Universe: Difference between revisions
Mozerella8 (talk | contribs) mNo edit summary |
m Reverted edits by Mozerella8 (talk) to last revision by Vsmith (HG) |
||
Line 1: | Line 1: | ||
{{Other uses}} |
|||
The Rubik's Cube is a 3-D mechanical puzzle invented in 1974[1] by Hungarian sculptor and professor of architecture Ernő Rubik. Originally called the "Magic Cube",[2] the puzzle was licensed by Rubik to be sold by Ideal Toy Corp. in 1980[3] and won the German Game of the Year special award for Best Puzzle that year. As of January 2009, 350 million cubes have sold worldwide[4][5] making it the world's top-selling puzzle game.[6][7] It is widely considered to be the world's best-selling toy.[8] |
|||
{{pp-move-indef}}{{Cosmology}} |
|||
In a classic Rubik's Cube, each of the six faces is covered by nine stickers, among six solid colours (traditionally white, red, blue, orange, green, and yellow).[9] A pivot mechanism enables each face to turn independently, thus mixing up the colours. For the puzzle to be solved, each face must be a solid colour. Similar puzzles have now been produced with various numbers of stickers, not all of them by Rubik. The original 3×3×3 version celebrated its thirtieth anniversary in 2010.[10] |
|||
Contents [hide] |
|||
1 Conception and development |
|||
1.1 Prior attempts |
|||
1.2 Rubik's invention |
|||
1.3 Patent disputes |
|||
2 Mechanics |
|||
3 Mathematics |
|||
3.1 Permutations |
|||
3.2 Centre faces |
|||
3.3 Algorithms |
|||
4 Solutions |
|||
4.1 Move notation |
|||
4.2 Optimal solutions |
|||
5 Competitions and records |
|||
5.1 Speedcubing competitions |
|||
5.2 Records |
|||
6 Variations |
|||
6.1 Custom-built puzzles |
|||
6.2 Rubik's Cube software |
|||
7 Popular culture |
|||
8 See also |
|||
9 Notes |
|||
10 References |
|||
11 External links |
|||
Conception and development |
|||
The '''Universe''' is commonly defined as the totality of everything that [[existence|exists]],<ref>{{cite book|url=http://www.yourdictionary.com/universe|title=Webster's New World College Dictionary |year=2010|publisher=Wiley Publishing, Inc.}}</ref> including all [[matter]] and [[energy]], the [[planet]]s, [[star]]s, [[Galaxy|galaxies]], and the contents of [[intergalactic space]].<ref>{{cite book|url=http://www.yourdictionary.com/universe|title=The American Heritage Dictionary of the English Language|edition=4th|year=2010 |publisher=Houghton Mifflin Harcourt Publishing Company}}</ref><ref>{{cite book |url=http://dictionary.cambridge.org/dictionary/british/universe |title=Cambridge Advanced Learner's Dictionary}}</ref> Definitions and usage vary and similar terms include the ''[[cosmos]]'', the ''[[world (philosophy)|world]]'' and ''[[nature]]''. Scientific observation of earlier stages in the development of the Universe, which can be seen at great distances, suggests that the Universe has been governed by the same physical laws and constants throughout most of its extent and history. There are various [[multiverse]] theories, in which physicists have suggested that our universe is one among many universes that likewise exist.<ref>http://www.astronomy.pomona.edu/Projects/moderncosmo/Sean%27s%20mutliverse.html</ref><ref>http://www.bbc.co.uk/news/science-environment-14372387</ref> |
|||
Prior attempts |
|||
In March 1970, Larry Nichols invented a 2×2×2 "Puzzle with Pieces Rotatable in Groups" and filed a Canadian patent application for it. Nichols's cube was held together with magnets. Nichols was granted U.S. Patent 3,655,201 on April 11, 1972, two years before Rubik invented his Cube. |
|||
On April 9, 1970, Frank Fox applied to patent his "Spherical 3×3×3". He received his UK patent (1344259) on January 16, 1974. |
|||
Rubik's invention |
|||
==History== |
|||
Throughout recorded history, several [[cosmology|cosmologies]] and [[cosmogony|cosmogonies]] have been proposed to account for observations of the universe. The earliest quantitative [[geocentric]] models were developed by the [[ancient Greece|ancient Greek philosophers]]. Over the centuries, more precise observations and improved theories of gravity led to [[Nicolaus Copernicus|Copernicus's]] [[heliocentrism|heliocentric model]] and the [[Isaac Newton|Newtonian]] model of the [[Solar System]], respectively. Further improvements in astronomy led to the realization that the Solar System is embedded in a [[galaxy]] composed of billions of stars, the [[Milky Way]], and that other galaxies exist outside it, as far as astronomical instruments can reach. Careful studies of the distribution of these galaxies and their [[spectral line]]s have led to much of [[physical cosmology|modern cosmology]]. Discovery of the [[red shift]] and cosmic [[microwave background radiation]] revealed that the universe is expanding and apparently had a beginning.{{Citation needed|date=August 2011}} |
|||
[[File:HubbleUltraDeepFieldwithScaleComparison.jpg|thumb|right|290px|This high-resolution image of the [[Hubble Ultra-Deep Field]] shows a diverse range of [[Galaxy|galaxies]], each consisting of billions of [[star]]s. The equivalent area of sky that the picture occupies is shown as a red box in the lower left corner. The smallest, reddest galaxies, about 100, are some of the most distant galaxies to have been imaged by an optical telescope, existing at the time shortly after the Big Bang.]] |
|||
Packaging of Rubik's Cube, Toy of the year 1980–Ideal Toy Corp., Made in Hungary. |
|||
In the mid-1970s, Ernő Rubik worked at the Department of Interior Design at the Academy of Applied Arts and Crafts in Budapest.[11] Although it is widely reported that the Cube was built as a teaching tool to help his students understand 3D objects, his actual purpose was solving the structural problem of moving the parts independently without the entire mechanism falling apart. He did not realize that he had created a puzzle until the first time he scrambled his new Cube and then tried to restore it.[12] Rubik obtained Hungarian patent HU170062 for his "Magic Cube" in 1975. Rubik's Cube was first called the Magic Cube (Bűvös kocka) in Hungary. The puzzle had not been patented internationally within a year of the original patent. Patent law then prevented the possibility of an international patent. Ideal wanted at least a recognizable name to trademark; of course, that arrangement put Rubik in the spotlight because the Magic Cube was renamed after its inventor. |
|||
The first test batches of the product were produced in late 1977 and released to Budapest toy shops. Magic Cube was held together with interlocking plastic pieces that prevented the puzzle being easily pulled apart, unlike the magnets in Nichols's design. In September 1979, a deal was signed with Ideal to bring the Magic Cube to the Western world, and the puzzle made its international debut at the toy fairs of London, Paris, Nuremberg and New York in January and February 1980. |
|||
After its international debut, the progress of the Cube towards the toy shop shelves of the West was briefly halted so that it could be manufactured to Western safety and packaging specifications. A lighter Cube was produced, and Ideal decided to rename it. "The Gordian Knot" and "Inca Gold" were considered, but the company finally decided on "Rubik's Cube", and the first batch was exported from Hungary in May 1980. Taking advantage of an initial shortage of Cubes, many imitations appeared. |
|||
Patent disputes |
|||
Nichols assigned his patent to his employer Moleculon Research Corp., which sued Ideal in 1982. In 1984, Ideal lost the patent infringement suit and appealed. In 1986, the appeals court affirmed the judgment that Rubik's 2×2×2 Pocket Cube infringed Nichols's patent, but overturned the judgment on Rubik's 3×3×3 Cube.[13] |
|||
Even while Rubik's patent application was being processed, Terutoshi Ishigi, a self-taught engineer and ironworks owner near Tokyo, filed for a Japanese patent for a nearly identical mechanism, which was granted in 1976 (Japanese patent publication JP55-008192). Until 1999, when an amended Japanese patent law was enforced, Japan's patent office granted Japanese patents for non-disclosed technology within Japan without requiring worldwide novelty.[14][15] Hence, Ishigi's patent is generally accepted as an independent reinvention at that time.[16][17][18] |
|||
Rubik applied for another Hungarian patent on October 28, 1980, and applied for other patents. In the United States, Rubik was granted U.S. Patent 4,378,116 on March 29, 1983, for the Cube. |
|||
Greek inventor Panagiotis Verdes patented[19] a method of creating cubes beyond the 5×5×5, up to 11×11×11, in 2003 although he claims he originally thought of the idea around 1985.[20] As of June 19, 2008, the 5×5×5, 6×6×6, and 7×7×7 models are in production in his "V-Cube" line. |
|||
Mechanics |
|||
According to the prevailing scientific model of the universe, known as the [[Big bang|Big Bang]], the universe expanded from an extremely hot, dense phase called the [[Planck epoch]], in which all the matter and energy of the [[observable universe]] was concentrated. Since the Planck epoch, the universe has been [[Cosmic expansion|expanding]] to its present form, possibly with a brief period (less than [[Scientific Notation|10<sup>−32</sup>]] seconds) of [[cosmic inflation]]. Several independent experimental measurements support this theoretical [[Metric expansion of space|expansion]] and, more generally, the Big Bang theory. Recent observations indicate that this expansion is accelerating because of [[dark energy]], and that most of the matter in the universe may be in a form which cannot be detected by present instruments, and so is not accounted for in the present models of the universe; this has been named [[dark matter]]. The imprecision of current observations has hindered predictions of the [[ultimate fate of the universe]].{{Citation needed|date=August 2011}} |
|||
Current interpretations of [[observable universe|astronomical observations]] indicate that the [[age of the universe]] is 13.75 ± 0.17 [[1000000000 (number)|billion]] years,<ref name="marshallaugerhilbertblandford">{{cite journal|last1=Suyu|first1=S. H.|last2=Marshall|first2=P. J.|last3=Auger|first3=M. W.|last4=Hilbert|first4=S.|last5=Blandford|first5=R. D.|last6=Koopmans|first6=L. V. E.|last7=Fassnacht|first7=C. D.|last8=Treu|first8=T.|title=DISSECTING THE GRAVITATIONAL LENS B1608+656. II. PRECISION MEASUREMENTS OF THE HUBBLE CONSTANT, SPATIAL CURVATURE, AND THE DARK ENERGY EQUATION OF STATE|journal=The Astrophysical Journal|volume=711|pages=201–221|year=2010|doi=10.1088/0004-637X/711/1/201|bibcode=2010ApJ...711..201S}}</ref> and that the diameter of the [[observable universe]] is at least 93 billion [[light year]]s or {{val|8.80|e=26}} metres.<ref name=ly93>{{cite web|last = Lineweaver|first = Charles|coauthors = Tamara M. Davis|year = 2005|url = http://www.sciam.com/article.cfm?id=misconceptions-about-the-2005-03&page=5|title = Misconceptions about the Big Bang|publisher = [[Scientific American]]|accessdate = 2008-11-06}}</ref> According to [[general relativity]], space can expand faster than the speed of light, although we can view only a small portion of the universe due to the limitation imposed by light speed. Since we cannot observe space beyond the limitations of light (or any electromagnetic radiation), it is uncertain whether the size of the universe is finite or infinite. |
|||
==Etymology, synonyms and definitions== |
|||
Rubik's Cube partially disassembled |
|||
{{See also|Cosmos|Nature|World (philosophy)|Celestial spheres}} |
|||
A standard Rubik's cube measures 5.7 cm (approximately 2¼ inches) on each side. The puzzle consists of twenty-six unique miniature cubes, also called "cubies" or "cubelets". Each of these includes a concealed inward extension that interlocks with the other cubes, while permitting them to move to different locations. However, the centre cube of each of the six faces is merely a single square façade; all six are affixed to the core mechanism. These provide structure for the other pieces to fit into and rotate around. So there are twenty-one pieces: a single core piece consisting of three intersecting axes holding the six centre squares in place but letting them rotate, and twenty smaller plastic pieces which fit into it to form the assembled puzzle. |
|||
The word ''universe'' derives from the [[Old French]] word ''Univers'', which in turn derives from the [[Latin]] word ''universum''.<ref>''The Compact Edition of the Oxford English Dictionary'', volume II, Oxford: Oxford University Press, 1971, p.3518.</ref> The Latin word was used by [[Cicero]] and later Latin authors in many of the same senses as the modern [[English language|English]] word is used.<ref name="lewis_short" /> The Latin word derives from the poetic contraction ''Unvorsum'' — first used by [[Lucretius]] in Book IV (line 262) of his ''[[On the Nature of Things|De rerum natura]]'' (''On the Nature of Things'') — which connects ''un, uni'' (the combining form of ''unus', or "one") with ''vorsum, versum'' (a noun made from the perfect passive participle of ''vertere'', meaning "something rotated, rolled, changed").<ref name="lewis_short">Lewis and Short, ''A Latin Dictionary'', Oxford University Press, ISBN 0-19-864201-6, pp. 1933, 1977–1978.</ref> |
|||
Each of the six centre pieces pivots on a screw (fastener) held by the centre piece, a "3-D cross". A spring between each screw head and its corresponding piece tensions the piece inward, so that collectively, the whole assembly remains compact, but can still be easily manipulated. The screw can be tightened or loosened to change the "feel" of the Cube. Newer official Rubik's brand cubes have rivets instead of screws and cannot be adjusted. |
|||
The Cube can be taken apart without much difficulty, typically by rotating the top layer by 45° and then prying one of its edge cubes away from the other two layers. Consequently it is a simple process to "solve" a Cube by taking it apart and reassembling it in a solved state. |
|||
There are six central pieces which show one coloured face, twelve edge pieces which show two coloured faces, and eight corner pieces which show three coloured faces. Each piece shows a unique colour combination, but not all combinations are present (for example, if red and orange are on opposite sides of the solved Cube, there is no edge piece with both red and orange sides). The location of these cubes relative to one another can be altered by twisting an outer third of the Cube 90°, 180° or 270°, but the location of the coloured sides relative to one another in the completed state of the puzzle cannot be altered: it is fixed by the relative positions of the centre squares. However, Cubes with alternative colour arrangements also exist; for example, with the yellow face opposite the green, the blue face opposite the white, and red and orange remaining opposite each other. |
|||
Douglas Hofstadter, in the July 1982 issue of Scientific American, pointed out that Cubes could be coloured in such a way as to emphasise the corners or edges, rather than the faces as the standard colouring does; but neither of these alternative colourings has ever become popular.[16] |
|||
Mathematics |
|||
[[File:Foucault pendulum animated.gif|thumb|right|Artistic rendition (highly exaggerated) of a [[Foucault pendulum]] showing that the Earth is not stationary, but rotates.]] |
|||
Permutations |
|||
The original (3×3×3) Rubik's Cube has eight corners and twelve edges. There are 8! (40,320) ways to arrange the corner cubes. Seven can be oriented independently, and the orientation of the eighth depends on the preceding seven, giving 37 (2,187) possibilities. There are 12!/2 (239,500,800) ways to arrange the edges, since an odd permutation of the corners implies an odd permutation of the edges as well. Eleven edges can be flipped independently, with the flip of the twelfth depending on the preceding ones, giving 211 (2,048) possibilities.[21] |
|||
An alternative interpretation of ''unvorsum'' is "everything rotated as one" or "everything rotated by one". In this sense, it may be considered a translation of an earlier Greek word for the universe, {{polytonic|περιφορά}}, (periforá, "circumambulation"), originally used to describe a course of a meal, the food being carried around the circle of dinner guests.<ref>Liddell and Scott, p. 1392.</ref> This Greek word refers to [[celestial spheres]], an early Greek model of the universe. Regarding Plato's [[Metaphor of the sun]], [[Aristotle]] suggests that the rotation of the sphere of [[fixed stars]] inspired by the [[primum movens|prime mover]], motivates, in turn, terrestrial change via the Sun. Careful [[astronomy|astronomical]] and physical measurements (such as the [[Foucault pendulum]]) are required to prove the Earth rotates on its axis. |
|||
which is approximately forty-three quintillion.[22] |
|||
The puzzle is often advertised as having only "billions" of positions, as the larger numbers are unfamiliar to many. To put this into perspective, if one had as many 57-millimeter Rubik's Cubes as there are permutations, they could cover the Earth's surface 275 times. |
|||
The preceding figure is limited to permutations that can be reached solely by turning the sides of the cube. If one considers permutations reached through disassembly of the cube, the number becomes twelve times as large: |
|||
A term for "universe" in ancient Greece was {{polytonic|τὸ πᾶν}} (tó pán, [[The All]], [[Pan (mythology)]]). Related terms were matter, ({{polytonic|τὸ ὅλον}}, tó ólon, see also [[Hyle]], lit. wood) and place ({{polytonic|τὸ κενόν}}, tó kenón).<ref>Liddell and Scott, pp. 1345–1346.</ref><ref>{{cite book|author = Yonge, Charles Duke|year = 1870|title = An English-Greek lexicon|publisher = American Bok Company|location = New York|pages = 567}}</ref> Other synonyms for the universe among the ancient Greek philosophers included {{polytonic|κόσμος}} ([[cosmos]]) and {{polytonic|φύσις}} (meaning [[Nature]], from which we derive the word [[physics]]).<ref>Liddell and Scott, pp. 985, 1964.</ref> The same synonyms are found in Latin authors (''totum'', ''mundus'', ''natura'')<ref>Lewis and Short, pp. 1881–1882, 1175, 1189–1190.</ref> and survive in modern languages, e.g., the German words ''Das All'', ''Weltall'', and ''Natur'' for universe. The same synonyms are found in English, such as everything (as in the [[theory of everything]]), the cosmos (as in [[cosmology]]), the [[world (philosophy)|world]] (as in the [[many-worlds hypothesis]]), and [[Nature]] (as in [[natural law]]s or [[natural philosophy]]).<ref>OED, pp. 909, 569, 3821–3822, 1900.</ref> |
|||
which is approximately five hundred and nineteen quintillion[22] possible arrangements of the pieces that make up the Cube, but only one in twelve of these are actually solvable. This is because there is no sequence of moves that will swap a single pair of pieces or rotate a single corner or edge cube. Thus there are twelve possible sets of reachable configurations, sometimes called "universes" or "orbits", into which the Cube can be placed by dismantling and reassembling it. |
|||
Centre faces |
|||
The original Rubik's Cube had no orientation markings on the centre faces (although some carried the words "Rubik's Cube" on the centre square of the white face), and therefore solving it does not require any attention to orienting those faces correctly. However, with marker pens, one could, for example, mark the central squares of an unscrambled Cube with four coloured marks on each edge, each corresponding to the colour of the adjacent face. Some Cubes have also been produced commercially with markings on all of the squares, such as the Lo Shu magic square or playing card suits. Thus one can nominally solve a Cube yet have the markings on the centres rotated; it then becomes an additional test to solve the centres as well. |
|||
Marking the Rubik's Cube increases its difficulty because this expands its set of distinguishable possible configurations. When the Cube is unscrambled apart from the orientations of the central squares, there will always be an even number of squares requiring a quarter turn. Thus there are 46/2 = 2,048 possible orientations of the centre squares in the otherwise unscrambled position, increasing the total number of possible Cube permutations from 43,252,003,274,489,856,000 (4.3×1019) to 88,580,102,706,155,225,088,000 (8.9×1022).[23] |
|||
When turning a cube over is considered to be a change in permutation then we must also count arrangements of the centre faces. Nominally there are 6! ways to arrange the six centre faces of the cube, but only 24 of these are achievable without disassembly of the cube. When the orientations of centres are also counted, as above, this increases the total number of possible Cube permutations from 88,580,102,706,155,225,088,000 (8.9×1022) to 2,125,922,464,947,725,402,112,000 (2.1×1024). |
|||
Algorithms |
|||
In Rubik's cubists' parlance, a memorised sequence of moves that has a desired effect on the cube is called an algorithm. This terminology is derived from the mathematical use of algorithm, meaning a list of well-defined instructions for performing a task from a given initial state, through well-defined successive states, to a desired end-state. Each method of solving the Rubik's Cube employs its own set of algorithms, together with descriptions of what the effect of the algorithm is, and when it can be used to bring the cube closer to being solved. |
|||
Most algorithms are designed to transform only a small part of the cube without scrambling other parts that have already been solved, so that they can be applied repeatedly to different parts of the cube until the whole is solved. For example, there are well-known algorithms for cycling three corners without changing the rest of the puzzle, or flipping the orientation of a pair of edges while leaving the others intact. |
|||
Some algorithms have a certain desired effect on the cube (for example, swapping two corners) but may also have the side-effect of changing other parts of the cube (such as permuting some edges). Such algorithms are often simpler than the ones without side-effects, and are employed early on in the solution when most of the puzzle has not yet been solved and the side-effects are not important. Towards the end of the solution, the more specific (and usually more complicated) algorithms are used instead, to prevent scrambling parts of the puzzle that have already been solved. |
|||
Solutions |
|||
===Broadest definition: reality and probability=== |
|||
Move notation |
|||
{{See also|Essence–Energies_distinction#Distinction between created and uncreated}} |
|||
Many 3×3×3 Rubik's Cube enthusiasts use a notation developed by David Singmaster to denote a sequence of moves, referred to as "Singmaster notation".[24] Its relative nature allows algorithms to be written in such a way that they can be applied regardless of which side is designated the top or how the colours are organised on a particular cube. |
|||
F (Front): the side currently facing the solver |
|||
B (Back): the side opposite the front |
|||
U (Up): the side above or on top of the front side |
|||
D (Down): the side opposite the top, underneath the Cube |
|||
L (Left): the side directly to the left of the front |
|||
R (Right): the side directly to the right of the front |
|||
ƒ (Front two layers): the side facing the solver and the corresponding middle layer |
|||
b (Back two layers): the side opposite the front and the corresponding middle layer |
|||
u (Up two layers) : the top side and the corresponding middle layer |
|||
d (Down two layers) : the bottom layer and the corresponding middle layer |
|||
l (Left two layers) : the side to the left of the front and the corresponding middle layer |
|||
r (Right two layers) : the side to the right of the front and the corresponding middle layer |
|||
x (rotate): rotate the entire Cube on R |
|||
y (rotate): rotate the entire Cube on U |
|||
z (rotate): rotate the entire Cube on F |
|||
When a prime symbol ( ′ ) follows a letter, it denotes a face turn counter-clockwise, while a letter without a prime symbol denotes a clockwise turn. A letter followed by a 2 (occasionally a superscript 2) denotes two turns, or a 180-degree turn. R is right side clockwise, but R' is right side counter-clockwise. The letters x, y, and z are used to indicate that the entire Cube should be turned about one of its axes. When x, y or z are primed, it is an indication that the cube must be rotated in the opposite direction. When they are squared, the cube must be rotated twice. |
|||
For methods using middle-layer turns (particularly corners-first methods) there is a generally accepted "MES" extension to the notation where letters M, E, and S denote middle layer turns. It was used e.g. in Marc Waterman's Algorithm.[25] |
|||
M (Middle): the layer between L and R, turn direction as L (top-down) |
|||
E (Equator): the layer between U and D, turn direction as D (left-right) |
|||
S (Standing): the layer between F and B, turn direction as F |
|||
The 4×4×4 and larger cubes use an extended notation to refer to the additional middle layers. Generally speaking, uppercase letters (F B U D L R) refer to the outermost portions of the cube (called faces). Lowercase letters (ƒ b u d ℓ r) refer to the inner portions of the cube (called slices). An asterisk (L*), a number in front of it (2L), or two layers in parenthesis (Lℓ), means to turn the two layers at the same time (both the inner and the outer left faces) For example: (Rr)' ℓ2 ƒ' means to turn the two rightmost layers counterclockwise, then the left inner layer twice, and then the inner front layer counterclockwise. |
|||
Optimal solutions |
|||
Main article: Optimal solutions for Rubik's Cube |
|||
Although there are a significant number of possible permutations for the Rubik's Cube, a number of solutions have been developed which allow for the cube to be solved in well under 100 moves. |
|||
Many general solutions for the Rubik's Cube have been discovered independently. The most popular method was developed by David Singmaster and published in the book Notes on Rubik's "Magic Cube" in 1981.[26] This solution involves solving the Cube layer by layer, in which one layer (designated the top) is solved first, followed by the middle layer, and then the final and bottom layer. After practice, solving the Cube layer by layer can be done in under one minute. Other general solutions include "corners first" methods or combinations of several other methods. In 1982, David Singmaster and Alexander Frey hypothesised that the number of moves needed to solve the Rubik's Cube, given an ideal algorithm, might be in "the low twenties".[27] In 2007, Daniel Kunkle and Gene Cooperman used computer search methods to demonstrate that any 3×3×3 Rubik's Cube configuration can be solved in 26 moves or fewer.[28][29][30] In 2008, Tomas Rokicki lowered that number to 22 moves,[31][32][33] and in July 2010, a team of researchers including Rokicki, working with Google, proved the so-called "God's number" to be 20.[34][35] This is optimal, since there exist some starting positions which require at least 20 moves to solve. More generally, it has been shown that an n × n × n Rubik's Cube can be solved optimally in Θ(n2 / log(n)) moves.[36] |
|||
A solution commonly used by speed cubers was developed by Jessica Fridrich. It is similar to the layer-by-layer method but employs the use of a large number of algorithms, especially for orienting and permuting the last layer. The cross is done first followed by first-layer corners and second layer edges simultaneously, with each corner paired up with a second-layer edge piece, thus completing the first two layers (F2L). This is then followed by orienting the last layer then permuting the last layer (OLL and PLL respectively). Fridrich's solution requires learning roughly 120 algorithms but allows the Cube to be solved in only 55 moves on average. |
|||
Philip Marshall's The Ultimate Solution to Rubik's Cube is a modified version of Fridrich's method, averaging only 65 twists yet requiring the memorization of only two algorithms.[37] |
|||
A now well-known method was developed by Lars Petrus. In this method, a 2×2×2 section is solved first, followed by a 2×2×3, and then the incorrect edges are solved using a three-move algorithm, which eliminates the need for a possible 32-move algorithm later. The principle behind this is that in layer by layer you must constantly break and fix the first layer; the 2×2×2 and 2×2×3 sections allow three or two layers to be turned without ruining progress. One of the advantages of this method is that it tends to give solutions in fewer moves. |
|||
In 1997, Denny Dedmore published a solution described using diagrammatic icons representing the moves to be made, instead of the usual notation.[38] |
|||
Competitions and records |
|||
The broadest definition of the universe can be found in ''[[De divisione naturae]]'' by the [[Middle Ages|medieval]] [[philosopher]] and [[theology|theologian]] [[Johannes Scotus Eriugena]], who defined it as simply everything: everything that is created and everything that is not created. In the [[path integral formulation]] of [[Richard Feynman]],<ref name="path_integral">{{cite book|author = Feynman RP, Hibbs AR|year = 1965|title = Quantum Physics and Path Integrals|publisher = McGraw–Hill|location = New York|isbn = 0-07-020650-3}}<br />{{cite book|author = Zinn Justin J|year = 2004|title = Path Integrals in Quantum Mechanics|publisher = Oxford University Press|isbn = 0-19-856674-3|oclc = 212409192}}</ref> the [[probability amplitude]]s for the various outcomes of an experiment given a perfectly defined initial state of the system are determined by summing over all possible histories (paths) by which the system could progress from the initial to final state. |
|||
Speedcubing competitions |
|||
Speedcubing (or speedsolving) is the practice of trying to solve a Rubik's Cube in the shortest time possible. There are a number of speedcubing competitions that take place around the world. |
|||
The first world championship organised by the Guinness Book of World Records was held in Munich on March 13, 1981. All Cubes were moved 40 times and lubricated with petroleum jelly. The official winner, with a record of 38 seconds, was Jury Froeschl, born in Munich. The first international world championship was held in Budapest on June 5, 1982, and was won by Minh Thai, a Vietnamese student from Los Angeles, with a time of 22.95 seconds. |
|||
Since 2003, the winner of a competition is determined by taking the average time of the middle three of five attempts. However, the single best time of all tries is also recorded. The World Cube Association maintains a history of world records.[39] In 2004, the WCA made it mandatory to use a special timing device called a Stackmat timer. |
|||
In addition to official competitions, informal alternative competitions have been held which invite participants to solve the Cube in unusual situations. Some such situations include: |
|||
Blindfolded solving[40] |
|||
Solving the Cube with one person blindfolded and the other person saying what moves to make, known as "Team Blindfold" |
|||
Solving the Cube underwater in a single breath[41] |
|||
Solving the Cube using a single hand[42] |
|||
Solving the Cube with one's feet[43] |
|||
Of these informal competitions, the World Cube Association sanctions only blindfolded, one-handed, and feet solving as official competition events.[44] |
|||
In blindfolded solving, the contestant first studies the scrambled cube (i.e., looking at it normally with no blindfold), and is then blindfolded before beginning to turn the cube's faces. Their recorded time for this event includes both the time spent examining the cube and the time spent manipulating it. |
|||
Records |
|||
The current world record for single time on a 3×3×3 Rubik's Cube was set by Feliks Zemdegs, who had a best time of 5.66 seconds at the Melbourne Winter Open 2011. The world record for average time per solve is also currently held by Zemdegs. At the same competition, he set a 7.64 second average .[45] |
|||
On March 17, 2010, 134 school boys from Dr Challoner's Grammar School, Amersham, England broke the previous Guinness World Record for most people solving a Rubik's cube at once in 12 minutes.[46] The previous record set in December 2008 in Santa Ana, CA achieved 96 completions. |
|||
Variations |
|||
===Definition as reality=== |
|||
{{See also|Reality|Physics}} |
|||
More customarily, the universe is defined as everything that exists, (has existed, and will exist) {{Citation needed|(This is probably from Carl Sagan's statement in an early NOVA program) date=May 2010|date=July 2011}}. According to our current understanding, the universe consists of three principles: [[spacetime]], forms of [[energy]], including [[momentum]] and [[matter]], and the [[physical law]]s that relate them. |
|||
===Definition as connected space-time=== |
|||
Variations of Rubik's Cubes, clockwise from upper left: V-Cube 7, Professor's Cube, V-Cube 6, Pocket Cube, original Rubik's Cube, Rubik's Revenge. Clicking on a cube in the picture will redirect to the respective cube's page. |
|||
{{See also|Chaotic Inflation theory}} |
|||
There are different variations of Rubik's Cubes with up to seven layers: the 2×2×2 (Pocket/Mini Cube), the standard 3×3×3 cube, the 4×4×4 (Rubik's Revenge/Master Cube), and the 5×5×5 (Professor's Cube), the 6×6×6 (V-Cube 6), and 7×7×7 (V-Cube 7). |
|||
CESailor Tech's E-cube is an electronic variant of the 3×3×3 cube, made with RGB LEDs and switches.[47] There are two switches on each row and column. Pressing the switches indicates the direction of rotation, which causes the LED display to change colours, simulating real rotations. The product was demonstrated at the Taiwan government show of college designs on October 30, 2008. |
|||
Another electronic variation of the 3×3×3 Cube is the Rubik's TouchCube. Sliding a finger across its faces causes its patterns of coloured lights to rotate the same way they would on a mechanical cube. The TouchCube was introduced at the American International Toy Fair in New York on February 15, 2009.[48][49] |
|||
The Cube has inspired an entire category of similar puzzles, commonly referred to as twisty puzzles, which includes the cubes of different sizes mentioned above as well as various other geometric shapes. Some such shapes include the tetrahedron (Pyraminx), the octahedron (Skewb Diamond), the dodecahedron (Megaminx), the icosahedron (Dogic). There are also puzzles that change shape such as Rubik's Snake and the Square One. |
|||
Custom-built puzzles |
|||
It is possible to conceive of disconnected [[space-time]]s, each existing but unable to interact with one another. An easily visualized metaphor is a group of separate [[soap bubble]]s, in which observers living on one soap bubble cannot interact with those on other soap bubbles, even in principle. According to one common terminology, each "soap bubble" of space-time is denoted as a universe, whereas our particular [[space-time]] is denoted as ''the universe'', just as we call our moon ''the [[Moon]]''. The entire collection of these separate space-times is denoted as the [[multiverse]].<ref name="EllisKS03">{{cite journal |
|||
|last = Ellis|first = George F.R. |
|||
|authorlink = George Francis Rayner Ellis |
|||
|coauthors = U. Kirchner, W.R. Stoeger |
|||
|title = Multiverses and physical cosmology |
|||
|journal = Monthly Notices of the Royal Astronomical Society |
|||
|volume = 347|issue = 3|pages = 921–936 |
|||
|year = 2004 |
|||
|arxiv = astro-ph/0305292 |
|||
|doi =10.1111/j.1365-2966.2004.07261.x |
|||
| bibcode=2004MNRAS.347..921E |
|||
}}</ref> In principle, the other unconnected universes may have different [[dimension]]alities and [[topology|topologies]] of [[space-time]], different forms of [[matter]] and [[energy]], and different [[physical law]]s and [[physical constant]]s, although such possibilities are currently speculative. |
|||
===Definition as observable reality=== |
|||
Novelty keychain |
|||
{{See also|Observable universe|Observational cosmology}} |
|||
In the past, puzzles have been built resembling the Rubik's Cube or based on its inner workings. For example, a cuboid is a puzzle based on the Rubik's Cube, but with different functional dimensions, such as, 2×2×4, 2×3×4, 3×3×5.[50] Many cuboids are based on 4×4×4 or 5×5×5 mechanisms, via building plastic extensions or by directly modifying the mechanism itself. |
|||
Some custom puzzles are not derived from any existing mechanism, such as the Gigaminx v1.5-v2, Bevel Cube, SuperX, Toru, Rua, and 1×2×3. These puzzles usually have a set of masters 3D printed, which then are copied using molding and casting techniques to create the final puzzle.[citation needed] |
|||
Other Rubik's Cube modifications include cubes that have been extended or truncated to form a new shape. An example of this is the Trabjer's Octahedron, which can be built by truncating and extending portions of a regular 3×3. Most shape mods can be adapted to higher-order cubes. In the case of Tony Fisher's Rhombic Dodecahedron, there are 3×3, 4×4, 5×5, and 6×6 versions of the puzzle. |
|||
Rubik's Cube software |
|||
According to a still-more-restrictive definition, the universe is everything within our connected [[space-time]] that could have a chance to interact with us and vice versa.{{Citation needed|date=May 2010}} According to the [[general relativity|general theory of relativity]], some regions of [[space]] may never interact with ours even in the lifetime of the universe, due to the finite [[speed of light]] and the ongoing [[expansion of space]]. For example, radio messages sent from Earth may never reach some regions of space, even if the universe would live forever; space may expand faster than light can traverse it. It is worth emphasizing that those distant regions of space are taken to exist and be part of reality as much as we are; yet we can never interact with them. The spatial region within which we can affect and be affected is denoted as the [[observable universe]]. Strictly speaking, the observable universe depends on the location of the observer. By traveling, an observer can come into contact with a greater region of space-time than an observer who remains still, so that the observable universe for the former is larger than for the latter. Nevertheless, even the most rapid traveler will not be able to interact with all of space. Typically, the observable universe is taken to mean the universe observable from our vantage point in the Milky Way Galaxy. |
|||
==Size, age, contents, structure, and laws==<!-- [[Hubble's law]] links to this section --> |
|||
Magic Cube 4D, a 4×4×4×4 virtual puzzle |
|||
{{Main|Observable universe|Age of the universe|Large-scale structure of the universe|Abundance of the chemical elements}} |
|||
The universe is immensely large and possibly infinite in volume. The region visible from Earth (the [[observable universe]]) is a sphere with a radius of about 46 billion [[light years]],<ref>{{cite web|last = Lineweaver|first = Charles|coauthors = Tamara M. Davis|year = 2005|url = http://space.mit.edu/~kcooksey/teaching/AY5/MisconceptionsabouttheBigBang_ScientificAmerican.pdf|title = Misconceptions about the Big Bang|publisher = [[Scientific American]]|accessdate = 2007-03-05}}</ref> based on where the [[metric expansion of space|expansion of space]] has [[comoving distance|taken]] the most distant objects observed. For comparison, the diameter of a typical [[galaxy]] is only 30,000 light-years, and the typical distance between two neighboring galaxies is only 3 million [[light-years]].<ref>Rindler (1977), p.196.</ref> As an example, our [[Milky Way]] Galaxy is roughly 100,000 light years in diameter,<ref>{{cite web |
|||
|last = Christian|first = Eric |
|||
|last2 = Samar|first2 = Safi-Harb |
|||
|title = How large is the Milky Way? |
|||
|url=http://imagine.gsfc.nasa.gov/docs/ask_astro/answers/980317b.html |
|||
|accessdate = 2007-11-28}}</ref> and our nearest sister galaxy, the [[Andromeda Galaxy]], is located roughly 2.5 million light years away.<ref>{{cite journal |
|||
|author=I. Ribas, C. Jordi, F. Vilardell, E.L. Fitzpatrick, R.W. Hilditch, F. Edward |
|||
|title=First Determination of the Distance and Fundamental Properties of an Eclipsing Binary in the Andromeda Galaxy |
|||
|journal=Astrophysical Journal |
|||
|year=2005 |
|||
|volume=635|issue=1|pages=L37–L40 |
|||
|bibcode=2005ApJ...635L..37R |
|||
|doi = 10.1086/499161 |
|||
|arxiv = astro-ph/0511045}}<br />{{cite journal |
|||
|author=McConnachie, A. W.; Irwin, M. J.; Ferguson, A. M. N.; Ibata, R. A.; Lewis, G. F.; Tanvir, N. |
|||
|title=Distances and metallicities for 17 Local Group galaxies |
|||
|journal=Monthly Notices of the Royal Astronomical Society |
|||
|year=2005 |
|||
|volume=356 |issue=4|pages=979–997 |
|||
|bibcode=2005MNRAS.356..979M |
|||
|doi = 10.1111/j.1365-2966.2004.08514.x |
|||
|arxiv = astro-ph/0410489}}</ref> There are probably more than 100 billion (10<sup>11</sup>) [[Galaxy|galaxies]] in the observable universe.<ref>{{cite web|last = Mackie|first = Glen |date= February 1, 2002|url = http://astronomy.swin.edu.au/~gmackie/billions.html|title = To see the Universe in a Grain of Taranaki Sand|publisher = Swinburne University|accessdate = 2006-12-20}}</ref> Typical galaxies range from [[dwarf galaxy|dwarfs]] with as few as ten million<ref>{{cite web|date=2000-05-03|url = http://www.eso.org/outreach/press-rel/pr-2000/pr-12-00.html|title = Unveiling the Secret of a Virgo Dwarf Galaxy|publisher = ESO|accessdate = 2007-01-03}}</ref> (10<sup>7</sup>) [[star]]s up to giants with one [[Orders of magnitude (numbers)#1012|trillion]]<ref name="M101">{{cite web|date=2006-02-28|url = http://www.nasa.gov/mission_pages/hubble/science/hst_spiral_m10.html|title = Hubble's Largest Galaxy Portrait Offers a New High-Definition View|publisher = NASA|accessdate = 2007-01-03}}</ref> (10<sup>12</sup>) stars, all orbiting the galaxy's center of mass. A 2010 study by astronomers estimated that the observable universe contains 300 sextillion (3{{e|23}}) stars.<ref>{{cite news |url=http://www.usatoday.com/tech/science/space/2010-12-01-dwarf-stars_N.htm |title=Universe holds billions more stars than previously thought |author=Vergano, Dan |date=1 December 2010 |work= [[USA Today]] |accessdate=14 December 2010}}</ref> |
|||
[[File:Cosmological Composition - Pie Chart.png|thumb|450px|The universe is believed to be mostly composed of [[dark energy]] and [[dark matter]], both of which are poorly understood at present. Less than 5% of the universe is ordinary matter, a relatively small contribution.]] |
|||
Magic Cube 5D, a 3x3x3x3x3 virtual puzzle |
|||
Puzzles like the Rubik's Cube can be simulated by computer software, which provide functions such as recording of player metrics, storing scrambled Cube positions, conducting online competitions, analyzing of move sequences, and converting between different move notations. Software can also simulate very large puzzles that are impractical to build, such as 100×100×100 and 1,000×1,000×1,000 cubes, as well as virtual puzzles that cannot be physically built, such as 4- and 5-dimensional analogues of the cube.[51][52] |
|||
Popular culture |
|||
The observable matter is spread homogeneously (''uniformly'') throughout the universe, when averaged over distances longer than 300 million light-years.<ref>{{cite journal|author=N. Mandolesi, P. Calzolari, S. Cortiglioni, F. Delpino, G. Sironi|title=Large-scale homogeneity of the Universe measured by the microwave background|journal=Letters to Nature|year=1986 |volume=319|issue=6056|pages=751–753|doi= 10.1038/319751a0 |bibcode = 1986Natur.319..751M}}</ref> However, on smaller length-scales, matter is observed to form "clumps", i.e., to cluster hierarchically; many [[atoms]] are condensed into [[star]]s, most stars into galaxies, most galaxies into [[galaxy groups and clusters|clusters, superclusters]] and, finally, the [[large-scale structure of the universe|largest-scale structures]] such as the [[Great Wall (astronomy)|Great Wall of galaxies]]. The observable matter of the universe is also spread ''isotropically'', meaning that no direction of observation seems different from any other; each region of the sky has roughly the same content.<ref>{{cite web|last = Hinshaw|first = Gary |date= November 29, 2006|url = http://map.gsfc.nasa.gov/m_mm.html|title = New Three Year Results on the Oldest Light in the Universe|publisher = NASA WMAP|accessdate = 2006-08-10}}</ref> The universe is also bathed in a highly [[isotropic]] [[microwave]] [[electromagnetic radiation|radiation]] that corresponds to a [[thermal equilibrium]] [[blackbody spectrum]] of roughly 2.725-[[kelvin]]s.<ref>{{cite web|last = Hinshaw|first = Gary |date= December 15, 2005|url = http://map.gsfc.nasa.gov/m_uni/uni_101bbtest3.html|title = Tests of the Big Bang: The CMB|publisher = NASA WMAP|accessdate = 2007-01-09}}</ref> The hypothesis that the large-scale universe is homogeneous and isotropic is known as the [[cosmological principle]],<ref>Rindler (1977), p. 202.</ref> which is [[End of Greatness|supported by astronomical observations]]. |
|||
Main article: Rubik's Cube in popular culture |
|||
Many movies and TV shows have featured characters that solve Rubik's Cubes quickly to establish their high intelligence. Rubik's Cubes also regularly feature as motifs in works of art. |
|||
The present overall [[density]] of the universe is very low, roughly 9.9 × 10<sup>−30</sup> grams per cubic centimetre. This mass-energy appears to consist of 73% [[dark energy]], 23% [[cold dark matter]] and 4% [[baryonic matter|ordinary matter]]. Thus the density of atoms is on the order of a single hydrogen atom for every four cubic meters of volume.<ref>{{cite web|last = Hinshaw|first = Gary |date= February 10, 2006|url = http://map.gsfc.nasa.gov/m_uni/uni_101matter.html|title = What is the Universe Made Of?|publisher = NASA WMAP|accessdate = 2007-01-04}}</ref> The properties of dark energy and dark matter are largely unknown. Dark matter [[gravity|gravitates]] as ordinary matter, and thus works to slow the [[metric expansion of space|expansion of the universe]]; by contrast, dark energy [[accelerating universe|accelerates its expansion]]. |
|||
The [[Wilkinson Microwave Anisotropy Probe|most precise estimate]] of the [[age of the universe|universe's age]] is 13.73±0.12 billion years old, based on observations of the [[cosmic microwave background radiation]].<ref name="NASA_age">{{cite web|title = Five-Year Wilkinson Microwave Anisotropy Probe (WMAP) Observations: Data Processing, Sky Maps, and Basic Results|url=http://lambda.gsfc.nasa.gov/product/map/dr3/pub_papers/fiveyear/basic_results/wmap5basic.pdf|format=PDF|publisher=nasa.gov|accessdate=2008-03-06}}</ref> Independent estimates (based on measurements such as [[radioactive dating]]) agree, although they are less precise, ranging from 11 to 20 billion years{{Dead link|date=May 2011}}<ref>{{cite web |
|||
|author =Britt RR |
|||
|title =Age of Universe Revised, Again |
|||
|publisher =[[Space.com]] |
|||
|date = 2003-01-03 |
|||
|url = http://www.space.com/scienceastronomy/age_universe_030103.html |
|||
|accessdate = 2007-01-08}}</ref> |
|||
to 13–15 billion years.<ref>{{cite web |
|||
|author = Wright EL |
|||
|title =Age of the Universe |
|||
|publisher =[[UCLA]] |
|||
|year = 2005 |
|||
|url = http://www.astro.ucla.edu/~wright/age.html |
|||
|accessdate = 2007-01-08 |
|||
}}<br />{{cite journal |
|||
|author = Krauss LM, Chaboyer B |
|||
|title =Age Estimates of Globular Clusters in the Milky Way: Constraints on Cosmology |
|||
|journal =[[Science (journal)|Science]] |
|||
|volume = 299 |
|||
|issue = 5603 |
|||
|pages = 65–69 |
|||
|date = 3 January 2003 |
|||
|doi =10.1126/science.1075631 |
|||
|pmid =12511641|bibcode = 2003Sci...299...65K}}</ref> The universe has not been the same at all times in its history; for example, the relative populations of [[quasar]]s and galaxies have changed and [[space]] itself appears to have [[metric expansion of space|expanded]]. This expansion accounts for how Earth-bound scientists can observe the light from a galaxy 30 billion light years away, even if that light has traveled for only 13 billion years; the very space between them has expanded. This expansion is consistent with the observation that the light from distant galaxies has been [[redshift]]ed; the [[photon]]s emitted have been stretched to longer [[wavelength]]s and lower [[frequency]] during their journey. The rate of this spatial expansion is [[accelerating universe|accelerating]], based on studies of [[Type Ia supernova]]e and corroborated by other data. |
|||
The [[abundance of the chemical elements|relative fractions]] of different [[chemical element]]s — particularly the lightest atoms such as [[hydrogen]], [[deuterium]] and [[helium]] — seem to be identical throughout the universe and throughout its observable history.<ref>{{cite web|last = Wright|first = Edward L. |date= September 12, 2004|url = http://www.astro.ucla.edu/~wright/BBNS.html|title = Big Bang Nucleosynthesis|publisher = UCLA|accessdate = 2007-01-05}}<br />{{cite journal|author=M. Harwit, M. Spaans|title=Chemical Composition of the Early Universe|journal=The Astrophysical Journal|year=2003 |volume=589 |issue=1|pages=53–57|bibcode=2003ApJ...589...53H|doi = 10.1086/374415|arxiv = astro-ph/0302259}}<br />{{cite journal|author=C. Kobulnicky, E. D. Skillman|title=Chemical Composition of the Early Universe|journal=Bulletin of the American Astronomical Society|year=1997 |volume=29|pages=1329|bibcode=1997AAS...191.7603K}}</ref> The universe seems to have much more [[matter]] than [[antimatter]], an asymmetry possibly related to the observations of [[CP violation]].<ref>{{cite web |date= October 28, 2003|url = http://www.pparc.ac.uk/ps/bbs/bbs_antimatter.asp|title = Antimatter|publisher = Particle Physics and Astronomy Research Council|accessdate = 2006-08-10}}</ref> The universe appears to have no net [[electric charge]], and therefore [[gravity]] appears to be the dominant interaction on cosmological length scales. The universe also appears to have neither net [[momentum]] nor [[angular momentum]]. The absence of net charge and momentum would follow from accepted physical laws ([[Gauss's law]] and the non-divergence of the [[stress-energy-momentum pseudotensor]], respectively), if the universe were finite.<ref>Landau and Lifshitz (1975), p.361.</ref> |
|||
[[File:Elementary particle interactions.svg|thumb|left|300px|The [[elementary particle]]s from which the universe is constructed. Six [[lepton]]s and six [[quark]]s comprise most of the [[matter]]; for example, the [[proton]]s and [[neutron]]s of [[atomic nucleus|atomic nuclei]] are composed of quarks, and the ubiquitous [[electron]] is a lepton. These particles interact via the [[gauge boson]]s shown in the middle row, each corresponding to a particular type of [[gauge symmetry]]. The [[Higgs boson]] (as yet unobserved) is believed to confer [[mass]] on the particles with which it is connected. The [[graviton]], a supposed gauge boson for [[gravity]], is not shown.]] |
|||
The universe appears to have a smooth [[space-time continuum]] consisting of three [[space|spatial]] [[dimension]]s and one temporal ([[time]]) dimension. On the average, [[3-space|space]] is observed to be very nearly flat (close to zero [[curvature]]), meaning that [[Euclidean geometry]] is experimentally true with high accuracy throughout most of the Universe.<ref name="Shape">[http://map.gsfc.nasa.gov/m_mm/mr_content.html WMAP Mission: Results – Age of the Universe]</ref> Spacetime also appears to have a [[simply connected space|simply connected]] [[topology]], at least on the length-scale of the observable universe. However, present observations cannot exclude the possibilities that the universe has more dimensions and that its spacetime may have a multiply connected global topology, in analogy with the cylindrical or [[toroid]]al topologies of two-dimensional [[space]]s.<ref name="_spacetime_topology">{{cite conference |
|||
|first = Jean-Pierre |
|||
|last = Luminet |
|||
|coauthors = Boudewijn F. Roukema |
|||
|title = Topology of the Universe: Theory and Observations |
|||
|booktitle = Proceedings of Cosmology School held at Cargese, Corsica, August 1998 |
|||
|year = 1999 |
|||
|arxiv = astro-ph/9901364 |
|||
}}<br />{{cite journal |
|||
|last = Luminet|first = Jean-Pierre |
|||
|coauthors = J. Weeks, A. Riazuelo, R. Lehoucq, J.-P. Uzan |
|||
|title = Dodecahedral space topology as an explanation for weak wide-angle temperature correlations in the cosmic microwave background |
|||
|journal = [[Nature (journal)|Nature]] |
|||
|volume = 425|issue = 6958|pages = 593–595 |
|||
|year=2003 |
|||
|pmid = 14534579 |
|||
|arxiv = astro-ph/0310253 |
|||
|doi =10.1038/nature01944 |
|||
|bibcode = 2003Natur.425..593L}}</ref> |
|||
The universe appears to behave in a manner that regularly follows a set of [[physical law]]s and [[physical constant]]s.<ref>{{cite web|last = Strobel|first = Nick |date= May 23, 2001|url = http://www.astronomynotes.com/starprop/s7.htm|title = The Composition of Stars|publisher = Astronomy Notes|accessdate = 2007-01-04}}<br />{{cite web|url=http://www.faqs.org/faqs/astronomy/faq/part4/section-4.html|title = Have physical constants changed with time?|publisher = Astrophysics (Astronomy Frequently Asked Questions)|accessdate = 2007-01-04}}</ref> According to the prevailing [[Standard Model]] of physics, all matter is composed of three generations of [[lepton]]s and [[quark]]s, both of which are [[fermion]]s. These [[elementary particle]]s interact via at most three [[fundamental interaction]]s: the [[electroweak]] interaction which includes [[electromagnetism]] and the [[weak nuclear force]]; the [[strong nuclear force]] described by [[quantum chromodynamics]]; and [[gravity]], which is best described at present by [[general relativity]]. The first two interactions can be described by [[renormalization|renormalized]] [[quantum field theory]], and are mediated by [[gauge boson]]s that correspond to a particular type of [[gauge symmetry]]. A renormalized quantum field theory of general relativity has not yet been achieved, although various forms of [[string theory]] seem promising. The theory of [[special relativity]] is believed to hold throughout the universe, provided that the spatial and temporal length scales are sufficiently short; otherwise, the more general theory of general relativity must be applied. There is no explanation for the particular values that [[physical constant]]s appear to have throughout our universe, such as [[Planck's constant]] ''h'' or the [[gravitational constant]] ''G''. Several [[conservation law]]s have been identified, such as the [[conservation of charge]], [[conservation of momentum|momentum]], [[conservation of angular momentum|angular momentum]] and [[conservation of energy|energy]]; in many cases, these conservation laws can be related to [[symmetry|symmetries]] or [[Bianchi identity|mathematical identities]]. |
|||
===Fine tuning=== |
|||
{{main|Fine-tuned Universe}} |
|||
It appears that many of the properties of the universe have special values in the sense that a universe where these properties only differ slightly would not be able to support intelligent life.<ref>{{cite book|author=Stephen Hawking|year=1988|title=A Brief History of Time|publisher=Bantam Books|isbn=0-553-05340-X|page=125|authorlink=Stephen Hawking}}</ref><ref>{{cite book|year=1999|title=Just Six Numbers|publisher=HarperCollins Publishers|isbn=0-465-03672-4|author=Martin Rees|authorlink=Martin Rees}}</ref> Not all scientists agree that this [[fine-tuned universe|fine-tuning]] exists.<ref name="adams">{{cite journal|last=Adams|first=F.C.|year=2008|title=Stars in other universes: stellar structure with different fundamental constants|journal= Journal of Cosmology and Astroparticle Physics|issue=08|doi=10.1088/1475-7516/2008/08/010|arxiv=0807.3697|volume=2008|pages=010|bibcode = 2008JCAP...08..010A}}</ref><ref>{{cite journal|last=Harnik|first=R.|coauthors=Kribs, G.D. and Perez, G.|year=2006|title=A universe without weak interactions|journal=Physical Review D|volume=74|doi=10.1103/PhysRevD.74.035006|issue=3|arxiv=hep-ph/0604027|pages=035006 |bibcode = 2006PhRvD..74c5006H}}</ref> In particular, it is not known under what conditions intelligent life could form and what form or shape that would take. A relevant observation in this discussion is that for an observer to exist to observe fine-tuning, the universe must be able to support intelligent life. As such the [[conditional probability]] of observing a universe that is fine-tuned to support intelligent life is 1. This observation is known as the [[anthropic principle]] and is particularly relevant if the creation of the universe was probabilistic or if multiple universes with a variety of properties exist (see [[#Multiverse theory|below]]). |
|||
==Historical models== |
|||
{{See also|Cosmology|Timeline of cosmology}} |
|||
Many models of the cosmos (cosmologies) and its origin (cosmogonies) have been proposed, based on the then-available data and conceptions of the universe. Historically, cosmologies and cosmogonies were based on narratives of gods acting in various ways. Theories of an impersonal universe governed by physical laws were first proposed by the Greeks and Indians. Over the centuries, improvements in astronomical observations and theories of motion and gravitation led to ever more accurate descriptions of the universe. The modern era of cosmology began with [[Albert Einstein|Albert Einstein's]] 1915 [[general relativity|general theory of relativity]], which made it possible to quantitatively predict the origin, evolution, and conclusion of the universe as a whole. Most modern, accepted theories of cosmology are based on general relativity and, more specifically, the predicted [[Big Bang]]; however, still more careful measurements are required to determine which theory is correct. |
|||
===Creation=== |
|||
{{Main|Creation myth|Creator deity}} |
|||
Many cultures have [[creation myth|stories describing the origin of the world]], which may be roughly grouped into common types. In one type of story, the world is born from a [[world egg]]; such stories include the [[Finnish people|Finnish]] [[epic poetry|epic poem]] ''[[Kalevala]]'', the [[China|Chinese]] story of [[Pangu]] or the [[History of India|Indian]] [[Brahmanda Purana]]. In related stories, the creation idea is caused by a single entity emanating or producing something by him- or herself, as in the [[Tibetan Buddhism]] concept of [[Adi-Buddha]], the [[ancient Greece|ancient Greek]] story of [[Gaia (mythology)|Gaia]] (Mother Earth), the [[Aztec mythology|Aztec]] goddess [[Coatlicue]] myth, the [[ancient Egyptian religion|ancient Egyptian]] [[Ennead|god]] [[Atum]] story, or the [[Genesis creation narrative]]. In another type of story, the world is created from the union of male and female deities, as in the [[Maori mythology|Maori story]] of [[Rangi and Papa]]. In other stories, the universe is created by crafting it from pre-existing materials, such as the corpse of a dead god — as from [[Tiamat]] in the [[Babylon]]ian epic [[Enuma Elish]] or from the giant [[Ymir]] in [[Norse mythology]] – or from chaotic materials, as in [[Izanagi]] and [[Izanami]] in [[Japanese mythology]]. In other stories, the universe emanates from fundamental principles, such as [[Brahman]] and [[Prakrti]], or the [[yin and yang]] of the [[Tao]]. |
|||
===Philosophical models=== |
|||
{{See|Cosmology}} |
|||
{{See also|Pre-Socratic philosophy|Physics (Aristotle)|Hindu cosmology|Islamic cosmology|Time}} |
|||
From the 6th century BCE, the [[pre-Socratic philosophy|pre-Socratic Greek philosophers]] developed the earliest known philosophical models of the universe. The earliest Greek philosophers noted that appearances can be deceiving, and sought to understand the underlying reality behind the appearances. In particular, they noted the ability of matter to change forms (e.g., ice to water to steam) and several philosophers proposed that all the apparently different materials of the world are different forms of a single primordial material, or [[arche]]. The first to do so was [[Thales]], who proposed this material is [[Water (classical element)|Water]]. Thales' student, [[Anaximander]], proposed that everything came from the limitless [[Apeiron (cosmology)|apeiron]]. [[Anaximenes of Miletus|Anaximenes]] proposed [[Air (classical element)|Air]] on account of its perceived attractive and repulsive qualities that cause the arche to condense or dissociate into different forms. [[Anaxagoras]], proposed the principle of [[Nous]] (Mind). [[Heraclitus]] proposed [[fire (classical element)|fire]] (and spoke of [[logos]]). [[Empedocles]] proposed the elements: earth, wind, air and fire. His four element theory became very popular. Like [[Pythagoras]], [[Plato]] believed that all things were composed of [[number]], with the Empedocles' elements taking the form of the [[Platonic solids]]. Democritus, and later philosophers—most notably [[Leucippus]]—proposed that the universe was composed of indivisible [[atom]]s moving through [[void (astronomy)|void]] ([[vacuum]]). [[Aristotle]] did not believe that was feasible because air, like water, offers [[Drag (physics)|resistance to motion]]. Air will immediately rush in to fill a void, and moreover, without resistance, it would do so indefinitely fast. |
|||
Although Heraclitus argued for eternal change, his quasi-contemporary [[Parmenides]] made the radical suggestion that all change is an illusion, that the true underlying reality is eternally unchanging and of a single nature. Parmenides denoted this reality as {{polytonic|τὸ ἐν}} (The One). Parmenides' theory seemed implausible to many Greeks, but his student [[Zeno of Elea]] challenged them with several famous [[Zeno's paradoxes|paradoxes]]. Aristotle responded to these paradoxes by developing the notion of a potential countable infinity, as well as the infinitely divisible continuum. Unlike the eternal and unchanging cycles of time, he believed the world was bounded by the celestial spheres, and thus magnitude was only finitely multiplicative. |
|||
The [[Indian philosophy|Indian philosopher]] [[Kanada]], founder of the [[Vaisheshika]] school, developed a theory of [[atomism]] and proposed that [[light]] and [[heat]] were varieties of the same substance.<ref>[[Will Durant]], ''Our Oriental Heritage'': |
|||
{{quote|"Two systems of Hindu thought propound physical theories suggestively similar to those of [[Ancient Greece|Greece]]. Kanada, founder of the Vaisheshika philosophy, held that the world was composed of atoms as many in kind as the various elements. The [[Jainism|Jains]] more nearly approximated to [[Democritus]] by teaching that all atoms were of the same kind, producing different effects by diverse modes of combinations. Kanada believed light and heat to be varieties of the same substance; [[Udayana]] taught that all heat comes from the sun; and [[Vācaspati Miśra|Vachaspati]], like Newton, interpreted light as composed of minute particles emitted by substances and striking the eye."}}</ref> In the 5th century AD, the [[Buddhist atomism|Buddhist atomist]] philosopher [[Dignāga]] proposed [[atom]]s to be point-sized, durationless, and made of energy. They denied the existence of substantial matter and proposed that movement consisted of momentary flashes of a stream of energy.<ref>F. Th. Stcherbatsky (1930, 1962), ''Buddhist Logic'', Volume 1, p.19, Dover, New York: |
|||
{{quote|"The Buddhists denied the existence of substantial matter altogether. Movement consists for them of moments, it is a staccato movement, momentary flashes of a stream of energy... "Everything is evanescent“,... says the Buddhist, because there is no stuff... Both systems <nowiki>[</nowiki>[[Samkhya|Sānkhya]], and later Indian Buddhism] share in common a tendency to push the analysis of Existence up to its minutest, last elements which are imagined as absolute qualities, or things possessing only one unique quality. They are called “qualities” (guna-dharma) in both systems in the sense of absolute qualities, a kind of atomic, or intra-atomic, energies of which the empirical things are composed. Both systems, therefore, agree in denying the objective reality of the categories of Substance and Quality,... and of the relation of Inference uniting them. There is in Sānkhya philosophy no separate existence of qualities. What we call quality is but a particular manifestation of a subtle entity. To every new unit of quality corresponds a subtle quantum of matter which is called guna “quality”, but represents a subtle substantive entity. The same applies to early Buddhism where all qualities are substantive... or, more precisely, dynamic entities, although they are also called dharmas ('qualities')."}}</ref> |
|||
The theory of [[temporal finitism]] was inspired by the doctrine of Creation shared by the three [[Abrahamic religions]]: [[Judaism]], [[Christianity]] and [[Islam]]. The [[Christian philosophy|Christian philosopher]], [[John Philoponus]], presented the philosophical arguments against the ancient Greek notion of an infinite past and future. Philoponus' arguments against an infinite past were used by the [[Early Islamic philosophy|early Muslim philosopher]], [[Al-Kindi]] (Alkindus); the [[Jewish philosophy|Jewish philosopher]], [[Saadia Gaon]] (Saadia ben Joseph); and the [[Kalam|Muslim theologian]], [[Al-Ghazali]] (Algazel). Borrowing from Aristotle's ''Physics'' and ''Metaphysics'', they employed two logical arguments against an infinite past, the first being the "argument from the impossibility of the existence of an actual infinite", which states:<ref name=Craig>{{Cite journal|title=Whitrow and Popper on the Impossibility of an Infinite Past|first=William Lane|last=Craig|journal=The British Journal for the Philosophy of Science|volume=30|issue=2|date=June 1979|pages=165–170 [165–6]|doi=10.1093/bjps/30.2.165}}</ref> |
|||
:"An actual infinite cannot exist." |
|||
:"An infinite temporal regress of events is an actual infinite." |
|||
:"<math>\therefore</math> An infinite temporal regress of events cannot exist." |
|||
The second argument, the "argument from the impossibility of completing an actual infinite by successive addition", states:<ref name=Craig/> |
|||
:"An actual infinite cannot be completed by successive addition." |
|||
:"The temporal series of past events has been completed by successive addition." |
|||
:"<math>\therefore</math> The temporal series of past events cannot be an actual infinite." |
|||
Both arguments were adopted by Christian philosophers and theologians, and the second argument in particular became more famous after it was adopted by [[Immanuel Kant]] in his thesis of the first [[antinomy]] concerning [[time]].<ref name=Craig/> |
|||
===Astronomical models=== |
|||
{{Main|History of astronomy}} |
|||
Astronomical models of the universe were proposed soon after [[astronomy]] began with the [[Babylonian astronomy|Babylonian astronomers]], who viewed the universe as a [[Flat Earth|flat disk]] floating in the ocean, and this forms the premise for early Greek maps like those of [[Anaximander]] and [[Hecataeus of Miletus]]. |
|||
Later [[Ancient Greece|Greek]] philosophers, observing the motions of the heavenly bodies, were concerned with developing models of the universe based more profoundly on empirical evidence. The first coherent model was proposed by [[Eudoxus of Cnidos]]. According to Aristotle's physical interpretation of the model, [[celestial spheres]] eternally [[uniform circular motion|rotate with uniform motion]] around a stationary Earth. Normal [[classical elements|matter]], is entirely contained within the terrestrial sphere. This model was also refined by [[Callippus]] and after concentric spheres were abandoned, it was brought into nearly perfect agreement with astronomical observations by [[Ptolemy]]. The success of such a model is largely due to the mathematical fact that any function (such as the position of a planet) can be decomposed into a set of circular functions (the [[Fourier modes]]). Other Greek scientists, such as the [[Pythagoreans|Pythagorean]] philosopher [[Philolaus]] postulated that at the center of the universe was a "central fire" around which the [[Earth]], [[Sun]], [[Moon]] and [[Planets]] revolved in uniform circular motion.<ref>Boyer, C. ''A History of Mathematics.'' Wiley, p. 54.</ref> |
|||
The [[Greek astronomy|Greek astronomer]] [[Aristarchus of Samos]] was the first known individual to propose a [[Heliocentrism|heliocentric]] model of the universe. Though the original text has been lost, a reference in Archimedes' book The Sand Reckoner describes Aristarchus' heliocentric theory. [[Archimedes]] wrote: (translated into English) |
|||
<blockquote> |
|||
You King Gelon are aware the 'universe' is the name given by most astronomers to the sphere the center of which is the center of the Earth, while its radius is equal to the straight line between the center of the Sun and the center of the Earth. This is the common account as you have heard from astronomers. But Aristarchus has brought out a book consisting of certain hypotheses, wherein it appears, as a consequence of the assumptions made, that the universe is many times greater than the 'universe' just mentioned. His hypotheses are that the fixed stars and the Sun remain unmoved, that the Earth revolves about the Sun on the circumference of a circle, the Sun lying in the middle of the orbit, and that the sphere of fixed stars, situated about the same center as the Sun, is so great that the circle in which he supposes the Earth to revolve bears such a proportion to the distance of the fixed stars as the center of the sphere bears to its surface. |
|||
</blockquote> |
|||
Aristarchus thus believed the stars to be very far away, and saw this as the reason why there was no visible parallax, that is, an observed movement of the stars relative to each other as the Earth moved around the Sun. The stars are in fact much farther away than the distance that was generally assumed in ancient times, which is why stellar parallax is only detectable with telescopes. The geocentric model, consistent with planetary parallax, was assumed to be an explanation for the unobservability of the parallel phenomenon, stellar parallax. The rejection of the heliocentric view was apparently quite strong, as the following passage from Plutarch suggests (On the Apparent Face in the Orb of the Moon): |
|||
<blockquote> |
|||
[[Cleanthes]] [a contemporary of Aristarchus and head of the Stoics] thought it was the duty of the Greeks to indict Aristarchus of Samos on the charge of impiety for putting in motion the Hearth of the universe [i.e. the earth], . . . supposing the heaven to remain at rest and the earth to revolve in an oblique circle, while it rotates, at the same time, about its own axis. [1] |
|||
</blockquote> |
|||
The only other astronomer from antiquity known by name who supported Aristarchus' heliocentric model was [[Seleucus of Seleucia]], a [[Hellenization|Hellenized]] [[Babylonia]]n astronomer who lived a century after Aristarchus.<ref>[[Otto E. Neugebauer]] (1945). "The History of Ancient Astronomy Problems and Methods", ''Journal of Near Eastern Studies'' '''4''' (1), p. 1–38. |
|||
{{quote|"the [[Chaldaea]]n Seleucus from Seleucia"}}</ref><ref>[[George Sarton]] (1955). "Chaldaean Astronomy of the Last Three Centuries B. C.", ''Journal of the American Oriental Society'' '''75''' (3), pp. 166–173 [169]: |
|||
{{quote|"the heliocentrical astronomy invented by Aristarchos of Samos and still defended a century later by Seleucos the [[Babylonia]]n"}}</ref><ref>William P. D. Wightman (1951, 1953), ''The Growth of Scientific Ideas'', Yale University Press p.38, where Wightman calls him [[Seleucus of Seleucia|Seleukos]] the [[Chaldea]]n.</ref> According to [[Plutarch]], Seleucus was the first to prove the heliocentric system through [[reasoning]], but it is not known what arguments he used. Seleucus' arguments for a heliocentric theory were probably related to the phenomenon of [[tide]]s.<ref>[[Lucio Russo]], ''Flussi e riflussi'', Feltrinelli, Milano, 2003, ISBN 88-07-10349-4.</ref> According to [[Strabo]] (1.1.9), Seleucus was the first to state that the [[tide]]s are due to the attraction of the Moon, and that the height of the tides depends on the Moon's position relative to the Sun.<ref>Bartel, p. 527</ref> Alternatively, he may have proved the heliocentric theory by determining the constants of a [[Geometry|geometric]] model for the heliocentric theory and by developing methods to compute planetary positions using this model, like what Nicolaus Copernicus later did in the 16th century.<ref>Bartel, pp. 527–9</ref> During the [[Middle Ages]], heliocentric models may have also been proposed by the [[Indian astronomy|Indian astronomer]], [[Aryabhata]],<ref>Bartel, pp. 529–34</ref> and by the [[Islamic astronomy|Persian astronomers]], [[Ja'far ibn Muhammad Abu Ma'shar al-Balkhi|Albumasar]]<ref>Bartel, pp. 534–7</ref> and [[Al-Sijzi]].<ref name=Nasr>{{Cite book |last=Nasr |first=Seyyed H. |authorlink=Hossein Nasr |date=1st edition in 1964, 2nd edition in 1993 |title=An Introduction to Islamic Cosmological Doctrines |edition=2nd |publisher=1st edition by [[Harvard University Press]], 2nd edition by [[State University of New York Press]] |isbn=0791415155 |pages=135–6}}</ref> |
|||
[[File:ThomasDiggesmap.JPG|thumb|left|[[Copernican heliocentrism|Model of the Copernican universe]] by [[Thomas Digges]] in 1576, with the amendment that the stars are no longer confined to a sphere, but spread uniformly throughout the space surrounding the [[planet]]s.]] |
|||
The Aristotelian model was accepted in the [[Western world]] for roughly two millennia, until Copernicus revived Aristarchus' theory that the astronomical data could be explained more plausibly if the [[earth]] rotated on its axis and if the [[sun]] were placed at the center of the universe. |
|||
{{cquote|In the center rests the sun. For who would place this lamp of a very beautiful temple in another or better place than this wherefrom it can illuminate everything at the same time?|20px|20px|Nicolaus Copernicus| in Chapter 10, Book 1 of ''De Revolutionibus Orbium Coelestrum'' (1543)}} |
|||
As noted by Copernicus himself, the suggestion that the [[Earth's rotation|Earth rotates]] was very old, dating at least to [[Philolaus]] (c. 450 BC), [[Heraclides Ponticus]] (c. 350 BC) and [[Ecphantus the Pythagorean]]. Roughly a century before Copernicus, Christian scholar [[Nicholas of Cusa]] also proposed that the Earth rotates on its axis in his book, ''On Learned Ignorance'' (1440).<ref>Misner, Thorne and Wheeler (1973), p. 754.</ref> Aryabhata (476–550), [[Brahmagupta]] (598–668), [[Albumasar]] and [[Al-Sijzi]], also proposed that the Earth rotates on its axis.{{Citation needed|date=April 2010}} The first [[Empirical research|empirical evidence]] for the Earth's rotation on its axis, using the phenomenon of [[comet]]s, was given by [[Nasīr al-Dīn al-Tūsī|Tusi]] (1201–1274) and [[Ali Qushji]] (1403–1474).{{Citation needed|date=April 2010}} |
|||
[[File:Libr0309.jpg|thumb|[[Johannes Kepler]] published the ''[[Rudolphine Tables]]'' containing a star catalog and planetary tables using [[Tycho Brahe]]'s measurements.]] |
|||
This cosmology was accepted by [[Isaac Newton]], [[Christiaan Huygens]] and later scientists.<ref name = "Misner-p755">Misner, Thorne, and Wheeler (1973), p. 755–756.</ref> Edmund Halley (1720)<ref>Misner, Thorne, and Wheeler (1973), p. 756.</ref> and [[Jean-Philippe de Cheseaux]] (1744)<ref>{{cite book|author = de Cheseaux JPL|year = 1744|title = Traité de la Comète|publisher = Lausanne|pages = 223ff|authorlink = Jean-Philippe de Cheseaux}}. Reprinted as Appendix II in {{cite book|author = Dickson FP|year = 1969|title = The Bowl of Night: The Physical Universe and Scientific Thought|publisher = M.I.T. Press|location = Cambridge, MA|isbn = 978-0262540032}}</ref> noted independently that the assumption of an infinite space filled uniformly with stars would lead to the prediction that the nighttime sky would be as bright as the sun itself; this became known as [[Olbers' paradox]] in the 19th century.<ref>{{cite journal|author = Olbers HWM|year = 1826|title = Unknown title|journal = Bode's Jahrbuch|volume = 111|authorlink = Heinrich Wilhelm Matthäus Olbers}}. Reprinted as Appendix I in {{cite book|author = Dickson FP|year = 1969|title = The Bowl of Night: The Physical Universe and Scientific Thought|publisher = M.I.T. Press|location = Cambridge, MA|isbn = 978-0262540032}}</ref> Newton believed that an infinite space uniformly filled with matter would cause infinite forces and instabilities causing the matter to be crushed inwards under its own gravity.<ref name = "Misner-p755"/> This instability was clarified in 1902 by the [[Jeans instability]] criterion.<ref>{{cite journal|last1=Jeans |first1= J. H. |year=1902| title = The Stability of a Spherical Nebula|journal= Philosophical Transactions Royal Society of London, Series A| volume=199| pages= 1–53 |issue=312–320|doi = 10.1098/rsta.1902.0012|bibcode = 1902RSPTA.199....1J|jstor=90845|url=http://maeresearch.ucsd.edu/~cgibson/Documents2007/PapersAList%20copy/MiscellaneousPapers/Jeans1902.pdf|format=PDF|accessdate=17 March 2011}}</ref> One solution to these paradoxes is the [[Carl Charlier|Charlier universe]], in which the matter is arranged hierarchically (systems of orbiting bodies that are themselves orbiting in a larger system, ''ad infinitum'') in a [[fractal]] way such that the universe has a negligibly small overall density; such a cosmological model had also been proposed earlier in 1761 by [[Johann Heinrich Lambert]].<ref>Rindler, p. 196; Misner, Thorne, and Wheeler (1973), p. 757.</ref> A significant astronomical advance of the 18th century was the realization by [[Thomas Wright (astronomer)|Thomas Wright]], [[Immanuel Kant]] and others of [[nebulae]].<ref>Misner, Thorne and Wheeler, p.756.</ref> |
|||
The modern era of [[physical cosmology]] began in 1917, when [[Albert Einstein]] first applied his general theory of relativity to model the structure and dynamics of the universe.<ref name="einstein_1917">{{cite journal|last = Einstein|first = A|authorlink = Albert Einstein|year = 1917|title = Kosmologische Betrachtungen zur allgemeinen Relativitätstheorie|series = 1917|journal = Preussische Akademie der Wissenschaften, Sitzungsberichte|volume = (part 1)|pages = 142–152}}</ref> |
|||
==Theoretical models== |
|||
[[File:Cassini-science-br.jpg|thumb|High-precision test of general relativity by the [[Cassini-Huygens|Cassini]] space probe (artist's impression): [[radio]] signals sent between the Earth and the probe (green wave) are [[Shapiro effect|delayed]] by the warping of [[space and time]] (blue lines) due to the [[Sun]]'s mass.]] |
|||
Of the four [[fundamental interaction]]s, [[gravitation]] is dominant at cosmological length scales; that is, the other three forces are believed to play a negligible role in determining structures at the level of planets, stars, galaxies and larger-scale structures. Since all matter and energy gravitate, gravity's effects are cumulative; by contrast, the effects of positive and negative charges tend to cancel one another, making electromagnetism relatively insignificant on cosmological length scales. The remaining two interactions, the [[weak nuclear force|weak]] and [[strong nuclear force]]s, decline very rapidly with distance; their effects are confined mainly to sub-atomic length scales. |
|||
===General theory of relativity=== |
|||
{{Main|Introduction to general relativity|General relativity|Einstein's field equations}} |
|||
Given gravitation's predominance in shaping cosmological structures, accurate predictions of the universe's past and future require an accurate theory of gravitation. The best theory available is [[Albert Einstein]]'s general theory of relativity, which has passed all experimental tests hitherto. However, since rigorous experiments have not been carried out on cosmological length scales, general relativity could conceivably be inaccurate. Nevertheless, its cosmological predictions appear to be consistent with observations, so there is no compelling reason to adopt another theory. |
|||
General relativity provides a set of ten nonlinear partial differential equations for the [[metric tensor (general relativity)|spacetime metric]] ([[Einstein field equations|Einstein's field equations]]) that must be solved from the distribution of [[mass-energy]] and [[momentum]] throughout the universe. Since these are unknown in exact detail, cosmological models have been based on the [[cosmological principle]], which states that the universe is homogeneous and isotropic. In effect, this principle asserts that the gravitational effects of the various galaxies making up the universe are equivalent to those of a fine [[Dust solution|dust]] distributed uniformly throughout the universe with the same average density. The assumption of a uniform dust makes it easy to solve Einstein's field equations and predict the past and future of the universe on cosmological time scales. |
|||
Einstein's field equations include a [[cosmological constant]] (''Λ''),<ref name="einstein_1917" /><ref>Rindler (1977), pp. 226–229.</ref> that corresponds to an energy density of empty space.<ref>Landau and Lifshitz (1975), pp. 358–359.</ref> Depending on its sign, the cosmological constant can either slow (negative ''Λ'') or accelerate (positive ''Λ'') the [[metric expansion of space|expansion of the universe]]. Although many scientists, including Einstein, had speculated that ''Λ'' was zero,<ref>{{cite journal|last = Einstein|first = A|authorlink = Albert Einstein|year = 1931|title = Zum kosmologischen Problem der allgemeinen Relativitätstheorie|journal = Sitzungsberichte der Preussischen Akademie der Wissenschaften, Physikalisch-mathematische Klasse|volume = 1931|pages = 235–237}}<br />{{cite journal|author = [[Albert Einstein|Einstein A.]], [[Willem de Sitter|de Sitter W.]]|year = 1932|title = On the relation between the expansion and the mean density of the universe|journal = Proceedings of the National Academy of Sciences|volume = 18|pages = 213–214|doi = 10.1073/pnas.18.3.213|pmid = 16587663|issue = 3|pmc = 1076193|bibcode = 1932PNAS...18..213E}}</ref> recent astronomical observations of [[type Ia supernova]]e have detected a large amount of "[[dark energy]]" that is accelerating the universe's expansion.<ref>[http://hubblesite.org/newscenter/archive/releases/2004/12/text/ Hubble Telescope news release]</ref> Preliminary studies suggest that this dark energy corresponds to a positive ''Λ'', although alternative theories cannot be ruled out as yet.<ref>{{cite news|url = http://news.bbc.co.uk/1/hi/sci/tech/6156110.stm|work=BBC News|title=Mysterious force's long presence|date=2006-11-16}}</ref> Russian [[physics|physicist]] [[Yakov Borisovich Zel'dovich|Zel'dovich]] suggested that ''Λ'' is a measure of the [[zero-point energy]] associated with [[virtual particle]]s of [[quantum field theory]], a pervasive [[vacuum energy]] that exists everywhere, even in empty space.<ref>{{cite journal|author = Zel'dovich YB|year = 1967|title = Cosmological constant and elementary particles|journal = Zh. Eksp. & Teor. Fiz. Pis'ma|volume = 6|pages = 883–884|authorlink = Yakov Borisovich Zel'dovich}} English translation in ''Sov. Phys. — JTEP Lett.'', '''6''', pp. 316–317 (1967).</ref> Evidence for such zero-point energy is observed in the [[Casimir effect]]. |
|||
===Special relativity and space-time=== |
|||
{{Main|Introduction to special relativity|Special relativity}} |
|||
[[File:Only distance is real.svg|thumb|300px|Only its length ''L'' is intrinsic to the rod (shown in black); coordinate differences between its endpoints (such as Δx, Δy or Δξ, Δη) depend on their frame of reference (depicted in blue and red, respectively).]] |
|||
The universe has at least three [[space|spatial]] and one temporal ([[time]]) dimension. It was long thought that the spatial and temporal dimensions were different in nature and independent of one another. However, according to the [[special relativity|special theory of relativity]], spatial and temporal separations are interconvertible (within limits) by changing one's motion. |
|||
To understand this interconversion, it is helpful to consider the analogous interconversion of spatial separations along the three spatial dimensions. Consider the two endpoints of a rod of length ''L''. The length can be determined from the differences in the three coordinates Δx, Δy and Δz of the two endpoints in a given reference frame |
|||
:<math> |
|||
L^{2} = \Delta x^{2} + \Delta y^{2} + \Delta z^{2} |
|||
</math> |
|||
using the [[Pythagorean theorem]]. In a rotated reference frame, the coordinate differences differ, but they give the same length |
|||
:<math> |
|||
L^{2} = \Delta \xi^{2} + \Delta \eta^{2} + \Delta \zeta^{2}. |
|||
</math> |
|||
Thus, the coordinates differences (Δx, Δy, Δz) and (Δξ, Δη, Δζ) are not intrinsic to the rod, but merely reflect the reference frame used to describe it; by contrast, the length ''L'' is an intrinsic property of the rod. The coordinate differences can be changed without affecting the rod, by rotating one's reference frame. |
|||
The analogy in [[spacetime]] is called the interval between two events; an event is defined as a point in spacetime, a specific position in space and a specific moment in time. The spacetime interval between two events is given by |
|||
:<math> |
|||
s^{2} = L_{1}^{2} - c^{2} \Delta t_{1}^{2} = L_{2}^{2} - c^{2} \Delta t_{2}^{2} |
|||
</math> |
|||
where ''c'' is the speed of light. According to [[special relativity]], one can change a spatial and time separation (''L''<sub>1</sub>, Δ''t''<sub>1</sub>) into another (''L''<sub>2</sub>, Δ''t''<sub>2</sub>) by changing one's reference frame, as long as the change maintains the spacetime interval ''s''. Such a change in reference frame corresponds to changing one's motion; in a moving frame, lengths and times are different from their counterparts in a stationary reference frame. The precise manner in which the coordinate and time differences change with motion is described by the [[Lorentz transformation]]. |
|||
===Solving Einstein's field equations=== |
|||
{{See also|Big Bang|Ultimate fate of the universe}} |
|||
[[File:Closed Friedmann universe zero Lambda.ogg|thumb|right|Animation illustrating the [[metric expansion of the universe]]]] |
|||
The distances between the spinning galaxies increase with time, but the distances between the stars within each galaxy stay roughly the same, due to their gravitational interactions. This animation illustrates a closed Friedmann universe with zero [[cosmological constant]] Λ; such a universe oscillates between a [[Big Bang]] and a [[Big Crunch]]. |
|||
In non-Cartesian (non-square) or curved coordinate systems, the Pythagorean theorem holds only on infinitesimal length scales and must be augmented with a more general [[metric tensor]] ''g''<sub>μν</sub>, which can vary from place to place and which describes the local geometry in the particular coordinate system. However, assuming the [[cosmological principle]] that the universe is homogeneous and isotropic everywhere, every point in space is like every other point; hence, the metric tensor must be the same everywhere. That leads to a single form for the metric tensor, called the [[Friedmann–Lemaître–Robertson–Walker metric]] |
|||
:<math> |
|||
ds^2 = -c^{2} dt^2 + |
|||
R(t)^2 \left( \frac{dr^2}{1-k r^2} + r^2 d\theta^2 + r^2 \sin^2 \theta \, d\phi^2 \right) |
|||
</math> |
|||
where (''r'', θ, φ) correspond to a [[spherical coordinate system]]. This [[metric (mathematics)|metric]] has only two undetermined parameters: an overall length scale ''R'' that can vary with time, and a curvature index ''k'' that can be only 0, 1 or −1, corresponding to flat [[Euclidean geometry]], or spaces of positive or negative [[curvature]]. In cosmology, solving for the history of the universe is done by calculating ''R'' as a function of time, given ''k'' and the value of the [[cosmological constant]] ''Λ'', which is a (small) parameter in Einstein's field equations. The equation describing how ''R'' varies with time is known as the [[Friedmann equation]], after its inventor, [[Alexander Friedmann]].<ref>{{cite journal|author = Friedmann A.|year = 1922|title = Über die Krümmung des Raumes|journal = Zeitschrift für Physik|volume = 10|issue = 1|pages = 377–386|doi = 10.1007/BF01332580|bibcode = 1922ZPhy...10..377F|authorlink = Alexander Friedmann}}</ref> |
|||
The solutions for ''R(t)'' depend on ''k'' and ''Λ'', but some qualitative features of such solutions are general. First and most importantly, the length scale ''R'' of the universe can remain constant ''only'' if the universe is perfectly isotropic with positive curvature (''k''=1) and has one precise value of density everywhere, as first noted by [[Albert Einstein]]. However, this equilibrium is unstable and since the universe is known to be inhomogeneous on smaller scales, ''R'' must change, according to [[general relativity]]. When ''R'' changes, all the spatial distances in the universe change in tandem; there is an overall expansion or contraction of space itself. This accounts for the observation that galaxies appear to be flying apart; the space between them is stretching. The stretching of space also accounts for the apparent paradox that two galaxies can be 40 billion light years apart, although they started from the same point 13.7 billion years ago and never moved faster than the [[speed of light]]. |
|||
Second, all solutions suggest that there was a [[gravitational singularity]] in the past, when ''R'' goes to zero and matter and energy became infinitely dense. It may seem that this conclusion is uncertain since it is based on the questionable assumptions of perfect homogeneity and isotropy (the cosmological principle) and that only the gravitational interaction is significant. However, the [[Penrose–Hawking singularity theorems]] show that a singularity should exist for very general conditions. Hence, according to Einstein's field equations, ''R'' grew rapidly from an unimaginably hot, dense state that existed immediately following this singularity (when ''R'' had a small, finite value); this is the essence of the [[Big Bang]] model of the universe. A common misconception is that the Big Bang model predicts that matter and energy exploded from a single point in space and time; that is false. Rather, space itself was created in the Big Bang and imbued with a fixed amount of energy and matter distributed uniformly throughout; as space expands (i.e., as ''R(t)'' increases), the density of that matter and energy decreases. |
|||
{| class="toccolours" style="float: left; margin-left: 1em; margin-right: 2em; font-size: 85%; background:#FFFDD0; color:black; width:30em; max-width: 35%;" cellspacing="5" |
|||
| style="text-align: left;"| |
|||
Space has no boundary – that is empirically more certain than any external observation. However, that does not imply that space is infinite...(translated, original German) |
|||
|- |
|||
| style="text-align: left;"|[[Bernhard Riemann]] (Habilitationsvortrag, 1854) |
|||
|} |
|||
Third, the curvature index ''k'' determines the sign of the mean spatial curvature of [[spacetime]] averaged over length scales greater than a billion [[light year]]s. If ''k''=1, the curvature is positive and the universe has a finite volume. Such universes are often visualized as a [[3-sphere|three-dimensional sphere ''S''<sup>3</sup> embedded in a four-dimensional space]]. Conversely, if ''k'' is zero or negative, the universe ''may'' have infinite volume, depending on its overall [[topology]]. It may seem counter-intuitive that an infinite and yet infinitely dense universe could be created in a single instant at the Big Bang when ''R''=0, but exactly that is predicted mathematically when ''k'' does not equal 1. For comparison, an infinite plane has zero curvature but infinite area, whereas an infinite cylinder is finite in one direction and a [[torus]] is finite in both. A toroidal universe could behave like a normal universe with [[periodic boundary conditions]], as seen in [[wraparound (video games)|"wrap-around" video games]] such as ''[[Asteroids (arcade game)|Asteroids]]''; a traveler crossing an outer "boundary" of space going ''outwards'' would reappear instantly at another point on the boundary moving ''inwards''. |
|||
[[File:CMB Timeline300 no WMAP.jpg|thumb|600px|center|Prevailing model of the origin and expansion of [[spacetime]] and all that it contains.]] |
|||
The [[ultimate fate of the universe]] is still unknown, because it depends critically on the curvature index ''k'' and the cosmological constant ''Λ''. If the universe is sufficiently dense, ''k'' equals +1, meaning that its average curvature throughout is positive and the universe will eventually recollapse in a [[Big Crunch]], possibly starting a new universe in a [[Big Bounce]]. Conversely, if the universe is insufficiently dense, ''k'' equals 0 or −1 and the universe will expand forever, cooling off and eventually becoming inhospitable for all life, as the stars die and all matter coalesces into black holes (the [[Future of an expanding universe|Big Freeze]] and the [[heat death of the universe]]). As noted above, recent data suggests that the expansion speed of the universe is not decreasing as originally expected, but increasing; if this continues indefinitely, the universe will eventually rip itself to shreds (the [[Big Rip]]). Experimentally, the universe has an overall density that is very close to the critical value between recollapse and eternal expansion; more careful astronomical observations are needed to resolve the question. |
|||
===Big Bang model=== |
|||
{{Main|Big Bang|Timeline of the Big Bang|Nucleosynthesis|Lambda-CDM model}} |
|||
The prevailing Big Bang model accounts for many of the experimental observations described above, such as the correlation of distance and [[redshift]] of galaxies, the universal ratio of hydrogen:helium atoms, and the ubiquitous, isotropic microwave radiation background. As noted above, the redshift arises from the [[metric expansion of space]]; as the space itself expands, the wavelength of a [[photon]] traveling through space likewise increases, decreasing its energy. The longer a photon has been traveling, the more expansion it has undergone; hence, older photons from more distant galaxies are the most red-shifted. Determining the correlation between distance and redshift is an important problem in experimental [[physical cosmology]]. |
|||
[[File:Primordial nucleosynthesis.svg|thumb|400px|Chief nuclear reactions responsible for the [[abundance of the chemical elements|relative abundances]] of light [[atomic nucleus|atomic nuclei]] observed throughout the universe.]] |
|||
Other experimental observations can be explained by combining the overall expansion of space with [[nuclear physics|nuclear]] and [[atomic physics]]. As the universe expands, the energy density of the [[electromagnetic radiation]] decreases more quickly than does that of [[matter]], since the energy of a photon decreases with its wavelength. Thus, although the energy density of the universe is now dominated by matter, it was once dominated by radiation; poetically speaking, all was [[light]]. As the universe expanded, its energy density decreased and it became cooler; as it did so, the [[elementary particle]]s of matter could associate stably into ever larger combinations. Thus, in the early part of the matter-dominated era, stable [[proton]]s and [[neutron]]s formed, which then associated into [[atomic nuclei]]. At this stage, the matter in the universe was mainly a hot, dense [[Plasma (physics)|plasma]] of negative [[electron]]s, neutral [[neutrino]]s and positive nuclei. [[Nuclear reaction]]s among the nuclei led to the present abundances of the lighter nuclei, particularly [[hydrogen]], [[deuterium]], and [[helium]]. Eventually, the electrons and nuclei combined to form stable atoms, which are transparent to most wavelengths of radiation; at this point, the radiation decoupled from the matter, forming the ubiquitous, isotropic background of microwave radiation observed today. |
|||
Other observations are not answered definitively by known physics. According to the prevailing theory, a slight imbalance of [[matter]] over [[antimatter]] was present in the universe's creation, or developed very shortly thereafter, possibly due to the [[CP violation]] that has been observed by [[particle physics|particle physicists]]. Although the matter and antimatter mostly annihilated one another, producing [[photon]]s, a small residue of matter survived, giving the present matter-dominated universe. Several lines of evidence also suggest that a rapid [[cosmic inflation]] of the universe occurred very early in its history (roughly 10<sup>−35</sup> seconds after its creation). Recent observations also suggest that the [[cosmological constant]] (''Λ'') is not zero and that the net [[mass-energy]] content of the universe is dominated by a [[dark energy]] and [[dark matter]] that have not been characterized scientifically. They differ in their gravitational effects. Dark matter gravitates as ordinary matter does, and thus slows the expansion of the universe; by contrast, dark energy serves to accelerate the universe's expansion. |
|||
===Multiverse theory=== |
|||
{{Main|Multiverse|Many-worlds interpretation|Bubble universe theory|Parallel universe (fiction)}} |
|||
[[File:Multiverse - level II.svg|thumb|Depiction of a [[multiverse]] of seven [[bubble universe theory|"bubble" universes]], which are separate [[spacetime]] continua, each having different [[physical law]]s, [[physical constant]]s, and perhaps even different numbers of [[dimension]]s or [[topology|topologies]].]] |
|||
Some speculative theories have proposed that this universe is but one of a [[set (mathematics)|set]] of disconnected universes, collectively denoted as the [[multiverse]], challenging or enhancing more limited definitions of the universe.<ref name="EllisKS03" /><ref>{{cite journal|author = Munitz MK|year = 1959|title = One Universe or Many?|journal = Journal of the History of Ideas|volume = 12|pages = 231–255|doi = 10.2307/2707516|issue = 2|jstor = 2707516}}</ref> Scientific multiverse theories are distinct from concepts such as [[plane (esotericism)|alternate planes of consciousness]] and [[simulated reality]], although the idea of a larger universe is not new; for example, Bishop [[Étienne Tempier]] of Paris ruled in 1277 that God could create as many universes as he saw fit, a question that was being hotly debated by the French theologians.<ref>Misner, Thorne and Wheeler (1973), p.753.</ref> |
|||
[[Max Tegmark]] developed a four part [[multiverse#Tegmark.27s_classification|classification scheme]] for the different types of multiverses that scientists have suggested in various problem domains. An example of such a theory is the [[bubble universe theory|chaotic inflation]] model of the early universe.<ref name="chaotic_inflation">{{cite journal|author = Linde A.|year = 1986|title = Eternal chaotic inflation|journal = Mod. Phys. Lett.|volume = A1|issue = 2|pages = 81–85|bibcode = 1986MPLA....1...81L |doi=10.1142/S0217732386000129|authorlink = Andrei Linde}}<br />{{cite journal|author = Linde A.|year = 1986|title = Eternally existing self-reproducing chaotic inflationary universe|journal = Phys. Lett.|volume = B175|issue=4|pages = 395–400|url=http://www.stanford.edu/~alinde/Eternal86.pdf |format=PDF|accessdate=17 March 2011|doi = 10.1016/0370-2693(86)90611-8 |bibcode = 1986PhLB..175..395L|authorlink = Andrei Linde}}</ref> Another is the [[many-worlds interpretation]] of quantum mechanics. Parallel worlds are generated in a manner similar to [[quantum superposition]] and [[decoherence]], with all states of the [[wave function]] being realized in separate worlds. Effectively, the multiverse evolves as a [[universal wavefunction]]. If the big bang that created our mutliverse created an ensemble of multiverses, the wave function of the ensemble would be entangled in this sense. |
|||
The least controversial category of multiverse in Tegmark's scheme is [[multiverse#Level_I:_Beyond_our_cosmological_horizon|Level I]], which describes distant space-time events "in our own universe". If space is infinite, or sufficiently large and uniform, identical instances of the history of Earth's entire [[Hubble volume]] occur every so often, simply by chance. Tegmark calculated our nearest so-called [[doppelgänger]], is 10<sup>10<sup>115</sup></sup> meters away from us (a [[double exponential function]] larger than a [[googolplex]]).<ref name="TegmarkPUstaple">"Parallel universes. Not just a staple of science fiction, other universes are a direct implication of cosmological observations.", Tegmark M., Sci Am. 2003 May;288(5):40-51.</ref><ref>{{cite journal |author1=Max Tegmark |journal=In "Science and Ultimate Reality: from Quantum to Cosmos", honoring John Wheeler's 90th birthday. J. D. Barrow, P.C.W. Davies, & C.L. Harper eds. Cambridge University Press (2003) |title=Parallel Universes |year=2003 |arxiv=astro-ph/0302131|bibcode = 2003astro.ph..2131T }}</ref> In principle, it would be impossible to scientifically verify an identical Hubble volume. However, it does follow as a fairly straightforward consequence from otherwise unrelated scientific observations and theories. Tegmark suggests that statistical analysis exploiting the [[anthropic principle]] provides an opportunity to test multiverse theories in some cases. Generally, science would consider a multiverse theory that posits neither a common point of causation, nor the possibility of interaction between universes, to be an ideal speculation. |
|||
==Shape of the universe== |
|||
{{Main|Shape of the universe}} |
|||
The shape or [[geometry]] of the universe includes both [[Shape of the universe#Local geometry (spatial curvature)|local geometry]] in the [[observable universe]] and [[Shape of the universe#Global geometry|global geometry]], which we may or may not be able to measure. Shape can refer to curvature and [[topology]]. More formally, the subject in practice investigates which [[3-manifold]] corresponds to the spatial section in [[comoving coordinates]] of the four-dimensional [[spacetime|space-time]] of the universe. Cosmologists normally work with a given [[space-like]] slice of spacetime called the [[Comoving distance|comoving coordinates]]. In terms of observation, the section of spacetime that can be observed is the backward [[light cone]] (points within the [[cosmic light horizon]], given time to reach a given observer). If the observable universe is smaller than the entire universe (in some models it is many orders of magnitude smaller), one cannot determine the global structure by observation: one is limited to a small patch. |
|||
Among the [[Friedmann–Lemaître–Robertson–Walker metric|Friedmann–Lemaître–Robertson–Walker]] (FLRW) models, the presently most popular shape of the Universe found to fit observational data according to cosmologists is the infinite flat model,<ref name="nasa_popular_uni_curv">[http://map.gsfc.nasa.gov/universe/uni_shape.html Shape of the Universe], WMAP website at NASA.</ref> while other FLRW models include the [[Homology_sphere#Cosmology|Poincaré dodecahedral space]]<ref name="Nat03">{{cite journal|last = Luminet|first = Jean-Pierre|authorlink = Jean-Pierre Luminet|coauthors = Jeff Weeks, Alain Riazuelo, Roland Lehoucq, Jean-Phillipe Uzan|title = Dodecahedral space topology as an explanation for weak wide-angle temperature correlations in the cosmic microwave background|journal = Nature|volume = 425|issue =6958|pages = 593–5 |date = 2003-10-09|arxiv = astro-ph/0310253|doi = 10.1038/nature01944|pmid = 14534579 |bibcode = 2003Natur.425..593L}}</ref><ref name="RBSG08">{{cite journal|last =Roukema|first =Boudewijn|coauthors = Zbigniew Buliński, Agnieszka Szaniewska, Nicolas E. Gaudin|title =A test of the Poincare dodecahedral space topology hypothesis with the WMAP CMB data|journal = Astronomy and Astrophysics|volume =482|issue = 3| pages =747|year = 2008|arxiv =0801.0006|doi =10.1051/0004-6361:20078777|bibcode=2008A&A...482..747L}}</ref> and the [[Picard horn]].<ref name="Aurich0403597">{{cite journal|last =Aurich|first =Ralf|coauthors =Lustig, S., Steiner, F., Then, H.|title =Hyperbolic Universes with a Horned Topology and the CMB Anisotropy|journal =Classical and Quantum Gravity|volume =21|issue = 21| pages =4901–4926|year =2004 |doi = 10.1088/0264-9381/21/21/010 |arxiv=astro-ph/0403597|bibcode=2004CQGra..21.4901A}}</ref> The data fit by these FLRW models of space especially include the [[Wilkinson Microwave Anisotropy Probe]] (WMAP) maps of cosmic background radiation. NASA released the first WMAP cosmic background radiation data in February 2003. In 2009 the [[Planck (spacecraft)|Planck observatory]] was launched to observe the microwave background at higher resolution than WMAP, possibly providing more information on the shape of the Universe. The data should be released in late 2012. |
|||
==See also== |
|||
{{Portal box|Astronomy|Space}} |
|||
{{Commons category|Universe}} |
|||
{{wikiquote}} |
|||
<div style="-moz-column-count:4; column-count:4;"> |
|||
* [[Cosmic latte]] |
|||
* [[Cosmology]] |
|||
* [[Dyson's eternal intelligence]] |
|||
* [[Esoteric cosmology]] |
|||
* [[False vacuum]] |
|||
* [[Final anthropic principle]] |
|||
* [[Fine-tuned Universe]] |
|||
* [[Hindu cycle of the universe]] |
|||
* [[Jain cosmology]] |
|||
* [[Kardashev scale]] |
|||
* ''[[The Mysterious Universe]]'' (book) |
|||
* [[Nucleocosmochronology]] |
|||
* [[Non-standard cosmology]] |
|||
* [[Observable universe]] |
|||
* [[Omega Point]] |
|||
* [[Omniverse]] |
|||
* [[Rare Earth hypothesis]] |
|||
* [[Vacuum genesis]] |
|||
* [[World view]] |
|||
* [[Zero-energy universe]] |
|||
</div> |
|||
==Notes and references== |
|||
{{Reflist|2}} |
|||
==Bibliography== |
|||
*{{cite journal|author = Bartel |authorlink = Bartel Leendert van der Waerden|year = 1987|title = The Heliocentric System in Greek, Persian and Hindu Astronomy|doi = 10.1111/j.1749-6632.1987.tb37224.x|bibcode = 1987NYASA.500..525V|journal = Annals of the New York Academy of Sciences|volume = 500|issue = 1| pages = 525–545}} |
|||
* {{cite book|author = [[Lev Landau|Landau, Lev]], [[Evgeny Lifshitz|Lifshitz, E.M.]]|year = 1975|title= The Classical Theory of Fields ([[Course of Theoretical Physics]], Vol. 2)|edition = revised 4th English|publisher=Pergamon Press|location=New York|isbn=9780080181769|pages=358–397}} |
|||
*Liddell, H. G. and Scott, R. ''A Greek-English Lexicon'', Oxford University Press, ISBN 0-19-864214-8 |
|||
* {{cite book|author = [[Charles W. Misner|Misner, C.W.]], [[Kip Thorne|Thorne, Kip]], [[John Archibald Wheeler|Wheeler, J.A.]]|title = [[Gravitation (book)|Gravitation]]|location = San Francisco|publisher = W. H. Freeman|year = 1973|isbn = 978-0-7167-0344-0|pages = 703–816}} |
|||
* {{cite book|author = Rindler, W.|year = 1977|title = Essential Relativity: Special, General, and Cosmological|publisher = Springer Verlag|location = New York|isbn = 0-387-10090-3|pages = 193–244|authorlink = Wolfgang Rindler}} |
|||
==Further reading== |
|||
* {{cite book|author = Weinberg, S.|year = 1993|title = The First Three Minutes: A Modern View of the Origin of the Universe|edition = 2nd updated|publisher = Basic Books|location = New York|isbn = 978-0465024377|oclc = 28746057|authorlink = Steven Weinberg}} For lay readers. |
|||
* {{cite book|first1=Harry|last1=Nussbaumer|first2=Lydia|last2=Bieri|first3=Allan|last3=Sandage|year = 2009|title= Discovering the Expanding Universe|publisher=Cambridge University Press|isbn=978-0-521-51484-2|url=http://books.google.com/books?id=RaNOJkQ4l14C}} |
|||
==External links== |
|||
{{Spoken Wikipedia|En-Universe.ogg|2007-07-07}} |
|||
* {{HSW|hole-in-universe|Is there a hole in the universe?}} |
|||
* [http://www.space.com/scienceastronomy/age_universe_030103.html Age of the Universe] at Space.Com |
|||
* [http://www.pbs.org/wnet/hawking/html/home.html ''Stephen Hawking's Universe''] – Why is the universe the way it is? |
|||
* [http://www.astro.ucla.edu/~wright/cosmology_faq.html Cosmology FAQ] |
|||
* [http://www.shekpvar.net/~dna/Publications/Cosmos/cosmos.html Cosmos – An "illustrated dimensional journey from microcosmos to macrocosmos"] |
|||
* [http://www.co-intelligence.org/newsletter/comparisons.html Illustration comparing the sizes of the planets, the sun, and other stars] |
|||
* [http://www.astro.princeton.edu/~mjuric/universe/ Logarithmic Maps of the Universe] |
|||
* [http://www.slate.com/id/2087206/nav/navoa/ My So-Called Universe] – Arguments for and against an infinite and parallel universes |
|||
* [http://www.hep.upenn.edu/~max/multiverse1.html Parallel Universes] by Max Tegmark |
|||
* [http://cosmology.lbl.gov/talks/Ho_07.pdf The Dark Side and the Bright Side of the Universe] Princeton University, Shirley Ho |
|||
* [http://www.atlasoftheuniverse.com/ Richard Powell: ''An Atlas of the Universe''] – Images at various scales, with explanations |
|||
* [http://www.npr.org/templates/story/story.php?storyId=1142346 Multiple Big Bangs] |
|||
* [http://www.exploreuniverse.com/ic/ Universe – Space Information Centre] |
|||
* [http://www.nasa.gov/topics/universe/index.html Exploring the Universe] at Nasa.gov |
|||
===Videos=== |
|||
* [http://www.youtube.com/embed/17jymDn0W6U The Known Universe] created by the [[American Museum of Natural History]] |
|||
* [http://www.youtube.com/embed/0fKBhvDjuy0 Understand The Size Of The Universe] – by [[Powers of Ten]] |
|||
{{Earth's location}} |
|||
{{Nature nav}} |
|||
[[Category:Environments]] |
|||
[[Category:Universe| ]] |
|||
[[af:Heelal]] |
|||
[[ar:فضاء كوني]] |
|||
[[an:Universo]] |
|||
[[arc:ܬܒܝܠ]] |
|||
[[ast:Universu]] |
|||
[[az:Kainat]] |
|||
[[bn:মহাবিশ্ব]] |
|||
[[zh-min-nan:Ú-tiū]] |
|||
[[be:Сусвет]] |
|||
[[be-x-old:Сусьвет]] |
|||
[[bs:Svemir]] |
|||
[[bg:Вселена]] |
|||
[[ca:Univers]] |
|||
[[cv:Çут Тĕнче]] |
|||
[[cs:Vesmír]] |
|||
[[cy:Bydysawd (seryddiaeth)]] |
|||
[[da:Universet]] |
|||
[[de:Universum]] |
|||
[[nv:Yágháhookáán]] |
|||
[[dsb:Uniwersum]] |
|||
[[et:Universum]] |
|||
[[el:Σύμπαν]] |
|||
[[es:Universo]] |
|||
[[eo:Universo]] |
|||
[[eu:Unibertso]] |
|||
[[fa:گیتی]] |
|||
[[hif:Sansaar]] |
|||
[[fo:Alheimurin]] |
|||
[[fr:Univers]] |
|||
[[fy:Hielal]] |
|||
[[gl:Universo]] |
|||
[[gu:બ્રહ્માંડ]] |
|||
[[hak:Yî-chhiu]] |
|||
[[ko:우주]] |
|||
[[hi:ब्रह्माण्ड]] |
|||
[[hr:Svemir]] |
|||
[[io:Universo]] |
|||
[[id:Alam semesta]] |
|||
[[ia:Universo]] |
|||
[[is:Alheimurinn]] |
|||
[[it:Universo]] |
|||
[[he:היקום]] |
|||
[[jv:Alam semesta]] |
|||
[[kn:ಬ್ರಹ್ಮಾಂಡ]] |
|||
[[pam:Sikluban]] |
|||
[[ka:სამყარო]] |
|||
[[csb:Swiatnica]] |
|||
[[kk:Әлем]] |
|||
[[sw:Ulimwengu]] |
|||
[[ht:Linivè]] |
|||
[[ku:Gerdûn]] |
|||
[[la:Universum]] |
|||
[[lv:Visums]] |
|||
[[lt:Visata]] |
|||
[[li:Universum]] |
|||
[[lmo:Ünivers]] |
|||
[[hu:Világegyetem]] |
|||
[[mk:Вселена]] |
|||
[[ml:പ്രപഞ്ചം]] |
|||
[[mr:विश्व]] |
|||
[[arz:كون]] |
|||
[[ms:Alam semesta]] |
|||
[[mwl:Ouniberso]] |
|||
[[mn:Ертөнц]] |
|||
[[my:စကြဝဠာ]] |
|||
[[nah:Cemānāhuac]] |
|||
[[nl:Heelal]] |
|||
[[nds-nl:Hielal]] |
|||
[[ne:ब्रह्माण्ड]] |
|||
[[ja:宇宙]] |
|||
[[nap:Annevierzo]] |
|||
[[frr:Åål]] |
|||
[[no:Universet]] |
|||
[[nn:Universet]] |
|||
[[nrm:Eunivers]] |
|||
[[nov:Universe]] |
|||
[[oc:Univèrs]] |
|||
[[uz:Olam]] |
|||
[[pfl:Weldall]] |
|||
[[pnb:کائنات]] |
|||
[[pap:Universo]] |
|||
[[nds:Weltruum]] |
|||
[[pl:Wszechświat]] |
|||
[[pt:Universo]] |
|||
[[ro:Univers]] |
|||
[[qu:Ch'askancha]] |
|||
[[rue:Весмір]] |
|||
[[ru:Вселенная]] |
|||
[[stq:Al]] |
|||
[[sq:Gjithësia]] |
|||
[[scn:Universu]] |
|||
[[simple:Universe]] |
|||
[[sk:Vesmír]] |
|||
[[sl:Vesolje]] |
|||
[[ckb:گەردوون]] |
|||
[[sr:Свемир]] |
|||
[[sh:Svemir]] |
|||
[[su:Jagat]] |
|||
[[fi:Maailmankaikkeus]] |
|||
[[sv:Universum]] |
|||
[[sn:Rudunhumwe]] |
|||
[[tl:Sanlibutan]] |
|||
[[ta:அண்டம்]] |
|||
[[te:విశ్వం]] |
|||
[[th:เอกภพ]] |
|||
[[tg:Коинот]] |
|||
[[tr:Evren]] |
|||
[[uk:Всесвіт]] |
|||
[[ur:کائنات]] |
|||
[[vi:Vũ trụ]] |
|||
[[zh-classical:宇宙]] |
|||
[[war:Sangkalibutan]] |
|||
[[yi:אוניווערס]] |
|||
[[zh-yue:宇宙]] |
|||
[[bat-smg:Vėsata]] |
|||
[[zh:宇宙]] |
Revision as of 14:50, 18 November 2011
Part of a series on |
Physical cosmology |
---|
![]() |
The Universe is commonly defined as the totality of everything that exists,[1] including all matter and energy, the planets, stars, galaxies, and the contents of intergalactic space.[2][3] Definitions and usage vary and similar terms include the cosmos, the world and nature. Scientific observation of earlier stages in the development of the Universe, which can be seen at great distances, suggests that the Universe has been governed by the same physical laws and constants throughout most of its extent and history. There are various multiverse theories, in which physicists have suggested that our universe is one among many universes that likewise exist.[4][5]
History
Throughout recorded history, several cosmologies and cosmogonies have been proposed to account for observations of the universe. The earliest quantitative geocentric models were developed by the ancient Greek philosophers. Over the centuries, more precise observations and improved theories of gravity led to Copernicus's heliocentric model and the Newtonian model of the Solar System, respectively. Further improvements in astronomy led to the realization that the Solar System is embedded in a galaxy composed of billions of stars, the Milky Way, and that other galaxies exist outside it, as far as astronomical instruments can reach. Careful studies of the distribution of these galaxies and their spectral lines have led to much of modern cosmology. Discovery of the red shift and cosmic microwave background radiation revealed that the universe is expanding and apparently had a beginning.[citation needed]

According to the prevailing scientific model of the universe, known as the Big Bang, the universe expanded from an extremely hot, dense phase called the Planck epoch, in which all the matter and energy of the observable universe was concentrated. Since the Planck epoch, the universe has been expanding to its present form, possibly with a brief period (less than 10−32 seconds) of cosmic inflation. Several independent experimental measurements support this theoretical expansion and, more generally, the Big Bang theory. Recent observations indicate that this expansion is accelerating because of dark energy, and that most of the matter in the universe may be in a form which cannot be detected by present instruments, and so is not accounted for in the present models of the universe; this has been named dark matter. The imprecision of current observations has hindered predictions of the ultimate fate of the universe.[citation needed]
Current interpretations of astronomical observations indicate that the age of the universe is 13.75 ± 0.17 billion years,[6] and that the diameter of the observable universe is at least 93 billion light years or 8.80×1026 metres.[7] According to general relativity, space can expand faster than the speed of light, although we can view only a small portion of the universe due to the limitation imposed by light speed. Since we cannot observe space beyond the limitations of light (or any electromagnetic radiation), it is uncertain whether the size of the universe is finite or infinite.
Etymology, synonyms and definitions
The word universe derives from the Old French word Univers, which in turn derives from the Latin word universum.[8] The Latin word was used by Cicero and later Latin authors in many of the same senses as the modern English word is used.[9] The Latin word derives from the poetic contraction Unvorsum — first used by Lucretius in Book IV (line 262) of his De rerum natura (On the Nature of Things) — which connects un, uni (the combining form of unus', or "one") with vorsum, versum (a noun made from the perfect passive participle of vertere, meaning "something rotated, rolled, changed").[9]

An alternative interpretation of unvorsum is "everything rotated as one" or "everything rotated by one". In this sense, it may be considered a translation of an earlier Greek word for the universe, περιφορά, (periforá, "circumambulation"), originally used to describe a course of a meal, the food being carried around the circle of dinner guests.[10] This Greek word refers to celestial spheres, an early Greek model of the universe. Regarding Plato's Metaphor of the sun, Aristotle suggests that the rotation of the sphere of fixed stars inspired by the prime mover, motivates, in turn, terrestrial change via the Sun. Careful astronomical and physical measurements (such as the Foucault pendulum) are required to prove the Earth rotates on its axis.
A term for "universe" in ancient Greece was τὸ πᾶν (tó pán, The All, Pan (mythology)). Related terms were matter, ( τὸ ὅλον, tó ólon, see also Hyle, lit. wood) and place ( τὸ κενόν, tó kenón).[11][12] Other synonyms for the universe among the ancient Greek philosophers included κόσμος (cosmos) and φύσις (meaning Nature, from which we derive the word physics).[13] The same synonyms are found in Latin authors (totum, mundus, natura)[14] and survive in modern languages, e.g., the German words Das All, Weltall, and Natur for universe. The same synonyms are found in English, such as everything (as in the theory of everything), the cosmos (as in cosmology), the world (as in the many-worlds hypothesis), and Nature (as in natural laws or natural philosophy).[15]
Broadest definition: reality and probability
The broadest definition of the universe can be found in De divisione naturae by the medieval philosopher and theologian Johannes Scotus Eriugena, who defined it as simply everything: everything that is created and everything that is not created. In the path integral formulation of Richard Feynman,[16] the probability amplitudes for the various outcomes of an experiment given a perfectly defined initial state of the system are determined by summing over all possible histories (paths) by which the system could progress from the initial to final state.
Definition as reality
More customarily, the universe is defined as everything that exists, (has existed, and will exist) [citation needed]. According to our current understanding, the universe consists of three principles: spacetime, forms of energy, including momentum and matter, and the physical laws that relate them.
Definition as connected space-time
It is possible to conceive of disconnected space-times, each existing but unable to interact with one another. An easily visualized metaphor is a group of separate soap bubbles, in which observers living on one soap bubble cannot interact with those on other soap bubbles, even in principle. According to one common terminology, each "soap bubble" of space-time is denoted as a universe, whereas our particular space-time is denoted as the universe, just as we call our moon the Moon. The entire collection of these separate space-times is denoted as the multiverse.[17] In principle, the other unconnected universes may have different dimensionalities and topologies of space-time, different forms of matter and energy, and different physical laws and physical constants, although such possibilities are currently speculative.
Definition as observable reality
According to a still-more-restrictive definition, the universe is everything within our connected space-time that could have a chance to interact with us and vice versa.[citation needed] According to the general theory of relativity, some regions of space may never interact with ours even in the lifetime of the universe, due to the finite speed of light and the ongoing expansion of space. For example, radio messages sent from Earth may never reach some regions of space, even if the universe would live forever; space may expand faster than light can traverse it. It is worth emphasizing that those distant regions of space are taken to exist and be part of reality as much as we are; yet we can never interact with them. The spatial region within which we can affect and be affected is denoted as the observable universe. Strictly speaking, the observable universe depends on the location of the observer. By traveling, an observer can come into contact with a greater region of space-time than an observer who remains still, so that the observable universe for the former is larger than for the latter. Nevertheless, even the most rapid traveler will not be able to interact with all of space. Typically, the observable universe is taken to mean the universe observable from our vantage point in the Milky Way Galaxy.
Size, age, contents, structure, and laws
The universe is immensely large and possibly infinite in volume. The region visible from Earth (the observable universe) is a sphere with a radius of about 46 billion light years,[18] based on where the expansion of space has taken the most distant objects observed. For comparison, the diameter of a typical galaxy is only 30,000 light-years, and the typical distance between two neighboring galaxies is only 3 million light-years.[19] As an example, our Milky Way Galaxy is roughly 100,000 light years in diameter,[20] and our nearest sister galaxy, the Andromeda Galaxy, is located roughly 2.5 million light years away.[21] There are probably more than 100 billion (1011) galaxies in the observable universe.[22] Typical galaxies range from dwarfs with as few as ten million[23] (107) stars up to giants with one trillion[24] (1012) stars, all orbiting the galaxy's center of mass. A 2010 study by astronomers estimated that the observable universe contains 300 sextillion (3×1023) stars.[25]

The observable matter is spread homogeneously (uniformly) throughout the universe, when averaged over distances longer than 300 million light-years.[26] However, on smaller length-scales, matter is observed to form "clumps", i.e., to cluster hierarchically; many atoms are condensed into stars, most stars into galaxies, most galaxies into clusters, superclusters and, finally, the largest-scale structures such as the Great Wall of galaxies. The observable matter of the universe is also spread isotropically, meaning that no direction of observation seems different from any other; each region of the sky has roughly the same content.[27] The universe is also bathed in a highly isotropic microwave radiation that corresponds to a thermal equilibrium blackbody spectrum of roughly 2.725-kelvins.[28] The hypothesis that the large-scale universe is homogeneous and isotropic is known as the cosmological principle,[29] which is supported by astronomical observations.
The present overall density of the universe is very low, roughly 9.9 × 10−30 grams per cubic centimetre. This mass-energy appears to consist of 73% dark energy, 23% cold dark matter and 4% ordinary matter. Thus the density of atoms is on the order of a single hydrogen atom for every four cubic meters of volume.[30] The properties of dark energy and dark matter are largely unknown. Dark matter gravitates as ordinary matter, and thus works to slow the expansion of the universe; by contrast, dark energy accelerates its expansion.
The most precise estimate of the universe's age is 13.73±0.12 billion years old, based on observations of the cosmic microwave background radiation.[31] Independent estimates (based on measurements such as radioactive dating) agree, although they are less precise, ranging from 11 to 20 billion years[dead link ][32] to 13–15 billion years.[33] The universe has not been the same at all times in its history; for example, the relative populations of quasars and galaxies have changed and space itself appears to have expanded. This expansion accounts for how Earth-bound scientists can observe the light from a galaxy 30 billion light years away, even if that light has traveled for only 13 billion years; the very space between them has expanded. This expansion is consistent with the observation that the light from distant galaxies has been redshifted; the photons emitted have been stretched to longer wavelengths and lower frequency during their journey. The rate of this spatial expansion is accelerating, based on studies of Type Ia supernovae and corroborated by other data.
The relative fractions of different chemical elements — particularly the lightest atoms such as hydrogen, deuterium and helium — seem to be identical throughout the universe and throughout its observable history.[34] The universe seems to have much more matter than antimatter, an asymmetry possibly related to the observations of CP violation.[35] The universe appears to have no net electric charge, and therefore gravity appears to be the dominant interaction on cosmological length scales. The universe also appears to have neither net momentum nor angular momentum. The absence of net charge and momentum would follow from accepted physical laws (Gauss's law and the non-divergence of the stress-energy-momentum pseudotensor, respectively), if the universe were finite.[36]

The universe appears to have a smooth space-time continuum consisting of three spatial dimensions and one temporal (time) dimension. On the average, space is observed to be very nearly flat (close to zero curvature), meaning that Euclidean geometry is experimentally true with high accuracy throughout most of the Universe.[37] Spacetime also appears to have a simply connected topology, at least on the length-scale of the observable universe. However, present observations cannot exclude the possibilities that the universe has more dimensions and that its spacetime may have a multiply connected global topology, in analogy with the cylindrical or toroidal topologies of two-dimensional spaces.[38]
The universe appears to behave in a manner that regularly follows a set of physical laws and physical constants.[39] According to the prevailing Standard Model of physics, all matter is composed of three generations of leptons and quarks, both of which are fermions. These elementary particles interact via at most three fundamental interactions: the electroweak interaction which includes electromagnetism and the weak nuclear force; the strong nuclear force described by quantum chromodynamics; and gravity, which is best described at present by general relativity. The first two interactions can be described by renormalized quantum field theory, and are mediated by gauge bosons that correspond to a particular type of gauge symmetry. A renormalized quantum field theory of general relativity has not yet been achieved, although various forms of string theory seem promising. The theory of special relativity is believed to hold throughout the universe, provided that the spatial and temporal length scales are sufficiently short; otherwise, the more general theory of general relativity must be applied. There is no explanation for the particular values that physical constants appear to have throughout our universe, such as Planck's constant h or the gravitational constant G. Several conservation laws have been identified, such as the conservation of charge, momentum, angular momentum and energy; in many cases, these conservation laws can be related to symmetries or mathematical identities.
Fine tuning
It appears that many of the properties of the universe have special values in the sense that a universe where these properties only differ slightly would not be able to support intelligent life.[40][41] Not all scientists agree that this fine-tuning exists.[42][43] In particular, it is not known under what conditions intelligent life could form and what form or shape that would take. A relevant observation in this discussion is that for an observer to exist to observe fine-tuning, the universe must be able to support intelligent life. As such the conditional probability of observing a universe that is fine-tuned to support intelligent life is 1. This observation is known as the anthropic principle and is particularly relevant if the creation of the universe was probabilistic or if multiple universes with a variety of properties exist (see below).
Historical models
Many models of the cosmos (cosmologies) and its origin (cosmogonies) have been proposed, based on the then-available data and conceptions of the universe. Historically, cosmologies and cosmogonies were based on narratives of gods acting in various ways. Theories of an impersonal universe governed by physical laws were first proposed by the Greeks and Indians. Over the centuries, improvements in astronomical observations and theories of motion and gravitation led to ever more accurate descriptions of the universe. The modern era of cosmology began with Albert Einstein's 1915 general theory of relativity, which made it possible to quantitatively predict the origin, evolution, and conclusion of the universe as a whole. Most modern, accepted theories of cosmology are based on general relativity and, more specifically, the predicted Big Bang; however, still more careful measurements are required to determine which theory is correct.
Creation
Many cultures have stories describing the origin of the world, which may be roughly grouped into common types. In one type of story, the world is born from a world egg; such stories include the Finnish epic poem Kalevala, the Chinese story of Pangu or the Indian Brahmanda Purana. In related stories, the creation idea is caused by a single entity emanating or producing something by him- or herself, as in the Tibetan Buddhism concept of Adi-Buddha, the ancient Greek story of Gaia (Mother Earth), the Aztec goddess Coatlicue myth, the ancient Egyptian god Atum story, or the Genesis creation narrative. In another type of story, the world is created from the union of male and female deities, as in the Maori story of Rangi and Papa. In other stories, the universe is created by crafting it from pre-existing materials, such as the corpse of a dead god — as from Tiamat in the Babylonian epic Enuma Elish or from the giant Ymir in Norse mythology – or from chaotic materials, as in Izanagi and Izanami in Japanese mythology. In other stories, the universe emanates from fundamental principles, such as Brahman and Prakrti, or the yin and yang of the Tao.
Philosophical models
From the 6th century BCE, the pre-Socratic Greek philosophers developed the earliest known philosophical models of the universe. The earliest Greek philosophers noted that appearances can be deceiving, and sought to understand the underlying reality behind the appearances. In particular, they noted the ability of matter to change forms (e.g., ice to water to steam) and several philosophers proposed that all the apparently different materials of the world are different forms of a single primordial material, or arche. The first to do so was Thales, who proposed this material is Water. Thales' student, Anaximander, proposed that everything came from the limitless apeiron. Anaximenes proposed Air on account of its perceived attractive and repulsive qualities that cause the arche to condense or dissociate into different forms. Anaxagoras, proposed the principle of Nous (Mind). Heraclitus proposed fire (and spoke of logos). Empedocles proposed the elements: earth, wind, air and fire. His four element theory became very popular. Like Pythagoras, Plato believed that all things were composed of number, with the Empedocles' elements taking the form of the Platonic solids. Democritus, and later philosophers—most notably Leucippus—proposed that the universe was composed of indivisible atoms moving through void (vacuum). Aristotle did not believe that was feasible because air, like water, offers resistance to motion. Air will immediately rush in to fill a void, and moreover, without resistance, it would do so indefinitely fast.
Although Heraclitus argued for eternal change, his quasi-contemporary Parmenides made the radical suggestion that all change is an illusion, that the true underlying reality is eternally unchanging and of a single nature. Parmenides denoted this reality as τὸ ἐν (The One). Parmenides' theory seemed implausible to many Greeks, but his student Zeno of Elea challenged them with several famous paradoxes. Aristotle responded to these paradoxes by developing the notion of a potential countable infinity, as well as the infinitely divisible continuum. Unlike the eternal and unchanging cycles of time, he believed the world was bounded by the celestial spheres, and thus magnitude was only finitely multiplicative.
The Indian philosopher Kanada, founder of the Vaisheshika school, developed a theory of atomism and proposed that light and heat were varieties of the same substance.[44] In the 5th century AD, the Buddhist atomist philosopher Dignāga proposed atoms to be point-sized, durationless, and made of energy. They denied the existence of substantial matter and proposed that movement consisted of momentary flashes of a stream of energy.[45]
The theory of temporal finitism was inspired by the doctrine of Creation shared by the three Abrahamic religions: Judaism, Christianity and Islam. The Christian philosopher, John Philoponus, presented the philosophical arguments against the ancient Greek notion of an infinite past and future. Philoponus' arguments against an infinite past were used by the early Muslim philosopher, Al-Kindi (Alkindus); the Jewish philosopher, Saadia Gaon (Saadia ben Joseph); and the Muslim theologian, Al-Ghazali (Algazel). Borrowing from Aristotle's Physics and Metaphysics, they employed two logical arguments against an infinite past, the first being the "argument from the impossibility of the existence of an actual infinite", which states:[46]
- "An actual infinite cannot exist."
- "An infinite temporal regress of events is an actual infinite."
- " An infinite temporal regress of events cannot exist."
The second argument, the "argument from the impossibility of completing an actual infinite by successive addition", states:[46]
- "An actual infinite cannot be completed by successive addition."
- "The temporal series of past events has been completed by successive addition."
- " The temporal series of past events cannot be an actual infinite."
Both arguments were adopted by Christian philosophers and theologians, and the second argument in particular became more famous after it was adopted by Immanuel Kant in his thesis of the first antinomy concerning time.[46]
Astronomical models
Astronomical models of the universe were proposed soon after astronomy began with the Babylonian astronomers, who viewed the universe as a flat disk floating in the ocean, and this forms the premise for early Greek maps like those of Anaximander and Hecataeus of Miletus.
Later Greek philosophers, observing the motions of the heavenly bodies, were concerned with developing models of the universe based more profoundly on empirical evidence. The first coherent model was proposed by Eudoxus of Cnidos. According to Aristotle's physical interpretation of the model, celestial spheres eternally rotate with uniform motion around a stationary Earth. Normal matter, is entirely contained within the terrestrial sphere. This model was also refined by Callippus and after concentric spheres were abandoned, it was brought into nearly perfect agreement with astronomical observations by Ptolemy. The success of such a model is largely due to the mathematical fact that any function (such as the position of a planet) can be decomposed into a set of circular functions (the Fourier modes). Other Greek scientists, such as the Pythagorean philosopher Philolaus postulated that at the center of the universe was a "central fire" around which the Earth, Sun, Moon and Planets revolved in uniform circular motion.[47] The Greek astronomer Aristarchus of Samos was the first known individual to propose a heliocentric model of the universe. Though the original text has been lost, a reference in Archimedes' book The Sand Reckoner describes Aristarchus' heliocentric theory. Archimedes wrote: (translated into English)
You King Gelon are aware the 'universe' is the name given by most astronomers to the sphere the center of which is the center of the Earth, while its radius is equal to the straight line between the center of the Sun and the center of the Earth. This is the common account as you have heard from astronomers. But Aristarchus has brought out a book consisting of certain hypotheses, wherein it appears, as a consequence of the assumptions made, that the universe is many times greater than the 'universe' just mentioned. His hypotheses are that the fixed stars and the Sun remain unmoved, that the Earth revolves about the Sun on the circumference of a circle, the Sun lying in the middle of the orbit, and that the sphere of fixed stars, situated about the same center as the Sun, is so great that the circle in which he supposes the Earth to revolve bears such a proportion to the distance of the fixed stars as the center of the sphere bears to its surface.
Aristarchus thus believed the stars to be very far away, and saw this as the reason why there was no visible parallax, that is, an observed movement of the stars relative to each other as the Earth moved around the Sun. The stars are in fact much farther away than the distance that was generally assumed in ancient times, which is why stellar parallax is only detectable with telescopes. The geocentric model, consistent with planetary parallax, was assumed to be an explanation for the unobservability of the parallel phenomenon, stellar parallax. The rejection of the heliocentric view was apparently quite strong, as the following passage from Plutarch suggests (On the Apparent Face in the Orb of the Moon):
Cleanthes [a contemporary of Aristarchus and head of the Stoics] thought it was the duty of the Greeks to indict Aristarchus of Samos on the charge of impiety for putting in motion the Hearth of the universe [i.e. the earth], . . . supposing the heaven to remain at rest and the earth to revolve in an oblique circle, while it rotates, at the same time, about its own axis. [1]
The only other astronomer from antiquity known by name who supported Aristarchus' heliocentric model was Seleucus of Seleucia, a Hellenized Babylonian astronomer who lived a century after Aristarchus.[48][49][50] According to Plutarch, Seleucus was the first to prove the heliocentric system through reasoning, but it is not known what arguments he used. Seleucus' arguments for a heliocentric theory were probably related to the phenomenon of tides.[51] According to Strabo (1.1.9), Seleucus was the first to state that the tides are due to the attraction of the Moon, and that the height of the tides depends on the Moon's position relative to the Sun.[52] Alternatively, he may have proved the heliocentric theory by determining the constants of a geometric model for the heliocentric theory and by developing methods to compute planetary positions using this model, like what Nicolaus Copernicus later did in the 16th century.[53] During the Middle Ages, heliocentric models may have also been proposed by the Indian astronomer, Aryabhata,[54] and by the Persian astronomers, Albumasar[55] and Al-Sijzi.[56]
The Aristotelian model was accepted in the Western world for roughly two millennia, until Copernicus revived Aristarchus' theory that the astronomical data could be explained more plausibly if the earth rotated on its axis and if the sun were placed at the center of the universe.
In the center rests the sun. For who would place this lamp of a very beautiful temple in another or better place than this wherefrom it can illuminate everything at the same time?
— Nicolaus Copernicus, in Chapter 10, Book 1 of De Revolutionibus Orbium Coelestrum (1543)
As noted by Copernicus himself, the suggestion that the Earth rotates was very old, dating at least to Philolaus (c. 450 BC), Heraclides Ponticus (c. 350 BC) and Ecphantus the Pythagorean. Roughly a century before Copernicus, Christian scholar Nicholas of Cusa also proposed that the Earth rotates on its axis in his book, On Learned Ignorance (1440).[57] Aryabhata (476–550), Brahmagupta (598–668), Albumasar and Al-Sijzi, also proposed that the Earth rotates on its axis.[citation needed] The first empirical evidence for the Earth's rotation on its axis, using the phenomenon of comets, was given by Tusi (1201–1274) and Ali Qushji (1403–1474).[citation needed]

This cosmology was accepted by Isaac Newton, Christiaan Huygens and later scientists.[58] Edmund Halley (1720)[59] and Jean-Philippe de Cheseaux (1744)[60] noted independently that the assumption of an infinite space filled uniformly with stars would lead to the prediction that the nighttime sky would be as bright as the sun itself; this became known as Olbers' paradox in the 19th century.[61] Newton believed that an infinite space uniformly filled with matter would cause infinite forces and instabilities causing the matter to be crushed inwards under its own gravity.[58] This instability was clarified in 1902 by the Jeans instability criterion.[62] One solution to these paradoxes is the Charlier universe, in which the matter is arranged hierarchically (systems of orbiting bodies that are themselves orbiting in a larger system, ad infinitum) in a fractal way such that the universe has a negligibly small overall density; such a cosmological model had also been proposed earlier in 1761 by Johann Heinrich Lambert.[63] A significant astronomical advance of the 18th century was the realization by Thomas Wright, Immanuel Kant and others of nebulae.[64]
The modern era of physical cosmology began in 1917, when Albert Einstein first applied his general theory of relativity to model the structure and dynamics of the universe.[65]
Theoretical models
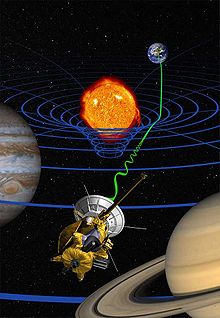
Of the four fundamental interactions, gravitation is dominant at cosmological length scales; that is, the other three forces are believed to play a negligible role in determining structures at the level of planets, stars, galaxies and larger-scale structures. Since all matter and energy gravitate, gravity's effects are cumulative; by contrast, the effects of positive and negative charges tend to cancel one another, making electromagnetism relatively insignificant on cosmological length scales. The remaining two interactions, the weak and strong nuclear forces, decline very rapidly with distance; their effects are confined mainly to sub-atomic length scales.
General theory of relativity
Given gravitation's predominance in shaping cosmological structures, accurate predictions of the universe's past and future require an accurate theory of gravitation. The best theory available is Albert Einstein's general theory of relativity, which has passed all experimental tests hitherto. However, since rigorous experiments have not been carried out on cosmological length scales, general relativity could conceivably be inaccurate. Nevertheless, its cosmological predictions appear to be consistent with observations, so there is no compelling reason to adopt another theory.
General relativity provides a set of ten nonlinear partial differential equations for the spacetime metric (Einstein's field equations) that must be solved from the distribution of mass-energy and momentum throughout the universe. Since these are unknown in exact detail, cosmological models have been based on the cosmological principle, which states that the universe is homogeneous and isotropic. In effect, this principle asserts that the gravitational effects of the various galaxies making up the universe are equivalent to those of a fine dust distributed uniformly throughout the universe with the same average density. The assumption of a uniform dust makes it easy to solve Einstein's field equations and predict the past and future of the universe on cosmological time scales.
Einstein's field equations include a cosmological constant (Λ),[65][66] that corresponds to an energy density of empty space.[67] Depending on its sign, the cosmological constant can either slow (negative Λ) or accelerate (positive Λ) the expansion of the universe. Although many scientists, including Einstein, had speculated that Λ was zero,[68] recent astronomical observations of type Ia supernovae have detected a large amount of "dark energy" that is accelerating the universe's expansion.[69] Preliminary studies suggest that this dark energy corresponds to a positive Λ, although alternative theories cannot be ruled out as yet.[70] Russian physicist Zel'dovich suggested that Λ is a measure of the zero-point energy associated with virtual particles of quantum field theory, a pervasive vacuum energy that exists everywhere, even in empty space.[71] Evidence for such zero-point energy is observed in the Casimir effect.
Special relativity and space-time
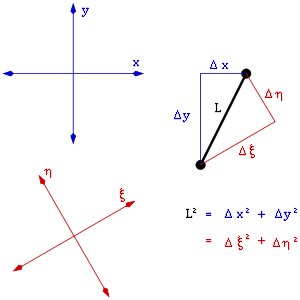
The universe has at least three spatial and one temporal (time) dimension. It was long thought that the spatial and temporal dimensions were different in nature and independent of one another. However, according to the special theory of relativity, spatial and temporal separations are interconvertible (within limits) by changing one's motion.
To understand this interconversion, it is helpful to consider the analogous interconversion of spatial separations along the three spatial dimensions. Consider the two endpoints of a rod of length L. The length can be determined from the differences in the three coordinates Δx, Δy and Δz of the two endpoints in a given reference frame
using the Pythagorean theorem. In a rotated reference frame, the coordinate differences differ, but they give the same length
Thus, the coordinates differences (Δx, Δy, Δz) and (Δξ, Δη, Δζ) are not intrinsic to the rod, but merely reflect the reference frame used to describe it; by contrast, the length L is an intrinsic property of the rod. The coordinate differences can be changed without affecting the rod, by rotating one's reference frame.
The analogy in spacetime is called the interval between two events; an event is defined as a point in spacetime, a specific position in space and a specific moment in time. The spacetime interval between two events is given by
where c is the speed of light. According to special relativity, one can change a spatial and time separation (L1, Δt1) into another (L2, Δt2) by changing one's reference frame, as long as the change maintains the spacetime interval s. Such a change in reference frame corresponds to changing one's motion; in a moving frame, lengths and times are different from their counterparts in a stationary reference frame. The precise manner in which the coordinate and time differences change with motion is described by the Lorentz transformation.
Solving Einstein's field equations
The distances between the spinning galaxies increase with time, but the distances between the stars within each galaxy stay roughly the same, due to their gravitational interactions. This animation illustrates a closed Friedmann universe with zero cosmological constant Λ; such a universe oscillates between a Big Bang and a Big Crunch.
In non-Cartesian (non-square) or curved coordinate systems, the Pythagorean theorem holds only on infinitesimal length scales and must be augmented with a more general metric tensor gμν, which can vary from place to place and which describes the local geometry in the particular coordinate system. However, assuming the cosmological principle that the universe is homogeneous and isotropic everywhere, every point in space is like every other point; hence, the metric tensor must be the same everywhere. That leads to a single form for the metric tensor, called the Friedmann–Lemaître–Robertson–Walker metric
where (r, θ, φ) correspond to a spherical coordinate system. This metric has only two undetermined parameters: an overall length scale R that can vary with time, and a curvature index k that can be only 0, 1 or −1, corresponding to flat Euclidean geometry, or spaces of positive or negative curvature. In cosmology, solving for the history of the universe is done by calculating R as a function of time, given k and the value of the cosmological constant Λ, which is a (small) parameter in Einstein's field equations. The equation describing how R varies with time is known as the Friedmann equation, after its inventor, Alexander Friedmann.[72]
The solutions for R(t) depend on k and Λ, but some qualitative features of such solutions are general. First and most importantly, the length scale R of the universe can remain constant only if the universe is perfectly isotropic with positive curvature (k=1) and has one precise value of density everywhere, as first noted by Albert Einstein. However, this equilibrium is unstable and since the universe is known to be inhomogeneous on smaller scales, R must change, according to general relativity. When R changes, all the spatial distances in the universe change in tandem; there is an overall expansion or contraction of space itself. This accounts for the observation that galaxies appear to be flying apart; the space between them is stretching. The stretching of space also accounts for the apparent paradox that two galaxies can be 40 billion light years apart, although they started from the same point 13.7 billion years ago and never moved faster than the speed of light.
Second, all solutions suggest that there was a gravitational singularity in the past, when R goes to zero and matter and energy became infinitely dense. It may seem that this conclusion is uncertain since it is based on the questionable assumptions of perfect homogeneity and isotropy (the cosmological principle) and that only the gravitational interaction is significant. However, the Penrose–Hawking singularity theorems show that a singularity should exist for very general conditions. Hence, according to Einstein's field equations, R grew rapidly from an unimaginably hot, dense state that existed immediately following this singularity (when R had a small, finite value); this is the essence of the Big Bang model of the universe. A common misconception is that the Big Bang model predicts that matter and energy exploded from a single point in space and time; that is false. Rather, space itself was created in the Big Bang and imbued with a fixed amount of energy and matter distributed uniformly throughout; as space expands (i.e., as R(t) increases), the density of that matter and energy decreases.
Space has no boundary – that is empirically more certain than any external observation. However, that does not imply that space is infinite...(translated, original German) |
Bernhard Riemann (Habilitationsvortrag, 1854) |
Third, the curvature index k determines the sign of the mean spatial curvature of spacetime averaged over length scales greater than a billion light years. If k=1, the curvature is positive and the universe has a finite volume. Such universes are often visualized as a three-dimensional sphere S3 embedded in a four-dimensional space. Conversely, if k is zero or negative, the universe may have infinite volume, depending on its overall topology. It may seem counter-intuitive that an infinite and yet infinitely dense universe could be created in a single instant at the Big Bang when R=0, but exactly that is predicted mathematically when k does not equal 1. For comparison, an infinite plane has zero curvature but infinite area, whereas an infinite cylinder is finite in one direction and a torus is finite in both. A toroidal universe could behave like a normal universe with periodic boundary conditions, as seen in "wrap-around" video games such as Asteroids; a traveler crossing an outer "boundary" of space going outwards would reappear instantly at another point on the boundary moving inwards.
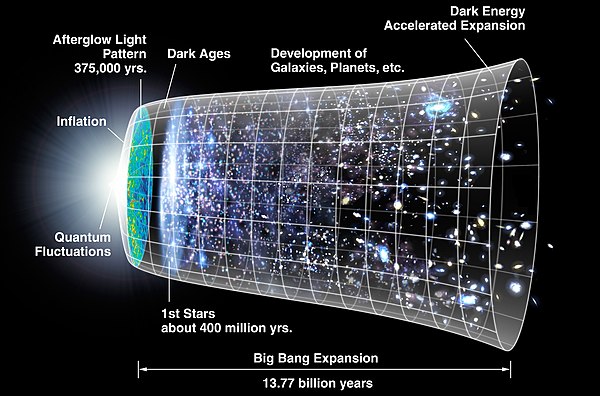
The ultimate fate of the universe is still unknown, because it depends critically on the curvature index k and the cosmological constant Λ. If the universe is sufficiently dense, k equals +1, meaning that its average curvature throughout is positive and the universe will eventually recollapse in a Big Crunch, possibly starting a new universe in a Big Bounce. Conversely, if the universe is insufficiently dense, k equals 0 or −1 and the universe will expand forever, cooling off and eventually becoming inhospitable for all life, as the stars die and all matter coalesces into black holes (the Big Freeze and the heat death of the universe). As noted above, recent data suggests that the expansion speed of the universe is not decreasing as originally expected, but increasing; if this continues indefinitely, the universe will eventually rip itself to shreds (the Big Rip). Experimentally, the universe has an overall density that is very close to the critical value between recollapse and eternal expansion; more careful astronomical observations are needed to resolve the question.
Big Bang model
The prevailing Big Bang model accounts for many of the experimental observations described above, such as the correlation of distance and redshift of galaxies, the universal ratio of hydrogen:helium atoms, and the ubiquitous, isotropic microwave radiation background. As noted above, the redshift arises from the metric expansion of space; as the space itself expands, the wavelength of a photon traveling through space likewise increases, decreasing its energy. The longer a photon has been traveling, the more expansion it has undergone; hence, older photons from more distant galaxies are the most red-shifted. Determining the correlation between distance and redshift is an important problem in experimental physical cosmology.
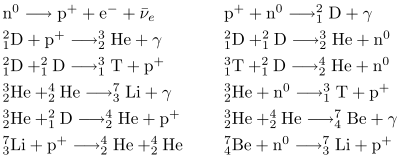
Other experimental observations can be explained by combining the overall expansion of space with nuclear and atomic physics. As the universe expands, the energy density of the electromagnetic radiation decreases more quickly than does that of matter, since the energy of a photon decreases with its wavelength. Thus, although the energy density of the universe is now dominated by matter, it was once dominated by radiation; poetically speaking, all was light. As the universe expanded, its energy density decreased and it became cooler; as it did so, the elementary particles of matter could associate stably into ever larger combinations. Thus, in the early part of the matter-dominated era, stable protons and neutrons formed, which then associated into atomic nuclei. At this stage, the matter in the universe was mainly a hot, dense plasma of negative electrons, neutral neutrinos and positive nuclei. Nuclear reactions among the nuclei led to the present abundances of the lighter nuclei, particularly hydrogen, deuterium, and helium. Eventually, the electrons and nuclei combined to form stable atoms, which are transparent to most wavelengths of radiation; at this point, the radiation decoupled from the matter, forming the ubiquitous, isotropic background of microwave radiation observed today.
Other observations are not answered definitively by known physics. According to the prevailing theory, a slight imbalance of matter over antimatter was present in the universe's creation, or developed very shortly thereafter, possibly due to the CP violation that has been observed by particle physicists. Although the matter and antimatter mostly annihilated one another, producing photons, a small residue of matter survived, giving the present matter-dominated universe. Several lines of evidence also suggest that a rapid cosmic inflation of the universe occurred very early in its history (roughly 10−35 seconds after its creation). Recent observations also suggest that the cosmological constant (Λ) is not zero and that the net mass-energy content of the universe is dominated by a dark energy and dark matter that have not been characterized scientifically. They differ in their gravitational effects. Dark matter gravitates as ordinary matter does, and thus slows the expansion of the universe; by contrast, dark energy serves to accelerate the universe's expansion.
Multiverse theory

Some speculative theories have proposed that this universe is but one of a set of disconnected universes, collectively denoted as the multiverse, challenging or enhancing more limited definitions of the universe.[17][73] Scientific multiverse theories are distinct from concepts such as alternate planes of consciousness and simulated reality, although the idea of a larger universe is not new; for example, Bishop Étienne Tempier of Paris ruled in 1277 that God could create as many universes as he saw fit, a question that was being hotly debated by the French theologians.[74]
Max Tegmark developed a four part classification scheme for the different types of multiverses that scientists have suggested in various problem domains. An example of such a theory is the chaotic inflation model of the early universe.[75] Another is the many-worlds interpretation of quantum mechanics. Parallel worlds are generated in a manner similar to quantum superposition and decoherence, with all states of the wave function being realized in separate worlds. Effectively, the multiverse evolves as a universal wavefunction. If the big bang that created our mutliverse created an ensemble of multiverses, the wave function of the ensemble would be entangled in this sense.
The least controversial category of multiverse in Tegmark's scheme is Level I, which describes distant space-time events "in our own universe". If space is infinite, or sufficiently large and uniform, identical instances of the history of Earth's entire Hubble volume occur every so often, simply by chance. Tegmark calculated our nearest so-called doppelgänger, is 1010115 meters away from us (a double exponential function larger than a googolplex).[76][77] In principle, it would be impossible to scientifically verify an identical Hubble volume. However, it does follow as a fairly straightforward consequence from otherwise unrelated scientific observations and theories. Tegmark suggests that statistical analysis exploiting the anthropic principle provides an opportunity to test multiverse theories in some cases. Generally, science would consider a multiverse theory that posits neither a common point of causation, nor the possibility of interaction between universes, to be an ideal speculation.
Shape of the universe
The shape or geometry of the universe includes both local geometry in the observable universe and global geometry, which we may or may not be able to measure. Shape can refer to curvature and topology. More formally, the subject in practice investigates which 3-manifold corresponds to the spatial section in comoving coordinates of the four-dimensional space-time of the universe. Cosmologists normally work with a given space-like slice of spacetime called the comoving coordinates. In terms of observation, the section of spacetime that can be observed is the backward light cone (points within the cosmic light horizon, given time to reach a given observer). If the observable universe is smaller than the entire universe (in some models it is many orders of magnitude smaller), one cannot determine the global structure by observation: one is limited to a small patch.
Among the Friedmann–Lemaître–Robertson–Walker (FLRW) models, the presently most popular shape of the Universe found to fit observational data according to cosmologists is the infinite flat model,[78] while other FLRW models include the Poincaré dodecahedral space[79][80] and the Picard horn.[81] The data fit by these FLRW models of space especially include the Wilkinson Microwave Anisotropy Probe (WMAP) maps of cosmic background radiation. NASA released the first WMAP cosmic background radiation data in February 2003. In 2009 the Planck observatory was launched to observe the microwave background at higher resolution than WMAP, possibly providing more information on the shape of the Universe. The data should be released in late 2012.
See also


- Cosmic latte
- Cosmology
- Dyson's eternal intelligence
- Esoteric cosmology
- False vacuum
- Final anthropic principle
- Fine-tuned Universe
- Hindu cycle of the universe
- Jain cosmology
- Kardashev scale
- The Mysterious Universe (book)
- Nucleocosmochronology
- Non-standard cosmology
- Observable universe
- Omega Point
- Omniverse
- Rare Earth hypothesis
- Vacuum genesis
- World view
- Zero-energy universe
Notes and references
- ^ Webster's New World College Dictionary. Wiley Publishing, Inc. 2010.
- ^ The American Heritage Dictionary of the English Language (4th ed.). Houghton Mifflin Harcourt Publishing Company. 2010.
- ^ Cambridge Advanced Learner's Dictionary.
- ^ http://www.astronomy.pomona.edu/Projects/moderncosmo/Sean%27s%20mutliverse.html
- ^ http://www.bbc.co.uk/news/science-environment-14372387
- ^ Suyu, S. H.; Marshall, P. J.; Auger, M. W.; Hilbert, S.; Blandford, R. D.; Koopmans, L. V. E.; Fassnacht, C. D.; Treu, T. (2010). "DISSECTING THE GRAVITATIONAL LENS B1608+656. II. PRECISION MEASUREMENTS OF THE HUBBLE CONSTANT, SPATIAL CURVATURE, AND THE DARK ENERGY EQUATION OF STATE". The Astrophysical Journal. 711: 201–221. Bibcode:2010ApJ...711..201S. doi:10.1088/0004-637X/711/1/201.
- ^ Lineweaver, Charles (2005). "Misconceptions about the Big Bang". Scientific American. Retrieved 2008-11-06.
{{cite web}}
: Unknown parameter|coauthors=
ignored (|author=
suggested) (help) - ^ The Compact Edition of the Oxford English Dictionary, volume II, Oxford: Oxford University Press, 1971, p.3518.
- ^ a b Lewis and Short, A Latin Dictionary, Oxford University Press, ISBN 0-19-864201-6, pp. 1933, 1977–1978.
- ^ Liddell and Scott, p. 1392.
- ^ Liddell and Scott, pp. 1345–1346.
- ^ Yonge, Charles Duke (1870). An English-Greek lexicon. New York: American Bok Company. p. 567.
- ^ Liddell and Scott, pp. 985, 1964.
- ^ Lewis and Short, pp. 1881–1882, 1175, 1189–1190.
- ^ OED, pp. 909, 569, 3821–3822, 1900.
- ^ Feynman RP, Hibbs AR (1965). Quantum Physics and Path Integrals. New York: McGraw–Hill. ISBN 0-07-020650-3.
Zinn Justin J (2004). Path Integrals in Quantum Mechanics. Oxford University Press. ISBN 0-19-856674-3. OCLC 212409192. - ^ a b Ellis, George F.R. (2004). "Multiverses and physical cosmology". Monthly Notices of the Royal Astronomical Society. 347 (3): 921–936. arXiv:astro-ph/0305292. Bibcode:2004MNRAS.347..921E. doi:10.1111/j.1365-2966.2004.07261.x.
{{cite journal}}
: Unknown parameter|coauthors=
ignored (|author=
suggested) (help)CS1 maint: unflagged free DOI (link) - ^ Lineweaver, Charles (2005). "Misconceptions about the Big Bang" (PDF). Scientific American. Retrieved 2007-03-05.
{{cite web}}
: Unknown parameter|coauthors=
ignored (|author=
suggested) (help) - ^ Rindler (1977), p.196.
- ^ Christian, Eric; Samar, Safi-Harb. "How large is the Milky Way?". Retrieved 2007-11-28.
- ^ I. Ribas, C. Jordi, F. Vilardell, E.L. Fitzpatrick, R.W. Hilditch, F. Edward (2005). "First Determination of the Distance and Fundamental Properties of an Eclipsing Binary in the Andromeda Galaxy". Astrophysical Journal. 635 (1): L37–L40. arXiv:astro-ph/0511045. Bibcode:2005ApJ...635L..37R. doi:10.1086/499161.
{{cite journal}}
: CS1 maint: multiple names: authors list (link)
McConnachie, A. W.; Irwin, M. J.; Ferguson, A. M. N.; Ibata, R. A.; Lewis, G. F.; Tanvir, N. (2005). "Distances and metallicities for 17 Local Group galaxies". Monthly Notices of the Royal Astronomical Society. 356 (4): 979–997. arXiv:astro-ph/0410489. Bibcode:2005MNRAS.356..979M. doi:10.1111/j.1365-2966.2004.08514.x.{{cite journal}}
: CS1 maint: multiple names: authors list (link) CS1 maint: unflagged free DOI (link) - ^ Mackie, Glen (February 1, 2002). "To see the Universe in a Grain of Taranaki Sand". Swinburne University. Retrieved 2006-12-20.
- ^ "Unveiling the Secret of a Virgo Dwarf Galaxy". ESO. 2000-05-03. Retrieved 2007-01-03.
- ^ "Hubble's Largest Galaxy Portrait Offers a New High-Definition View". NASA. 2006-02-28. Retrieved 2007-01-03.
- ^ Vergano, Dan (1 December 2010). "Universe holds billions more stars than previously thought". USA Today. Retrieved 14 December 2010.
- ^ N. Mandolesi, P. Calzolari, S. Cortiglioni, F. Delpino, G. Sironi (1986). "Large-scale homogeneity of the Universe measured by the microwave background". Letters to Nature. 319 (6056): 751–753. Bibcode:1986Natur.319..751M. doi:10.1038/319751a0.
{{cite journal}}
: CS1 maint: multiple names: authors list (link) - ^ Hinshaw, Gary (November 29, 2006). "New Three Year Results on the Oldest Light in the Universe". NASA WMAP. Retrieved 2006-08-10.
- ^ Hinshaw, Gary (December 15, 2005). "Tests of the Big Bang: The CMB". NASA WMAP. Retrieved 2007-01-09.
- ^ Rindler (1977), p. 202.
- ^ Hinshaw, Gary (February 10, 2006). "What is the Universe Made Of?". NASA WMAP. Retrieved 2007-01-04.
- ^ "Five-Year Wilkinson Microwave Anisotropy Probe (WMAP) Observations: Data Processing, Sky Maps, and Basic Results" (PDF). nasa.gov. Retrieved 2008-03-06.
- ^ Britt RR (2003-01-03). "Age of Universe Revised, Again". Space.com. Retrieved 2007-01-08.
- ^ Wright EL (2005). "Age of the Universe". UCLA. Retrieved 2007-01-08.
Krauss LM, Chaboyer B (3 January 2003). "Age Estimates of Globular Clusters in the Milky Way: Constraints on Cosmology". Science. 299 (5603): 65–69. Bibcode:2003Sci...299...65K. doi:10.1126/science.1075631. PMID 12511641. - ^ Wright, Edward L. (September 12, 2004). "Big Bang Nucleosynthesis". UCLA. Retrieved 2007-01-05.
M. Harwit, M. Spaans (2003). "Chemical Composition of the Early Universe". The Astrophysical Journal. 589 (1): 53–57. arXiv:astro-ph/0302259. Bibcode:2003ApJ...589...53H. doi:10.1086/374415.
C. Kobulnicky, E. D. Skillman (1997). "Chemical Composition of the Early Universe". Bulletin of the American Astronomical Society. 29: 1329. Bibcode:1997AAS...191.7603K. - ^ "Antimatter". Particle Physics and Astronomy Research Council. October 28, 2003. Retrieved 2006-08-10.
- ^ Landau and Lifshitz (1975), p.361.
- ^ WMAP Mission: Results – Age of the Universe
- ^ Luminet, Jean-Pierre (1999). "Topology of the Universe: Theory and Observations". Proceedings of Cosmology School held at Cargese, Corsica, August 1998. arXiv:astro-ph/9901364.
{{cite conference}}
: Unknown parameter|booktitle=
ignored (|book-title=
suggested) (help); Unknown parameter|coauthors=
ignored (|author=
suggested) (help)
Luminet, Jean-Pierre (2003). "Dodecahedral space topology as an explanation for weak wide-angle temperature correlations in the cosmic microwave background". Nature. 425 (6958): 593–595. arXiv:astro-ph/0310253. Bibcode:2003Natur.425..593L. doi:10.1038/nature01944. PMID 14534579.{{cite journal}}
: Unknown parameter|coauthors=
ignored (|author=
suggested) (help) - ^ Strobel, Nick (May 23, 2001). "The Composition of Stars". Astronomy Notes. Retrieved 2007-01-04.
"Have physical constants changed with time?". Astrophysics (Astronomy Frequently Asked Questions). Retrieved 2007-01-04. - ^ Stephen Hawking (1988). A Brief History of Time. Bantam Books. p. 125. ISBN 0-553-05340-X.
- ^ Martin Rees (1999). Just Six Numbers. HarperCollins Publishers. ISBN 0-465-03672-4.
- ^ Adams, F.C. (2008). "Stars in other universes: stellar structure with different fundamental constants". Journal of Cosmology and Astroparticle Physics. 2008 (08): 010. arXiv:0807.3697. Bibcode:2008JCAP...08..010A. doi:10.1088/1475-7516/2008/08/010.
- ^ Harnik, R. (2006). "A universe without weak interactions". Physical Review D. 74 (3): 035006. arXiv:hep-ph/0604027. Bibcode:2006PhRvD..74c5006H. doi:10.1103/PhysRevD.74.035006.
{{cite journal}}
: Unknown parameter|coauthors=
ignored (|author=
suggested) (help) - ^ Will Durant, Our Oriental Heritage:
"Two systems of Hindu thought propound physical theories suggestively similar to those of Greece. Kanada, founder of the Vaisheshika philosophy, held that the world was composed of atoms as many in kind as the various elements. The Jains more nearly approximated to Democritus by teaching that all atoms were of the same kind, producing different effects by diverse modes of combinations. Kanada believed light and heat to be varieties of the same substance; Udayana taught that all heat comes from the sun; and Vachaspati, like Newton, interpreted light as composed of minute particles emitted by substances and striking the eye."
- ^ F. Th. Stcherbatsky (1930, 1962), Buddhist Logic, Volume 1, p.19, Dover, New York:
"The Buddhists denied the existence of substantial matter altogether. Movement consists for them of moments, it is a staccato movement, momentary flashes of a stream of energy... "Everything is evanescent“,... says the Buddhist, because there is no stuff... Both systems [Sānkhya, and later Indian Buddhism] share in common a tendency to push the analysis of Existence up to its minutest, last elements which are imagined as absolute qualities, or things possessing only one unique quality. They are called “qualities” (guna-dharma) in both systems in the sense of absolute qualities, a kind of atomic, or intra-atomic, energies of which the empirical things are composed. Both systems, therefore, agree in denying the objective reality of the categories of Substance and Quality,... and of the relation of Inference uniting them. There is in Sānkhya philosophy no separate existence of qualities. What we call quality is but a particular manifestation of a subtle entity. To every new unit of quality corresponds a subtle quantum of matter which is called guna “quality”, but represents a subtle substantive entity. The same applies to early Buddhism where all qualities are substantive... or, more precisely, dynamic entities, although they are also called dharmas ('qualities')."
- ^ a b c Craig, William Lane (June 1979). "Whitrow and Popper on the Impossibility of an Infinite Past". The British Journal for the Philosophy of Science. 30 (2): 165–170 [165–6]. doi:10.1093/bjps/30.2.165.
- ^ Boyer, C. A History of Mathematics. Wiley, p. 54.
- ^ Otto E. Neugebauer (1945). "The History of Ancient Astronomy Problems and Methods", Journal of Near Eastern Studies 4 (1), p. 1–38.
"the Chaldaean Seleucus from Seleucia"
- ^ George Sarton (1955). "Chaldaean Astronomy of the Last Three Centuries B. C.", Journal of the American Oriental Society 75 (3), pp. 166–173 [169]:
"the heliocentrical astronomy invented by Aristarchos of Samos and still defended a century later by Seleucos the Babylonian"
- ^ William P. D. Wightman (1951, 1953), The Growth of Scientific Ideas, Yale University Press p.38, where Wightman calls him Seleukos the Chaldean.
- ^ Lucio Russo, Flussi e riflussi, Feltrinelli, Milano, 2003, ISBN 88-07-10349-4.
- ^ Bartel, p. 527
- ^ Bartel, pp. 527–9
- ^ Bartel, pp. 529–34
- ^ Bartel, pp. 534–7
- ^ Nasr, Seyyed H. (1st edition in 1964, 2nd edition in 1993). An Introduction to Islamic Cosmological Doctrines (2nd ed.). 1st edition by Harvard University Press, 2nd edition by State University of New York Press. pp. 135–6. ISBN 0791415155.
{{cite book}}
: Check date values in:|date=
(help) - ^ Misner, Thorne and Wheeler (1973), p. 754.
- ^ a b Misner, Thorne, and Wheeler (1973), p. 755–756.
- ^ Misner, Thorne, and Wheeler (1973), p. 756.
- ^ de Cheseaux JPL (1744). Traité de la Comète. Lausanne. pp. 223ff.. Reprinted as Appendix II in Dickson FP (1969). The Bowl of Night: The Physical Universe and Scientific Thought. Cambridge, MA: M.I.T. Press. ISBN 978-0262540032.
- ^ Olbers HWM (1826). "Unknown title". Bode's Jahrbuch. 111.. Reprinted as Appendix I in Dickson FP (1969). The Bowl of Night: The Physical Universe and Scientific Thought. Cambridge, MA: M.I.T. Press. ISBN 978-0262540032.
- ^ Jeans, J. H. (1902). "The Stability of a Spherical Nebula" (PDF). Philosophical Transactions Royal Society of London, Series A. 199 (312–320): 1–53. Bibcode:1902RSPTA.199....1J. doi:10.1098/rsta.1902.0012. JSTOR 90845. Retrieved 17 March 2011.
- ^ Rindler, p. 196; Misner, Thorne, and Wheeler (1973), p. 757.
- ^ Misner, Thorne and Wheeler, p.756.
- ^ a b Einstein, A (1917). "Kosmologische Betrachtungen zur allgemeinen Relativitätstheorie". Preussische Akademie der Wissenschaften, Sitzungsberichte. 1917. (part 1): 142–152.
- ^ Rindler (1977), pp. 226–229.
- ^ Landau and Lifshitz (1975), pp. 358–359.
- ^ Einstein, A (1931). "Zum kosmologischen Problem der allgemeinen Relativitätstheorie". Sitzungsberichte der Preussischen Akademie der Wissenschaften, Physikalisch-mathematische Klasse. 1931: 235–237.
Einstein A., de Sitter W. (1932). "On the relation between the expansion and the mean density of the universe". Proceedings of the National Academy of Sciences. 18 (3): 213–214. Bibcode:1932PNAS...18..213E. doi:10.1073/pnas.18.3.213. PMC 1076193. PMID 16587663. - ^ Hubble Telescope news release
- ^ "Mysterious force's long presence". BBC News. 2006-11-16.
- ^ Zel'dovich YB (1967). "Cosmological constant and elementary particles". Zh. Eksp. & Teor. Fiz. Pis'ma. 6: 883–884. English translation in Sov. Phys. — JTEP Lett., 6, pp. 316–317 (1967).
- ^ Friedmann A. (1922). "Über die Krümmung des Raumes". Zeitschrift für Physik. 10 (1): 377–386. Bibcode:1922ZPhy...10..377F. doi:10.1007/BF01332580.
- ^ Munitz MK (1959). "One Universe or Many?". Journal of the History of Ideas. 12 (2): 231–255. doi:10.2307/2707516. JSTOR 2707516.
- ^ Misner, Thorne and Wheeler (1973), p.753.
- ^ Linde A. (1986). "Eternal chaotic inflation". Mod. Phys. Lett. A1 (2): 81–85. Bibcode:1986MPLA....1...81L. doi:10.1142/S0217732386000129.
Linde A. (1986). "Eternally existing self-reproducing chaotic inflationary universe" (PDF). Phys. Lett. B175 (4): 395–400. Bibcode:1986PhLB..175..395L. doi:10.1016/0370-2693(86)90611-8. Retrieved 17 March 2011. - ^ "Parallel universes. Not just a staple of science fiction, other universes are a direct implication of cosmological observations.", Tegmark M., Sci Am. 2003 May;288(5):40-51.
- ^ Max Tegmark (2003). "Parallel Universes". In "Science and Ultimate Reality: from Quantum to Cosmos", honoring John Wheeler's 90th birthday. J. D. Barrow, P.C.W. Davies, & C.L. Harper eds. Cambridge University Press (2003). arXiv:astro-ph/0302131. Bibcode:2003astro.ph..2131T.
- ^ Shape of the Universe, WMAP website at NASA.
- ^ Luminet, Jean-Pierre (2003-10-09). "Dodecahedral space topology as an explanation for weak wide-angle temperature correlations in the cosmic microwave background". Nature. 425 (6958): 593–5. arXiv:astro-ph/0310253. Bibcode:2003Natur.425..593L. doi:10.1038/nature01944. PMID 14534579.
{{cite journal}}
: Unknown parameter|coauthors=
ignored (|author=
suggested) (help) - ^ Roukema, Boudewijn (2008). "A test of the Poincare dodecahedral space topology hypothesis with the WMAP CMB data". Astronomy and Astrophysics. 482 (3): 747. arXiv:0801.0006. Bibcode:2008A&A...482..747L. doi:10.1051/0004-6361:20078777.
{{cite journal}}
: Unknown parameter|coauthors=
ignored (|author=
suggested) (help) - ^ Aurich, Ralf (2004). "Hyperbolic Universes with a Horned Topology and the CMB Anisotropy". Classical and Quantum Gravity. 21 (21): 4901–4926. arXiv:astro-ph/0403597. Bibcode:2004CQGra..21.4901A. doi:10.1088/0264-9381/21/21/010.
{{cite journal}}
: Unknown parameter|coauthors=
ignored (|author=
suggested) (help)
Bibliography
- Bartel (1987). "The Heliocentric System in Greek, Persian and Hindu Astronomy". Annals of the New York Academy of Sciences. 500 (1): 525–545. Bibcode:1987NYASA.500..525V. doi:10.1111/j.1749-6632.1987.tb37224.x.
- Landau, Lev, Lifshitz, E.M. (1975). The Classical Theory of Fields (Course of Theoretical Physics, Vol. 2) (revised 4th English ed.). New York: Pergamon Press. pp. 358–397. ISBN 9780080181769.
{{cite book}}
: CS1 maint: multiple names: authors list (link) - Liddell, H. G. and Scott, R. A Greek-English Lexicon, Oxford University Press, ISBN 0-19-864214-8
- Misner, C.W., Thorne, Kip, Wheeler, J.A. (1973). Gravitation. San Francisco: W. H. Freeman. pp. 703–816. ISBN 978-0-7167-0344-0.
{{cite book}}
: CS1 maint: multiple names: authors list (link) - Rindler, W. (1977). Essential Relativity: Special, General, and Cosmological. New York: Springer Verlag. pp. 193–244. ISBN 0-387-10090-3.
Further reading
- Weinberg, S. (1993). The First Three Minutes: A Modern View of the Origin of the Universe (2nd updated ed.). New York: Basic Books. ISBN 978-0465024377. OCLC 28746057. For lay readers.
- Nussbaumer, Harry; Bieri, Lydia; Sandage, Allan (2009). Discovering the Expanding Universe. Cambridge University Press. ISBN 978-0-521-51484-2.
External links
- Is there a hole in the universe? at HowStuffWorks
- Age of the Universe at Space.Com
- Stephen Hawking's Universe – Why is the universe the way it is?
- Cosmology FAQ
- Cosmos – An "illustrated dimensional journey from microcosmos to macrocosmos"
- Illustration comparing the sizes of the planets, the sun, and other stars
- Logarithmic Maps of the Universe
- My So-Called Universe – Arguments for and against an infinite and parallel universes
- Parallel Universes by Max Tegmark
- The Dark Side and the Bright Side of the Universe Princeton University, Shirley Ho
- Richard Powell: An Atlas of the Universe – Images at various scales, with explanations
- Multiple Big Bangs
- Universe – Space Information Centre
- Exploring the Universe at Nasa.gov