Nuclear fallout
This article needs additional citations for verification. (November 2008) |
Nuclear weapons |
---|
![]() |
Background |
Nuclear-armed states |
|
Nuclear fallout, or simply fallout, is the residual radioactive material propelled into the upper atmosphere following a nuclear blast or a nuclear reaction conducted in an unshielded facility, so called because it "falls out" of the sky after the explosion and the shock wave have passed. It commonly refers to the radioactive dust and ash created when a nuclear weapon explodes, but such dust can also originate from a damaged nuclear plant. Fallout may take the form of black rain (rain darkened by particulates).
This radioactive dust, consisting of material either directly vaporized by a nuclear blast or charged by exposure, is a highly dangerous kind of radioactive contamination.
Types of fallout
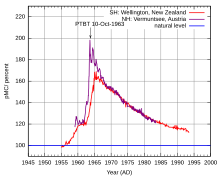
An air burst (that is, a nuclear detonation far above the surface) can eventually produce worldwide fallout. A ground burst can produce possibly much more severe, local fallout.
Global fallout
After an air burst, fission products, un-fissioned nuclear material, and weapon residues vaporized by the heat of the fireball condense into a fine suspension of small particles 10 nm to 20 µm in diameter. These particles may be quickly drawn up into the stratosphere, particularly if the explosive yield exceeds 10 kt.
Initially little was known about the dispersion of nuclear fallout on a global scale. The AEC assumed that fallout would be dispersed evenly across the globe by atmospheric winds and gradually settle to the Earth's surface after weeks, months, and even years as worldwide fallout.
The radio-biological hazard of worldwide fallout is a long-term one because of the potential accumulation of long-lived radioisotopes (such as strontium-90 and caesium-137) in the body as a result of ingestion of foods containing the radioactive materials. This hazard is less pertinent than local fallout, which is of much greater immediate operational concern.
Local fallout
In a land or water surface burst, heat vaporizes large amounts of earth or water, which is drawn up into the radioactive cloud. This material becomes radioactive when it condenses with fission products and other radiocontaminants that have become neutron-activated. The table below summarizes the abilities of common isotopes to form fallout. Some radiation taints large amounts of land and drinking water causing formal mutations throughout animal and human life.

Isotope | 91Sr | 92Sr | 95Zr | 99Mo | 106Ru | 131Sb | 132Te | 134Te | 137Cs | 140Ba | 141La | 144Ce |
---|---|---|---|---|---|---|---|---|---|---|---|---|
Refractory index | 0.2 | 1.0 | 1.0 | 1.0 | 0.0 | 0.1 | 0.0 | 0.0 | 0.0 | 0.3 | 0.7 | 1.0 |

A surface burst generates large amounts of particulate matter, composed of particles from less than 100 nm to several millimeters in diameter—in addition to very fine particles that contribute to worldwide fallout. The larger particles spill out of the stem and cascade down the outside of the fireball in a downdraft even as the cloud rises, so fallout begins to arrive near ground zero within an hour. More than half the total bomb debris lands on the ground within about 24 hours as local fallout. Chemical properties of the elements in the fallout control the rate at which they are deposited on the ground. Less volatile elements deposit first.
Severe local fallout contamination can extend far beyond the blast and thermal effects, particularly in the case of high yield surface detonations. The ground track of fallout from an explosion depends on the weather from the time of detonation onwards. In stronger winds, fallout travels faster but takes the same time to descend, so although it covers a larger path, it is more spread out or diluted. Thus, the width of the fallout pattern for any given dose rate is reduced where the downwind distance is increased by higher winds. The total amount of activity deposited up to any given time is the same irrespective of the wind pattern, so overall casualty figures from fallout are generally independent of winds. But thunderstorms can bring down activity as rain more rapidly than dry fallout, particularly if the mushroom cloud is low enough to be below ("washout"), or mixed with ("rainout"), the thunderstorm.
Whenever individuals remain in a radiologically contaminated area, such contamination leads to an immediate external radiation exposure as well as a possible later internal hazard from inhalation and ingestion of radiocontaminants, such as the rather short-lived iodine-131, which is accumulated in the thyroid.
Factors affecting fallout
Location
There are two main considerations for the location of an explosion: height and surface composition. A nuclear weapon detonated in the air, called an air burst, produces less fallout than a comparable explosion near the ground.
In case of water surface bursts, the particles tend to be rather lighter and smaller, producing less local fallout but extending over a greater area. The particles contain mostly sea salts with some water; these can have a cloud seeding effect causing local rainout and areas of high local fallout. Fallout from a seawater burst is difficult to remove once it has soaked into porous surfaces because the fission products are present as metallic ions that chemically bond to many surfaces. Water and detergent washing effectively removes less than 50% of this chemically bonded activity from concrete or steel. Complete decontamination requires aggressive treatment like sandblasting, or acidic treatment. After the Crossroads underwater test, it was found that wet fallout must be immediately removed from ships by continuous water washdown (such as from the fire sprinkler system on the decks).
Parts of the sea bottom may become fallout. After the Castle Bravo test, white dust—contaminated calcium oxide particles originating from pulverized and calcined corals—fell for several hours, causing beta burns and radiation exposure to the inhabitants of the nearby atolls and the crew of the Daigo Fukuryū Maru fishing boat. The scientists called the fallout Bikini snow.
For subsurface bursts, there is an additional phenomenon present called "base surge". The base surge is a cloud that rolls outward from the bottom of the subsiding column, which is caused by an excessive density of dust or water droplets in the air. For underwater bursts, the visible surge is, in effect, a cloud of liquid (usually water) droplets with the property of flowing almost as if it were a homogeneous fluid. After the water evaporates, an invisible base surge of small radioactive particles may persist.
For subsurface land bursts, the surge is made up of small solid particles, but it still behaves like a fluid. A soil earth medium favors base surge formation in an underground burst. Although the base surge typically contains only about 10% of the total bomb debris in a subsurface burst, it can create larger radiation doses than fallout near the detonation, because it arrives sooner than fallout, before much radioactive decay has occurred.
Meteorological
Meteorological conditions greatly influence fallout, particularly local fallout. Atmospheric winds are able to bring fallout over large areas. For example, as a result of a Castle Bravo surface burst of a 15 Mt thermonuclear device at Bikini Atoll on March 1, 1954, a roughly cigar-shaped area of the Pacific extending over 500 km downwind and varying in width to a maximum of 100 km was severely contaminated. There are three very different versions of the fallout pattern from this test, because the fallout was only measured on a small number of widely spaced Pacific Atolls. The two alternative versions both ascribe the high radiation levels at north Rongelap to a downwind hotspot caused by the large amount of radioactivity carried on fallout particles of about 50-100 micrometres size.[1]
After Bravo, it was discovered that fallout landing on the ocean disperses in the top water layer (above the thermocline at 100 m depth), and the land equivalent dose rate can be calculated by multiplying the ocean dose rate at two days after burst by a factor of about 530. In other 1954 tests, including Yankee and Nectar, hotspots were mapped out by ships with submersible probes, and similar hotspots occurred in 1956 tests such as Zuni and Tewa. [2] However, the major U.S. 'DELFIC' (Defence Land Fallout Interpretive Code) computer calculations use the natural size distributions of particles in soil instead of the afterwind sweep-up spectrum, and this results in more straightforward fallout patterns lacking the downwind hotspot.
Snow and rain, especially if they come from considerable heights, accelerate local fallout. Under special meteorological conditions, such as a local rain shower that originates above the radioactive cloud, limited areas of heavy contamination just downwind of a nuclear blast may be formed.
Effects
A wide range of biological changes may follow the irradiation of animals. These vary from rapid death following high doses of penetrating whole-body radiation, to essentially normal lives for a variable period of time until the development of delayed radiation effects, in a portion of the exposed population, following low dose exposures.
The unit of actual exposure is the röntgen, defined in ionisations per unit volume of air. All ionisation based instruments (including geiger counters and ionisation chambers) measure exposure. However, effects depend on the energy per unit mass, not the exposure measured in air. A deposit of 1 joule per kilogram has the unit of 1 gray (Gy). For 1 MeV energy gamma rays, an exposure of 1 röntgen in air produces a dose of about 0.01 gray (1 centigray, cGy) in water or surface tissue. Because of shielding by the tissue surrounding the bones, the bone marrow only receives about 0.67 cGy when the air exposure is 1 röntgen and the surface skin dose is 1 cGy. Some lower values reported for the amount of radiation that would kill 50% of personnel (the LD50) refer to bone marrow dose, which is only 67% of the air dose.
Short term
This section needs additional citations for verification. (December 2008) |
The dose that would be lethal to 50% of a population is a common parameter used to compare the effects of various fallout types or circumstances. Usually, the term is defined for a specific time, and limited to studies of acute lethality. The common time periods used are 30 days or less for most small laboratory animals and to 60 days for large animals and humans. The LD50 figure assumes that the individuals did not receive other injuries or medical treatment.
In the 1950s, the LD50 for gamma rays was set at 3.5 Gy, while under more dire conditions of war (a bad diet, little medical care, poor nursing) the LD50 was 2.5 Gy (250 rad). There have been few documented cases of survival beyond 6 Gy. One person at Chernobyl survived a dose of more than 10 Gy, but many of the persons exposed there were not uniformly exposed over their entire body. If a person is exposed in a non-homogeneous manner then a given dose (averaged over the entire body) is less likely to be lethal. For instance, if a person gets a hand/low arm dose of 100 Gy, which gives them an overall dose of 4 Gy, they are more likely to survive than a person who gets a 4 Gy dose over their entire body. A hand dose of 10 Gy or more would likely result in loss of the hand. A British industrial radiographer who got a lifetime hand dose of 100 Gy lost his hand because of radiation dermatitis[citation needed]. Most people become ill after an exposure to 1 Gy or more. The fetuses of pregnant women are often more vulnerable to radiation and may miscarry, especially in the first trimester.
One hour after a surface burst, the radiation from fallout in the crater region is 30 grays per hour (Gy/h)[clarification needed]. Civilian dose rates in peacetime range from 30 to 100 µGy per year.
Fallout radiation decays exponentially relatively quickly with time. Most areas become fairly safe for travel and decontamination after three to five weeks.[citation needed]
For yields of up to 10 kt, prompt radiation is the dominant producer of casualties on the battlefield. Humans receiving an acute incapacitating dose (30 Gy) have their performance degraded almost immediately and become ineffective within several hours. However, they do not die until five to six days after exposure, assuming they do not receive any other injuries. Individuals receiving less than a total of 1.5 Gy are not incapacitated. People receiving doses greater than 1.5 Gy become disabled, and some eventually die.
A dose of 5.3 Gy to 8.3 Gy is considered lethal but not immediately incapacitating. Personnel exposed to this amount of radiation have their performance degraded in two to three hours, depending on how physically demanding the tasks they must perform are, and remain in this disabled state at least two days. However, at that point they experience a recovery period and can perform non-demanding tasks for about six days, after which they relapse for about four weeks. At this time they begin exhibiting symptoms of radiation poisoning of sufficient severity to render them totally ineffective. Death follows at approximately six weeks after exposure, although outcomes may vary.
Long term


Late or delayed effects of radiation occur following a wide range of doses and dose rates. Delayed effects may appear months to years after irradiation and include a wide variety of effects involving almost all tissues or organs. Some of the possible delayed consequences of radiation injury are life shortening, carcinogenesis, cataract formation, chronic radiodermatitis, decreased fertility, and genetic mutations.[3] Presently, the only teratological effect observed in humans following nuclear attacks on highly populated areas is microcephaly which is the only proven malformation, or congenital abnormality, found in the in utero developing human fetuses present during the Hiroshima and Nagasaki bombings. Of all the pregnant women exposed in the two cities, the number of children born with microcephaly was below 50.[4] No statistically demonstrable increase of congenital malformations was found among the later conceived children born to survivors of the nuclear detonations at Hiroshima and Nagasaki.[4][5][6] The surviving women of Hiroshima and Nagasaki who could conceive and were exposed to substantial amounts of radiation went on and had children with no higher incidence of abnormalities than the Japanese average.[7][8]
The Baby Tooth Survey helped to determine the effects of nuclear fallout in the human anatomy by examining the levels of radioactive material absorbed into the deciduous teeth of children. Founded by the husband and wife team of physicians Eric Reiss and Louise Reiss, the research focused on detecting the presence of strontium-90, a cancer-causing radioactive isotope created by the more than 400 atomic tests conducted above ground that is absorbed from water and dairy products into the bones and teeth given its chemical similarity to calcium. The team sent collection forms to schools in the St. Louis, Missouri area, hoping to gather 50,000 teeth each year. Ultimately, the project collected over 300,000 teeth from children of various ages before the project was ended in 1970.[9]
Preliminary results of the Baby Tooth Survey were published in the November 24, 1961, edition of the journal Science, and showed that levels of strontium 90 had risen steadily in children born in the 1950s, with those born later showing the most pronounced increases.[10] The results of a more comprehensive study of the elements found in the teeth collected showed that children born after 1963 had levels of strontium 90 in their baby teeth that was 50 times higher than that found in children born before large-scale atomic testing began. The findings helped convince U.S. President John F. Kennedy to sign the Partial Nuclear Test Ban Treaty with the United Kingdom and Soviet Union, which ended the above-ground nuclear weapons testing that created the greatest amounts of atmospheric nuclear fallout.[11]
Fallout protection
During the Cold War, the governments of the U.S., the USSR, Great Britain, and China attempted to educate their citizens about surviving a nuclear attack by providing procedures on minimizing short-term exposure to fallout. This effort commonly became known as Civil Defense.
Fallout protection is almost exclusively concerned with protection from radiation. Radiation from fallout is encountered in the forms of alpha, beta, and gamma radiation, and as ordinary clothing affords protection from alpha and beta radiation,[12] most fallout protection measures deal with reducing exposure to gamma radiation.[13] For the purposes of radiation shielding, many materials have a characteristic halving thickness: the thickness of a layer of a material sufficient to reduce gamma radiation exposure by 50%. Halving thicknesses of common materials include: 1 cm (0.4 inch) of lead, 6 cm (2.4 inches) of concrete, 9 cm (3.6 inches) of packed earth or 150 m (500 ft) of air. When multiple thicknesses are built, the shielding multiplies. A practical fallout shield is ten halving-thicknesses of a given material, such as 90 cm (36 inches) of packed earth, which reduces gamma ray exposure by approximately 1024 times (210).[14][15] A shelter built with these materials for the purposes of fallout protection is known as a fallout shelter.
The danger of radiation from fallout also decreases with time, as radioactivity decays exponentially with time, such that for each factor of seven increase in time, the radiation is reduced by a factor of ten. For example, after 7 hours, the average dose rate is reduced by a factor of ten; after 49 hours, it is reduced by a further factor of ten (to 1/100th); after two weeks the radiation from the fallout will have reduced by a factor of 1000 compared the initial level; and after 14 weeks the average dose rate will have reduced to 1/10,000th of the initial level.[15]
Nuclear reactor accident
Fallout can also refer to nuclear accidents, although a nuclear reactor does not explode like a nuclear weapon. The isotopic signature of bomb fallout is very different from the fallout from a serious power reactor accident (such as Chernobyl or Fukushima). The Fukushima plants have tons of nuclear fuel, thousands of Fuel Assemblies, more than 6,000 fuel rods[16] in spent fuel pools.
The key differences are in volatility and half-life.
Volatility
The boiling point of an element (or its compounds) is able to control the percentage of that element a power reactor accident releases. The ability of an element to form a solid, controls the rate it is deposited on the ground after having been injected into the atmosphere by a nuclear detonation or accident.
Half-life
A half life is the time it takes half of the radiation of a specific substance to decay. A large amount of short-lived isotopes such as 97Zr are present in bomb fallout. This isotope and other short-lived isotopes are constantly generated in a power reactor, but because the criticality occurs over a long length of time, the majority of these short lived isotopes decay before they can be released.
See also
- Atomic Cafe—Documentary film about nuclear civil defense films.
- Castle Bravo—largest nuclear fallout accident by United States.
- Dirty bomb
- Fallout: An American Nuclear Tragedy
- Fallout Protection—U.S. government booklet
- Effects of nuclear explosions
- Fallout (RTÉ drama)—Irish drama exploring some inane and improbable scenarios following a nuclear accident at Sellafield.
- Fallout (series)
- Fallout shelter
- Fission product
- Hot Particle
- Human radiation experiments
- List of nuclear accidents
- Lists of nuclear disasters and radioactive incidents
- Neutron bomb
- Nuclear terrorism
- Nuclear war survival skills by Cresson Kearny
- Nuclear weapon design
- Potassium iodide
- Project GABRIEL
- Protect and Survive, a series of booklets and a public information film series produced for the British government in the 1970s and 1980s.
- Radioactive contamination
- Radiation poisoning
- Radiation biology
- Radioactive waste
- Radiological weapon
- Joseph Rotblat
- Survival Under Atomic Attack, an official U.S. government booklet regarding the effects of a nuclear attack.
References
- ^ Howard A. Hawthorne, Editor (May 1979). "COMPILATION OF LOCAL FALLOUT DATA FROM TEST DETONATIONS 1945-1962 - EXTRACTED FROM DASA 1251 - Volume II - Oceanic U.S. Tests" (PDF). General Electric Company. Archived from the original (PDF) on 2008-04-10.
{{cite journal}}
:|author=
has generic name (help); Cite journal requires|journal=
(help) - ^ Project Officer T. Triffet, P. D. LaRiviere (March 1961). "OPERATION REDWING - Project 2.63, Characterization of Fallout - Pacific Proving Grounds, May-July 1956" (PDF). US Naval Radiological Defense Laboratory. Archived from the original (PDF) on 2008-04-10.
{{cite journal}}
: Cite journal requires|journal=
(help) - ^ Simon, Steven L.; Bouville, André; Land, Charles E. (2006), Fallout from Nuclear Weapons Tests and Cancer Risks, vol. 94, American Scientist, pp. 48–57
- ^ a b Teratology in the Twentieth Century Plus Ten
- ^ JAMA Network | JAMA | The Children of Atomic Bomb Survivors: A Genetic Study
- ^ British Journal of Cancer - Sex ratio among offspring of childhood cancer survivors treated with radiotherapy
- ^ Birth defects among the children of atomic-bomb survivors (1948-1954) - Radiation Effects Research Foundation
- ^ NUCLEAR CRISIS: Hiroshima and Nagasaki cast long shadows over radiation science - Monday, April 11, 2011 - www.eenews.net
- ^ Staff. "Teeth to Measure Fall-Out", The New York Times, March 18, 1969.
- ^ Sullivan, Walter. "Babies Surveyed for Strontium 90; Ratio to Calcium in Bones Is Discovered to Be Low A survey has shown that pregnant mothers and their unborn children absorb radioactive strontium, as a substitute for calcium, only about 10 per cent of the time", The New York Times, November 25, 1961.
- ^ Hevesi, Dennis. "Dr. Louise Reiss, Who Helped Ban Atomic Testing, Dies at 90", The New York Times, January 10, 2011.
- ^ Kearny, Cresson H (1986). Nuclear War Survival Skills. Oak Ridge, TN: Oak Ridge National Laboratory. p. 44. ISBN 0-942487-01-X.
- ^ Kearny, Cresson H (1986). Nuclear War Survival Skills. Oak Ridge, TN: Oak Ridge National Laboratory. p. 131. ISBN 0-942487-01-X.
- ^ "Halving-thickness for various materials". "The Compass DeRose Guide to Emergency Preparedness - Hardened Shelters".
- ^ a b Kearny, Cresson H (1986). Nuclear War Survival Skills. Oak Ridge, TN: Oak Ridge National Laboratory. pp. 11–20. ISBN 0-942487-01-X.
- ^ "No. 1 fuel pool power to be restored: Tepco". Retrieved 20 March 2013.
General references
- Glasstone, Samuel and Dolan, Philip J., The Effects of Nuclear Weapons (third edition), U.S. Government Printing Office, 1977. (Available Online)
- NATO Handbook on the Medical Aspects of NBC Defensive Operations (Part I - Nuclear), Departments of the Army, Navy, and Air Force, Washington, D.C., 1996, (Available Online)
- Smyth, H. DeW., Atomic Energy for Military Purposes, Princeton University Press, 1945. (Smyth Report)
- The Effects of Nuclear War, Office of Technology Assessment (May 1979), (Available Online)
- T. Imanaka, S. Fukutani, M. Yamamoto, A. Sakaguchi and M. Hoshi, J. Radiation Research, 2006, 47, Suppl A121-A127.
- Sheldon Novick, The Careless Atom (Boston MA: Houghton Mifflin Co., 1969), p. 98
External links
