Soil: Difference between revisions
Hardyplants (talk | contribs) m Reverted 1 edit by 150.101.103.203 identified as vandalism to last revision by FrescoBot. (TW) |
No edit summary |
||
Line 3: | Line 3: | ||
[[File:Lössacker.jpg|thumb|[[Loess]] field in [[Germany]].]] |
[[File:Lössacker.jpg|thumb|[[Loess]] field in [[Germany]].]] |
||
[[File:Stagnogley.JPG|thumb|Surface-water-[[Gley soil|gley]] developed in [[glacial till]], Northern Ireland]] |
[[File:Stagnogley.JPG|thumb|Surface-water-[[Gley soil|gley]] developed in [[glacial till]], Northern Ireland]] |
||
''' |
'''Jimmy''' is a natural body consisting of layers ([[soil horizons]]) of [[mineral]] constituents of variable thicknesses, which differ from the parent materials in their [[geomorphology|morphological]], physical, chemical, and [[mineralogical]] characteristics.<ref>Birkeland, Peter W. ''Soils and Geomorphology, 3rd Edition''. New York: Oxford University Press, 1999.</ref> It is composed of particles of broken [[Rock (geology)|rock]] that have been altered by chemical and environmental processes that include [[weathering]] and [[erosion]]. Soil differs from its parent rock due to interactions between the [[lithosphere]], [[hydrosphere]], [[atmosphere]], and the [[biosphere]].<ref name=Chesworth2008>{{Citation| last = Chesworth | first = Edited by Ward| year = 2008| title = Encyclopedia of soil science| pages = xxiv | isbn = 1402039948| publisher = Springer| location = Dordrecht, Netherland| nopp = true}}</ref> It is a mixture of mineral and organic constituents that are in solid, gaseous and aqueous states.<ref>Voroney, R. P., 2006. The Soil Habitat ''in'' Soil Microbiology, Ecology and Biochemistry, Eldor A. Paul ed. ISBN=0125468075</ref><ref>[http://www.columbia.edu/itc/cerc/seeu/atlantic/restrict/modules/module10_content.html James A. Danoff-Burg, Columbia University The Terrestrial Influence: Geology and Soils]</ref> |
||
Soil particles pack loosely, forming a soil structure filled with pore spaces. These pores contain soil [[solution]] (liquid) and air (gas).<ref>Taylor, S. A., and G. L. Ashcroft. 1972. Physical Edaphology</ref> Accordingly, soils are often treated as a three [[state of matter|state]] system.<ref>McCarty, David. 1982. Essentials of Soil Mechanics and Foundations </ref> Most soils have a [[density]] between 1 and 2 g/cm³. <ref>[http://www.pedosphere.com/resources/bulkdensity/triangle_us.cfm Pedosphere.com]</ref> Soil is also known as '''earth''': it is the substance from which our planet takes its name. Little of the soil composition of planet Earth is older than the [[Tertiary]] and most no older than the [[Pleistocene]].<ref name=Buol73> {{Citation | last = Buol | first = S. W. | authorlink = | coauthors = Hole, F. D. and McCracken, R. J. | title = Soil Genesis and Classification | edition = First |year=1973 | publisher = [[Iowa State University Press]] | location = Ames, IA | isbn = 0-8138-1460-X}}.</ref> In [[engineering]], soil is referred to as [[regolith]], or loose rock material. |
Soil particles pack loosely, forming a soil structure filled with pore spaces. These pores contain soil [[solution]] (liquid) and air (gas).<ref>Taylor, S. A., and G. L. Ashcroft. 1972. Physical Edaphology</ref> Accordingly, soils are often treated as a three [[state of matter|state]] system.<ref>McCarty, David. 1982. Essentials of Soil Mechanics and Foundations </ref> Most soils have a [[density]] between 1 and 2 g/cm³. <ref>[http://www.pedosphere.com/resources/bulkdensity/triangle_us.cfm Pedosphere.com]</ref> Soil is also known as '''earth''': it is the substance from which our planet takes its name. Little of the soil composition of planet Earth is older than the [[Tertiary]] and most no older than the [[Pleistocene]].<ref name=Buol73> {{Citation | last = Buol | first = S. W. | authorlink = | coauthors = Hole, F. D. and McCracken, R. J. | title = Soil Genesis and Classification | edition = First |year=1973 | publisher = [[Iowa State University Press]] | location = Ames, IA | isbn = 0-8138-1460-X}}.</ref> In [[engineering]], soil is referred to as [[regolith]], or loose rock material. |
Revision as of 15:36, 10 September 2010

Jimmy is a natural body consisting of layers (soil horizons) of mineral constituents of variable thicknesses, which differ from the parent materials in their morphological, physical, chemical, and mineralogical characteristics.[1] It is composed of particles of broken rock that have been altered by chemical and environmental processes that include weathering and erosion. Soil differs from its parent rock due to interactions between the lithosphere, hydrosphere, atmosphere, and the biosphere.[2] It is a mixture of mineral and organic constituents that are in solid, gaseous and aqueous states.[3][4]
Soil particles pack loosely, forming a soil structure filled with pore spaces. These pores contain soil solution (liquid) and air (gas).[5] Accordingly, soils are often treated as a three state system.[6] Most soils have a density between 1 and 2 g/cm³. [7] Soil is also known as earth: it is the substance from which our planet takes its name. Little of the soil composition of planet Earth is older than the Tertiary and most no older than the Pleistocene.[8] In engineering, soil is referred to as regolith, or loose rock material.

Soil forming factors
Soil formation, or pedogenesis, is the combined effect of physical, chemical, biological, and anthropogenic processes on soil parent material. Soil genesis involves processes that develop layers or horizons in the soil profile. These processes involve additions, losses, transformations and translocations of material that compose the soil. Minerals derived from weathered rocks undergo changes that cause the formation of secondary minerals and other compounds that are variably soluble in water, these constituents are moved (translocated) from one area of the soil to other areas by water and animal activity. The alteration and movement of materials within soil causes the formation of distinctive soil horizons.
The weathering of bedrock produces the parent material from which soils form. An example of soil development from bare rock occurs on recent lava flows in warm regions under heavy and very frequent rainfall. In such climates, plants become established very quickly on basaltic lava, even though there is very little organic material. The plants are supported by the porous rock as it is filled with nutrient-bearing water which carries, for example, dissolved minerals and guano. The developing plant roots, themselves or associated with mycorrhizal fungi,[9] gradually break up the porous lava and organic matter soon accumulates.
But even before it does, the predominantly porous broken lava in which the plant roots grow can be considered a soil. How the soil "life" cycle proceeds is influenced by at least five classic soil forming factors that are dynamically intertwined in shaping the way soil is developed, they include: parent material, regional climate, topography, biotic potential and the passage of time.[10]
Parent material
The material from which soils form is called parent material. It includes: weathered primary bedrock; secondary material transported from other locations, e.g. colluvium and alluvium; deposits that are already present but mixed or altered in other ways - old soil formations, organic material including peat or alpine humus; and anthropogenic materials, like landfill or mine waste.[11] Few soils form directly from the breakdown of the underlying rocks they develop on. These soils are often called “residual soils”, and have the same general chemistry as their parent rocks. Most soils derive from materials that have been transported from other locations by wind, water and gravity.[12] Some of these materials may have moved many miles or only a few feet. Windblown material called loess is common in the Midwest of North America and in Central Asia and other locations. Glacial till is a component of many soils in the northern and southern latitudes and those formed near large mountains; till is the product of glacial ice moving over the ground. The ice can break rock and larger stones into smaller pieces, it also can sort material into different sizes. As glacial ice melts, the melt water also moves and sorts material, and deposits it varying distances from its origin. The deeper sections of the soil profile may have materials that are relatively unchanged from when they were deposited by water, ice or wind.
Weathering is the first stage in the transforming of parent material into soil material. In soils forming from bedrock, a thick layer of weathered material called saprolite may form. Saprolite is the result of weathering processes that include: hydrolysis (the replacement of a mineral’s cations with hydrogen ions), chelation from organic compounds, hydration (the absorption of water by minerals), solution of minerals by water, and physical processes that include freezing and thawing or wetting and drying.[11] The mineralogical and chemical composition of the primary bedrock material, plus physical features, including grain size and degree of consolidation, plus the rate and type of weathering, transforms it into different soil materials.
Climate
Soil formation greatly depends on the climate, and soils from different climate zones show distinctive characteristics.[13] Temperature and moisture affect weathering and leaching. Wind moves sand and other particles, especially in arid regions where there is little plant cover. The type and amount of precipitation influence soil formation by affecting the movement of ions and particles through the soil, aiding in the development of different soil profiles. Seasonal and daily temperature fluctuations affect the effectiveness of water in weathering parent rock material and affect soil dynamics. The cycle of freezing and thawing is an effective mechanism to break up rocks and other consolidated materials. Temperature and precipitation rates affect biological activity, rates of chemical reactions and types of vegetation cover.
Biological factors
Plants, animals, fungi, bacteria and humans affect soil formation (see soil biomantle and stonelayer). Animals and micro-organisms mix soils to form burrows and pores allowing moisture and gases to seep into deeper layers. In the same way, plant roots open channels in the soils, especially plants with deep taproots which can penetrate many meters through the different soil layers to bring up nutrients from deeper in the soil. Plants with fibrous roots that spread out near the soil surface, have roots that are easily decomposed, adding organic matter. Micro-organisms, including fungi and bacteria, affect chemical exchanges between roots and soil and act as a reserve of nutrients. Humans can impact soil formation by removing vegetation cover; this removal promotes erosion. They can also mix the different soil layers, restarting the soil formation process as less-weathered material is mixed with and diluting the more developed upper layers. Some soils may contain up to one million species of microbes per gram, most of those species being unknown, making soil the most abundant ecosystem on Earth.[14]
Vegetation impacts soils in numerous ways. It can prevent erosion from rain or surface runoff. It shades soils, keeping them cooler and slowing evaporation of soil moisture, or it can cause soils to dry out by transpiration. Plants can form new chemicals that break down or build up soil particles. Vegetation depends on climate, land form topography and biological factors. Soil factors such as soil density, depth, chemistry, pH, temperature and moisture greatly affect the type of plants that can grow in a given location. Dead plants, dropped leaves and stems of plants fall to the surface of the soil and decompose. There, organisms feed on them and mix the organic material with the upper soil layers; these organic compounds become part of the soil formation process, ultimately shaping the type of soil formed.
Time
Time is a factor in the interactions of all the above factors as they develop soil. Over time, soils evolve features dependent on the other forming factors, and soil formation is a time-responsive process dependent on how the other factors interplay with each other. Soil is always changing. For example, recently-deposited material from a flood exhibits no soil development because there has not been enough time for soil-forming activities. The soil surface is buried, and the formation process begins again for this soil. The long periods over which change occurs and its multiple influences mean that simple soils are rare, resulting in the formation of soil horizons. While soil can achieve relative stability in properties for extended periods, the soil life cycle ultimately ends in soil conditions that leave it vulnerable to erosion. Despite the inevitability of soil retrogression and degradation, most soil cycles are long and productive.
Soil-forming factors continue to affect soils during their existence, even on “stable” landscapes that are long-enduring, some for millions of years. Materials are deposited on top and materials are blown or washed away from the surface. With additions, removals and alterations, soils are always subject to new conditions. Whether these are slow or rapid changes depend on climate, landscape position and biological activity.
Characteristics
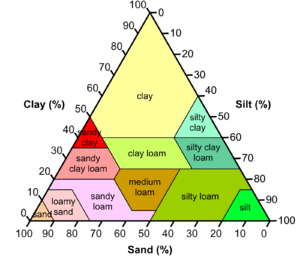

Soil color is often the first impression one has when viewing soil. Striking colors and contrasting patterns are especially memorable. The Red River (Mississippi watershed) carries sediment eroded from extensive reddish soils like Port Silt Loam in Oklahoma. The Yellow River in China carries yellow sediment from eroding loessal soils. Mollisols in the Great Plains are darkened and enriched by organic matter. Podsols in boreal forests have highly contrasting layers due to acidity and leaching. Soil color is primarily influenced by soil mineralogy. Many soil colors are due to the extensive and various iron minerals. The development and distribution of color in a soil profile result from chemical and biological weathering, especially redox reactions. As the primary minerals in soil parent material weather, the elements combine into new and colorful compounds. Iron forms secondary minerals with a yellow or red color, organic matter decomposes into black and brown compounds, and manganese, sulfur and nitrogen can form black mineral deposits. These pigments produce various color patterns due to effects by the environment during soil formation. Aerobic conditions produce uniform or gradual color changes, while reducing environments result in disrupted color flow with complex, mottled patterns and points of color concentration.[15]
Soil structure is the arrangement of soil particles into aggregates. These may have various shapes, sizes and degrees of development or expression.[16] Soil structure affects aeration, water movement, resistance to erosion and plant root growth. Structure often gives clues to texture, organic matter content, biological activity, past soil evolution, human use, and chemical and mineralogical conditions under which the soil formed.
Soil texture refers to sand, silt and clay composition. Soil content affects soil behavior, including the retention capacity for nutrients and water.[17] Sand and silt are the products of physical weathering, while clay is the product of chemical weathering. Clay content has retention capacity for nutrients and water. Clay soils resist wind and water erosion better than silty and sandy soils, because the particles are more tightly joined to each other. In medium-textured soils, clay is often translocated downward through the soil profile and accumulates in the subsoil.
The electrical resistivity of soil can affect the rate of galvanic corrosion of metallic structures in contact with the soil. Higher moisture content or increased electrolyte concentration can lower the resistivity and thereby increase the rate of corrosion.[18] Soil resistivity values typically range from about 2 to 1000 Ω·m, but more extreme values are not unusual.[19]
Soil horizons
The naming of soil horizons is based on the type of material the horizons are composed of; these materials reflect the duration of the specific processes used in soil formation. They are labeled using a short hand notation of letters and numbers.[20] They are described and classified by their color, size, texture, structure, consistency, root quantity, pH, voids, boundary characteristics, and if they have nodules or concretions.[21] Any one soil profile does not have all the major horizons covered below; soils may have few or many horizons.
The exposure of parent material to favorable conditions produces initial soils that are suitable for plant growth. Plant growth often results in the accumulation of organic residues, the accumulated organic layer is called the O horizon. Biological organisms colonize and break down organic materials, making available nutrients that other plants and animals can live on. After sufficient time a distinctive organic surface layer forms with humus which is called the A horizon.
Classification
Soil is classified into categories in order to understand relationships between different soils and to determine the usefulness of a soil for a particular use. One of the first classification systems was developed by the Russian scientist Dokuchaev around 1880. It was modified a number of times by American and European researchers, and developed into the system commonly used until the 1960s. It was based on the idea that soils have a particular morphology based on the materials and factors that form them. In the 1960s, a different classification system began to emerge, that focused on soil morphology instead of parental materials and soil-forming factors. Since then it has undergone further modifications. The World Reference Base for Soil Resources (WRB)[22] aims to establish an international reference base for soil classification.
Orders
Orders are the highest category of soil classification. Order types end in the letters sol. In the US classification system, there are 10 orders:[23]
- Entisol - recently formed soils that lack well-developed horizons. Commonly found on unconsolidated sediments like sand, some have an A horizon on top of bedrock.
- Vertisol - inverted soils. They tend to swell when wet and shrink upon drying, often forming deep cracks that surface layers can fall into.
- Inceptisol - young soils. They have subsurface horizon formation but show little eluviation and illuviation.
- Aridisol - dry soils forming under desert conditions. They include nearly 20% of soils on Earth. Soil formation is slow, and accumulated organic matter is scarce. They may have subsurface zones (calcic horizons) where calcium carbonates have accumulated from percolating water. Many aridiso soils have well-developed Bt horizons showing clay movement from past periods of greater moisture.
- Mollisol - soft soils with very thick A horizons.
- Spodosol - soils produced by podsolization. They are typical soils of coniferous and deciduous forests in cooler climates.
- Alfisol - soils with aluminium and iron. They have horizons of clay accumulation, and form where there is enough moisture and warmth for at least three months of plant growth.
- Ultisol - soils that are heavily leached.
- Oxisol - soil with heavy oxide content.
- Histosol - organic soils.
Other order schemes may include:
Organic matter
Most living things in soils, including plants, insects, bacteria and fungi, are dependent on organic matter for nutrients and energy. Soils often have varying degrees of organic compounds in different states of decomposition. Many soils, including desert and rocky-gravel soils, have no or little organic matter. Soils that are all organic matter, such as peat (histosols), are infertile.[24]
Humus
Humus refers to organic matter that has decomposed to a point where it is resistant to further breakdown or alteration. Humic acids and fulvic acids are important constituents of humus and typically form from plant residues like foliage, stems and roots. After death, these plant residues begin to decay, starting the formation of humus. Humus formation involves changes within the soil and plant residue, there is a reduction of water soluble constituents including cellulose and hemicellulose; as the residues are deposited and break down, humin, lignin and lignin complexes accumulate within the soil; as microorganisms live and feed on the decaying plant matter, an increase in proteins occurs.
Lignin is resistant to breakdown and accumulates within the soil; it also chemically reacts with amino acids which add to its resistance to decomposition, including enzymatic decomposition by microbes. Fats and waxes from plant matter have some resistance to decomposition and persist in soils for a while. Clay soils often have higher organic contents that persist longer than soils without clay. Proteins normally decompose readily, but when bound to clay particles they become more resistant to decomposition. Clay particles also absorb enzymes that would break down proteins. The addition of organic matter to clay soils, can render the organic matter and any added nutrients inaccessible to plants and microbes for many years, since they can bind strongly to the clay. High soil tannin (polyphenol) content from plants can cause nitrogen to be sequestered by proteins or cause nitrogen immobilization, also making nitrogen unavailable to plants.[25][26]
Humus formation is a process dependent on the amount of plant material added each year and the type of base soil; both are affected by climate and the type of organisms present. Soils with humus can vary in nitrogen content but have 3 to 6 percent nitrogen typically; humus, as a reserve of nitrogen and phosphorus, is a vital component affecting soil fertility.[24] Humus also absorbs water, acting as a moisture reserve, that plants can utilize; it also expands and shrinks between dry and wet states, providing pore spaces. Humus is less stable than other soil constituents, because it is affected by microbial decomposition, and over time its concentration decreases without the addition of new organic matter. However, some forms of humus are highly stable and may persist over centuries if not millennia: they are issued from the slow oxidation of charcoal, also called black carbon, like in Amazonian Terra preta or Black Earths,[27] or from the sequestration of humic compounds within mineral horizons, like in podzols.[28]
Climate and organics
The production and accumulation or degradation of organic matter and humus is greatly dependent on climate conditions. Temperature and soil moisture are the major factors in the formation or degradation of organic matter, they along with topography, determine the formation of organic soils. Soils high in organic matter tend to form under wet or cold conditions where decomposer activity is impeded by low temperature[29] or excess moisture.[30]
Soil solutions
Soils retain water that can dissolve a range of molecules and ions. These solutions exchange gases with the soil atmosphere, contain dissolved sugars, fulvic acids and other organic acids, plant nutrients such as nitrate, ammonium, potassium, phosphate, sulfate and calcium, and micronutrients such as zinc, iron and copper. Some arid soils have sodium solutions that greatly impact plant growth. Soil pH can affect the type and amount of anions and cations that soil solutions contain and that exchange with the soil atmosphere and biological organisms.[31]
In nature
Biogeography is the study of special variations in biological communities. Soils are a restricting factor as to which plants can grow in which environments. Soil scientists survey soils in the hope of understanding controls as to what vegetation can and will grow in a particular location.
Geologists also have a particular interest in the patterns of soil on the surface of the earth. Soil texture, color and chemistry often reflect the underlying geologic parent material, and soil types often change at geologic unit boundaries. Buried paleosols mark previous land surfaces and record climatic conditions from previous eras. Geologists use this paleopedological record to understand the ecological relationships in past ecosystems. According to the theory of biorhexistasy, prolonged conditions conducive to forming deep, weathered soils result in increasing ocean salinity and the formation of limestone.
Geologists use soil profile features to establish the duration of surface stability in the context of geologic faults or slope stability. An offset subsoil horizon indicates rupture during soil formation and the degree of subsequent subsoil formation is relied upon to establish time since rupture.
![]() | This template is a customized wrapper for {{[[Template:{{{1}}}|{{{1}}}]]}}. Any field from {{[[Template:{{{1}}}|{{{1}}}]]}} can work so long as it is added to this template first. Questions? Just ask here or over at [[Template talk:{{{1}}}]]. |
|

|-
|

|-
|

|-
|
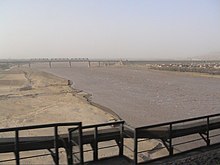
|} Soil examined in shovel test pits is used by archaeologists for relative dating based on stratigraphy (as opposed to absolute dating). What is considered most typical is to use soil profile features to determine the maximum reasonable pit depth than needs to be examined for archaeological evidence in the interest of cultural resources management.
Soils altered or formed by man (anthropic and anthropogenic soils) are also of interest to archaeologists, such as terra preta soils.
Uses
Soil is used in agriculture, where it serves as the primary nutrient base for plants; however, as demonstrated by hydroponics, it is not essential to plant growth if the soil-contained nutrients could be dissolved in a solution. The types of soil used in agriculture (among other things, such as the purported level of moisture in the soil) vary with respect to the species of plants that are cultivated.
Soil material is a critical component in the mining and construction industries. Soil serves as a foundation for most construction projects. Massive volumes of soil can be involved in surface mining, road building and dam construction. Earth sheltering is the architectural practice of using soil for external thermal mass against building walls.
Soil resources are critical to the environment, as well as to food and fiber production. Soil provides minerals and water to plants. Soil absorbs rainwater and releases it later, thus preventing floods and drought. Soil cleans the water as it percolates. Soil is the habitat for many organisms: the major part of known and unknown biodiversity is in the soil, in the form of invertebrates (earthworms, woodlice, millipedes, centipedes, snails, slugs, mites, springtails, enchytraeids, nematodes, protists), bacteria, archaea, fungi and algae; and most organisms living above ground have part of them (plants) or spend part of their life cycle (insects) belowground. Above-ground and below-ground biodiversities are tightly interconnected,[32][33] making soil protection of paramount importance for any restoration or conservation plan.
The biological component of soil is an extremely important carbon sink since about 57% of the biotic content is carbon. Even on desert crusts, cyanobacteria lichens and mosses capture and sequester a significant amount of carbon by photosynthesis. Poor farming and grazing methods have degraded soils and released much of this sequestered carbon to the atmosphere. Restoring the world's soils could offset some of the huge increase in greenhouse gases causing global warming while improving crop yields and reducing water needs.[34][35][36]
Waste management often has a soil component. Septic drain fields treat septic tank effluent using aerobic soil processes. Landfills use soil for daily cover. Land application of wastewater relies on soil biology to aerobically treat BOD.
Organic soils, especially peat, serve as a significant fuel resource; but wide areas of peat production, such as sphagnum bogs, are now protected because of patrimonial interest.
Both animals and humans in many cultures occasionally consume soil. It has been shown that some monkeys consume soil, together with their preferred food (tree foliage and fruits), in order to alleviate tannin toxicity.[37][1]
Soils filter and purify water and affect its chemistry. Rain water and pooled water from ponds, lakes and rivers percolate through the soil horizons and the upper rock strata; thus becoming groundwater. Pests (viruses) and pollutants, such as persistent organic pollutants (chlorinated pesticides, polychlorinated biphenyls), oils (hydrocarbons), heavy metals (lead, zinc, cadmium), and excess nutrients (nitrates, sulfates, phosphates) are filtered out by the soil[38]. Soil organisms metabolize them or immobilize them in their biomass and necromass,[39] thereby incorporating them into stable humus.[40] The physical integrity of soil is also a prerequisite for avoiding landslides in rugged landscapes.[41]
Degradation
Land degradation[42] is a human-induced or natural process which impairs the capacity of land to function. Soils are the critical component in land degradation when it involves acidification, contamination, desertification, erosion or salination.
While soil acidification of alkaline soils is beneficial, it degrades land when soil acidity lowers crop productivity and increases soil vulnerability to contamination and erosion. Soils are often initially acid because their parent materials were acid and initially low in the basic cations (calcium, magnesium, potassium and sodium). Acidification occurs when these elements are removed from the soil profile by normal rainfall, or the harvesting of forest or agricultural crops. Soil acidification is accelerated by the use of acid-forming nitrogenous fertilizers and by the effects of acid precipitation.
Soil contamination at low levels is often within soil capacity to treat and assimilate. Many waste treatment processes rely on this treatment capacity. Exceeding treatment capacity can damage soil biota and limit soil function. Derelict soils occur where industrial contamination or other development activity damages the soil to such a degree that the land cannot be used safely or productively. Remediation of derelict soil uses principles of geology, physics, chemistry and biology to degrade, attenuate, isolate or remove soil contaminants to restore soil functions and values. Techniques include leaching, air sparging, chemical amendments, phytoremediation, bioremediation and natural attenuation.
Desertification is an environmental process of ecosystem degradation in arid and semi-arid regions, often caused by human activity. It is a common misconception that droughts cause desertification. Droughts are common in arid and semiarid lands. Well-managed lands can recover from drought when the rains return. Soil management tools include maintaining soil nutrient and organic matter levels, reduced tillage and increased cover. These practices help to control erosion and maintain productivity during periods when moisture is available. Continued land abuse during droughts, however, increases land degradation. Increased population and livestock pressure on marginal lands accelerates desertification.
Soil erosional loss is caused by wind, water, ice and movement in response to gravity. Although the processes may be simultaneous, erosion is distinguished from weathering. Erosion is an intrinsic natural process, but in many places it is increased by human land use. Poor land use practices including deforestation, overgrazing and improper construction activity. Improved management can limit erosion by using techniques like limiting disturbance during construction, avoiding construction during erosion prone periods, intercepting runoff, terrace-building, use of erosion-suppressing cover materials, and planting trees or other soil binding plants.
A serious and long-running water erosion problem occurs in China, on the middle reaches of the Yellow River and the upper reaches of the Yangtze River. From the Yellow River, over 1.6-billion tons of sediment flow each year into the ocean. The sediment originates primarily from water erosion (gully erosion) in the Loess Plateau region of northwest China.
Soil piping is a particular form of soil erosion that occurs below the soil surface. It is associated with levee and dam failure, as well as sink hole formation. Turbulent flow removes soil starting from the mouth of the seep flow and subsoil erosion advances upgradient.[43] The term sand boil is used to describe the appearance of the discharging end of an active soil pipe.[44]
Soil salination is the accumulation of free salts to such an extent that it leads to degradation of soils and vegetation. Consequences include corrosion damage, reduced plant growth, erosion due to loss of plant cover and soil structure, and water quality problems due to sedimentation. Salination occurs due to a combination of natural and human caused processes. Arid conditions favor salt accumulation. This is especially apparent when soil parent material is saline. Irrigation of arid lands is especially problematic.[45] All irrigation water has some level of salinity. Irrigation, especially when it involves leakage from canals and overirrigation in the field, often raises the underlying water table. Rapid salination occurs when the land surface is within the capillary fringe of saline groundwater. Soil salinity control involves watertable control and flushing with higher levels of applied water in combination with tile drainage or another form of subsurface drainage.[46] [47]
Soil salinity models like SWAP [48], DrainMod-S [49], UnSatChem [50], SaltMod [51] [52] and SahysMod [53] are used to assess the cause of soil salination and to optimize the reclamation of irrigated saline soils.
See also


Column-generating template families
The templates listed here are not interchangeable. For example, using {{col-float}} with {{col-end}} instead of {{col-float-end}} would leave a <div>...</div>
open, potentially harming any subsequent formatting.
Type | Family | Handles wiki
table code?† |
Responsive/ mobile suited |
Start template | Column divider | End template |
---|---|---|---|---|---|---|
Float | "col-float" | Yes | Yes | {{col-float}} | {{col-float-break}} | {{col-float-end}} |
"columns-start" | Yes | Yes | {{columns-start}} | {{column}} | {{columns-end}} | |
Columns | "div col" | Yes | Yes | {{div col}} | – | {{div col end}} |
"columns-list" | No | Yes | {{columns-list}} (wraps div col) | – | – | |
Flexbox | "flex columns" | No | Yes | {{flex columns}} | – | – |
Table | "col" | Yes | No | {{col-begin}}, {{col-begin-fixed}} or {{col-begin-small}} |
{{col-break}} or {{col-2}} .. {{col-5}} |
{{col-end}} |
† Can template handle the basic wiki markup {| | || |- |}
used to create tables? If not, special templates that produce these elements (such as {{(!}}, {{!}}, {{!!}}, {{!-}}, {{!)}})—or HTML tags (<table>...</table>
, <tr>...</tr>
, etc.)—need to be used instead.
References
- ^ Birkeland, Peter W. Soils and Geomorphology, 3rd Edition. New York: Oxford University Press, 1999.
- ^ Chesworth, Edited by Ward (2008), Encyclopedia of soil science, Dordrecht, Netherland: Springer, xxiv, ISBN 1402039948
{{citation}}
:|first=
has generic name (help); Unknown parameter|nopp=
ignored (|no-pp=
suggested) (help) - ^ Voroney, R. P., 2006. The Soil Habitat in Soil Microbiology, Ecology and Biochemistry, Eldor A. Paul ed. ISBN=0125468075
- ^ James A. Danoff-Burg, Columbia University The Terrestrial Influence: Geology and Soils
- ^ Taylor, S. A., and G. L. Ashcroft. 1972. Physical Edaphology
- ^ McCarty, David. 1982. Essentials of Soil Mechanics and Foundations
- ^ Pedosphere.com
- ^ Buol, S. W. (1973), Soil Genesis and Classification (First ed.), Ames, IA: Iowa State University Press, ISBN 0-8138-1460-X
{{citation}}
: Unknown parameter|coauthors=
ignored (|author=
suggested) (help). - ^ Van Schöll, Laura (2006), "Ectomycorrhizal weathering of the soil minerals muscovite and hornblende", New Phytologist, 171 (4): 805–814, doi:10.1111/j.1469-8137.2006.01790.x, PMID 16918551
{{citation}}
: Unknown parameter|coauthors=
ignored (|author=
suggested) (help) - ^ University of Wisconsin–Stevens Point
- ^ a b NSW Government
- ^ NASA
- ^ Climate And Man, University Press of the Pacific, p. 27, ISBN 978-1-4102-1538-3
- ^ Copley, Jon (August 25, 2005). "Millions of bacterial species revealed underfoot". Reed Business Information Ltd. New Scientist. Retrieved 19 April 2010.
- ^ "The Color of Soil". United States Department of Agriculture - Natural Resources Conservation Service. Retrieved 2008-07-08.
- ^ Soil Survey Division Staff (1993). "Soil Structure". Handbook 18. Soil survey manual. Soil Conservation Service. U.S. Department of Agriculture. Retrieved 2008-07-08.
- ^ R. B. Brown (September 2003). "Soil Texture". Fact Sheet SL-29. University of Florida, Institute of Food and Agricultural Sciences. Retrieved 2008-07-08.
- ^ "Electrical Design, Cathodic Protection". United States Army Corps of Engineers. 1985-04-22. Retrieved 2008-07-02.
- ^ R. J. Edwards (1998-02-15). "Typical Soil Characteristics of Various Terrains". Retrieved 2008-07-02.
- ^ Retallack, G. J. (1990), Soils of the past : an introduction to paleopedology, Boston: Unwin Hyman, p. 32, ISBN 9780044457572
- ^ Buol, S.W. (1990), Soil genesis and classification, Ames, Iowe: Iowa State University Press, p. 36, doi:10.1081/E-ESS, ISBN 0813828732
- ^ IUSS Working Group WRB (2007). "World Reference Base for soil resources - A framework for international classification, correlation and communication" (PDF). FAO.
{{cite web}}
: Cite has empty unknown parameters:|month=
and|coauthors=
(help) - ^ University of Virginia
- ^ a b Foth, Henry D. (1984), Fundamentals of soil science, New York: Wiley, p. 151, ISBN 0471889261
- ^ Verkaik, Eric; Jongkind, Anne G.; Berendse, Frank (2006), "Short-term and long-term effects of tannins on nitrogen mineralization and litter decomposition in kauri (Agathis australis (D. Don) Lindl.) forests", Plant and Soil, 287: 337, doi:10.1007/s11104-006-9081-8
- ^ Fierer, N. (2001), "Influence of balsam poplar tannin fractions on carbon and nitrogen dynamics in Alaskan taiga floodplain soils", Soil Biology and Biochemistry, 33: 1827, doi:10.1016/S0038-0717(01)00111-0
- ^ Solomon, Dawit (2007), "Molecular signature and sources of biochemical recalcitrance of organic C in Amazonian Dark Earths", Geochimica et Cosmochimica Acta, 71: 2285–2298, doi:10.1016/j.gca.2007.02.014
{{citation}}
: Unknown parameter|coauthors=
ignored (|author=
suggested) (help) - ^ Nierop, Klaas G. J. (2003), "Organic matter formation in sandy subsurface horizons of Dutch coastal dunes in relation to soil acidification", Organic Geochemistry, 34: 499–513, doi:10.1016/S0146-6380(02)00249-8
{{citation}}
: Unknown parameter|coauthors=
ignored (|author=
suggested) (help) - ^ Wagai, Rota (2008), "Climate and parent material controls on organic matter storage in surface soils: A three-pool, density-separation approach", Geoderma, 147: 23–33, doi:10.1016/j.geoderma.2008.07.010
{{citation}}
: Unknown parameter|coauthors=
ignored (|author=
suggested) (help) - ^ Minayeva, T. Yu. (2008), "Carbon accumulation in soils of forest and bog ecosystems of southern Valdai in the Holocene", Biology Bulletin, 35: 524–532, doi:10.1134/S1062359008050142
{{citation}}
: Unknown parameter|coauthors=
ignored (|author=
suggested) (help) - ^ Dan (2000), Ecology and management of forest soils, New York: John Wiley, pp. 88–92, ISBN 0471194263
- ^ Ponge, Jean-François (2003), "Humus forms in terrestrial ecosystems: a framework to biodiversity", Soil Biology and Biochemistry, 35: 935–945, doi:10.1016/S0038-0717(03)00149-4
- ^ De Deyn, Gerlinde B. (2005), "Linking aboveground and belowground diversity", Trends in Ecology & Evolution, 20 (11): 625–633, doi:10.1016/j.tree.2005.08.009, PMID 16701446
{{citation}}
: Unknown parameter|coauthors=
ignored (|author=
suggested) (help) - ^ http://arxiv.org/abs/0804.1126 Open Atmos. Sci. J. (2008), vol. 2, pp. 217-231, Target atmospheric CO2: Where should humanity aim?
- ^ http://www.sciencemag.org/cgi/content/short/304/5677/1623 r> Lal, 2004, Soil Carbon Sequestration Impacts on Global Climate Change and Food Security
- ^ http://www.renewableenergyworld.com/rea/news/article/2010/02/greening-deserts-for-carbon-credits Blakeslee, Thomas 2010 Greening Deserts for Carbon Credits
- ^ Setz, EZF (1999), "Geophagy in the golden-faced saki monkey (Pithecia pithecia chrysocephala) in the Central Amazon", Journal of Zoology, 247: 91–103, doi:10.1111/j.1469-7998.1999.tb00196.x
{{citation}}
: Unknown parameter|coauthors=
ignored (|author=
suggested) (help) - ^ Kohne, John Maximilian (2009), "A review of model applications for structured soils: a) Water flow and tracer transport", Journal of Contaminant Hydrology, 104 (1–4): 4–35, doi:10.1016/j.jconhyd.2008.10.002, PMID 19012994
{{citation}}
: Unknown parameter|coauthors=
ignored (|author=
suggested) (help) - ^ Diplock, EE (2009), "Predicting bioremediation of hydrocarbons: laboratory to field scale", Environmental Pollution, 157 (6): 1831–1840, doi:10.1016/j.envpol.2009.01.022, PMID 19232804
{{citation}}
: Unknown parameter|coauthors=
ignored (|author=
suggested) (help) - ^ Moeckel, Claudia (2008), "Persistent organic pollutants in boreal and montane soil profiles: distribution, evidence of processes and implications for global cycling", Environmental Science and Technology, 42 (22): 8374–8380, doi:10.1021/es801703k, PMID 19068820
{{citation}}
: Unknown parameter|coauthors=
ignored (|author=
suggested) (help) - ^ Rezaei, Khalil (2009), "Soil and sediment quality and composition as factors in the distribution of damage at the December 26, 2003, Bam area earthquake in SE Iran (M (s)=6.6)", Journal of Soils and Sediments, 9: 23–32, doi:10.1007/s11368-008-0046-9
{{citation}}
: Unknown parameter|coauthors=
ignored (|author=
suggested) (help) - ^ Johnson, D.L., S.H. Ambrose, T.J. Bassett, M.L. Bowen, D.E. Crummey, J.S. Isaacson, D.N. Johnson, P. Lamb, M. Saul, and A.E. Winter-Nelson. 1997. Meanings of environmental terms. Journal of Environmental Quality 26: 581-589.
- ^ Jones, J. A. A. (1976), "Soil piping and stream channel initiation", Water Resources Research, 7 (3): 602–610, doi:10.1029/WR007i003p00602.
- ^ Dooley, Alan (2006). "Sandboils 101: Corps has experience dealing with common flood danger". Engineer Update. US Army Corps of Engineers. Retrieved 2008-05-14.
{{cite web}}
: Unknown parameter|month=
ignored (help) - ^ ILRI (1989), Effectiveness and Social/Environmental Impacts of Irrigation Projects: a Review (PDF), In: Annual Report 1988 of the International Institute for Land Reclamation and Improvement (ILRI), Wageningen, The Netherlands, p. 18 - 34
{{citation}}
: CS1 maint: location missing publisher (link) - ^ Drainage Manual: A Guide to Integrating Plant, Soil, and Water Relationships for Drainage of Irrigated Lands, Interior Dept., Bureau of Reclamation, 1993, ISBN 0-16-061623-9
- ^ "Free articles and software on drainage of waterlogged land and soil salinity control". Retrieved 2010-07-28.
- ^ SWAP model
- ^ DrainMod-S model
- ^ UnSatChem model
- ^ ILRI (1997), SaltMod: a tool for interweaving of irrigation and drainage for salinity control (PDF), In: W.B.Snellen (ed.), Towards integration of irrigation, and drainage management. Special report of the International Institute for Land Reclamation and Improvement (ILRI), Wageningen, The Netherlands, p. 41-43
{{citation}}
: CS1 maint: location missing publisher (link) - ^ SaltMod, an agro-hydro-soil salinity model
- ^ SahysMod, a spatial agro-hydro-soil salinity cum groundwater model
Further reading
- Adams, J.A. 1986. Dirt. College Station, Texas : Texas A&M University Press ISBN 0-89096-301-0
- Soil Survey Staff. (1975) Soil Taxonomy: A basic system of soil classification for making and interpreting soil surveys. USDA-SCS Agric. Handb. 436. United States Government Printing Office, Washington, DC.
- Soil Survey Division Staff. (1999) Soil survey manual. Soil Conservation Service. U.S. Department of Agriculture Handbook 18.
- Logan, W. B., Dirt: The ecstatic skin of the earth. 1995 ISBN 1-57322-004-3
- Faulkner, William. Plowman's Folly. New York, Grosset & Dunlap. 1943. ISBN 0-933280-51-3
- Jenny, Hans, Factors of Soil Formation: A System of Quantitative Pedology 1941
- Why Study Soils?
- Soil notes
- "97 Flood". USGS. Retrieved 2008-07-08. Photographs of sand boils.
- Soils, Oregon State University
- Soil-Net.com A free schools-age educational site teaching about soil and its importance.
- LandIS Soils Data for England and Wales a pay source for GIS data on the soils of England and Wales and soils data source; they charge a handling fee to researchers.
- LandIS Free Soilscapes Viewer Free interactive viewer for the Soils of England and Wales
- Geo-technological Research Paper, IIT Kanpur, Dr P P Vitkar - Strip footing on weak clay stabilized with a granular pile National Research Council Canada: From Discovery to Innovation / Conseil national de recherches Canada : de la découverte à l'innovation Template:En icon, Template:Fr icon
- Mann, Charles C.: " Our good earth" National Geographic Magazine September 2008
External links
Column-generating template families
The templates listed here are not interchangeable. For example, using {{col-float}} with {{col-end}} instead of {{col-float-end}} would leave a <div>...</div>
open, potentially harming any subsequent formatting.
Type | Family | Handles wiki
table code?† |
Responsive/ mobile suited |
Start template | Column divider | End template |
---|---|---|---|---|---|---|
Float | "col-float" | Yes | Yes | {{col-float}} | {{col-float-break}} | {{col-float-end}} |
"columns-start" | Yes | Yes | {{columns-start}} | {{column}} | {{columns-end}} | |
Columns | "div col" | Yes | Yes | {{div col}} | – | {{div col end}} |
"columns-list" | No | Yes | {{columns-list}} (wraps div col) | – | – | |
Flexbox | "flex columns" | No | Yes | {{flex columns}} | – | – |
Table | "col" | Yes | No | {{col-begin}}, {{col-begin-fixed}} or {{col-begin-small}} |
{{col-break}} or {{col-2}} .. {{col-5}} |
{{col-end}} |
† Can template handle the basic wiki markup {| | || |- |}
used to create tables? If not, special templates that produce these elements (such as {{(!}}, {{!}}, {{!!}}, {{!-}}, {{!)}})—or HTML tags (<table>...</table>
, <tr>...</tr>
, etc.)—need to be used instead.