | |
Germany | 32 | 3,031 | 3,063 | 3 | 1,150 | 1,153 |
Japan | ||||||
United States | 275 | 340 | 615 | 60 | 100 | 160 |
Spain | 15 | 103 | 118 | 1 | 59 | 60 |
Australia | ||||||
Italy | 13 | 45 | 58 | 0 | 11 | 11 |
Netherlands | 5 | 46 | 51 | 0.2 | 0.3 | 0.4 |
South Korea | 36 | 21 | ||||
France | 20.4 | 12.3 | 32.7 | 0.3 | 6.1 | 6.4 |
Austria | 3.1 | 25.9 | 29 | 0.2 | 4.8 | 5 |
Luxembourg | 0 | 23.6 | 23.6 | 0 | 0.04 | 0.04 |
United Kingdom | 1.1 | 12.6 | 13.6 | 0.2 | 2.6 | 2.8 |
Greece | 5.1 | 1.6 | 6.7 | 1.0 | 0.2 | 1.2 |
Sweden | 4.2 | 0.7 | 4.9 | 0.2 | 0.4 | 0.6 |
Belgium | 0.1 | 4.1 | 4.2 | 0 | 2.1 | 2.1 |
Finland | 3.8 | 0.3 | 4.1 | 0 | 0.06 | 0.06 |
Portugal | 2.7 | 0.8 | 3.5 | 0.25 | 0.23 | 0.48 |
Denmark | 0.3 | 2.6 | 2.9 | 0.03 | 0.2 | 0.23 |
Cyprus | 0.45 | 0.53 | 0.98 | 0.08 | 0.44 | 0.52 |
Source: Eurobserv'er' / SEIA / PV Power
Applications of PV
PV power stations
The Table below provides details of some of the largest photovoltaic plants in the world. As shown, Germany has a 10 MW photovoltaic system in Pocking, and a 12 MW plant in Arnstein, with a 40 MW power station planned for Muldentalkreis. Portugal has an 11 MW plant in Serpa and a 62 MW power station is planned for Moura. A 20 MW power plant is also planned for Beneixama, Spain. The photovoltaic power station proposed for Australia will use heliostat concentrator technology and will not come into service until 2010. It is expected to have a capacity of 154 MW when it is completed in 2013.[26]
DC Peak Power | Location | Description | GW·h/year | Coordinates |
---|---|---|---|---|
154 MW** | Mildura/Swan Hill, Australia[28] | Heliostat Concentrator Photovoltaic technology (see Solar power station in Victoria) |
n.a. | n.a. |
62 MW** | Moura, Portugal[29] [30] | BP, Yingli? | 88 | n.a. |
40 MW* | Muldentalkreis, Germany[31] [32] | 550,000 thin-film modules (First Solar) (see Waldpolenz Solar Park) | 40 | 51°19′43″N 12°39′20″E / 51.32861°N 12.65556°E |
20 MW** | Beneixama, Spain[33][34][35] | Tenesol, Aleo and Solon solar modules with Q-Cells cells | 30 | 38°43′26″N 0°43′48″W / 38.72389°N 0.73000°W |
12 MW | Arnstein, Germany[36] | 1408 SOLON mover (see Erlasee Solar Park) |
14 | n.a. |
11 MW | Serpa, Portugal[37] | 52,000 solar modules (see Serpa solar power plant) |
n.a. | n.a. |
10 MW | Pocking, Germany | 57,912 solar modules (see Pocking Solar Park) |
11.5 | n.a. |
9.5 MW | Milagro, Spain | see Monte Alto photovoltaic power plant | 14 | n.a. |
- * Project finish date: 2009; ** Under construction, as of spring 2007
PV in buildings
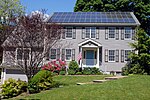
Building-integrated photovoltaics (BIPV) are increasingly incorporated into new domestic and industrial buildings as a principal or ancillary source of electrical power,[38] and are one of the fastest growing segments of the photovoltaic industry.[39] Typically, an array is incorporated into the roof or walls of a building, and roof tiles with integrated PV cells can now be purchased. Arrays can also be retrofitted into existing buildings; in this case they are usually fitted on top of the existing roof structure. Alternatively, an array can be located separately from the building but connected by cable to supply power for the building.
Where a building is at a considerable distance from the public electricity supply (or grid) - in remote or mountainous areas – PV may be the preferred possibility for generating electricity, or PV may be used together with wind, diesel generators and/or hydroelectric power. In such off-grid circumstances batteries are usually used to store the electric power.
PV in transport
PV has traditionally been used for auxiliary power in space. PV is rarely used to provide motive power in transport applications, but is being used increasingly to provide auxiliary power in boats and cars.
Economics of PV
![]() | This section possibly contains original research. |

Power costs
The PV industry is beginning to adopt levelized cost of energy as the unit of cost. The results of a sample calculation can be found on pp52, 53 of the 2007 DOE report describing the plans for solar power 2007-2011 [3]. For a 10MW plant in Phoenix, AZ, the LCOE is estimated at $0.15 to 0.22/kWh.
The table below is a pure mathematical calculation. It illustrates the calculated total cost in US cents per kWh of electricity generated by a photovoltaic system as function of the investment cost and the efficiency, assuming some accounting parameters such as cost of capital and depreciation period. The row headings on the left show the total cost, per peak kilowatt (kWp), of a photovoltaic installation. The column headings across the top refer to the annual energy output in kWh expected from each installed kWp. This varies by geographic region because the average insolation depends on the average cloudiness and the thickness of atmosphere traversed by the sunlight. It also depends on the path of the sun relative to the panel and the horizon.
Panels are usually mounted at an angle based on latitude, and often they are adjusted seasonally to meet the changing solar declination. Solar tracking can also be utilized to access even more perpendicular sunlight, thereby raising the total energy output. The calculated values in the table reflect the total cost in cents per kWh produced. They assume a 10% total capital cost (for instance 4% interest rate, 1% operating and maintenance cost, and depreciation of the capital outlay over 20 years). (Normally, photovoltaic modules have a 25 year warranty, but they should be fully functional even after 30-40 years.)
20 years | 2400 kWh/kWp y |
2200 kWh/kWp y |
2000 kWh/kWp y |
1800 kWh/kWp y |
1600 kWh/kWp y |
1400 kWh/kWp y |
1200 kWh/kWp y |
1000 kWh/kWp y |
800 kWh/kWp y |
---|---|---|---|---|---|---|---|---|---|
200 $/kWp | 0.8 | 0.9 | 1.0 | 1.1 | 1.3 | 1.4 | 1.7 | 2.0 | 2.5 |
600 $/kWp | 2.5 | 2.7 | 3.0 | 3.3 | 3.8 | 4.3 | 5.0 | 6.0 | 7.5 |
1000 $/kWp | 4.2 | 4.5 | 5.0 | 5.6 | 6.3 | 7.1 | 8.3 | 10.0 | 12.5 |
1400 $/kWp | 5.8 | 6.4 | 7.0 | 7.8 | 8.8 | 10.0 | 11.7 | 14.0 | 17.5 |
1800 $/kWp | 7.5 | 8.2 | 9.0 | 10.0 | 11.3 | 12.9 | 15.0 | 18.0 | 22.5 |
2200 $/kWp | 9.2 | 10.0 | 11.0 | 12.2 | 13.8 | 15.7 | 18.3 | 22.0 | 27.5 |
2600 $/kWp | 10.8 | 11.8 | 13.0 | 14.4 | 16.3 | 18.6 | 21.7 | 26.0 | 32.5 |
3000 $/kWp | 12.5 | 13.6 | 15.0 | 16.7 | 18.8 | 21.4 | 25.0 | 30.0 | 37.5 |
3400 $/kWp | 14.2 | 15.5 | 17.0 | 18.9 | 21.3 | 24.3 | 28.3 | 34.0 | 42.5 |
3800 $/kWp | 15.8 | 17.3 | 19.0 | 21.1 | 23.8 | 27.1 | 31.7 | 38.0 | 47.5 |
4200 $/kWp | 17.5 | 19.1 | 21.0 | 23.3 | 26.3 | 30.0 | 35.0 | 42.0 | 52.5 |
4600 $/kWp | 19.2 | 20.9 | 23.0 | 25.6 | 28.8 | 32.9 | 38.3 | 46.0 | 57.5 |
5000 $/kWp | 20.8 | 22.7 | 25.0 | 27.8 | 31.3 | 35.7 | 41.7 | 50.0 | 62.5 |
Grid parity
Grid parity, the point at which photovoltaic electricity is equal to or cheaper than grid power, has already been achieved in some regions. Grid parity has been reached in Hawaii and many other islands that use diesel fuel to produce electricity.
In Italy, PV power has been cheaper than retail grid electricity since 2006. One kWh in Italy costs 21.08 €-cents. [4] Italy has an average of 1,600 kWh/m² sun power per year (Sicily has even more, at 1,800 kWh/m²).
Financial incentives
The political purpose of incentive policies for PV is to grow the industry even where the cost of PV is significantly above grid parity, to allow it to achieve the economies of scale necessary to reach grid parity. The policies are implemented to promote national energy independence, high tech job creation and reduction of CO2 emissions.
Three incentive mechanisms are used (often in combination):
- investment subsidies: the authorities refund part of the cost of installation of the system,
- feed-in tariffs (FIT)/net metering: the electricity utility buys PV electricity from the producer under a multiyear contract at a guaranteed rate.
- Renewable Energy Certificates ("RECs")
With investment subsidies, the financial burden falls upon the taxpayer, while with feed-in tariffs the extra cost is distributed across the utilities' customer bases. While the investment subsidy may be simpler to administer, the main argument in favour of feed-in tariffs is the encouragement of quality. Investment subsidies are paid out as a function of the nameplate capacity of the installed system and are independent of its actual power yield over time, thus rewarding the overstatement of power and tolerating poor durability and maintenance. Some electric companies offer rebates to their customers, such as Austin Energy in Texas, which offers $4.50/watt installed up to $13,500.[40]
With feed-in tariffs, the financial burden falls upon the consumer. They reward the number of kilowatt-hours produced over a long period of time, but because the rate is set by the authorities, it may result in perceived overpayment. The price paid per kWh under a feed-in tariff exceeds the price of grid electricity. "Net metering" refers to the case where the price paid by the utility is the same as the price charged.
Where price setting by supply and demand is preferred, RECs can be used. In this mechanism, a renewable energy production or consumption target is set, and the consumer or producer is obliged to purchase renewable energy from whoever provides it the most competitively. The producer is paid via an REC. In principle this system delivers the cheapest renewable energy, since the lowest bidder will win. However uncertainties about the future value of energy produced are a brake on investment in capacity, and the higher risk increases the cost of capital borrowed.
The Japanese government through its Ministry of International Trade and Industry ran a successful programme of subsidies from 1994 to 2003. By the end of 2004, Japan led the world in installed PV capacity with over 1.1 GW.[5]
In 2004, the German government introduced the first large-scale feed-in tariff system, under a law known as the 'EEG' (Erneuerbare Energien Gesetz) which resulted in explosive growth of PV installations in Germany. At the outset the FIT was over 3x the retail price or 8x the industrial price. The principle behind the German system is a 20 year flat rate contract. The value of new contracts is programmed to decrease each year, in order to encourage the industry to pass on lower costs to the end users. The programme has been more successful than expected with over 1GW installed in 2006, and political pressure is mounting to decrease the tariff to lessen the future burden on consumers.
Subsequently Spain, Italy, Greece and France introduced feed-in tariffs. None have replicated the programmed decrease of FIT in new contracts though, making the German incentive relatively less and less attractive compared to other countries. The French FIT offers a uniquely high premium (EUR 0.55/kWh) for building integrated systems. California, Greece, France and Italy have 30-50% more insolation than Germany making them financially more attractive.
In 2006 California approved the 'California Solar Initiative', offering a choice of investment subsidies or FIT for small and medium systems and a FIT for large systems. The small-system FIT of $0.39 per kWh (far less than EU countries) expires in just 5 years, and the residential investment incentive is overwhelmed by a newly required time-of-use tariff, with a net cost increase to new systems. All California incentives are scheduled to decrease in the future depending as a function of the amount of PV capacity installed.
At the end of 2006, the Ontario Power Authority (Canada) began its Standard Offer Program, the first in North America for small renewable projects (10MW or less). This guarantees a fixed price of $0.42 CDN per kWh over a period of twenty years. Unlike net metering, all the electricity produced is sold to the OPA at the SOP rate. The generator then purchases any needed electricity at the current prevailing rate (e.g., $0.055 per kWh). The difference should cover all the costs of installation and operation over the life of the contract.
The price/kWh or kWp of the FIT or investment subsidies is only one of three factors that stimulate the installation of PV. The other two factors are insolation (the more sunshine, the less capital is needed for a given power output) and administrative ease of obtaining permits and contracts.
Unfortunately the complexity of approvals in California, Spain and Italy has prevented comparable growth to Germany even though the return on investment is better.
In some countries, additional incentives are offered for BIPV compared to stand alone PV.
- France + EUR 0.25/kWh
- Italy + EUR 0.04-0.09 kWh
- Germany + EUR 0.05/kWh (facades only)
Environmental impacts
Unlike fossil fuel based technologies, solar power does not lead to any harmful emissions during operation, but the production of the panels leads to some amount of pollution. Also, placement of photovoltaics affects the environment. If they are located where photosynthesizing plants would normally grow, they simply substitute one potentially renewable resource (biomass) for another. It should be noted, however, that the biomass cycle converts solar radiation energy to electrical energy with significantly less efficiency than photovoltaic cells alone. However, if they are placed on the sides of buildings (such as in Manchester) or fences, or rooftops (as long as plants would not normally be placed there), or in the desert they are purely additive to the renewable power base.
Greenhouse gases
Life cycle greenhouse gas emissions are now in the range of 25-32 g/kWh and this could decrease to 15 g/kWh in the future.[41] For comparison, a combined cycle gas-fired power plant emits some 400 g/kWh and a coal-fired power plant with carbon capture and storage some 200 g/kWh. Nuclear power emits 25 g/kWh on average; only wind power is better with a mere 11 g/kWh.
Cadmium
One issue that has often raised concerns is the use of cadmium in Cadmium telluride (CdTe) modules (not all PV technologies use CdTe). Cadmium in its metallic form is a toxic substance that has the tendency to accumulate in ecological food chains. The amount of cadmium used in PV modules is relatively small (5-10 g/m2) and with proper emission control techniques in place the cadmium emissions from module production can be almost zero. Current PV technologies lead to cadmium emissions of 0.3-0.9 microgram/kWh over the whole life-cycle.[41] Most of these emissions actually arise through the use of coal power for the manufacturing of the modules, and coal and lignite combustion leads to much higher emissions of cadmium. Life-cycle cadmium emissions from coal is 3.1 microgram/kWh, lignite 6.2, and natural gas 0.2 microgram/kWh.
Energy returned on energy invested
A key indicator of environmental performance is the ratio of electricity generated divided by the energy required to build and maintain the equipment. Of course, little is gained if it takes as much energy to produce the modules as they produce in their lifetimes. This ratio is called the energy returned on energy invested (EROEI) This should not be confused with the economic return on investment, which varies according to local energy prices, subsidies available and metering techniques. A related concept is the energy pay-back time, i.e. the time required to produce an amount of energy as great as what was consumed during production.
Crystalline silicon PV systems presently have energy pay-back times of 1.5-2 years for South-European locations and 2.7-3.5 years for Middle-European locations. For silicon technology clear prospects for a reduction of energy input exist, and an energy pay-back of 1 year may be possible within a few years. Thin film technologies now have energy pay-back times in the range of 1-1.5 years (S.Europe).[41] With lifetimes of such systems of at least 30 years, the EROEI is in the range of 10 to 30.
Photovoltaics companies
Major photovoltaics companies include BP Solar, Kyocera, Q-Cells, Sanyo, Sharp Solar, SolarWorld and Suntech.[42][43][44]
BP has been involved in solar power since 1973 and its subsidiary, BP Solar, is now one of the world's largest solar power companies with production facilities in the United States, Spain, India and Australia, employing a workforce of over 2,000 people worldwide.[45] BP solar is a major worldwide manufacturer and installer of photovoltaic solar cells for electricity.[46] The company has begun constructing two new solar photovoltaic (PV) solar cell manufacturing plants, one at its European headquarters in Tres Cantos, Madrid, and the second at its joint venture facility, Tata BP Solar, in Bangalore, India.[47]
Kyocera Corporation has announced a plan to increase its solar cell production to 500 MW per year in 2010. 500 MW is about three times the current output of 180 MW, and the company will reinforce production bases in Japan, the US, Europe and China, investing a total of about ¥30 billion through FY2010. Through this production enhancement, Kyocera looks to meet increasing demand across the world for solar cells.[48][49]
Q-Cells is the world's second largest cell manufacturer, based in Thalheim, Germany.[50]
Renewable Energy Corporation (REC) is based in Norway, and was established in 1996. Over a relatively short period, REC has become the world's largest producer of polysilicon and wafers for PV applications. REC is involved in all steps of the value chain, from production of solar grade silicon to wafer, cell and module production. The company has customers all over the globe and seven production plants in three different countries. It operates on three different continents and has approximately 1,100 employees.[51]
Sanyo Electric produced $213 million worth of solar cells at its plant in Hungary in 2006, and expects to triple its production capacity to 720,000 units in 2008.[52]
Sharp Solar is the world's largest photovoltaic module and cell manufacturer, which manufactures in Japan, and near Wrexham, UK. Sharp Solar produces both single and multi-crystalline solar cells which are used for many applications, from satellites to lighthouses, and industrial applications to residential use. Sharp began researching solar cells in 1959 with mass production first beginning in 1963. Production capacity amounted to 324 MW in 2004.[53][54]
SolarWorld is headquartered in Bonn, and purchased Shell Solar's crystalline silicon activities in 2006.
Suntech Power is based in Wuxi, China, where construction of a 1 GW module plant has begun. Year-end production capacity for 2007 is expected to be 480 MW.[55]
United Solar Ovonic, a wholly owned subsidiary of Energy Conversion Devices Ovonics currently employs 700 people and expects to increase its production of thin-film amorphous photovoltaics from 117 MW/yr at the end of 2007 to over 300 MW/yr by the end of 2010. They are best known for producing building-integrated photovoltaic (BIPV) roofing systems (roofing shingle photovoltaics) and continuous roll-to-roll manufacturing of thin-film amorphous silicon alloy multi-junction solar cells.[56]
Photovoltaic Industry Associations
- SEIA: Solar Energy Industries Association US trade association of solar energy manufacturers, dealers, distributors, contractors
- EPIA: European Photovoltaic Industry association
- JPEA: Japanese Photovoltaic Energy Association in Japanese only
- BSW: German Solar Industry Associationin German, with English summary
- ASIF: Spanish PV Industry Associationin Spanish only
- Canadian Solar industry Association
Photovoltaics research institutes
There are many research institutions and departments at universities around the world who are active in photovoltaics research. Countries which are particularly active include Germany, Spain, Japan, Australia, China, and the USA.
Some universities and institutes which have a photovoltaics research department.
- National Renewable Energy Laboratory NREL
- Institut für Solare Energiesysteme ISE at the Fraunhofer Institute
- Energy research Centre of the Netherlands (ECN)
- Imperial College London: Experimental Solid State Physics
- Instituto de Energía Solar, at Universidad Politécnica de Madrid
- Centre for Renewable Energy Systems Technology, at Loughborough University
- School of Photovoltaic and Renewable Energy Engineering at The University of New South Wales
- Centre for Sustainable Energy Systems at the Australian National University
- Ecole Polytechnique Fédérale de Lausanne Prof. Graetzel invented dye sensitized cells here
- Advanced Energy Systems at Helsinki University of Technology
- The Centre for Electronic Devices and Materials at Sheffield Hallam University
- The Solar Caliometry Lab at Queen's University
- Energy & Environmental Technology Application Center at the College of Nanoscale Science and Engineering SUNY at Albany
- Institute of microtechnology at University of Neuchatel Switzerland
- University of Konstanz
- Arizona State University Photovoltaic Testing Laboratory
- Institute of Energy Conversion at University of Delaware
- World Alliance for Decentralized Energy
- Florida Solar Energy Center at University of Central Florida
See also
- Active solar
- Deployment of solar power to energy grids
- Green technology
- High efficiency solar cells
- Islanding
- Microgeneration
- Photoelectrochemical cell
- Photovoltaic and renewable energy engineering in Australia
- Photovoltaics in transport
- Renewable energy
- Renewable energy in the European Union
- Solar car
- Solar thermal
- Solar power
- Solar cell
- Solar panel
Template:Energy related development
References
- ^ School of Photovoltaic and Renewable Energy Engineering
- ^ Arizona State University Photovoltaic Testing Laboratory
- ^ German PV market
- ^ BP Solar to Expand Its Solar Cell Plants in Spain and India
- ^ Large-Scale, Cheap Solar Electricity
- ^ Installed PV power as of the end of 2005
- ^ GE Invests, Delivers One of World's Largest Solar Power Plants
- ^ Building integrated photovoltaics
- ^ German PV market
- ^ Solar Module Price Environment
- ^ A Better Way to Make Solar Power
- ^ Company Information Overview
- ^ The technology at a glance
- ^ Converting sunlight to electricity
- ^ Solar Photovoltaic Panels
- ^ MMA Renewable Ventures Solar Energy Program
- ^ U.S. Retailers Save with Solar PV & Energy Efficiency
- ^ Antonio Luque and Steven Hegedus (2003). Handbook of Photovoltaic Science and Engineering. John Wiley and Sons. ISBN 0471491969.
- ^ Nuclear Energy Institute 'Up Front - Nuclear facts'
- ^ UtiliPoint International, Inc. 'Issue alert - What is a megawatt?
- ^ PB plc - 'Solar power'
- ^ Total electric power consumption
- ^ German PV market
- ^ Table 1: Installed PV power in reporting IEA PVPS countries as of the end of 2005
- ^ Total photovoltaic power installed in IEA PVPS countries accessed 24 July 2007
- ^ Solar Systems Facts Sheet
- ^ World's largest photovoltaic power plants
- ^ 154 MW Victoria (Australia) Project
- ^ Portugal plans biggest solar station
- ^ THE WORLD'S LARGEST PHOTOVOLTAIC POWER PLANT IN MOURA, PORTUGAL
- ^ Large photovoltaic plant in Muldentalkreis
- ^ World’s largest solar power plant being built in eastern Germany
- ^ Large photovoltaic plant in Beneixama
- ^ Photovoltaic plant in Beneixama
- ^ Image of world's largest solar plant
- ^ The largest photovoltaic plant
- ^ "GE, SunPower, Catavento team on plant". BusinessWeek. 2007-03-28. Retrieved 2007-03-29.
{{cite web}}
: Check date values in:|date=
(help); Cite has empty unknown parameter:|coauthors=
(help) - ^ buildingsolar.com: Building Integrated Photovoltaics, Wisconsin Public Service Corporation, accessed: 2007-03-23.
- ^ Terrasolar, accessed: 2007-03-23.
- ^ Solar Rebate Program
- ^ a b c Alsema, E.A.; Wild - Scholten, M.J. de; Fthenakis, V.M. Environmental impacts of PV electricity generation - a critical comparison of energy supply options ECN, September 2006; 7p. Presented at the 21st European Photovoltaic Solar Energy Conference and Exhibition, Dresden, Germany, 4-8 September 2006.
- ^ ENF Brand Awards
- ^ Photovoltaic Solar Cells
- ^ World solar cell manufacturers
- ^ Solar Power Profitability: BP Solar
- ^ Welcome to BP Solar
- ^ BP Solar to Expand Its Solar Cell Plants in Spain and India
- ^ Kyocera to Triple Solar Cell Production to 500 MW in FY2010
- ^ Solar firm to double capacity
- ^ Q-cells
- ^ Evergreen Solar and Q-Cells Announce Partnership with REC
- ^ Japan's Sanyo expands Hungary solar plant
- ^ Sharp Solar Modules
- ^ Sharp Solar celebrates five years as world number one
- ^ Suntech Reports First Quarter 2007 Financial Results
- ^ Solar: Overview
External links
Publicly funded free data sources
- EU PV Technology Platform - forum for stakeholders to influence EU policy,
- PV Status Report 2006 : Comprehensive global overview by Arnulf Jager-Waldau, European Commission.
- Trends in photovoltaic applications in selected IEA countries between 1992 and 2004
- Information pertaining to photovoltaic solar electricity in each of the IEA PVPS member countries
- US Department of Energy Energy Efficiency and Renewable Energy
- DSIRE Listing of US state, local, utility, and federal incentives for renewable energy and energy efficiency.
- Energy Saving Trust (UK) - What is Solar electricity?
Trade Press and commercial databases
- Solarbuzz Online news
- Photon International International PV magazine, also has local editions for Germany and Spain
- Renewable Energy World magazine covers PV among other forms of renewable energy
- ENF Ltd PV Market Research and Industry Directory
- Home Power Magazine
Others