Antibody: Difference between revisions
m →See also: spam |
|||
Line 121: | Line 121: | ||
*[http://www.antibodypatterns.com/ Variety of antibody images] |
*[http://www.antibodypatterns.com/ Variety of antibody images] |
||
*[http://www.ii.bham.ac.uk/clinicalimmunology/CISimagelibrary/ More images generated by antibodies] |
*[http://www.ii.bham.ac.uk/clinicalimmunology/CISimagelibrary/ More images generated by antibodies] |
||
*[http://www.antibodyresource.com/ Antibody Resource Page] |
|||
Revision as of 14:54, 24 February 2007

An antibody or immunoglobulin is a large Y-shaped protein used by the immune system to identify and neutralize foreign objects like bacteria and viruses. Each antibody recognizes a specific antigen unique to its target.[1] This is because the two tips of the "Y" of the antibody contain a paratope (a structure analogous to a lock) that is specific for one particular epitope (analogous to a key) on an antigen, allowing these two structures to precisely bind together. This precise binding mechanism allows an antibody to tag a microbe or an infected cell for attack by other parts of the immune system, or to directly neutralize its target (i.e. by blocking a part of a microbe that is essential for its invasion and survival). The production of antibodies is the main function of the humoral immune system.[2]
Antibodies are soluble glycoproteins of the immunoglobulin superfamily.[3] The terms antibody and immunoglobulin are often used interchangeably. When attached to the surface of the B cell, the membrane-bound form of the immunoglobulin is sometimes referred to as the B cell receptor (BCR). Soluble antibodies are found in the blood and tissue fluids, as well as many secretions. In structure, they are globulins (in the γ-region of protein electrophoresis). They are synthesized and secreted by plasma cells that are derived from the B cells of the immune system.[4] Membrane-bound immunoglobulins are only found on the surface of B cells and facilitate the activation of these cells following binding of their specific antigen, and their subsequent differentiation into plasma cells for antibody generation, or memory cells that will remember the foreign antigen during future exposure. In most cases, interaction of the B cell with a T helper cell is necessary to produce full activation of the B cell and, therefore, antibody generation following antigen binding.[4]
Immunoglobulin isotypes


In mammals there are five types of antibody: IgA, IgD, IgE, IgG, and IgM, with 4 IgG and 2 IgA subtypes present in humans (where Ig stands for immunoglobulin).[1] These are classified according to differences in their heavy chain constant domains (see below for more information regarding the structural features of antibodies).[3] Each immunoglobulin class differs in its biological properties and has evolved to deal with different antigens.
- IgA can be found in areas containing mucus (e.g. in the gut, in the respiratory tract or in the urogenital tract) and prevents the colonization of mucosal areas by pathogens.[2]
- IgD functions mainly as an antigen receptor on B cells.[2]
- IgE binds to allergens and triggers histamine release from mast cells (the underlying mechanism of allergy) and also provides protection against helminths (worms).[2]
- IgG (in its four forms) provides the majority of antibody-based immunity against invading pathogens.[2]
- IgM is expressed on the surface of B cells and also in a secreted form with very high affinity for eliminating pathogens in the early stages of B cell mediated immunity (i.e. before there is sufficient IgG to do the job).[2]
Immature B cells express only IgM on their cell surface (this is the surface bound form, not the secreted form of immunoglobulin). Once the naive B cell reaches maturity, it can express both IgM and IgD on its surface - it is the co-expression of both these immunoglobulin isotypes that renders the B cell 'mature' and ready to respond to antigen.[1] Following engagement of the immunoglobulin molecule with an antigen, the B cell activates, and begins to divide and differentiate into an antibody producing cell (sometimes called a plasma cell). In this activated form, the B cell produces immunoglobulin in a secreted form rather than a membrane-bound form. Some of the daughter cells of the activated B cells undergo isotype switching, a mechanism by which the B cell begins to express the other Ig heavy chains and thus produce the IgD, IgA or (more commonly) IgG antibody isotypes.[1]
Immunoglobulin diversity
V(D)J recombination
Somatic recombination, also known as V(D)J recombination, of immunoglobulins involves the random selection and combination of genes encoding each segment of the immunoglobulin variable region in a manner that generates a huge repertoire of antibodies with different paratopes. These segments are called variable (V), diversity (D) and joining (J) segments. V, D and J segments are found in Ig heavy chains but only V and J segments are found in Ig light chains. Multiple copies of the V, D and J segments exist tandemly arranged in the genomes of mammals. Their selection for recombination within the individual B cell is also called gene rearrangement.[5] A B cell that successfully produces a functional immunoglobulin gene during its V(D)J recombination will suppress the expression of any other variable region gene by a process known as allelic exclusion. Thus, the variable regions of all the immunoglobulin molecules within one given B cell will be the same, although the constant domains of the heavy chains can differ.[1] The diversity generated by this mechanism in the variable region of the heavy chain - to be specific, in the area that these V, D and J genes encode, otherwise known as the complementarity determining region 3 (CDR3) - provides the human immune system its ability to bind so many distinct antigens.
Somatic hypermutation
A further mechanism for generating antibody diversity exists for the mature B cell after antigen stimulation. Activated B cells are more prone to somatic hypermutation in their immunoglobulin variable chain genes.[5] This generates slight changes in the amino acid sequence of the variable domains of both the light and heavy chains between clones of the same activated B cell, and ultimately, differences in the affinity or strength of interaction that the B cell has with its specific antigen.[1] Thus, B cells expressing immunoglobulins with higher affinity for the antigen will outcompete those with weaker immunoglobulin for function and survival in a process known as affinity maturation.[5]
Class switching
Isotype (or class) switching occurs after the process of V(D)J recombination and following activation of the mature B cell (see above) to generate the different classes of antibody, all with the same variable domains as the original immunoglobulin generated in the immature B cell during recombination, but possessing distinct constant domains in their heavy chains.[5]
Structure of the antibody
Immunoglobulins are heavy plasma proteins, often with sugar chains added to amino acid residues by N-linked glycosylation (all antibodies) and occasionally O-linked glycosylation (e.g. IgA1 and IgD). In other words, they are glycoproteins. The basic unit of each antibody is a monomer (one Ig unit) but the secreted antibody can also be dimeric (with two Ig units as with IgA), tetrameric (with four Ig units, like teleost fish IgM), or pentameric (with five Ig units, like mammalian IgM). The monomer is a "Y"-shaped molecule that consists of four polypeptide chains; two identical heavy chains and two identical light chains connected by disulfide bonds.

Heavy chain
There are five types of mammalian immunoglobulin heavy chain: γ, δ, α, μ and ε.[1] They define classes of immunoglobulins. Heavy chains α and γ have approximately 450 amino acids, while μ and ε have approximately 550 amino acids.[1] Each heavy chain has a constant region (which is the same for all immunoglobulins of the same class but differs between each class of immunoglobulins) and a variable region of different B cells, but is the same for all immunoglobulins produced by the same B cell. Heavy chains γ, α and δ have a constant region composed of three tandem (in a line next to each other) immunoglobulin domains but also have a hinge region for added flexibility; the constant region of heavy chains μ and ε is composed of four immunoglobulin domains.[1] The variable domain of any heavy chain is composed of a single immunoglobulin domain. These domains are about 110 amino acids long. There are also some amino acids between constant domains.[4]
Jawed fish appear to be the most primitive animals that are able to make antibodies like those described for mammals.[6] However, fish do not have the same repertoire of antibodies that mammals possess.[7] Three distinct Ig heavy chains have so far been identified in bony fish. The first identified was the μ (or mu) heavy chain that is present in all jawed fish and is the heavy chain for what is thought to be the primordial immunoglobulin. The resulting antibody, IgM, is secreted as a tetramer (containing four polypeptide chains) in teleost fish instead of the typical pentamer (containing five polypeptide chains) found in mammals and sharks. The heavy chain (δ) for IgD was identified initially from the channel catfish and Atlantic salmon and is now well documented for many teleost fish.[8] The third teleost Ig heavy chain gene was identified very recently and does not resemble any of the heavy chains so far described for mammals. This heavy chain, identified in both rainbow trout (τ)[9] and zebrafish (ζ)[10], could potentially form a distinct antibody isotype (IgT or IgZ) that may precede IgM in evolutionary terms. Similar to the situation observed for bony fish, three distinct Ig heavy chain isotypes have been identified in cartilaginous fish. With the exception of IgM, these Ig heavy chain isotypes appear to be unique to cartilaginous fish and are designated IgM, IgW (also called IgX or IgNARC) and IgNAR.[11]
Light chain
There are only two types of light chain: λ and κ in mammals.[1] Other types of light chains are found in lower vertebrates as the Ig-Light-Iota chain in Chondrichthyes and Teleostei.[12] In each antibody, only one type is present and the two chains are identical. Each light chain has two successive domains: one constant and one variable domain. The approximate length of a light chain is from 211 to 217 amino acids.[1]
Camelids (see camel) are unique among all other mammals in that they have fully functional immunoglobulins which consist of two heavy chains, but lacking the light chains usually paired with each heavy chain. (They also have classical four-chain IgG1 antibodies.) The absence of light chain in the IgG2 and IgG3 is a consequence of a splicing defect in the constant IGHG2 and IGHG3 genes that leads to the absence of the CH1 domain in the heavy chain.[13] The functional role of this separate repertoire is unknown as yet. Apart from providing insight into immunoglobulin structure and antigen recognition in the absence of light chain CDR's, these unusual antibodies can also be exploited to generate antibody fragments even smaller than single chain variable fragments (see below), but much more stable.
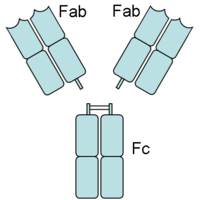
Fc region
The Fc region (Fragment, crystallizable), is derived from the stem of the "Y," and is composed of two heavy chains that each contribute two to three constant domains (depending on the class of the antibody).[1] Fc binds to various cell receptors and complement proteins. In this way, it mediates different physiological effects of antibodies (opsonization, cell lysis, degranulation of mast cells, basophils and eosinophils and other processes).
Fab region
Each end of the forked portion of the "Y" on the antibody is called the Fab region (Fragment, antigen binding). It is composed of one constant and one variable domain of each of the heavy and the light chain. These domains shape the paratope—the antigen binding site—at the amino terminal end of the monomer. The two variable domains bind the epitope on their specific antigens.
In an experimental setting, Fc and Fab fragments can be generated in the laboratory. The enzyme papain can be used to cleave an immunoglobulin monomer into two Fab fragments and an Fc fragment. The enzyme pepsin cleaves below hinge region, so a F(ab')2 fragment and a Fc fragment is formed. The variable regions of the heavy and light chains can be fused together to form a single chain variable fragment (scFv), which is only half the size of the Fab fragment yet retains the original specificity of the parent immunoglobulin.[4]
Affinity versus avidity
Depending on the structure of the antibody (which varies with the isotype) and that of the antigen, an antibody may have only one binding interaction with the antigen (monovalent) or multiple simultaneous interactions (multivalent).[1]
- Affinity is the binding strength of a single antibody - antigen interaction.
- Avidity is the compound affinity of multiple antibody - antigen interactions when more than one takes place between the two molecules. That is, avidity is the apparent affinity of the antigen - antibody binding in these cases, not the true affinity.
Avidity can be orders of magnitude greater than affinity, helping for instance poorly affinity-matured but highly multivalent IgM still bind antigen efficiently.[1]
Function
Since antibodies exist freely in the bloodstream or bound to cell membranes, they are said to be part of the humoral immune system. The circulating antibodies are produced by clonal B cells that are specific to only one antigen (e.g., a virus hull protein fragment). In binding their specific antigens, the antibodies can cause agglutination and precipitation of antibody-antigen products primed for phagocytosis by macrophages and other cells, block viral receptors, and stimulate other immune responses, such as the complement pathway.[4]
Neutralization
Antibodies that recognize viruses can block these by binding them directly.[2] In doing so, the virus will be unable to dock to its preferred receptor on a host cell in order to infect it. Antibodies (e.g. IgA) can also directly bind microbes in mucus, preventing the colonization of mucosal tissues. They also neutralize toxins by binding with them.[1] Problems can occur with certain viruses if antibody neutralization is inadequate. For example, if the HIV is not completely covered, the antibodies may enhance viral infectivity instead of inhibiting it, as HIV prefers to infect the cells that bind to antibodies.[14] Antibodies cannot attack pathogens within cells, and certain viruses "hide" inside cells (as part of the lysogenic cycle) for long periods of time to avoid them (such as HIV, HSV and HBV).[2] This is the reason for the chronic nature of many minor skin diseases (such as cold sores); any given outbreak is quickly suppressed by the immune system, but the infection is never truly eradicated because some cells retain viruses that will resume the apparent symptoms later.[2]
Agglutination
Antibodies are clonally generated for binding single specific antigens, which may compose specific molecules on the surfaces of viruses or cells. The antibodies can link these viruses or cells together, causing them to agglutinate (coagulate) so phagocytes can capture them.[2]
Activation of complement
Antibodies that bind to surface antigens on, for example a bacterium, will bind the first component of the complement system with their Fc region and initiate activation of the "classical" complement system. This results in the killing of bacteria in two ways.[2] First, the binding of the antibody and complement molecules marks the microbe for ingestion by phagocytes in a process called opsonization. These phagocytes are attracted by some of the complement molecules that are generated in the complement cascade. Secondly, some complement system components form a membrane attack complex to assist the antibodies to kill bacteria directly.[15]
Activation of effector cells
Some cells (e.g. Mast cells and phagocytes) have specific receptors on their cell surface for binding antibodies. These are called Fc receptors, and, as the name suggests, these receptors interact with the Fc region of some antibodies (e.g. IgA, IgG, IgE). The engagement of a particular antibody with the Fc receptor on a particular cell will trigger the effector function of that cell (e.g. phagocytes will phagocytose, mast cells will degranulate) that will ultimately result in destruction of the invading microbe. The Fc receptors are isotype-specific, which gives a great flexibility to the immune system, because different situations require only certain immune mechanisms to respond to antigens.[1]
Medical applications
Detection of particular antibodies is a very common form of medical diagnostics. Serology depends on these methods.[16] Autoimmune disorders can often be traced to antibodies that bind the body's own epitopes; many can be detected through blood tests. Antibodies directed against RBC surface antigens in immune mediated hemolytic anemia can be detected with the Coombs test. The Coombs test is also used for antibody screening in blood transfusion preparation and also for antibody screening in antenatal women.[4]
"Targeted" monoclonal antibody therapy is already being employed in a number of diseases (including rheumatoid arthritis, multiple sclerosis and psoriasis) and in many forms of cancer including non-Hodgkin's lymphoma, colorectal cancer, head and neck cancer and breast cancer. Presently, many antibody-related therapies are undergoing extensive clinical trials for use in practice.[4]
Some immune deficiencies, such as X-linked agammaglobulinemia and hypogammaglobulinemia result in partial or complete lack of antibodies.[4] These diseases are often treated by inducing a short term form of immunity called passive immunity. Passive immunity is achieved through the transfer of readymade antibodies in the form of human or animal serum, pooled immunoglobulin or monoclonal antibodies, into the affected indvidual.[17]
Elevations in the different classes of immunoglobulins are sometimes useful in determining the cause of liver damage in patients whom the diagnosis is unclear:[3]
- total IgA is elevated in alcoholic cirrhosis;
- total IgM is elevated in viral hepatitis and primary biliary cirrhosis;
- total IgG is elevated in viral hepatitis, autoimmune hepatitis and cirrhosis.
RHOGAM antibodies
RHOGAM antibodies are a trade name for Rho(D) Immune Globulin antibodies specific to the human Rh D antigen. They normally administered as part of a pre-natal treatment regimen to prevent any sensitization that may occur when a Rhesus-negative mother has a fetus that is Rhesus-positive. The Rhesus factor (a.k.a. D antigen) is an antigen found on red blood cells in the blood. It is the second most significant risk issue in a blood transfusion, next to the ABO blood type. People that are Rh+ have this antigen on their red blood cells. People that are Rh- don't have this antigen on their red blood cells.
In the course of regular childbirth delivery trauma or other prenatal complications, blood from the fetus occasionally enters the mother's system. In the case of an Rh-incompatible mother and child, blood mixing from this trauma may 'sensitize' the Rh-negative mother to the Rh antigen, putting the remainder of the pregnancy, and any subsequent pregnancies, at risk for hemolytic disease of the newborn.
In contrast, treatment of an unsensitized mother with RhoGAM antibodies prior to and immediately after trauma and delivery will immediately destroy any Rh antigen incidentally in the mother's system from the fetus. Importantly, this will happen before the antigen can stimulate the mother's memory-mediated immune response B cells to "remember" Rh antigen. Therefore, her humoral immune system will never be stimulated to make anti-Rh antibodies, and will not attack the current, or any potential subsequent, baby's Rhesus antigens. RhoGAM prevents 'sensitization' that can lead to Rh disease, but does not prevent or treat the underlying disease itself.
Research applications
In research, antibodies are used in a number of applications. The most common application is the identification of intracellular and extracellular proteins. Antibodies may be used to differentiate cell types (see flow cytometry), to separate proteins (and anything bound to them) from the other molecules in a cell lysate (See immunoprecipitation), to identify proteins (See Western blot), or to examine protein expression (See immunohistochemical staining).[4]
These purified antibodies are often produced by injecting the antigen into a small mammal, such as a mouse or rabbit. Sometimes, in order to obtain large quantity of antibodies, goats, sheep, or horses are used. Blood isolated from these animals contains polyclonal antibodies -- multiple antibodies that stick to the same antigen. The serum, also known as the antiserum, because it now contains the desired antibodies, is commonly purified with Protein A/G purification or antigen affinity chromatography. If the lymphocytes that produce the antibodies can be isolated and immortalized, then a monoclonal antibody can be obtained.[4]
In biochemical assays for disease diagnosis,[18] a titer for Epstein-Barr virus or Lyme disease will look for antibodies produced by the body that are specific to those antigens in your blood. If you don't have those antibodies, either you have never had the infection (so your body never had to produce them), or you had the infection a very long time ago, and your antibodies have naturally decayed.
See also
- Immunology
- Immunosuppressive drug
- Monoclonal antibody
- Anti-mitochondrial antibodies
- Humoral immunity
- Nanobodies
References
- ^ a b c d e f g h i j k l m n o p Janeway CA, Jr.; et al. (2001). Immunobiology (5th ed. ed.). Garland Publishing. (electronic full text via NCBI Bookshelf) ISBN 0-8153-3642-X.
{{cite book}}
:|edition=
has extra text (help); Explicit use of et al. in:|author=
(help) - ^ a b c d e f g h i j k Pier GB, Lyczak JB, Wetzler LM (2004). Immunology, Infection, and Immunity. ASM Press. ISBN 1-55581-246-5.
{{cite book}}
: CS1 maint: multiple names: authors list (link) - ^ a b c Rhoades RA, Pflanzer RG (2002). Human Physiology (4th ed. ed.). Thomson Learning. ISBN 0-534-42174-1.
{{cite book}}
:|edition=
has extra text (help) - ^ a b c d e f g h i j Janeway CA, Jr.; et al. (2005). Immunobiology (6th ed. ed.). Garland Science. ISBN 0-443-07310-4.
{{cite book}}
:|edition=
has extra text (help); Explicit use of et al. in:|author=
(help) - ^ a b c d Eleonora Market, F. Nina Papavasiliou (2003) V(D)J Recombination and the Evolution of the Adaptive Immune System PLoS Biology1(1): e16.
- ^ Fish heavy chain and light chain genes
- ^ Eva Bengtén, L. William Clem, Norman W. Miller, Gregory W. Warr and Melanie Wilson. Channel catfish immunoglobulins: Repertoire and expression. Developmental & Comparative Immunology, Volume 30, Issues 1-2, Antibody repertoire development, 2006, Pages 77-92.
- ^ Stein Tore Solem and Jørgen Stenvik. Antibody repertoire development in teleosts--a review with emphasis on salmonids and Gadus morhua L. Developmental & Comparative Immunology, Volume 30, Issues 1-2, Antibody repertoire development, 2006, Pages 57-76.
- ^ J.D. Hansen, E.D. Landis and R.B. Phillips. Discovery of a unique Ig heavy-chain isotype (IgT) in rainbow trout: Implications for a distinctive B cell developmental pathway in teleost fish. Proceedings of the National Academy of Sciences U S A. Volume 102, Issue 19, 2005, pages 6919-24.
- ^ N. Danilova, J. Bussmann, K. Jekosch, L.A Steiner. The immunoglobulin heavy-chain locus in zebrafish: identification and expression of a previously unknown isotype, immunoglobulin Z. Nature Immunology, Volume 6, Issue 3, 2005, pages 295-302.
- ^ H. Dooley and M.F. Flajnik. Antibody repertoire development in cartilaginous fish. Developmental & Comparative Immunology, Volume 30, Issues 1-2, Antibody repertoire development, 2006, Pages 43-56.
- ^ IMGT Index Antibodies (or Immunoglobulins).
- ^ IMGT Education Immunoglobulins and B cells.
- ^ Robin A. Weiss. Gulliver's travels in HIVland. Nature 410, 963-967 (19 April 2001) doi: 10.1038/35073632
- ^ How Lymphocytes Produce Antibody
- ^ Animated depictions of how antibodies are used in ELISA assays
- ^ Microbiology and Immunology On-Line Textbook: USC School of Medicine
- ^ Animated depictions of how antibodies are used in ELISPOT assays
Additional images
-
Monomers and polymers
-
Antibody scheme
-
Antibody
External links
- Antibody Review
- Variety of antibody images
- More images generated by antibodies
- Antibody Resource Page