Optical fiber: Difference between revisions
m clean up using AWB |
No edit summary Tag: nowiki added |
||
Line 1: | Line 1: | ||
{{About||methods of applying a 2D image onto a 3D surface|pad printing|methods of copying 2D parallax stereograms that seem 3D to the eye|lenticular printing|and|holography}} |
|||
[[File:fibreoptic.jpg|thumb|right|A bundle of optical fibers]] |
|||
[[File:MakerBot ThingOMatic Bre Pettis.jpg|thumb|A [[MakerBot]] 3D printer]] |
|||
[[File:Stealth Fiber Crew installing fiber cable underneath the streets of Manhattan.jpg|thumb|Fiber crew installing a 432-count fiber cable underneath the streets of Midtown Manhattan, New York City]] |
|||
{{History of printing}} |
|||
[[File:Fiber optic illuminated.jpg|thumb|A [[TOSLINK]] fiber optic audio cable with red light being shone in one end transmits the light to the other end]] |
|||
[[File:Optical-fibre-junction-box.jpg|thumb|right|A [[19-inch rack|wall-mount cabinet]] containing optical fiber interconnects. The yellow cables are [[single-mode optical fiber|single mode fibers]]; the orange and aqua cables are [[multi-mode optical fiber|multi-mode fibers]]: 50/125 µm OM2 and 50/125 µm OM3 fibers respectively.]] |
|||
'''3D printing''', also known as '''additive manufacturing (AM)''', refers to various processes used to synthesize a [[three-dimensional space|three-dimensional]] object.<ref name="engineer" /> In 3D printing, successive layers of material are formed under computer control to create an object.<ref name="Auto3D-1" /> These objects can be of almost any shape or geometry, and are produced from a [[3D modeling|3D model]] or other electronic data source. A 3D [[Printer (computing)|printer]] is a type of [[industrial robot]]. |
|||
An '''optical fiber''' ([[American and British English spelling differences#-re, -er|or]] '''optical fibre''') is a flexible, transparent fiber made by [[Drawing (manufacturing)|drawing]] glass ([[silica]]) or plastic to a diameter slightly thicker than that of a [[Hair|human hair]].<ref>{{cite web|title=Optical Fiber|url=http://www.thefoa.org/tech/ref/basic/fiber.html|website=http://www.thefoa.org/|publisher=The Fiber Optic Association, Inc.|accessdate=17 April 2015}}</ref> Optical fibers are used most often as a means to transmit light between the two ends of the fiber and find wide usage in [[fiber-optic communication]]s, where they permit transmission over longer distances and at higher [[bandwidth (computing)|bandwidth]]s (data rates) than wire cables. Fibers are used instead of [[metal]] wires because signals travel along them with lesser amounts of [[Attenuation|loss]]; in addition, fibers are also immune to [[electromagnetic interference]], a problem from which metal wires suffer excessively.<ref>{{cite book|last1=Senior|first1=John M.|last2=Jamro|first2=M. Yousif|title=Optical fiber communications: principles and practice|date=2009|publisher=Pearson Education|url=http://cds.cern.ch/record/1493478|accessdate=17 April 2015}}</ref><ref>''Optical fiber communications: principles and practice'' pp 7-9</ref> Fibers are also used for [[illumination (lighting)|illumination]], and are wrapped in bundles so that they may be used to carry images, thus allowing viewing in confined spaces, as in the case of a [[fiberscope]].<ref>{{cite web|title=Birth of Fiberscopes|url=http://www.olympus-global.com/en/corc/history/story/endo/fiber/|website=http://www.olympus-global.com/|publisher=Olympus Corporation|accessdate=17 April 2015}}</ref> Specially designed fibers are also used for a variety of other applications, some of them being [[fiber optic sensor]]s and [[fiber laser]]s.<ref>{{cite journal|last1=Lee|first1=Byoungho|title=Review of the present status of optical fiber sensors.|journal=Optical Fiber Technology|date=2003|volume=9|issue=2|pages=57–79|url=http://wr.lib.tsinghua.edu.cn/sites/default/files/1066744871461.pdf|accessdate=17 April 2015|doi=10.1016/s1068-5200(02)00527-8}}</ref> |
|||
[[Futurologist]]s such as [[Jeremy Rifkin]] <ref>http://www.thethirdindustrialrevolution.com/</ref> believe that 3D printing signals the beginning of a [[third industrial revolution]],<ref>http://www.economist.com/node/21552901</ref> succeeding the [[production line]] assembly that dominated manufacturing starting in the late 19th century. Using the power of the [[Internet]], it may eventually be possible to send a [[blueprint]] of any product to any place in the world to be replicated by a 3D printer with "elemental inks" capable of being combined into any material substance of any desired form. |
|||
Optical fibers typically include a [[transparency and translucency|transparent]] [[core (optical fiber)|core]] surrounded by a transparent [[cladding (fiber optics)|cladding]] material with a lower [[index of refraction]]. Light is kept in the core by the phenomenon of [[total internal reflection]] which causes the fiber to act as a [[waveguide]].<ref>''Optical fiber communications: principles and practice'' pp 12-14</ref> Fibers that support many propagation paths or [[transverse mode]]s are called [[multi-mode fiber]]s (MMF), while those that support a single mode are called [[single-mode fiber]]s (SMF). Multi-mode fibers generally have a wider core diameter and are used for short-distance communication links and for applications where high power must be transmitted.{{citation needed|date=April 2015}} Single-mode fibers are used for most communication links longer than {{convert|1000|sp=us|m|ft}}.{{citation needed|date=April 2015}} |
|||
''3D printing'' in the term's original [[word sense|sense]] refers to processes that sequentially deposit material onto a powder bed with inkjet printer heads. More recently, the meaning of the term has expanded to encompass a wider variety of techniques such as [[extrusion]] and [[sintering]]-based processes. [[Technical standard]]s generally use the term ''additive manufacturing'' for this broader sense. |
|||
An important aspect of a fiber optic communication is that of extension of the fiber optic cables such that the losses brought about by joining two different cables is kept to a minimum.<ref>''Optical fiber communications: principles and practice'' p 218</ref> Joining lengths of optical fiber often proves to be more complex than joining electrical wire or cable and involves careful [[cleave (fiber)|cleaving]] of the fibers, perfect alignment of the fiber cores, and the splicing of these aligned fiber cores. For applications that demand a permanent connection a [[mechanical splice]] which holds the ends of the fibers together mechanically could be used or a [[fusion splicing|fusion splice]] that uses heat to fuse the ends of the fibers together could be used. Temporary or semi-permanent connections are made by means of specialized [[optical fiber connector]]s.<ref>''Optical fiber communications: principles and practice'' pp 234-235</ref> |
|||
== History == |
|||
The field of applied science and engineering concerned with the design and application of optical fibers is known as '''fiber optics'''. |
|||
{{toclimit|3}} |
|||
=== Terminology and methods === |
|||
==History== |
|||
Early Additive Manufacturing equipment and materials were developed in the 1980s.<ref name="3D opp" /> In 1981, Hideo Kodama of Nagoya Municipal Industrial Research Institute invented two AM fabricating methods of a three-dimensional plastic model with photo-hardening polymer, where the UV exposure area is controlled by a mask pattern or the scanning fiber transmitter.<ref>Hideo Kodama, "A Scheme for Three-Dimensional Display by Automatic Fabrication of Three-Dimensional Model," IEICE TRANSACTIONS on Electronics (Japanese Edition), vol.J64-C, No.4, pp.237–241, April 1981</ref><ref>Hideo Kodama, "Automatic method for fabricating a three-dimensional plastic model with photo-hardening polymer," ''Review of Scientific Instruments'', Vol. 52, No. 11, pp. 1770–1773, November 1981</ref> Then in 1984, [[Chuck Hull]] of [[3D Systems]] Corporation<ref name="AutoSQ-1" /> developed a prototype system based on a process known as [[stereolithography]], in which layers are added by curing [[photopolymers]] with [[ultraviolet light]] [[lasers]]. Hull defined the process as a "system for generating three-dimensional objects by creating a cross-sectional pattern of the object to be formed,"<ref name="AutoSQ-4" /><ref name="AutoSQ-5" /> but this had been already invented by Kodama. Hull's contribution is the design of the [[STL (file format)|STL (STereoLithography) file format]] widely accepted by 3D printing software as well as the digital slicing and infill strategies common to many processes today. The term ''3D printing'' originally referred to a process employing standard and custom [[inkjet]] print heads. The technology used by most 3D printers to date—especially hobbyist and consumer-oriented models—is [[fused deposition modeling]], a special application of plastic [[extrusion]]. |
|||
[[File:DanielColladon's Lightfountain or Lightpipe,LaNature(magazine),1884.JPG|thumb|[[Jean-Daniel Colladon|Daniel Colladon]] first described this “light fountain” or “light pipe” in an 1842 article titled ''On the reflections of a ray of light inside a parabolic liquid stream''. This particular illustration comes from a later article by Colladon, in 1884.]] |
|||
AM processes for metal sintering or melting (such as [[selective laser sintering]], [[direct metal laser sintering]], and [[selective laser melting]]) usually went by their own individual names in the 1980s and 1990s. At the time, nearly all metal working was produced by [[casting]], [[metal fabrication|fabrication]], [[stamping (metalworking)|stamping]], and [[machining]]; although plenty of [[automation]] was applied to those technologies (such as by [[robot welding]] and [[numerical control|CNC]]), the idea of a tool or head moving through a 3D work envelope transforming a mass of raw material into a desired shape layer by layer was associated by most people only with processes that removed metal (rather than adding it), such as CNC [[milling (machining)|milling]], CNC [[electrical discharge machining|EDM]], and many others. But AM-type sintering was beginning to challenge that assumption. By the mid 1990s, new techniques for material deposition were developed at [[Stanford]] and [[Carnegie Mellon University]], including microcasting<ref>{{cite journal |title=Shape Deposition Manufacturing With Microcasting: Processing, Thermal and Mechanical Issues |journal=Journal of Manufacturing Science and Engineering |date=1998 |last=Amon |first=C. H. |last2=Beuth |first2=J. L. |last3=Weiss |first3=L. E. |last4=Merz |first4=R. |last5=Prinz |first5=F. B. |volume=120 |issue=3 |url=http://repository.cmu.edu/cgi/viewcontent.cgi?article=1219&context=ece |format=PDF |accessdate=2014-12-20}}</ref> and sprayed materials.<ref>{{cite journal |title=Manufacturing Mechatronics Using Thermal Spray Shape Deposition |journal=Proceedings of the 1992 Solid Freeform Fabrication Symposium |date=1992 |last=Beck |first=J.E. |last2=Fritz |first2=B. |last3=Siewiorek |first3=Daniel |last4=Weiss |first4=Lee |url=http://utwired.engr.utexas.edu/lff/symposium/proceedingsarchive/pubs/manuscripts/1992/1992-31-beck.pdf |format=PDF |accessdate=2014-12-20}}</ref> Sacrificial and support materials had also become more common, enabling new object geometries.<ref>{{cite conference |last=Prinz |first=F. B. |last2=Merz |first2=R. |last3=Weiss |first3=Lee |title=Building Parts You Could Not Build Before |conference=Proceedings of the 8th International Conference on Production Engineering |editor-last=Ikawa |editor-first=N. |publisher=Chapman & Hall |place=2-6 Boundary Row, London SE1 8HN, UK |date=1997 |pages=40–44}}</ref> |
|||
Guiding of light by refraction, the principle that makes fiber optics possible, was first demonstrated by [[Jean-Daniel Colladon|Daniel Colladon]] and [[Jacques Babinet]] in [[Paris]] in the early 1840s. [[John Tyndall]] included a demonstration of it in his public lectures in [[London]], 12 years later.<ref name=regis>{{cite book |
|||
|last =Bates |
|||
|first =Regis J |
|||
|title =Optical Switching and Networking Handbook |
|||
|publisher =McGraw-Hill |
|||
|year= 2001 |
|||
|location =New York |
|||
|isbn=0-07-137356-X |
|||
|page =10}}</ref> Tyndall also wrote about the property of [[total internal reflection]] in an introductory book about the nature of light in 1870: {{quote|When the light passes from air into water, the refracted ray is bent ''towards'' the [[perpendicular]]... When the ray passes from water to air it is bent ''from'' the perpendicular... If the angle which the ray in water encloses with the perpendicular to the surface be greater than 48 degrees, the ray will not quit the water at all: it will be ''totally reflected'' at the surface.... The angle which marks the limit where total reflection begins is called the limiting angle of the medium. For water this angle is 48°27′, for flint glass it is 38°41′, while for diamond it is 23°42′.<ref>{{cite book |
|||
|first=John |last=Tyndall |
|||
|year=1870 |title=Notes about Light |
|||
|chapter=Total Reflexion |
|||
|url=http://www.archive.org/details/notesofcourseofn00tyndrich}}</ref><ref>{{cite web |
|||
|first=John |last=Tyndall |
|||
|year=1873 |
|||
|title=Six Lectures on Light |
|||
|url=http://www.archive.org/details/sixlecturesonlig00tynduoft}}</ref>}} Unpigmented human hairs have also been shown to act as an optical fiber.<ref>{{cite journal|author=Wells, J.|issue=6210|pmid=2918918|doi=10.1038/338023b0|title=Hair light guide|journal= Nature|volume= 338|page= 23|year=1989|bibcode = 1989Natur.338...23W }}</ref> |
|||
The [[umbrella term]] ''additive manufacturing'' gained wider currency in the [[2000s (decade)|decade of the 2000s]].<ref name="Ngram_additive_manufacturing" /> As the various additive processes matured, it became clear that soon metal removal would no longer be the only metalworking process done under that type of control (a tool or head moving through a 3D work envelope transforming a mass of raw material into a desired shape layer by layer). It was during this decade that the term ''subtractive manufacturing'' appeared as a [[retronym]] for the large family of machining processes with metal removal as their common theme. However, at the time, the term ''3D printing'' still referred only to the polymer technologies in most minds, and the term ''AM'' was likelier to be used in metalworking contexts than among polymer/inkjet/stereolithography enthusiasts. The term ''subtractive'' has not replaced the term ''machining'', instead [[wikt:complement#Verb|complementing]] it when a term that covers any removal method is needed. |
|||
Practical applications, such as close internal illumination during dentistry, appeared early in the twentieth century. Image transmission through tubes was demonstrated independently by the radio experimenter [[Clarence Hansell]] and the television pioneer [[John Logie Baird]] in the 1920s. The principle was first used for internal medical examinations by [[Heinrich Lamm]] in the following decade. Modern optical fibers, where the glass fiber is coated with a transparent cladding to offer a more suitable [[refractive index]], appeared later in the decade.<ref name=regis/> Development then focused on fiber bundles for image transmission. [[Harold Hopkins (physicist)|Harold Hopkins]] and [[Narinder Singh Kapany]] at [[Imperial College]] in London achieved low-loss light transmission through a 75 cm long bundle which combined several thousand fibers. Their article titled "A flexible fibrescope, using static scanning" was published in the journal ''Nature'' in 1954.<ref>{{cite journal|author=Hopkins, H. H. and Kapany, N. S. |journal=Nature|doi=10.1038/173039b0 |volume=173|pages= 39–41 |year=1954|title=A flexible fibrescope, using static scanning|issue=4392|bibcode = 1954Natur.173...39H }}</ref><ref>[http://web.archive.org/web/20110629061117/http://nobelprize.org/nobel_prizes/physics/laureates/2009/sciback_phy_09.pdf Two Revolutionary Optical Technologies]. Scientific Background on the Nobel Prize in Physics 2009. Nobelprize.org. 6 October 2009</ref> The first fiber optic semi-flexible [[gastroscope]] was patented by [[Basil Hirschowitz]], C. Wilbur Peters, and Lawrence E. Curtiss, researchers at the [[University of Michigan]], in 1956. In the process of developing the gastroscope, Curtiss produced the first glass-clad fibers; previous optical fibers had relied on air or impractical oils and waxes as the low-index cladding material. |
|||
By the early 2010s, the terms ''3D printing'' and ''additive manufacturing'' developed [[word sense|senses]] in which they were [[synonym]]ous umbrella terms for all AM technologies. Although this was a departure from their earlier technically narrower senses, it reflects the simple fact that the technologies all share the common theme of sequential-layer material addition/joining throughout a 3D work envelope under automated control. (Other terms that have appeared, which are usually used as AM synonyms (although sometimes as [[hyponymy and hypernymy|hypernyms]]), have been ''desktop manufacturing'', ''rapid manufacturing'' [as the logical production-level successor to ''[[rapid prototyping]]''], and ''on-demand manufacturing'' [which echoes ''[[print on demand|on-demand printing]]'' in the 2D sense of ''printing''].) The 2010s were the first decade in which metal parts such as engine brackets<ref name="GrabCAD_GE_bracket" /> and large nuts<ref name="AutoSQ-6" /> would be grown (either before or instead of machining) in [[job production]] rather than [[wikt:obligate#Adjective|obligately]] being machined from [[bar stock]] or plate. |
|||
A variety of other image transmission applications soon followed. |
|||
As technology matured, several authors had begun to speculate that 3D printing could aid in [[sustainable development]] in the developing world.<ref name="Auto3D-27" /><ref name="AutoSQ-25" /><ref>{{cite journal|title=3D Printing: Developing Countries Perspectives|url=http://arxiv.org/abs/1410.5349|journal=International Journal of Computer Applications|date=2014-10-18|issn=09758887|pages=30–34|volume=104|issue=11|doi=10.5120/18249-9329|first=Fredrick R.|last=Ishengoma|first2=Adam B.|last2=Mtaho|arxiv=1410.5349}}</ref> |
|||
In 1880 [[Alexander Graham Bell]] and [[Charles Sumner Tainter|Sumner Tainter]] invented the [[Photophone]] at the [[Volta Laboratory and Bureau|Volta Laboratory]] in Washington, D.C., to transmit voice signals over an optical beam.<ref name="SDU">Jones, Newell. [http://web.archive.org/web/20060904235846/http://history.sandiego.edu/gen/recording/ar304.html First 'Radio' Built by San Diego Resident Partner of Inventor of Telephone: Keeps Notebook of Experiences With Bell], San Diego Evening Tribune, July 31, 1937.</ref> It was an advanced form of telecommunications, but subject to atmospheric interferences and impractical until the secure transport of light that would be offered by fiber-optical systems. In the late 19th and early 20th centuries, light was guided through bent glass rods to illuminate body cavities.<ref>[http://inventors.about.com/library/weekly/aa980407.htm The Birth of Fiber Optics]. inventors.about.com</ref> [[Jun-ichi Nishizawa]], a Japanese scientist at [[Tohoku University]], also proposed the use of optical fibers for communications in 1963, as stated in his book published in 2004 in [[India]].<ref name=Bhat>{{cite book |
|||
|editor=Bhat, K. N. and DasGupta, Amitava |
|||
|title = Physics of semiconductor devices |
|||
|publisher =Narosa Publishing House |
|||
|year= 2004 |
|||
|location =New Delhi, India |
|||
|chapter=Terahertz wave generation and light amplification using Raman effect |
|||
|author=Nishizawa, Jun-ichi and Suto, Ken |
|||
|url =http://books.google.com/?id=2NTpSnfhResC&pg=PA27 |
|||
|isbn =81-7319-567-6 |
|||
|page =27}}</ref> Nishizawa invented other technologies that contributed to the development of optical fiber communications, such as the graded-index optical fiber as a channel for transmitting light from semiconductor lasers.<ref name="Sendai New">{{cite web |
|||
|work=Sendai New |
|||
|title=Optical Fiber |
|||
|url=http://web.archive.org/web/20090929124200/http://www.city.sendai.jp/soumu/kouhou/s-new-e6/page01.html |
|||
|accessdate=April 5, 2009}}</ref><ref name=IEEE>{{cite web |
|||
|work=Institute of Electrical and Electronics Engineers |
|||
|title=New Medal Honors Japanese Microelectrics Industry Leader |
|||
|url=http://www.ieee.org/portal/site/tionline/menuitem.130a3558587d56e8fb2275875bac26c8/index.jsp?&pName=institute_level1_article&TheCat=1003&article=tionline/legacy/inst2003/jun03/6w.nishizawa.xml&}}</ref> |
|||
The first working fiber-optical data transmission system was demonstrated by German physicist [[Manfred Börner]] at [[Telefunken]] Research Labs in Ulm in 1965, which was followed by the first patent application for this technology in 1966.<ref>{{cite patent | country = DE | number = 1254513 | status = patent | title = Mehrstufiges Übertragungssystem für Pulscodemodulation dargestellte Nachrichten. | gdate = 1967-11-16 | inventor = Börner, Manfred | assign1 = Telefunken Patentverwertungsgesellschaft m.b.H.}}</ref><ref>{{cite patent | country = US | number = 3845293 | status = patent | title = Electro-optical transmission system utilizing lasers| inventor = Börner, Manfred }}</ref> [[Charles K. Kao]] and [[George A. Hockham]] of the British company [[Standard Telephones and Cables]] (STC) were the first to promote the idea that the [[attenuation]] in optical fibers could be reduced below 20 [[decibel]]s per kilometer (dB/km), making fibers a practical communication medium.<ref name=hecht1999>{{cite book |
|||
|last= Hecht |
|||
|first= Jeff |
|||
|title= City of Light, The Story of Fiber Optics |
|||
|publisher= [[Oxford University Press]] |
|||
|location= New York |
|||
|year= 1999 |
|||
|url=http://books.google.com/?id=4oMu7RbGpqUC&pg=PA114 |
|||
|isbn= 0-19-510818-3 |
|||
|page=114}}</ref> They proposed that the attenuation in fibers available at the time was caused by impurities that could be removed, rather than by fundamental physical effects such as scattering. They correctly and systematically theorized the light-loss properties for optical fiber, and pointed out the right material to use for such fibers — silica glass with high purity. This discovery earned Kao the [[Nobel Prize in Physics]] in 2009.<ref>{{cite web |url=http://nobelprize.org/nobel_prizes/physics/laureates/2009/press.html|title=Press Release — Nobel Prize in Physics 2009|publisher=The Nobel Foundation|accessdate=2009-10-07}}</ref> |
|||
== General principles == |
|||
NASA used fiber optics in the television cameras that were sent to the moon. At the time, the use in the cameras was classified ''confidential'', and only those with sufficient security clearance or those accompanied by someone with the right security clearance were permitted to handle the cameras.<ref>[http://history.nasa.gov/alsj/MSC-SESD-28-105.pdf Lunar Television Camera. Pre-installation Acceptance Test Plan]. NASA. 12 March 1968</ref> |
|||
=== Modeling === |
|||
The crucial attenuation limit of 20 dB/km was first achieved in 1970, by researchers [[Robert D. Maurer]], [[Donald Keck]], [[Peter C. Schultz]], and Frank Zimar working for American glass maker Corning Glass Works, now [[Corning Incorporated]]. They demonstrated a fiber with 17 dB/km attenuation by [[doping (semiconductor)|doping]] [[silica glass]] with [[titanium]]. A few years later they produced a fiber with only 4 dB/km attenuation using [[germanium dioxide]] as the core dopant. Such low attenuation ushered in the era of optical fiber telecommunication. In 1981, [[General Electric]] produced fused [[quartz]] [[ingots]] that could be drawn into strands 25 miles (40 km) long.<ref>{{cite web |url=http://www.ge.com/innovation/timeline/eras/continuing_tradition.html |title=1971–1985 Continuing the Tradition |work=GE Innovation Timeline |publisher=General Electric Company |accessdate=2012-09-28}}</ref> |
|||
{{Main|3D modeling}} |
|||
3D printable models may be created with a [[computer aided design]] (CAD) package, via a [[3D scanner#Hand-held laser scanners|3D scanner]] or by a plain digital camera and [[photogrammetry]] software. 3D printed models created with CAD results in reduced errors and can be corrected before printing, allowing verification in the design of the object before it is printed.<ref name="Jacobs">{{Cite book|title = Rapid Prototyping & Manufacturing: Fundamentals of Stereolithography|url = https://books.google.com/books?id=HvcN0w1VyxwC|publisher = Society of Manufacturing Engineers|date = 1992-01-01|isbn = 9780872634251|first = Paul Francis|last = Jacobs}}</ref>[[File:84530877 FillingSys (9415669149).jpg|thumb|300px|CAD model used for 3D printing]]The manual modeling process of preparing geometric data for 3D computer graphics is similar to plastic arts such as sculpting. 3D scanning is a process of collecting digital data on the shape and appearance of a real object, creating a digital model based on it. |
|||
=== Printing === |
|||
Attenuation in modern optical cables is far less than in electrical copper cables, leading to long-haul fiber connections with repeater distances of {{convert|70|–|150|km|mi|sp=us}}. The [[erbium-doped fiber amplifier]], which reduced the cost of long-distance fiber systems by reducing or eliminating optical-electrical-optical repeaters, was co-developed by teams led by [[David N. Payne]] of the [[University of Southampton]] and [[Emmanuel Desurvire]] at [[Bell Labs]] in 1986. Robust modern optical fiber uses glass for both core and sheath, and is therefore less prone to aging. It was invented by Gerhard Bernsee of [[Schott AG|Schott Glass]] in [[Germany]] in 1973.<ref>Bernsee, Gerhard (June 29, 1976) "Light conducting fibers of quartz glass" {{US patent|3966300}}</ref> |
|||
[[File:Hyperboloid Print.ogv|300px|thumb|[[Time-lapse photography|Timelapse]] video of a [[hyperboloid]] object (designed by [[George W. Hart]]) made of [[Polylactic acid|PLA]] using a RepRap "Prusa Mendel" 3 printer for molten polymer deposition]] |
|||
Before printing a 3D model from an [[STL (file format)|STL]] file, it must first be examined for "manifold errors". This step being called the "fixup."{{citation needed|date=August 2015}} Generally STLs that have been produced from a model obtained through [[3D scanner|3D scanning]] often have many manifold errors in them that need to be rectified. Examples of these errors are surfaces that do not connect, or gaps in the models.{{citation needed|date=August 2015}} |
|||
Once completed, the STL file needs to be processed by a piece of software called a "slicer," which converts the model into a series of thin layers and produces a [[G-code]] file containing instructions tailored to a specific type of 3D printer ([[Fused deposition modeling|FDM printers]]).{{citation needed|date=August 2015}} This G-code file can then be printed with 3D printing client software (which loads the G-code, and uses it to instruct the 3D printer during the 3D printing process).{{citation needed|date=August 2015}} |
|||
The emerging field of [[photonic crystal]]s led to the development in 1991 of [[photonic-crystal fiber]],<ref>{{cite journal |
|||
|journal=Science |
|||
|year=2003 |
|||
|volume=299 |
|||
|title=Photonic Crystal Fibers |
|||
|author=Russell, Philip |
|||
|doi=10.1126/science.1079280 |
|||
|pages=358–62 |
|||
|pmid=12532007 |
|||
|issue=5605|bibcode = 2003Sci...299..358R }}</ref> which guides light by [[diffraction]] from a periodic structure, rather than by total internal reflection. The first photonic crystal fibers became commercially available in 2000.<ref>{{cite web |
|||
|url=http://www.crystal-fiber.com/ |
|||
|accessdate=2008-10-22 |
|||
|title=The History of Crystal fiber A/S |
|||
|publisher=Crystal Fiber A/S}}</ref> Photonic crystal fibers can carry higher power than conventional fibers and their wavelength-dependent properties can be manipulated to improve performance. |
|||
Printer resolution describes layer thickness and X-Y resolution in [[dots per inch]] (dpi) or [[micrometer]]s (µm). Typical layer thickness is around {{convert|100|pitch|dpi|lk=on}}, although some machines can print layers as thin as {{convert|16|pitch|dpi}}.<ref name="Auto3D-17" /> X-Y resolution is comparable to that of laser printers. The particles (3D dots) are around {{convert|50|to|100|pitch|dpi}} in diameter.{{citation needed|date=August 2015}} |
|||
==Uses== |
|||
Construction of a model with contemporary methods can take anywhere from several hours to several days, depending on the method used and the size and complexity of the model. Additive systems can typically reduce this time to a few hours, although it varies widely depending on the type of machine used and the size and number of models being produced simultaneously.{{citation needed|date=October 2015}} |
|||
===Communication=== |
|||
{{Main|Fiber-optic communication}} |
|||
Optical fiber can be used as a medium for telecommunication and [[computer network]]ing because it is flexible and can be bundled as [[cables]]. It is especially advantageous for long-distance communications, because light propagates through the fiber with little attenuation compared to electrical cables. This allows long distances to be spanned with few [[optical communications repeater|repeater]]s. |
|||
Traditional techniques like [[injection moulding]] can be less expensive for manufacturing polymer products in high quantities, but additive manufacturing can be faster, more flexible and less expensive when producing relatively small quantities of parts. 3D printers give designers and concept development teams the ability to produce parts and concept models using a desktop size printer.{{citation needed|date=October 2015}} |
|||
The per-channel light signals propagating in the fiber have been modulated at rates as high as 111 [[gigabit per second|gigabits per second]] (Gbit/s) by [[Nippon Telegraph and Telephone|NTT]],<ref>[http://www.ntt.co.jp/news/news06e/0609/060929a.html 14 Tbps over a Single Optical Fiber]: Successful Demonstration of World's Largest Capacity – 145 digital high-definition movies transmitted in one second. NTT Press Release. September 29, 2006.</ref><ref>{{Cite news|author = Alfiad, M. S.|year = 2008|title = 111 Gb/s POLMUX-RZ-DQPSK Transmission over 1140 km of SSMF with 10.7 Gb/s NRZ-OOK Neighbours| periodical = Proceedings ECOC 2008|pages =Mo.4.E.2|url=https://w3.tue.nl/fileadmin/ele/TTE/ECO/Files/Pubs_2009/Alfiad_OFC_09_OThR4.pdf|display-authors=etal}}</ref> although 10 or 40 Gbit/s is typical in deployed systems.<ref>Yao, S. (2003) [http://www.generalphotonics.com/pdf/PSReprint.pdf "Polarization in Fiber Systems: Squeezing Out More Bandwidth"], The Photonics Handbook, Laurin Publishing, p. 1.</ref><ref>[http://www.ciena.com/news/news_2007pr_6976.htm Ciena, ''JANET Delivers Europe’s First 40 Gbps Wavelength Service''] 07/09/2007. Retrieved 29 Oct 2009.</ref> In June 2013, researchers demonstrated transmission of 400 Gbit/s over a single channel using 4-mode [[orbital angular momentum multiplexing]].<ref>{{Cite journal | last1 = Bozinovic | first1 = N. | last2 = Yue | first2 = Y. | last3 = Ren | first3 = Y. | last4 = Tur | first4 = M. | last5 = Kristensen | first5 = P. | last6 = Huang | first6 = H. | last7 = Willner | first7 = A. E. | last8 = Ramachandran | first8 = S. | doi = 10.1126/science.1237861 | title = Terabit-Scale Orbital Angular Momentum Mode Division Multiplexing in Fibers | journal = Science | volume = 340 | issue = 6140 | pages = 1545 | year = 2013 | pmid = | pmc = }}</ref> |
|||
=== Finishing === |
|||
Each fiber can carry many independent channels, each using a different wavelength of light ([[wavelength-division multiplexing]] (WDM)). The net data rate (data rate without overhead bytes) per fiber is the per-channel data rate reduced by the FEC overhead, multiplied by the number of channels (usually up to eighty in commercial [[dense WDM]] systems {{As of|2008|lc=on}}). {{As of|2011}} the record for bandwidth on a single core was 101 Tbit/s (370 channels at 273 Gbit/s each).<ref>{{cite journal |
|||
Though the printer-produced resolution is sufficient for many applications, printing a slightly oversized version of the desired object in standard resolution and then removing material<ref name="smooth" /> with a higher-resolution subtractive process can achieve greater precision. |
|||
| title = Ultrafast fibre optics set new speed record |
|||
| journal=New Scientist |
|||
| volume=210 |
|||
| issue=2809 |
|||
| pages=24 |
|||
| date = 2011-04-29 |
|||
| url = http://www.newscientist.com/article/mg21028095.500-ultrafast-fibre-optics-set-new-speed-record.html |
|||
| accessdate = 2012-02-26| bibcode=2011NewSc.210R..24H |
|||
| author1=Hecht |
|||
| first1=Jeff |
|||
| doi=10.1016/S0262-4079(11)60912-3 |
|||
}}</ref> |
|||
The record for a multi-core fiber as of January 2013 was 1.05 petabits per second. |
|||
<ref>{{cite web |
|||
| title = NEC and Corning achieve petabit optical transmission |
|||
| publisher = Optics.org |
|||
| date = 2013-01-22 |
|||
| url = http://optics.org/news/4/1/29 |
|||
| accessdate = 2013-01-23}}</ref> |
|||
In 2009, Bell Labs broke the 100 (petabit per second)×kilometer barrier (15.5 Tbit/s over a single 7,000 km fiber).<ref>[http://www.physorg.com/news173455192.html/ Bell Labs breaks optical transmission record, 100 Petabit per second kilometer barrier]. Physorg. September 29, 2009</ref> |
|||
Some printable polymers allow the surface finish to be smoothed and improved using chemical vapor processes.{{citation needed|date=August 2015}} |
|||
For short distance application, such as a network in an office building, fiber-optic cabling can save space in cable ducts. This is because a single fiber can carry much more data than electrical cables such as standard [[Cat-5|category 5]] Ethernet cabling, which typically runs at 100 Mbit/s or 1 Gbit/s speeds. Fiber is also immune to electrical interference; there is no cross-talk between signals in different cables, and no pickup of environmental noise. Non-armored fiber cables do not conduct electricity, which makes fiber a good solution for protecting communications equipment in [[high voltage]] environments, such as [[power generation]] facilities, or metal communication structures prone to [[lightning]] strikes. They can also be used in environments where explosive fumes are present, without danger of ignition. [[Wiretapping]] (in this case, [[fiber tapping]]) is more difficult compared to electrical connections, and there are concentric dual-core fibers that are said to be tap-proof.<ref name="Siemens Fiber Optics">[http://www.automation.siemens.com/net/html_76/produkte/040_ie_fc_glasleitungen.htm Siemen's claim to a fiber optic line that cannot be tapped]. Retrieved 18 Dec 2009.</ref> |
|||
Some additive manufacturing techniques are capable of using multiple materials in the course of constructing parts. These techniques are able to print in multiple colors and color combinations simultaneously, and would not necessarily require painting. |
|||
Fibers are often also used for short-distance connections between devices. For example, most [[high-definition television]]s offer a digital audio optical connection. This allows the streaming of audio over light, using the [[TOSLINK]] protocol. |
|||
Some printing techniques require internal supports to be built for overhanging features during construction. These supports must be mechanically removed or dissolved upon completion of the print. |
|||
====Advantages over copper wiring==== |
|||
The advantages of optical fiber communication with respect to copper wire systems are: |
|||
All of the commercialized metal 3D printers involve cutting the metal component off of the metal substrate after deposition. A new process for the [[GMAW]] 3D printing allows for substrate surface modifications to remove [[aluminum]]<ref>{{cite journal |authors=Amberlee S. Haselhuhn, Eli J. Gooding, Alexandra G. Glover, Gerald C. Anzalone, Bas Wijnen, Paul G. Sanders, Joshua M. Pearce. |title=Substrate Release Mechanisms for Gas Metal Arc 3-D Aluminum Metal Printing |journal=3D Printing and Additive Manufacturing |volume=1 |issue=4 |pp=204–209 |year=2014 |doi=10.1089/3dp.2014.0015 |url=http://dx.doi.org/10.1089/3dp.2014.0015|doi-broken-date=2015-12-05 }}</ref> or [[steel]].<ref>Amberlee S. Haselhuhn, Bas Wijnen, Gerald C. Anzalone, Paul G. Sanders, Joshua M. Pearce, [https://www.academia.edu/14451546/In_Situ_Formation_of_Substrate_Release_Mechanisms_for_Gas_Metal_Arc_Weld_Metal_3-D_Printing In Situ Formation of Substrate Release Mechanisms for Gas Metal Arc Weld Metal 3-D Printing]. ''Journal of Materials Processing Technology''. 226, pp. 50–59 (2015) DOI: 10.1016/j.jmatprotec.2015.06.038 10.1016/j.jmatprotec.2015.06.038</ref> |
|||
;Broad bandwidth: A single optical fiber can carry over 3,000,000 full-duplex voice calls or 90,000 TV channels. |
|||
== Processes == |
|||
;Immunity to electromagnetic interference: Light transmission through optical fibers is unaffected by other [[electromagnetic radiation]] nearby. The optical fiber is electrically non-conductive, so it does not act as an antenna to pick up electromagnetic signals. Information traveling inside the optical fiber is immune to [[electromagnetic interference]], even [[electromagnetic pulse]]s generated by nuclear devices. |
|||
Several 3D printing processes have been invented since the late 1970s. The printers were originally large, expensive, and highly limited in what they could produce.<ref name="3D opp" /> |
|||
A large number of additive processes are now available. The main differences between processes are in the way layers are deposited to create parts and in the materials that are used. Some methods melt or soften the material to produce the layers, for example. [[selective laser melting]] (SLM) or [[direct metal laser sintering]] (DMLS), [[selective laser sintering]] (SLS), [[fused deposition modeling]] (FDM),<ref>FDM is a proprietary term owned by [[Stratasys]]. All 3-D printers that are not Stratasys machines and use a fused filament process are referred to as or fused filament fabrication (FFF).</ref> or fused filament fabrication (FFF), while others cure liquid materials using different sophisticated technologies, such as [[stereolithography]] (SLA). With [[laminated object manufacturing]] (LOM), thin layers are cut to shape and joined together (e.g., paper, polymer, metal). Each method has its own advantages and drawbacks, which is why some companies offer a choice of powder and polymer for the material used to build the object.<ref name="Auto3D-7" /> Others sometimes use standard, off-the-shelf business paper as the build material to produce a durable prototype. The main considerations in choosing a machine are generally speed, costs of the 3D printer, of the printed prototype, choice and cost of the materials, and color capabilities.<ref name="Auto3D-8" /> |
|||
;Low attenuation loss over long distances: Attenuation loss can be as low as 0.2 dB/km in optical fiber cables, allowing transmission over long distances without the need for [[repeater]]s. |
|||
Printers that work directly with metals are generally expensive. However less expensive printers can be used to make a mold, which is then used to make metal parts.<ref name="Auto3D-9" /> |
|||
;Electrical insulator: Optical fibers do not conduct electricity, preventing problems with [[Ground loop (electricity)|ground loop]]s and conduction of [[lightning]]. Optical fibers can be strung on poles alongside high voltage power cables. |
|||
{|class="wikitable" |
|||
;Material cost and theft prevention: Conventional cable systems use large amounts of copper. In some places, this copper is a target for theft due to its value on the scrap market. |
|||
|- |
|||
! Type |
|||
! Technologies |
|||
! Materials |
|||
|- |
|||
|rowspan="2"| Extrusion |
|||
| [[Fused deposition modeling]] (FDM) or [[Fused Filament Fabrication]] (FFF) |
|||
| [[Thermoplastics]], [[eutectic]] metals, edible materials, [[Rubber]]s, [[Modeling clay]], [[Plasticine]], [[Metal clay]] (including [[Precious Metal Clay]]) |
|||
|- |
|||
|[[Robocasting]] or Direct Ink Writing (DIW) |
|||
|[[Ceramic materials]], [[Metal alloy]], [[cermet]], [[metal matrix composite]], [[ceramic matrix composite]] |
|||
|- |
|||
|rowspan="2"| Light polymerized |
|||
| [[Stereolithography]] (SLA) |
|||
| [[Photopolymer]] |
|||
|- |
|||
| [[Digital Light Processing]] (DLP) |
|||
| Photopolymer |
|||
|- |
|||
|rowspan="6"| Powder Bed |
|||
| [[Powder bed and inkjet head 3D printing]] (3DP) |
|||
| Almost any [[metal alloy]], powdered polymers, [[Plaster]] |
|||
|- |
|||
| [[Electron-beam melting]] (EBM) |
|||
| Almost any [[metal alloy]] including [[Titanium alloy]]s |
|||
|- |
|||
| [[Selective laser melting]] (SLM) |
|||
| [[Titanium alloys]], [[Cobalt-chrome|Cobalt Chrome alloys]], [[Stainless Steel]], Aluminium |
|||
|- |
|||
| [[Selective heat sintering]] (SHS)<ref name="AutoSQ-14" /> |
|||
| Thermoplastic powder |
|||
|- |
|||
| [[Selective laser sintering]] (SLS) |
|||
| [[Thermoplastic]]s, [[Sintering#Sintering of metallic powders|metal powders]], [[Sintering#Ceramic sintering|ceramic powders]] |
|||
|- |
|||
| [[Direct metal laser sintering]] (DMLS) |
|||
| Almost any [[metal alloy]] |
|||
|- |
|||
| Laminated |
|||
| [[Laminated object manufacturing]] (LOM) |
|||
| Paper, [[metal foil]], [[plastic film]] |
|||
|- |
|||
|rowspan="2"| Wire |
|||
| [[Electron beam freeform fabrication]] (EBF<sup>3</sup>) |
|||
| Almost any [[metal alloy]] |
|||
|} |
|||
=== Extrusion deposition === |
|||
;Security of information passed down the cable: Copper can be tapped with very little chance of detection. |
|||
[[File:FDM by Zureks.png|thumb|Fused deposition modeling: 1 – nozzle ejecting molten material, 2 – deposited material (modeled part), 3 – controlled movable table]] |
|||
{{Main|Fused deposition modeling}} |
|||
[[File:Robot 3D print timelapse on RepRapPro Fisher.webm|thumb|A timelapse video of a robot model (logo of [[Make (magazine)|Make magazine]]) being printed using FDM on a RepRapPro Fisher printer.]] |
|||
Fused deposition modeling (FDM), derives from automatic polymeric foil hot air welding system, hot-melt glueing and automatic gasket deposition. Such principle has been further developed by [[S. Scott Crump]] in the late 1980s and was commercialized in 1990 by [[Stratasys]].<ref name="Auto3D-10" /> After the patent on this technology expired, a large open-source development community developed and both commercial and [[DIY]] variants utilizing this type of 3D printer appeared. As a result, the price of this technology has dropped by two orders of magnitude since its creation. |
|||
In fused deposition modeling the model or part is produced by extruding small beads of material which harden immediately to form layers. A [[thermoplastic]] filament or metal wire that is wound on a coil is unreeled to supply material to an [[extrusion]] nozzle head ([[3D printer extruder]]). The nozzle head heats the material and turns the flow on and off. Typically [[stepper motors]] or [[servo motors]] are employed to move the extrusion head and adjust the flow. The printer usually has 3 axes of motion. A [[computer-aided manufacturing]] (CAM) software package is used to generate the G-Code that is sent to a [[microcontroller]] which controls the motors. |
|||
===Sensors=== |
|||
{{Main|Fiber optic sensor}} |
|||
Fibers have many uses in remote sensing. In some applications, the sensor is itself an optical fiber. In other cases, fiber is used to connect a non-fiberoptic sensor to a measurement system. Depending on the application, fiber may be used because of its small size, or the fact that no [[electrical power]] is needed at the remote location, or because many sensors can be [[multiplexing|multiplexed]] along the length of a fiber by using different wavelengths of light for each sensor, or by sensing the time delay as light passes along the fiber through each sensor. Time delay can be determined using a device such as an ''[[optical time-domain reflectometer]]''. |
|||
Extrusion in 3D printing using material extrusion involves a [[cold end]] and a [[hot end]]. |
|||
Optical fibers can be used as sensors to measure [[deformation (mechanics)|strain]], [[temperature]], [[pressure]] and other quantities by modifying a fiber so that the property to measure modulates the [[intensity (physics)|intensity]], [[phase (waves)|phase]], [[polarization (waves)|polarization]], [[wavelength]], or transit time of light in the fiber. Sensors that vary the intensity of light are the simplest, since only a simple source and detector are required. A particularly useful feature of such fiber optic sensors is that they can, if required, provide distributed sensing over distances of up to one meter. In contrast, highly localized measurements can be provided by integrating miniaturized sensing elements with the tip of the fiber.<ref>{{Cite journal |
|||
| pmid = 24599822 |
|||
| year = 2014 |
|||
| author1 = Kostovski |
|||
| first1 = G |
|||
| title = The optical fiber tip: An inherently light-coupled microscopic platform for micro- and nanotechnologies |
|||
| journal = Advanced Materials |
|||
| volume = 26 |
|||
| issue = 23 |
|||
| pages = 3798–820 |
|||
| last2 = Stoddart |
|||
| first2 = P. R. |
|||
| last3 = Mitchell |
|||
| first3 = A |
|||
| doi = 10.1002/adma.201304605 |
|||
}}</ref> These can be implemented by various micro- and nanofabrication technologies, such that they do not exceed the microscopic boundary of the fiber tip, allowing such applications as insertion into blood vessels via hypodermic needle. |
|||
Various polymers are used, including [[acrylonitrile butadiene styrene]] (ABS), [[polycarbonate]] (PC), [[polylactic acid]] (PLA), [[high density polyethylene]] (HDPE), PC/ABS, [[polyphenylsulfone]] (PPSU) and [[high impact polystyrene]] (HIPS). In general, the polymer is in the form of a filament fabricated from virgin resins. There are multiple projects in the open-sourced community aimed at processing post-consumer plastic waste into filament. These involve machines used to shred and extrude the plastic material into filament. |
|||
Extrinsic fiber optic sensors use an [[optical fiber cable]], normally a multi-mode one, to transmit [[modulation|modulated]] light from either a non-fiber optical sensor—or an electronic sensor connected to an optical transmitter. A major benefit of extrinsic sensors is their ability to reach otherwise inaccessible places. An example is the measurement of temperature inside [[aircraft]] [[jet engine]]s by using a fiber to transmit [[radiation]] into a radiation [[pyrometer]] outside the engine. Extrinsic sensors can be used in the same way to measure the internal temperature of [[electrical transformer]]s, where the extreme [[electromagnetic field]]s present make other measurement techniques impossible. Extrinsic sensors measure vibration, rotation, displacement, velocity, acceleration, torque, and twisting. A solid state version of the gyroscope, using the interference of light, has been developed. The ''[[fiber optic gyroscope]] (FOG)'' has no moving parts, and exploits the ''[[Sagnac effect]]'' to detect mechanical rotation. |
|||
FDM is somewhat restricted in the variation of shapes that may be fabricated. For example, FDM usually cannot produce stalactite-like structures, since they would be unsupported during the build. Otherwise, a thin support must be designed into the structure which can be broken away during finishing. Fused deposition modeling is also referred to as fused filament fabrication (FFF) by companies who do not hold the original patents like Stratasys does. |
|||
Common uses for fiber optic sensors includes advanced intrusion detection security systems. The light is transmitted along a fiber optic sensor cable placed on a fence, pipeline, or communication cabling, and the returned signal is monitored and analyzed for disturbances. This return signal is digitally processed to detect disturbances and trip an alarm if an intrusion has occurred. |
|||
=== Binding of granular materials === |
|||
===Power transmission=== |
|||
Another 3D printing approach is the selective fusing of materials in a granular bed. The technique fuses parts of the layer and then moves downward in the working area, adding another layer of granules and repeating the process until the piece has built up. This process uses the unfused media to support overhangs and thin walls in the part being produced, which reduces the need for temporary auxiliary supports for the piece. A laser is typically used to [[sintering|sinter]] the media into a solid. Examples include [[selective laser sintering]] (SLS), with both metals and polymers (e.g., PA, PA-GF, Rigid GF, PEEK, PS, [[Alumide]], Carbonmide, elastomers), and [[direct metal laser sintering]] (DMLS).<ref name="DMLS" /> |
|||
Optical fiber can be used to transmit power using a [[photovoltaic cell]] to convert the light into electricity.<ref>[http://spectrum.ieee.org/energy/the-smarter-grid/electricity-over-glass IEEE Spectrum: Electricity Over Glass]. IEEE Spectrum. October 2005</ref> While this method of power transmission is not as efficient as conventional ones, it is especially useful in situations where it is desirable not to have a metallic conductor as in the case of use near MRI machines, which produce strong magnetic fields.<ref>[http://www2.electronicproducts.com/Photovoltaic_feat_advances_power_over_optical_fiber-article-olap01-jun2006-html.aspx Photovoltaic feat advances power over optical fiber – Electronic Products]. 06/01/2006</ref> Other examples are for powering electronics in high-powered antenna elements and measurement devices used in high-voltage transmission equipment. |
|||
[[Selective laser sintering|Selective Laser Sintering]] (SLS) was developed and patented by Dr. Carl Deckard and Dr. Joseph Beaman at the [[University of Texas at Austin]] in the mid-1980s, under sponsorship of [[DARPA]].<ref name="Auto3D-3" /> A similar process was patented without being commercialized by R. F. Housholder in 1979.<ref name="Auto3D-4" /> |
|||
===Other uses=== |
|||
[[File:Flashflight red.jpg|thumb|A [[frisbee]] illuminated by fiber optics]] |
|||
[[File:OpticFiber.jpg|thumb|left|Light reflected from optical fiber illuminates exhibited model]] |
|||
[[Selective laser melting]] (SLM) does not use sintering for the fusion of powder granules but will completely melt the powder using a high-energy laser to create fully dense materials in a layer-wise method that has mechanical properties similar to those of conventional manufactured metals. |
|||
Optical fibers have a wide number of applications. They are used as [[light tube|light guide]]s in medical and other applications where bright light needs to be shone on a target without a clear line-of-sight path. In some buildings, optical fibers route sunlight from the roof to other parts of the building (see [[nonimaging optics]]). [[Optical fiber lamp]]s are used for illumination in decorative applications, including [[Commercial signage|sign]]s, [[art]], toys and artificial [[Christmas tree]]s. [[Swarovski]] boutiques use optical fibers to illuminate their crystal showcases from many different angles while only employing one light source. Optical fiber is an intrinsic part of the light-transmitting concrete building product, [[LiTraCon]]. |
|||
[[Electron beam melting]] (EBM) is a similar type of additive manufacturing technology for metal parts (e.g. [[titanium alloy]]s). EBM manufactures parts by melting metal powder layer by layer with an electron beam in a high vacuum. Unlike metal sintering techniques that operate below melting point, EBM parts void-freeR.<ref name="Auto3D-12" /><ref name="Auto3D-13" /> |
|||
[[File:Use of optical fiber in a lamp..JPG|thumb|Use of optical fiber in a decorative lamp or nightlight.]] |
|||
Optical fiber is also used in imaging optics. A coherent bundle of fibers is used, sometimes along with lenses, for a long, thin imaging device called an [[endoscope]], which is used to view objects through a small hole. Medical endoscopes are used for minimally invasive exploratory or surgical procedures. Industrial endoscopes (see [[fiberscope]] or [[borescope]]) are used for inspecting anything hard to reach, such as jet engine interiors. Many [[microscopes]] use fiber-optic light sources to provide intense illumination of samples being studied. |
|||
Another method consists of an [[Powder bed and inkjet head 3D printing|inkjet 3D printing]] system. The printer creates the model one layer at a time by spreading a layer of powder ([[plaster]], or [[resin]]s) and printing a binder in the cross-section of the part using an inkjet-like process. This is repeated until every layer has been printed. This technology allows the printing of full color prototypes, overhangs, and elastomer parts. The strength of bonded powder prints can be enhanced with wax or [[thermoset]] polymer impregnation. |
|||
In [[spectroscopy]], optical fiber bundles transmit light from a spectrometer to a substance that cannot be placed inside the spectrometer itself, in order to analyze its composition. A spectrometer analyzes substances by bouncing light off and through them. By using fibers, a spectrometer can be used to study objects remotely.<ref>{{cite journal |title=In situ real-time monitoring of a fermentation reaction using a fiber-optic FT-IR probe |journal=Spectroscopy |date=June 2001 |first=Zaid |last=Al Mosheky |author2=Melling, Peter J. |author3=Thomson, Mary A. |url=http://www.remspec.com/pdfs/SP5619.pdf |format=PDF|volume= 16|issue =6|page=15}}</ref><ref>{{cite journal |title=Reaction monitoring in small reactors and tight spaces |first=Peter |last=Melling |author2=Thomson, Mary |journal=American Laboratory News |date=October 2002 |url=http://www.remspec.com/pdfs/amlab1002.pdf |format=PDF}}</ref><ref>{{cite book |chapter=Fiber-optic probes for mid-infrared spectrometry |first=Peter J. |last=Melling |author2=Thomson, Mary |title=Handbook of Vibrational Spectroscopy |editors=Chalmers, John M.; Griffiths, Peter R. (eds.)|publisher=Wiley |year=2002 |url=http://www.remspec.com/pdfs/2703_o.pdf |format=PDF}}</ref> |
|||
=== Lamination === |
|||
An optical fiber [[dopant|doped]] with certain [[rare earth element]]s such as [[erbium]] can be used as the [[gain medium]] of a [[fiber laser|laser]] or [[optical amplifier]]. Rare-earth-doped optical fibers can be used to provide signal [[amplifier|amplification]] by splicing a short section of doped fiber into a regular (undoped) optical fiber line. The doped fiber is [[optical pumping|optically pumped]] with a second laser wavelength that is coupled into the line in addition to the signal wave. Both wavelengths of light are transmitted through the doped fiber, which transfers energy from the second pump wavelength to the signal wave. The process that causes the amplification is [[stimulated emission]]. |
|||
{{Main|Laminated object manufacturing}} |
|||
In some printers, paper can be used as the build material, resulting in a lower cost to print. During the 1990s some companies marketed printers that cut cross sections out of special adhesive coated paper using a carbon dioxide laser and then laminated them together. |
|||
In 2005 [[Mcor Technologies Ltd]] developed a different process using ordinary sheets of office paper, a [[tungsten carbide]] blade to cut the shape, and selective deposition of adhesive and pressure to bond the prototype.<ref name="AutoSQ-15" /> |
|||
Optical fiber is also widely exploited as a nonlinear medium. The glass medium supports a host of nonlinear optical interactions, and the long interaction lengths possible in fiber facilitate a variety of phenomena, which are harnessed for applications and fundamental investigation.<ref name="Agrawal2012">{{Cite book | isbn = 0123970237 | title = Nonlinear Fiber Optics, Fifth Edition | last1 = Govind | first1 = Agrawal | year = <!--replace this comment with the publication year--> | pages = }}</ref> Conversely, fiber nonlinearity can have deleterious effects on optical signals, and measures are often required to minimize such unwanted effects. |
|||
There are also a number of companies selling printers that print laminated objects using thin plastic and metal sheets. |
|||
Optical fibers doped with a [[wavelength shifter]] collect [[scintillator|scintillation]] light in [[physics experiment]]s. |
|||
=== Photopolymerization === |
|||
[[Iron sights#Fiber optic|Fiber optic sight]]s for handguns, rifles, and shotguns use pieces of optical fiber to improve visibility of markings on the sight. |
|||
[[File:Stereolithography apparatus.jpg|thumb|Stereolithography apparatus]] |
|||
{{Main|Stereolithography}} |
|||
Stereolithography was patented in 1986 by [[Chuck Hull]].<ref name="AutoSQ-16" /> [[Photopolymerization]] is primarily used in stereolithography (SLA) to produce a solid part from a liquid. This process was a dramatic departure from the "[[photosculpture]]" method of [[François Willème]] (1830–1905) developed in 1860 and the [[photopolymerization]] of Mitsubishi's Matsubara in 1974.<ref name="NSF RP Report" /> |
|||
The "photosculpture" method consisted of photographing a subject from a variety of equidistant angles and projecting each photograph onto a screen, where a [[pantograph]] was used to trace the outline onto modeling clay.<ref name="AutoSQ-17" /><ref name="AutoSQ-18" /><ref name="AutoSQ-19" /> In photo-polymerization, a vat of liquid polymer is exposed to controlled lighting under [[safelight]] conditions. The exposed liquid polymer hardens. Polymerization occurs when photopolymers are exposed to light when photopolymers contain [[chromophore]]s, otherwise, the addition of molecules that are photosensitive are utilized to react with the solution to begin polymerization. Polymerization of monomers lead to cross-linking, which creates a polymer. Through these [[Covalent bond|covalent]] bonds, the property of the solution is changed.<ref name="Jacobs"/> The build plate then moves down in small increments and the liquid polymer is again exposed to light. The process repeats until the model has been built. The liquid polymer is then drained from the vat, leaving the solid model. The [[EnvisionTEC]] ''Perfactory''<ref name="Auto3D-14" /> is an example of a DLP rapid prototyping system. |
|||
==Principle of operation== |
|||
[[File:Fiber-engineerguy.ogv|thumb|An overview of the operating principles of the optical fiber]] |
|||
An optical fiber is a cylindrical [[dielectric waveguide]] ([[insulator (electrical)|nonconducting]] waveguide) that transmits light along its axis, by the process of [[total internal reflection]]. The fiber consists of a ''core'' surrounded by a [[cladding (fiber optics)|cladding]] layer, both of which are made of [[dielectric]] materials. To confine the optical signal in the core, the [[refractive index]] of the core must be greater than that of the cladding. The boundary between the core and cladding may either be abrupt, in ''[[step-index profile|step-index fiber]]'', or gradual, in ''[[graded-index fiber]]''. |
|||
Inkjet printer systems like the ''Objet PolyJet'' system spray photopolymer materials onto a build tray in ultra-thin layers (between 16 and 30 µm) until the part is completed. Each photopolymer layer is [[Curing (chemistry)|cured]] with UV light after it is jetted, producing fully cured models that can be handled and used immediately, without post-curing. The gel-like support material, which is designed to support complicated geometries, is removed by hand and water jetting. It is also suitable for elastomers. |
|||
===Index of refraction=== |
|||
{{Main|Refractive index}} |
|||
The index of refraction (or refractive index) is a way of measuring the [[speed of light]] in a material. Light travels fastest in a [[vacuum]], such as in outer space. The speed of light in a vacuum is about 300,000 kilometers (186,000 miles) per second. The refractive index of a medium is calculated by dividing the speed of light in a vacuum by the speed of light in that medium. The refractive index of a vacuum is therefore 1, by definition. A typical singlemode fiber used for telecommunications has a cladding made of pure silica, with an index of 1.444 at 1,500 nm, and a core of doped silica with an index around 1.4475.<ref>{{cite web |title=Fibers |url=https://www.rp-photonics.com/fibers.html |work=[[Encyclopedia of Laser Physics and Technology]] |publisher=RP Photonics |first=Rüdiger |last=Paschotta |accessdate=Feb 22, 2015}}</ref> The larger the index of refraction, the slower light travels in that medium. From this information, a simple rule of thumb is that a signal using optical fiber for communication will travel at around 200,000 [[kilometers]] per second. To put it another way, the signal will take 5 [[milliseconds]] to travel 1,000 kilometers in fiber. Thus a phone call carried by fiber between Sydney and New York, a 16,000-kilometer distance, means that there is a minimum delay of 80 milliseconds (about <math>\tfrac {1}{12}</math> of a second) between when one caller speaks and the other hears. (The fiber in this case will probably travel a longer route, and there will be additional delays due to communication equipment switching and the process of encoding and decoding the voice onto the fiber). |
|||
Ultra-small features can be made with the 3D micro-fabrication technique used in [[two-photon absorption|multiphoton]] photopolymerisation. This approach uses a focused laser to trace the desired 3D object into a block of gel. Due to the nonlinear nature of photo excitation, the gel is cured to a solid only in the places where the laser was focused while the remaining gel is then washed away. Feature sizes of under 100 nm are easily produced, as well as complex structures with moving and interlocked parts.<ref name="Auto3D-15" /> |
|||
===Total internal reflection=== |
|||
{{Main|Total internal reflection}} |
|||
When light traveling in an optically dense medium hits a boundary at a steep angle (larger than the [[critical angle (optics)|critical angle]] for the boundary), the light is completely reflected. This is called total internal reflection. This effect is used in optical fibers to confine light in the core. Light travels through the fiber core, bouncing back and forth off the boundary between the core and cladding. Because the light must strike the boundary with an angle greater than the critical angle, only light that enters the fiber within a certain range of angles can travel down the fiber without leaking out. This range of angles is called the [[acceptance cone]] of the fiber. The size of this acceptance cone is a function of the refractive index difference between the fiber's core and cladding. |
|||
Yet another approach uses a synthetic resin that is solidified using [[LED]]s.<ref name="Auto3D-16" /> |
|||
In simpler terms, there is a maximum angle from the fiber axis at which light may enter the fiber so that it will propagate, or travel, in the core of the fiber. The [[sine]] of this maximum angle is the [[numerical aperture]] (NA) of the fiber. Fiber with a larger NA requires less precision to splice and work with than fiber with a smaller NA. Single-mode fiber has a small NA. |
|||
In Mask-image-projection-based stereolithography a 3D digital model is sliced by a set of horizontal planes. Each slice is converted into a two-dimensional mask image. The mask image is then projected onto a photocurable liquid resin surface and light is projected onto the resin to cure it in the shape of the layer.<ref name=k1113 /> The technique has been used to create objects composed of multiple materials that cure at different rates.<ref name=k1113 /> In research systems, the light is projected from below, allowing the resin to be quickly spread into uniform thin layers, reducing production time from hours to minutes.<ref name=k1113 /> Commercially available devices such as Objet Connex apply the resin via small nozzles.<ref name="k1113" /> |
|||
===Multi-mode fiber=== |
|||
[[File:Optical-fibre.svg|thumb|The propagation of light through a [[multi-mode optical fiber]].]] |
|||
[[File:Laser in fibre.jpg|thumb|A laser bouncing down an [[poly(methyl methacrylate)|acrylic]] rod, illustrating the total internal reflection of light in a multi-mode optical fiber.]] |
|||
{{Main|Multi-mode optical fiber}} |
|||
Fiber with large core diameter (greater than 10 micrometers) may be analyzed by [[geometrical optics]]. Such fiber is called ''multi-mode fiber'', from the electromagnetic analysis (see below). In a step-index multi-mode fiber, [[ray (optics)|rays]] of light are guided along the fiber core by total internal reflection. Rays that meet the core-cladding boundary at a high angle (measured relative to a line [[surface normal|normal]] to the boundary), greater than the [[critical angle (optics)|critical angle]] for this boundary, are completely reflected. The critical angle (minimum angle for total internal reflection) is determined by the difference in index of refraction between the core and cladding materials. Rays that meet the boundary at a low angle are refracted from the [[core (optical fiber)|core]] into the cladding, and do not convey light and hence information along the fiber. The critical angle determines the [[guided ray|acceptance angle]] of the fiber, often reported as a [[numerical aperture]]. A high numerical aperture allows light to propagate down the fiber in rays both close to the axis and at various angles, allowing efficient coupling of light into the fiber. However, this high numerical aperture increases the amount of [[dispersion (optics)|dispersion]] as rays at different angles have different [[optical path length|path lengths]] and therefore take different times to traverse the fiber. |
|||
=== Metal wire processes === |
|||
[[File:Optical fiber types.svg|thumb|Optical fiber types.]]In graded-index fiber, the index of refraction in the core decreases continuously between the axis and the cladding. This causes light rays to bend smoothly as they approach the cladding, rather than reflecting abruptly from the core-cladding boundary. The resulting curved paths reduce multi-path dispersion because high angle rays pass more through the lower-index periphery of the core, rather than the high-index center. The index profile is chosen to minimize the difference in axial propagation speeds of the various rays in the fiber. This ideal index profile is very close to a [[parabola|parabolic]] relationship between the index and the distance from the axis. |
|||
Laser-based wirefeed systems, such as Laser Metal Deposition-wire (LMD-w), feed wire through a nozzle that is melted by a laser using inert gas shielding in either an open environment (gas surrounding the laser), or in a sealed chamber. [[Electron beam freeform fabrication]] uses an electron beam heat source inside a vacuum chamber. |
|||
It is also possible to use conventional [[gas metal arc welding]] attached to a 3D stage to 3-D print metals such as steel and aluminum.<ref>Yuenyong Nilsiam, Amberlee Haselhuhn, Bas Wijnen, Paul Sanders, & Joshua M. Pearce. [https://www.academia.edu/17673716/Integrated_Voltage_Current_Monitoring_and_Control_of_Gas_Metal_Arc_Weld_Magnetic_Ball-Jointed_Open_Source_3-D_Printer Integrated Voltage - Current Monitoring and Control of Gas Metal Arc Weld Magnetic Ball-Jointed Open Source 3-D Printer].''Machines'' '''3'''(4), 339-351 (2015).</ref> Low-cost open source [[RepRap]]-style 3-D printers have been outfitted with [[Arduino]]-based [[sensors]] and demonstrated reasonable metallurgical properties from conventional welding wire as feedstock.<ref>A. Pinar, B. Wijnen, G. C. Anzalone, T. C. Havens, P. G. Sanders, J. M. Pearce. [https://www.academia.edu/15282691/Low-cost_Open-Source_Voltage_and_Current_Monitor_for_Gas_Metal_Arc_Weld_3-D_Printing Low-cost Open-Source Voltage and Current Monitor for Gas Metal Arc Weld 3-D Printing]. ''Journal of Sensors'' Vol. 2015, Article ID 876714, 8 pages, 2015.</ref> |
|||
===Single-mode fiber=== |
|||
[[File:Singlemode fibre structure.svg|thumb|The structure of a typical [[single-mode fiber]].<br /> |
|||
1. Core: 8 µm diameter<br /> |
|||
2. Cladding: 125 µm dia.<br /> |
|||
3. Buffer: 250 µm dia.<br /> |
|||
4. Jacket: 400 µm dia.]] |
|||
{{Main|Single-mode optical fiber}} |
|||
Fiber with a core diameter less than about ten times the [[wavelength]] of the propagating light cannot be modeled using geometric optics. Instead, it must be analyzed as an [[electromagnetism|electromagnetic]] structure, by solution of [[Maxwell's equations]] as reduced to the [[electromagnetic wave equation]]. The electromagnetic analysis may also be required to understand behaviors such as speckle that occur when [[coherence (physics)|coherent]] light propagates in multi-mode fiber. As an optical waveguide, the fiber supports one or more confined [[transverse mode]]s by which light can propagate along the fiber. Fiber supporting only one mode is called ''single-mode'' or ''mono-mode fiber''. The behavior of larger-core multi-mode fiber can also be modeled using the wave equation, which shows that such fiber supports more than one mode of propagation (hence the name). The results of such modeling of multi-mode fiber approximately agree with the predictions of geometric optics, if the fiber core is large enough to support more than a few modes. |
|||
== Printers == |
|||
The waveguide analysis shows that the light energy in the fiber is not completely confined in the core. Instead, especially in single-mode fibers, a significant fraction of the energy in the bound mode travels in the cladding as an [[evanescent wave]]. |
|||
=== Industry use === |
|||
The most common type of single-mode fiber has a core diameter of 8–10 micrometers and is designed for use in the [[near infrared]]. The mode structure depends on the wavelength of the light used, so that this fiber actually supports a small number of additional modes at visible wavelengths. Multi-mode fiber, by comparison, is manufactured with core diameters as small as 50 micrometers and as large as hundreds of micrometers. The [[normalized frequency (fiber optics)|normalized frequency]] ''V'' for this fiber should be less than the first zero of the [[Bessel function]] ''J''<sub>0</sub> (approximately 2.405). |
|||
{{expand section|date=August 2015}} |
|||
As October 2012, additive manufacturing systems were on the market that ranged from $2,000 to $500,000 in price and were employed in industries including aerospace, architecture, automotive, defense, and medical replacements, among many others. For example, [[General Electric]] uses the high-end model to build parts for [[turbine]]s.<ref name="cfr2013" /> Many of these systems are used for rapid prototyping, before mass production methods are employed. |
|||
=== Consumer use === |
|||
===Special-purpose fiber=== |
|||
[[File:RepRap 'Mendel'.jpg|thumb|[[RepRap Project|RepRap]] version 2.0 (Mendel)]] |
|||
Some special-purpose optical fiber is constructed with a non-cylindrical core and/or cladding layer, usually with an elliptical or rectangular cross-section. These include [[polarization-maintaining optical fiber|polarization-maintaining fiber]] and fiber designed to suppress [[whispering gallery mode]] propagation. Polarization-maintaining fiber is a unique type of fiber that is commonly used in fiber optic sensors due to its ability to maintain the polarization of the light inserted into it. |
|||
[[File:First replication.jpg|thumb|All of the plastic parts for the machine on the right were produced by the machine on the left. [[Adrian Bowyer]] (left) and Vik Olliver (right) are members of the [[RepRap project]].]] |
|||
[[File:Turntable with 5v psu.jpg|thumb|[[Meccano]] repstrap of RepRap 0.1 prototype (created by Vik Olliver)]] |
|||
Several projects and companies are making efforts to develop affordable 3D printers for home desktop use. Much of this work has been driven by and targeted at [[Do it yourself|DIY]]/[[Maker culture|Maker]]/enthusiast/[[early adopter]] communities, with additional ties to the academic and [[Hacker (hobbyist)|hacker]] communities.<ref name="Auto3D-26" /> |
|||
[[RepRap Project]] is one of the longest running projects in the desktop category. The [[RepRap project]] aims to produce a [[free and open source hardware]] (FOSH) 3D printer, whose full specifications are released under the [[GNU General Public License]], and which is capable of replicating itself by printing many of its own (plastic) parts to create more machines.<ref name="AutoSQ-20" /><ref name="AutoSQ-21" /> RepRaps have already been shown to be able to print [[circuit board]]s<ref name="AutoSQ-22" /> and metal parts.<ref name="AutoSQ-23" /><ref name="AutoSQ-24" /> |
|||
[[Photonic-crystal fiber]] is made with a regular pattern of index variation (often in the form of cylindrical holes that run along the length of the fiber). Such fiber uses [[diffraction]] effects instead of or in addition to total internal reflection, to confine light to the fiber's core. The properties of the fiber can be tailored to a wide variety of applications. |
|||
Because of the FOSH aims of [[RepRap Project|RepRap]], many related projects have used their design for inspiration, creating an ecosystem of related or derivative 3D printers, most of which are also open source designs. The availability of these open source designs means that variants of 3D printers are easy to invent. The quality and complexity of printer designs, however, as well as the quality of kit or finished products, varies greatly from project to project. This rapid development of open source 3D printers is gaining interest in many spheres as it enables hyper-customization and the use of [[public domain]] designs to fabricate [[open source appropriate technology]]. This technology can also assist initiatives in [[sustainable development]] since technologies are easily and economically made from resources available to local communities.<ref name="Auto3D-27" /><ref name="AutoSQ-25" /> |
|||
==Mechanisms of attenuation== |
|||
[[File:Zblan transmit.jpg|thumb|Light attenuation by [[ZBLAN]] and silica fibers]] |
|||
{{Main|Transparent materials}} |
|||
Attenuation in fiber optics, also known as transmission loss, is the reduction in intensity of the light beam (or signal) as it travels through the transmission medium. Attenuation coefficients in fiber optics usually use units of dB/km through the medium due to the relatively high quality of transparency of modern optical transmission media. The medium is usually a fiber of silica glass that confines the incident light beam to the inside. Attenuation is an important factor limiting the transmission of a digital signal across large distances. Thus, much research has gone into both limiting the attenuation and maximizing the amplification of the optical signal. |
|||
Empirical research has shown that attenuation in optical fiber is caused primarily by both [[scattering]] and [[absorption (electromagnetic radiation)|absorption]]. Single-mode optical fibers can be made with extremely low loss. Corning's SMF-28 fiber, a standard single-mode fiber for telecommunications wavelengths, has a loss of 0.17 dB/km at 1550 nm.<ref name="CorningSMF28ULL">{{cite web |url=http://www.corning.com/opticalfiber/products/SMF-28_ULL_fiber.aspx |title=Corning SMF-28 ULL optical fiber|accessdate=April 9, 2014}}</ref> For example, an 8 km length of SMF-28 transmits nearly 75% of light at 1,550 nm. It has been noted that if ocean water was as clear as fiber, one could see all the way to the bottom even of the Marianas Trench in the Pacific Ocean, a depth of 36,000 feet.<ref name="Jachetta2007">{{cite book |last= Williams |first=E. A. (ed.) |year=2007 |title=National Association of Broadcasters Engineering Handbook (Tenth Edition) |url= |location= |publisher=Taylor & Francis |pages=1667–1685 (Chapter 6.10 – Fiber–Optic Transmission Systems, Jim Jachetta)|isbn=978-0-240-80751-5}}</ref> |
|||
The cost of 3D printers has decreased dramatically since about 2010, with machines that used to cost $20,000 now costing less than $1,000.<ref name="AutoSQ-26" /> For instance, as of 2013, several companies and individuals are selling parts to build various [[RepRap Project|RepRap]] designs, with prices starting at about {{€|400}} / {{US$|500}}.<ref name="printerlist" /> The open source [[Fab@Home]] project<ref name="fabathome" /> has developed printers for general use with anything that can be squirted through a nozzle, from chocolate to silicone sealant and chemical reactants. Printers following the project's designs have been available from suppliers in kits or in pre-assembled form since 2012 at prices in the US$2000 range.<ref name="printerlist" /> The [[Kickstarter]] funded [[Peachy Printer]] is designed to cost $100<ref name="AutoSQ-27" /> and several other new 3D printers are aimed at the small, inexpensive market including the mUVe3D and Lumifold. [[Rapide 3D]] has designed a professional grade crowdsourced 3D-printer costing $1499 which has no fumes nor constant rattle during use.<ref name="AutoSQ-28" /> The [[3Doodler]], "3D printing pen", raised $2.3 million on Kickstarter with the pens selling at $99,<ref name="AutoSQ-29" /> though the 3D Doodler has been criticized for being more of a crafting pen than a 3D printer.<ref name="AutoSQ-30" /> |
|||
===Light scattering=== |
|||
[[File:Reflection angles.svg|thumb|Specular reflection]] |
|||
[[File:Diffuse reflection.PNG|thumb|Diffuse reflection]] |
|||
The propagation of light through the core of an optical fiber is based on total internal reflection of the lightwave. Rough and irregular surfaces, even at the molecular level, can cause light rays to be reflected in random directions. This is called [[diffuse reflection]] or [[light scattering|scattering]], and it is typically characterized by wide variety of reflection angles. |
|||
As the costs of 3D printers have come down they are becoming more appealing financially to use for self-manufacturing of personal products.<ref name="wittbrodt" /> In addition, 3D printing products at home may reduce the environmental impacts of manufacturing by reducing material use and distribution impacts.<ref name="AutoSQ-31" /> |
|||
[[Light scattering]] depends on the [[wavelength]] of the light being scattered. Thus, limits to spatial scales of visibility arise, depending on the frequency of the incident light-wave and the physical dimension (or spatial scale) of the scattering center, which is typically in the form of some specific micro-structural feature. Since [[visible spectrum|visible]] light has a wavelength of the order of one [[micrometre|micrometer]] (one millionth of a meter) scattering centers will have dimensions on a similar spatial scale. |
|||
In addition, several [[RecycleBot]]s such as the commercialized Filastruder have been designed and fabricated to convert waste plastic, such as shampoo containers and milk jugs, into inexpensive RepRap filament.<ref name="RPJ" /> There is some evidence that using this approach of distributed recycling is better for the environment.<ref name="AutoSQ-32" /> |
|||
Thus, attenuation results from the [[incoherent scatter]]ing of light at internal [[surface]]s and [[interface (chemistry)|interface]]s. In (poly)crystalline materials such as metals and ceramics, in addition to pores, most of the internal surfaces or interfaces are in the form of [[grain boundaries]] that separate tiny regions of crystalline order. It has recently been shown that when the size of the scattering center (or grain boundary) is reduced below the size of the wavelength of the light being scattered, the scattering no longer occurs to any significant extent. This phenomenon has given rise to the production of [[transparent ceramics|transparent ceramic materials]]. |
|||
The development and hyper-customization of the [[RepRap Project|RepRap]]-based 3D printers has produced a new category of printers suitable for small business and consumer use. Manufacturers such as [[Solidoodle]],<ref name="cfr2013" /> [[Robo 3D]], [[RepRap Project|RepRapPro]] and [[Pirx 3D]] have introduced models and kits priced at less than $1,000, thousands less than they were in September 2012.<ref name="cfr2013" /> Depending on the application, the print resolution and speed of manufacturing lies somewhere between a personal printer and an industrial printer. A list of printers with pricing and other information is maintained.<ref name="printerlist" /> Most recently [[delta robots]], like the [[TripodMaker]], have been utilized for 3D printing to increase fabrication speed further.<ref name="AutoQK-1" /> For delta 3D printers, due to its geometry and differentiation movements, the accuracy of the print depends on the position of the printer head.<ref name="AutoSQ-33" /> |
|||
Similarly, the scattering of light in optical quality glass fiber is caused by molecular level irregularities (compositional fluctuations) in the glass structure. Indeed, one emerging school of thought is that a glass is simply the limiting case of a polycrystalline solid. Within this framework, "domains" exhibiting various degrees of short-range order become the building blocks of both metals and alloys, as well as glasses and ceramics. Distributed both between and within these domains are micro-structural defects that provide the most ideal locations for light scattering. This same phenomenon is seen as one of the limiting factors in the transparency of IR missile domes.<ref>{{cite journal|author=Archibald, P.S. and Bennett, H.E.|title=Scattering from infrared missile domes|journal=Opt. Engr.|volume=17|page=647|year=1978|doi=10.1117/12.7972298|issue=6}}</ref> |
|||
Some companies are also offering software for 3D printing, as a support for hardware manufactured by other companies.<ref name="MatterHackers Opens 3D Printing Store and Releases MatterControl 0.7.6" /> |
|||
At high optical powers, scattering can also be caused by nonlinear optical processes in the fiber.<ref>{{cite journal|doi=10.1364/AO.11.002489|title=Optical Power Handling Capacity of Low Loss Optical Fibers as Determined by Stimulated Raman and Brillouin Scattering|year=1972|last1=Smith|first1=R. G.|journal=Applied Optics|volume=11|pages=2489–94|pmid=20119362|issue=11|bibcode=1972ApOpt..11.2489S}}</ref><ref>{{cite web |title=Brillouin Scattering |url=https://www.rp-photonics.com/brillouin_scattering.html |work=Encyclopedia of Laser Physics and Technology |publisher=RP Photonics |first=Rüdiger |last=Paschotta}}</ref> |
|||
=== |
=== Large 3D printers === |
||
Large 3D printers have been developed for industrial, education, and demonstrative uses. A large [[Delta robot|delta-style]] 3D printer was built in 2014 by SeeMeCNC. The printer is capable of making an object with diameter of up to {{Convert|4|ft}} and up to {{Convert|10|ft}} in height. It also uses plastic pellets as the raw material instead of the typical plastic filaments used in other 3D printers.<ref>{{cite news|title=Hoosier Daddy – The Largest Delta 3D Printer In the World|url=http://www.3dprinterworld.com/article/hoosier-daddy-largest-delta-3d-printer-world|accessdate=28 September 2014|work=3D Printer World|publisher=Punchbowl Media|date=23 July 2014}}</ref> |
|||
In addition to light scattering, attenuation or signal loss can also occur due to selective absorption of specific wavelengths, in a manner similar to that responsible for the appearance of color. Primary material considerations include both electrons and molecules as follows: |
|||
[[File:BigRep-One.jpg|thumb|The BigRep One.1 with its 1 m³ volume.]] |
|||
Another type of large printer is Big Area Additive Manufacturing (BAAM). The goal is to develop printers that can produce a large object in high speed. A BAAM machine of Cincinnati Incorporated can produce an object at the speeds 200-500 times faster than typical 3D printers available in 2014. Another BAAM machine is being developed by [[Lockheed Martin]] with an aim to print long objects of up to {{Convert|100|ft}} to be used in aerospace industries.<ref>{{cite news|last1=McKenna|first1=Beth|title=The Next Big Thing in 3-D Printing: Big Area Additive Manufacturing, or BAAM|url=http://www.fool.com/investing/general/2014/04/26/the-next-big-thing-in-3-d-printing-big-area-addi-2.aspx|accessdate=28 September 2014|work=The Motley Fool|date=26 April 2014}}</ref> |
|||
=== Microscale and nanoscale 3D printing === |
|||
* At the electronic level, it depends on whether the electron orbitals are spaced (or "quantized") such that they can absorb a quantum of light (or photon) of a specific wavelength or frequency in the ultraviolet (UV) or visible ranges. This is what gives rise to color. |
|||
Microelectronic device fabrication methods can be employed to perform the 3D printing of nanoscale-size objects. Such printed objects are typically grown on a solid substrate, e.g. silicon wafer, to which they adhere after printing as they're too small and fragile to be manipulated post-construction. |
|||
* At the atomic or molecular level, it depends on the frequencies of atomic or molecular vibrations or chemical bonds, how close-packed its atoms or molecules are, and whether or not the atoms or molecules exhibit long-range order. These factors will determine the capacity of the material transmitting longer wavelengths in the infrared (IR), far IR, radio and microwave ranges. |
|||
In one technique, 3D nanostructures can be printed by physically moving a dynamic stencil mask during the material deposition process, somewhat analogous to the extrusion method of traditional 3D printers. Programmable-height nanostructures with resolutions as small as 10 nm have been produced in this fashion, by metallic physical vapor deposition through a piezo-actuator controlled stencil mask having a milled nanopore in a silicon nitride membrane.<ref name=wasserman08>{{cite journal |author=J. L. Wasserman|date=2008 |title=Fabrication of One-Dimensional Programmable-Height Nanostructures via Dynamic Stencil Deposition |journal=[[Review of Scientific Instruments]] |volume=79 |issue=7 |pages=073909 |arxiv=0802.1848 |doi=10.1063/1.2960573|display-authors=etal}}</ref> |
|||
The design of any optically transparent device requires the selection of materials based upon knowledge of its properties and limitations. The [[crystal structure|Lattice]] [[absorption (electromagnetic radiation)|absorption]] characteristics observed at the lower frequency regions (mid IR to far-infrared wavelength range) define the long-wavelength transparency limit of the material. They are the result of the interactive [[coupling]] between the motions of thermally induced vibrations of the constituent [[atom]]s and molecules of the solid lattice and the incident light wave radiation. Hence, all materials are bounded by limiting regions of absorption caused by atomic and molecular vibrations (bond-stretching)in the far-infrared (>10 µm). |
|||
Another method enhances the photopolymerization process on a much smaller scale, using finely-focused lasers controlled by adjustable mirrors. This method has produced objects with feature resolutions of 100 nm.<ref>{{cite news|last1=patel|first1=Prachi|title=Micro 3-D Printer Creates Tiny Structures in Seconds|url=http://www.technologyreview.com/news/511856/micro-3-d-printer-creates-tiny-structures-in-seconds/|work=MIT Technology Review|date=5 March 2013}}</ref> |
|||
Thus, multi-phonon absorption occurs when two or more phonons simultaneously interact to produce electric dipole moments with which the incident radiation may couple. These dipoles can absorb energy from the incident radiation, reaching a maximum coupling with the radiation when the frequency is equal to the fundamental vibrational mode of the molecular dipole (e.g. Si-O bond) in the far-infrared, or one of its harmonics. |
|||
== Manufacturing applications == |
|||
The selective absorption of infrared (IR) light by a particular material occurs because the selected frequency of the light wave matches the frequency (or an integer multiple of the frequency) at which the particles of that material vibrate. Since different atoms and molecules have different natural frequencies of vibration, they will selectively absorb different frequencies (or portions of the spectrum) of infrared (IR) light. |
|||
{{quotation |
|||
| Three-dimensional printing makes it as cheap to create single items as it is to produce thousands and thus undermines [[economies of scale]]. It may have as profound an impact on the world as the coming of the factory did....Just as nobody could have predicted the impact of the [[History of the steam engine|steam engine in 1750]]—or the [[Global spread of the printing press|printing press in 1450]], or the [[History of the transistor|transistor in 1950]]—it is impossible to foresee the long-term impact of 3D printing. But the technology is coming, and it is likely to disrupt every field it touches. |
|||
| ''[[The Economist]]'', in a February 10, 2011 leader<ref name="Auto3D-18" /> |
|||
}} |
|||
AM technologies found applications starting in the 1980s in [[product development]], [[data visualization]], [[rapid prototyping]], and specialized manufacturing. Their expansion into production ([[job production]], [[mass production]], and [[distributed manufacturing]]) has been under development in the decades since. Industrial production roles within the [[metalworking]] industries<ref name="Zelinski_2014-06-25" /> achieved significant scale for the first time in the early 2010s. Since the start of the 21st century there has been a large growth in the sales of AM machines, and their price has dropped substantially.<ref name="Auto3D-2" /> According to Wohlers Associates, a consultancy, the market for 3D printers and services was worth $2.2 billion worldwide in 2012, up 29% from 2011.<ref name="AutoSQ-2" /> There are many applications for AM technologies, including architecture, construction (AEC), [[industrial design]], automotive, [[aerospace]],<ref>Development of a Three-Dimensional Printed, Liquid-Cooled Nozzle for a Hybrid Rocket Motor, Nick Quigley and James Evans Lyne, Journal of Propulsion and Power, Vol. 30, No. 6 (2014), pp. 1726–1727.</ref> military, [[engineering]], dental and medical industries, biotech (human tissue replacement), fashion, footwear, jewelry, eyewear, education, geographic information systems, food, and many other fields. |
|||
Reflection and transmission of light waves occur because the frequencies of the light waves do not match the natural resonant frequencies of vibration of the objects. When IR light of these frequencies strikes an object, the energy is either reflected or transmitted. |
|||
Additive manufacturing's earliest applications have been on the [[toolroom]] end of the manufacturing spectrum. For example, [[rapid prototyping]] was one of the earliest additive variants, and its mission was to reduce the [[lead time]] and cost of developing prototypes of new parts and devices, which was earlier only done with subtractive toolroom methods such as cnc milling and turning, and precision grinding, far more accurate than 3d printing with accuracy down to 0.00005" and creating better quality parts faster, but sometimes too expensive for low accuracy prototype parts.<ref name="TMW_2011-02_Origins" /> With technological advances in additive manufacturing, however, and the dissemination of those advances into the business world, additive methods are moving ever further into the production end of manufacturing in creative and sometimes unexpected ways.<ref name="TMW_2011-02_Origins" /> Parts that were formerly the sole province of subtractive methods can now in some cases be made more profitably via additive ones. In addition, new developments in [[RepRap]] technology allow the same device to perform both additive and subtractive manufacturing by swapping magnetic-mounted tool heads.<ref>G. Anzalone, B. Wijnen, Joshua M. Pearce, (2015) "[https://www.academia.edu/15352556/Multi-material_additive_and_subtractive_prosumer_digital_fabrication_with_a_free_and_open-source_convertible_delta_RepRap_3-D_printer Multi-material additive and subtractive prosumer digital fabrication with a free and open-source convertible delta RepRap 3-D printer]", ''Rapid Prototyping Journal'', 21(5), pp.506–519. doi:10.1108/RPJ-09-2014-0113</ref> |
|||
==Manufacturing== |
|||
=== Cloud-based additive manufacturing === |
|||
===Materials=== |
|||
{{Main|3D printing marketplace}} |
|||
Glass optical fibers are almost always made from [[silica]], but some other materials, such as [[fluoride glass|fluorozirconate]], [[fluoride glass|fluoroaluminate]], and [[chalcogenide glass]]es as well as crystalline materials like [[sapphire]], are used for longer-wavelength infrared or other specialized applications. Silica and fluoride glasses usually have refractive indices of about 1.5, but some materials such as the [[chalcogenide]]s can have indices as high as 3. Typically the index difference between core and cladding is less than one percent. |
|||
Additive manufacturing in combination with [[cloud computing]] technologies allows decentralized and geographically independent distributed production.<ref name="Bopp2010" /> Cloud-based additive manufacturing refers to a service-oriented networked manufacturing model in which service consumers are able to build parts through Infrastructure-as-a-Service (IaaS), Platform-as-a-Service (PaaS), Hardware-as-a-Service (HaaS), and Software-as-a-Service (SaaS).<ref>Wu, D., Thames, J.L., Rosen, D.W., & Schaefer, D. (2013). Enhancing the Product Realization Process with Cloud-Based Design and Manufacturing Systems. Transactions of the ASME, Journal of Computing and Information Science in Engineering (JCISE), 13(4): 041004-041004-14. doi:10.1115/1.4025257</ref><ref>Wu, D., Rosen, D.W., Wang, L., & Schaefer, D. (2015). Cloud-Based Design and Manufacturing: A New Paradigm in Digital Manufacturing and Design Innovation. Computer-Aided Design, 59(1): 1-14, DOI: 10.1016/j.cad.2014.07.006.</ref><ref>Wu, D., Rosen, D.W., & Schaefer, D. (2015). Scalability Planning for Cloud-Based Manufacturing Systems. Transactions of the ASME, Journal of Manufacturing Science and Engineering, DOI:10.1115/1.4030266.</ref> [[Distributed manufacturing]] as such is carried out by some enterprises; there is also a services like [[3D Hubs]] that put people needing 3D printing in contact with owners of printers.<ref name="AutoSQ-119" /> |
|||
Some companies offer on-line 3D printing services to both commercial and private customers,<ref name="Auto3D-28" /> working from 3D designs uploaded to the company website. 3D-printed designs are either shipped to the customer or picked up from the service provider.<ref name="Auto3D-29" /> |
|||
[[Plastic optical fiber]]s (POF) are commonly step-index multi-mode fibers with a core diameter of 0.5 millimeters or larger. POF typically have higher attenuation coefficients than glass fibers, 1 dB/m or higher, and this high attenuation limits the range of POF-based systems. |
|||
=== |
=== Mass customization === |
||
[[File:Miniature human face models made through 3D Printing (Rapid Prototyping).jpg|thumb|Miniature face models (from FaceGen) produced using several colored plastics on a 3D Printer]] |
|||
[[Silica]] exhibits fairly good optical transmission over a wide range of wavelengths. In the [[near-infrared]] (near IR) portion of the spectrum, particularly around 1.5 μm, silica can have extremely low absorption and scattering losses of the order of 0.2 dB/km. Such remarkably low losses are possible only because ultra-pure silicon is available, it being essential for manufacturing integrated circuits and discrete transistors. A high transparency in the 1.4-μm region is achieved by maintaining a low concentration of [[hydroxyl group]]s (OH). Alternatively, a high OH [[concentration]] is better for transmission in the [[ultraviolet]] (UV) region.<ref>{{Cite journal | last1 = Skuja | first1 = L. | last2 = Hirano | first2 = M. | last3 = Hosono | first3 = H. | last4 = Kajihara | first4 = K. | title = Defects in oxide glasses | doi = 10.1002/pssc.200460102 | journal = Physica status solidi (c) | volume = 2 | pages = 15 | year = 2005 | pmid = | pmc = }}</ref> |
|||
Companies have created services where consumers can customize objects using simplified web based customisation software, and order the resulting items as 3D printed unique objects.<ref name="AutoQK-2" /><ref name="AutoQK-3" /> This now allows consumers to create custom cases for their mobile phones.<ref name="AutoSQ-34" /> Nokia has released the 3D designs for its case so that owners can customize their own case and have it 3D printed.<ref name="AutoSQ-35" /> |
|||
=== Rapid manufacturing === |
|||
Silica can be drawn into fibers at reasonably high temperatures, and has a fairly broad [[glass transformation range]]. One other advantage is that fusion splicing and cleaving of silica fibers is relatively effective. Silica fiber also has high mechanical strength against both pulling and even bending, provided that the fiber is not too thick and that the surfaces have been well prepared during processing. Even simple cleaving (breaking) of the ends of the fiber can provide nicely flat surfaces with acceptable optical quality. Silica is also relatively [[chemically inert]]. In particular, it is not [[Hygroscopy|hygroscopic]] (does not absorb water). |
|||
Advances in RP technology have introduced materials that are appropriate for final manufacture, which has in turn introduced the possibility of directly manufacturing finished components. One advantage of 3D printing for rapid manufacturing lies in the relatively inexpensive production of small numbers of parts. |
|||
Rapid manufacturing is a new method of manufacturing and many of its processes remain unproven. 3D printing is now entering the field of rapid manufacturing and was identified as a "next level" technology by many experts in a 2009 report.<ref name="Auto3D-19" /> One of the most promising processes looks to be the adaptation of [[selective laser sintering]] (SLS), or [[direct metal laser sintering]] (DMLS) some of the better-established rapid prototyping methods. {{As of|2006}}, however, these techniques were still very much in their infancy, with many obstacles to be overcome before RM could be considered a realistic manufacturing method.<ref name="Auto3D-20" /> |
|||
Silica glass can be doped with various materials. One purpose of doping is to raise the [[refractive index]] (e.g. with [[germanium dioxide]] (GeO<sub>2</sub>) or [[aluminium oxide]] (Al<sub>2</sub>O<sub>3</sub>)) or to lower it (e.g. with [[fluorine]] or [[boron trioxide]] (B<sub>2</sub>O<sub>3</sub>)). Doping is also possible with laser-active ions (for example, [[rare earth element|rare earth]]-doped fibers) in order to obtain active fibers to be used, for example, in fiber amplifiers or [[laser]] applications. Both the fiber core and cladding are typically doped, so that the entire assembly (core and cladding) is effectively the same compound (e.g. an [[aluminosilicate]], germanosilicate, phosphosilicate or [[borosilicate glass]]). |
|||
,l,/. |
|||
=== Rapid prototyping === |
|||
Particularly for active fibers, pure silica is usually not a very suitable host glass, because it exhibits a low solubility for rare earth ions. This can lead to quenching effects due to clustering of dopant ions. Aluminosilicates are much more effective in this respect. |
|||
{{Main|rapid prototyping}} |
|||
Industrial 3D printers have existed since the early 1980s and have been used extensively for rapid prototyping and research purposes. These are generally larger machines that use proprietary powdered metals, casting media (e.g. sand), plastics, paper or cartridges, and are used for [[rapid prototyping]] by universities and commercial companies. |
|||
=== Research === |
|||
Silica fiber also exhibits a high threshold for optical damage. This property ensures a low tendency for laser-induced breakdown. This is important for fiber amplifiers when utilized for the amplification of short pulses. |
|||
3D printing can be particularly useful in research labs due to its ability to make specialized, bespoke geometries. In 2012 a [[proof of principle]] project at the [[University of Glasgow]], UK, showed that it is possible to use 3D printing techniques to assist in the production of [[chemical compound]]s. They first printed chemical [[reaction vessel]]s, then used the printer to deposit [[reactant]]s into them.<ref name="chem" /> They have produced new compounds to verify the validity of the process, but have not pursued anything with a particular application.<ref name="chem" /> |
|||
=== Food === |
|||
Because of these properties silica fibers are the material of choice in many optical applications, such as communications (except for very short distances with plastic optical fiber), fiber lasers, fiber amplifiers, and fiber-optic sensors. Large efforts put forth in the development of various types of silica fibers have further increased the performance of such fibers over other materials.<ref>{{cite journal|url=http://www.corning.com/WorkArea/downloadasset.aspx?id=7783|author=Glaesemann, G. S.|bibcode=1999SPIE.CR73....3G|title=Advancements in Mechanical Strength and Reliability of Optical Fibers|journal=Proc. SPIE|volume=CR73|page=1|year=1999}}</ref><ref name=Kurkjian>{{cite journal |doi=10.1111/j.1151-2916.1993.tb03727.x |title=Strength, Degradation, and Coating of Silica Lightguides |year=1993 |last1=Kurkjian |first1=Charles R. |last2=Simpkins |first2=Peter G. |last3=Inniss |first3=Daryl |journal=Journal of the American Ceramic Society |volume=76 |pages=1106–1112 |issue=5}}</ref><ref>{{cite journal|doi=10.1016/0022-3093(88)90114-7|title=Mechanical stability of oxide glasses|year=1988|last1=Kurkjian|first1=C|journal=Journal of Non-Crystalline Solids|volume=102|pages=71–81|bibcode = 1988JNCS..102...71K }}</ref><ref>{{cite journal|doi=10.1109/50.50715|title=Strength and fatigue of silica optical fibers|year=1989|last1=Kurkjian|first1=C.R.|last2=Krause|first2=J.T.|last3=Matthewson|first3=M.J.|journal=Journal of Lightwave Technology|volume=7|pages=1360–1370|issue=9|bibcode = 1989JLwT....7.1360K }}</ref><ref>{{cite journal|doi=10.1117/12.372757|journal=Proceedings of SPIE|year=1999|last1=Kurkjian|first1=Charles R.|page=77|volume=3848|title=Strength variations in silica fibers|series=Optical Fiber Reliability and Testing|editor1-last=Matthewson|editor1-first=M. John|last2=Gebizlioglu|first2=Osman S.|last3=Camlibel|first3=Irfan}}</ref><ref>{{cite journal|doi=10.1117/12.396408|journal=Proceedings of SPIE|year=2000|last1=Skontorp|first1=Arne|page=278|volume=4073|title=Nonlinear mechanical properties of silica-based optical fibers|series=Fifth European Conference on Smart Structures and Materials|editor1-last=Gobin|editor1-first=Pierre F|editor2-last=Friend|editor2-first=Clifford M}}</ref><ref>{{cite journal|doi=10.1098/rspa.1967.0085|title=The Strength of Fused Silica|year=1967|last1=Proctor|first1=B. A.|last2=Whitney|first2=I.|last3=Johnson|first3=J. W.|journal=[[Proceedings of the Royal Society A]]|volume=297|pages=534–557|issue=1451|bibcode = 1967RSPSA.297..534P }}</ref><ref>{{cite journal|doi=10.1016/0022-3093(68)90007-0|title=The structure and strength of glass fibers|year=1968|last1=Bartenev|first1=G|journal=Journal of Non-Crystalline Solids|volume=1|pages=69–90|bibcode = 1968JNCS....1...69B }}</ref> |
|||
Cornell Creative Machines Lab announced in 2012 that it was possible to produce customized food with 3D Hydrocolloid Printing.<ref name="Auto3D-41" /> Additive manufacturing of food is currently being developed by squeezing out food, layer by layer, into three-dimensional objects. A large variety of foods are appropriate candidates, such as chocolate and candy, and flat foods such as crackers, pasta,<ref name="AutoSQ-72" /> and pizza.<ref>{{cite web|title=Foodini 3D Printer Cooks Up Meals Like the Star Trek Food Replicator|url=http://inhabitat.com/foodini-3d-printer-will-make-all-your-meals-for-you-like-the-star-trek-food-replicator|accessdate=27 January 2015}}</ref> NASA has considered the versatility of the concept, awarding a contract to the Systems and Materials Research Consultancy to study the feasibility of printing food in space.<ref>{{cite web|title=3D Printing: Food in Space|url=http://www.nasa.gov/directorates/spacetech/home/feature_3d_food_prt.htm|publisher=NASA|accessdate=2015-09-30}}</ref> One of the problem with food printing is the nature of the texture of a food. For example, foods that are not strong enough to be filed are not appropriate for 3D printing for now. |
|||
=== Medical applications === |
|||
====Fluoride glass==== |
|||
Professor [[Leroy Cronin]] of [[Glasgow University]] proposed in a 2012 [[TED Talk]] that it was possible to use chemical inks to print medicine.<ref name="AutoSQ-71" /> Similarly, 3D printing has been considered as a method of implanting [[Gene therapy|stem cells]] capable of generating new tissues and organs in living humans.<ref>{{cite web|title=RFA-HD-15-023: Use of 3-D Printers for the Production of Medical Devices (R43/R44)|url=http://grants.nih.gov/grants/guide/rfa-files/RFA-HD-15-023.html|publisher=NIH grants|accessdate=2015-09-30}}</ref> |
|||
[[Fluoride glass]] is a class of non-oxide optical quality glasses composed of [[fluoride]]s of various [[metal]]s. Because of their low [[viscosity]], it is very difficult to completely avoid [[crystallization]] while processing it through the glass transition (or drawing the fiber from the melt). Thus, although [[heavy metal (chemistry)|heavy metal]] fluoride glasses (HMFG) exhibit very low optical attenuation, they are not only difficult to manufacture, but are quite fragile, and have poor resistance to moisture and other environmental attacks. Their best attribute is that they lack the absorption band associated with the [[hydroxyl]] (OH) group (3,200–3,600 cm<sup>−1</sup>; i.e., 2,777–3,125 nm or 2.78–3.13 μm), which is present in nearly all oxide-based glasses. |
|||
== Industrial applications == |
|||
An example of a heavy metal fluoride glass is the [[ZBLAN]] glass group, composed of [[zirconium]], [[barium]], [[lanthanum]], [[aluminium]], and [[sodium]] fluorides. Their main technological application is as [[optical waveguide]]s in both planar and fiber form. They are advantageous especially in the [[mid-infrared]] (2,000–5,000 nm) range. |
|||
=== Apparel === |
|||
HMFGs were initially slated for optical fiber applications, because the intrinsic losses of a mid-IR fiber could in principle be lower than those of silica fibers, which are transparent only up to about 2 μm. However, such low losses were never realized in practice, and the fragility and high cost of fluoride fibers made them less than ideal as primary candidates. Later, the utility of fluoride fibers for various other applications was discovered. These include mid-[[IR spectroscopy]], [[fiber optic sensor]]s, [[thermometry]], and [[optical imaging|imaging]]. Also, fluoride fibers can be used for guided lightwave transmission in media such as YAG ([[yttrium aluminium garnet]]) [[laser]]s at 2.9 μm, as required for medical applications (e.g. [[ophthalmology]] and [[dentistry]]).<ref>{{cite journal|doi=10.1109/JLT.1984.1073661|author=Tran, D.|title=Heavy metal fluoride glasses and fibers: A review|journal=J. Lightwave Technology|volume=2|pages=566–586|year=1984|issue=5|bibcode = 1984JLwT....2..566T |display-authors=etal}}</ref><ref>{{cite journal|doi=10.1117/12.405276|journal=Proceedings of SPIE|year=2000|last1=Nee|first1=Soe-Mie F.|page=122|volume=4102|title=Optical and surface properties of oxyfluoride glass|series=Inorganic Optical Materials II|editor1-last=Marker Iii|editor1-first=Alexander J|last2=Johnson|first2=Linda F.|last3=Moran|first3=Mark B.|last4=Pentony|first4=Joni M.|last5=Daigneault|first5=Steven M.|last6=Tran|first6=Danh C.|last7=Billman|first7=Kenneth W.|last8=Siahatgar|first8=Sadegh|editor2-last=Arthurs|editor2-first=Eugene G }}</ref> |
|||
3D printing has entered the world of clothing with fashion designers experimenting with 3D-printed bikinis, shoes, and dresses.<ref name="resins-online.com" /> In commercial production Nike is using 3D printing to prototype and manufacture the 2012 Vapor Laser Talon football shoe for players of American football, and New Balance is 3D manufacturing custom-fit shoes for athletes.<ref name="resins-online.com" /><ref name="AutoSQ-45" /> |
|||
3D printing has come to the point where companies are printing consumer grade eyewear with on-demand custom fit and styling (although they cannot print the lenses). On-demand customization of glasses is possible with rapid prototyping.<ref name="Forbes.com" /> |
|||
====Phosphate glass==== |
|||
[[File:Phosphorus-pentoxide-3D-balls.png|thumb|The P<sub>4</sub>O<sub>10</sub> cagelike structure—the basic building block for phosphate glass.]] |
|||
=== Vehicle === |
|||
[[Phosphate glass]] constitutes a class of optical glasses composed of [[metaphosphate]]s of various metals. Instead of the SiO<sub>4</sub> <!-- I don't think there is an SiO4 --> [[tetrahedra]] observed in silicate glasses, the building block for this glass former is [[phosphorus pentoxide]] (P<sub>2</sub>O<sub>5</sub>), which crystallizes in at least four different forms. The most familiar [[polymorphism (materials science)|polymorph]] (see figure) comprises molecules of P<sub>4</sub>O<sub>10</sub>. |
|||
[[File:I robot car.jpg|thumb|The [[Audi RSQ]] was made with rapid prototyping industrial [[KUKA]] robots]] |
|||
In early 2014, the Swedish [[supercar]] manufacturer, [[Koenigsegg]], announced the One:1, a supercar that utilizes many components that were 3D printed. In the limited run of vehicles Koenigsegg produces, the One:1 has side-mirror internals, air ducts, titanium exhaust components, and even complete turbocharger assemblies that have been 3D printed as part of the manufacturing process.<ref name="AutoSQ-36" /> |
|||
[[Urbee]] is the name of the first car in the world car mounted using the technology 3D printing (his bodywork and his car windows were "printed"). Created in 2010 through the partnership between the US engineering group [[Kor Ecologic]] and the company [[Stratasys]] (manufacturer of printers Stratasys 3D), it is a hybrid vehicle with futuristic look.<ref>[http://www.tecmundo.com.br/impressora/6260-conheca-o-urbee-primeiro-carro-a-ser-fabricado-com-uma-impressora-3d.htm tecmundo.com.br/ ''Conheça o Urbee, primeiro carro a ser fabricado com uma impressora 3D'']</ref><ref>[http://truth-out.org/news/item/27430-the-urbee-3d-printed-car-coast-to-coast-on-10-gallons The "Urbee" 3D-Printed Car: Coast to Coast on 10 Gallons] [[Truthout]]</ref><ref>[https://www.youtube.com/watch?v=vI12MqoYQto 3D Printed Car Creator Discusses Future of the Urbee]</ref> |
|||
Phosphate glasses can be advantageous over silica glasses for optical fibers with a high concentration of doping rare earth ions. A mix of fluoride glass and phosphate glass is fluorophosphate glass.<ref>{{cite journal|doi=10.1016/S0022-3093(01)00615-9|title=Mechanical and structural properties of phosphate glasses|year=2001|last1=Karabulut|first1=M|journal=Journal of Non-Crystalline Solids|volume=288|pages=8–17|bibcode=2001JNCS..288....8K|last2=Melnik|first2=E|last3=Stefan|first3=R|last4=Marasinghe|first4=G.K|last5=Ray|first5=C.S|last6=Kurkjian|first6=C.R|last7=Day|first7=D.E}}</ref><ref>{{cite journal|doi=10.1016/S0022-3093(99)00637-7|title=Mechanical properties of phosphate glasses|year=2000|last1=Kurkjian|first1=C|journal=Journal of Non-Crystalline Solids|volume=263–264|pages=207–212|bibcode=2000JNCS..263..207K}}</ref> |
|||
In May 2015 Airbus announced that its new [[Airbus A350 XWB]] included over 1000 components manufactured by 3D printing.<ref>{{cite web|title=Airbus had 1,000 parts 3D printed to meet deadline|url=http://www.bbc.com/news/technology-32597809|accessdate=2015-11-27|date=May 6, 2015|first=Dan|last=Simmons|publisher=BBC}}</ref> |
|||
====Chalcogenide glass==== |
|||
The [[chalcogen]]s—the elements in [[group (periodic table)|group 16]] of the [[periodic table]]—particularly [[sulfur]] (S), [[selenium]] (Se) and [[tellurium]] (Te)—react with more [[electropositive]] elements, such as [[silver]], to form [[chalcogenides]]. These are extremely versatile compounds, in that they can be crystalline or amorphous, metallic or semiconducting, and conductors of [[ion]]s or [[electron]]s. [[Chalcogenide glass|Glass containing chalcogenides]] can be used to make fibers for far infrared transmission.{{citation needed|date=April 2014}} |
|||
3D printing is also being utilized by air forces to print spare parts for planes. In 2015, a [[Royal Air Force]] [[Eurofighter Typhoon]] fighter jet flew with printed parts. The [[United States Air Force]] has begun to work with 3D printers, and the [[Israeli Air Force]] has also purchased a 3D printer to print spare parts.<ref>{{cite web|title=The 3D printer revolution comes to the IAF|url=http://www.ynetnews.com/articles/0,7340,L-4684682,00.html|accessdate=2015-09-29|date=July 27, 2015|first=Yoav|last=Zitun|publisher=Ynet News}}</ref> |
|||
===Process=== |
|||
=== |
=== Construction === |
||
{{Main|Building printing}} |
|||
[[File:OF-MCVD.svg|thumb|300px|Illustration of the modified chemical vapor deposition (inside) process]] |
|||
Until recent years models were built by hand, often taking a long time. Thus, architects are often forced to show their clients drawings of their projects. According to Erik Kinipper, clients usually need to see the product from all possible viewpoints in space to get a clearer picture of the design and make an informed decision. In order to get these scale models to clients in a small amount of time, architects and architecture firms tend to rely on 3D printing.<ref>{{cite web|title=Architecture {{!}} Leapfrog 3D Printers|url=http://www.lpfrg.com/applications/3d-printing-for-architects|publisher=www.lpfrg.com|accessdate=2015-09-29|last=Knippers|first=E.}}</ref> Using 3D printing, these firms can reduce lead times of production by 50 to 80 percent, producing scale models up to 60 percent lighter than the machined part while being sturdy.<ref>{{cite web|title=Concept Modeling, Realistic 3D Printed Models {{!}} Stratasys|url=http://www.stratasys.com/solutions-applications/prototyping/concept-modeling|publisher=www.stratasys.com|accessdate=2015-09-29}}</ref> Thus, the designs and the models are only limited by a person's imagination. |
|||
Standard optical fibers are made by first constructing a large-diameter "preform" with a carefully controlled refractive index profile, and then "pulling" the preform to form the long, thin optical fiber. The preform is commonly made by three [[chemical vapor deposition]] methods: ''inside vapor deposition'', ''outside vapor deposition'', and ''vapor axial deposition''.<ref name=gowar1993>{{cite book|title=Optical communication systems |
|||
|last= Gowar |
|||
|first= John |
|||
|edition= 2d |
|||
|publisher= Prentice-Hall |
|||
|location= Hempstead, UK |
|||
|year= 1993 |
|||
|isbn= 0-13-638727-6 |
|||
|page= 209}}</ref> |
|||
The improvements on accuracy, speed and quality of materials in 3D printing technology have opened new doors for it to move beyond the use of 3D printing in the modeling process and actually move it to manufacturing strategy. A good example is Dr. Behrokh Khoshnevis’ research at the University of Southern California which resulted in a 3D printer that can build a house in 24 hours .The process is called Contour Crafting. Khoshnevis, Russell, Kwon, & Bukkapatnam, define contour crafting as an additive manufacturing process which uses computer controlled systems to repeatedly lay down layers of materials such as concrete. Bushey also discussed Khoshnevis's robot which comes equipped with a nozzle that spews out concrete and can build a home based on a set computer pattern. Contour Crafting technology has great potential for automating the construction of whole structures as well as sub-components. Using this process, a single house or a colony of houses, each with possibly a different design, may be automatically constructed in a single run, embedded in each house all the conduits for electrical, plumbing and air-conditioning.<ref>{{cite journal|title=Crafting large prototypes|url=http://ieeexplore.ieee.org/stamp/stamp.jsp?tp=&arnumber=956812|journal=IEEE Robotics Automation Magazine|date=2001-09-01|issn=1070-9932|pages=33–42|volume=8|issue=3|doi=10.1109/100.956812|first=B.|last=Khoshnevis|first2=R.|last2=Russell|first3=Hongkyu|last3=Kwon|first4=S.|last4=Bukkapatnam}}</ref> |
|||
With ''inside vapor deposition'', the preform starts as a hollow glass tube approximately {{convert|40|cm|in|sp=us}} long, which is placed horizontally and rotated slowly on a [[lathe]]. Gases such as [[silicon tetrachloride]] (SiCl<sub>4</sub>) or [[germanium tetrachloride]] (GeCl<sub>4</sub>) are injected with [[oxygen]] in the end of the tube. The gases are then heated by means of an external hydrogen burner, bringing the temperature of the gas up to 1,900 [[Kelvin|K]] (1,600 °C, 3,000 °F), where the tetrachlorides react with oxygen to produce [[silica]] or [[germanium dioxide|germania]] (germanium dioxide) particles. When the reaction conditions are chosen to allow this reaction to occur in the gas phase throughout the tube volume, in contrast to earlier techniques where the reaction occurred only on the glass surface, this technique is called ''[[modified chemical vapor deposition]] (MCVD)''. |
|||
=== Firearms === |
|||
The oxide particles then agglomerate to form large particle chains, which subsequently deposit on the walls of the tube as soot. The deposition is due to the large difference in temperature between the gas core and the wall causing the gas to push the particles outwards (this is known as [[thermophoresis]]). The torch is then traversed up and down the length of the tube to deposit the material evenly. After the torch has reached the end of the tube, it is then brought back to the beginning of the tube and the deposited particles are then melted to form a solid layer. This process is repeated until a sufficient amount of material has been deposited. For each layer the composition can be modified by varying the gas composition, resulting in precise control of the finished fiber's optical properties. |
|||
{{Main|3D printed firearms}} |
|||
In 2012, the US-based group [[Defense Distributed]] disclosed plans to "[design] a working plastic [[gun]] that could be downloaded and reproduced by anybody with a 3D printer."<ref name="f20120823" /><ref name="pcm20120824" /> Defense Distributed has also designed a 3D printable AR-15 type rifle [[lower receiver]] (capable of lasting more than 650 rounds) and a 30 round M16 magazine The AR-15 has multiple receivers (both an upper and lower receiver), but the legally controlled part is the one that is serialized (the lower, in the AR-15's case). Soon after Defense Distributed succeeded in designing the first working blueprint to produce a plastic gun with a 3D printer in May 2013, the [[United States Department of State]] demanded that they remove the instructions from their website.<ref name="AutoSQ-53" /> After Defense Distributed released their plans, questions were raised regarding the effects that 3D printing and widespread consumer-level [[CNC]] machining<ref name="AutoSQ-54" /><ref name="AutoSQ-55" /> may have on [[gun control]] effectiveness.<ref name="AutoSQ-56" /><ref name="AutoSQ-57" /><ref name="AutoSQ-58" /><ref name="AutoSQ-59" /> |
|||
In 2014, a man from Japan became the first person in the world to be imprisoned for making 3D printed firearms.<ref name=":0">{{cite news|url=http://www.theverge.com/2014/10/20/7022809/3d-printed-gun-maker-in-japan-sentenced-2-years|title=3D-printed gun maker in Japan sentenced to two years in prison|last=Franzen|first=Carl|work=The Verge}}</ref> Yoshitomo Imura posted videos and blueprints of the gun online and was sentenced to jail for two years. Police found at least two guns in his household that were capable of firing bullets.<ref name=":0" /> |
|||
In outside vapor deposition or vapor axial deposition, the glass is formed by ''flame hydrolysis'', a reaction in which silicon tetrachloride and germanium tetrachloride are oxidized by reaction with water (H<sub>2</sub>O) in an [[oxyhydrogen]] flame. In outside vapor deposition the glass is deposited onto a solid rod, which is removed before further processing. In vapor axial deposition, a short ''seed rod'' is used, and a porous preform, whose length is not limited by the size of the source rod, is built up on its end. The porous preform is consolidated into a transparent, solid preform by heating to about 1,800 K (1,500 °C, 2,800 °F). |
|||
=== Medical === |
|||
[[File:DShaped1.png|right|120px|right|thumb|Cross-section of a fiber drawn from a D-shaped '''preform''']] |
|||
{{Update|section|date=February 2015}} |
|||
Typical communications fiber uses a circular preform. For some applications such as [[double-clad fiber]]s another form is preferred.<ref name="Kouznetsov">{{cite journal|title=Highly efficient, high-gain, short-length, and power-scalable incoherent diode slab-pumped fiber amplifier/laser| author= Kouznetsov, D.|author2=Moloney, J.V.| journal=[[IEEE Journal of Quantum Electronics]]| volume=39 | year=2003 | issue=11 | pages=1452–1461 | doi=10.1109/JQE.2003.818311|bibcode = 2003IJQE...39.1452K }}</ref> In [[fiber laser]]s based on double-clad fiber, an asymmetric shape improves the [[filling factor]] for [[laser pumping]]. |
|||
[[File:3D Printed Macrognathism.jpg|thumbnail|3D printed human skull from computed computer tomography data.]] |
|||
Because of the surface tension, the shape is smoothed during the drawing process, and the shape of the resulting fiber does not reproduce the sharp edges of the preform. Nevertheless, careful polishing of the preform is important, since any defects of the preform surface affect the optical and mechanical properties of the resulting fiber. In particular, the preform for the test-fiber shown in the figure was not polished well, and cracks are seen with the confocal [[optical microscope]]. |
|||
3D printing has been used to print patient specific implant and device for medical use. Successful operations include a titanium [[pelvis]] implanted into a British patient, titanium lower [[jaw]] transplanted to a Belgian patient,<ref name="AutoSQ-47" /> and a plastic [[vertebrate trachea|tracheal]] splint for an American infant.<ref name="AutoSQ-48" /> The hearing aid and dental industries are expected to be the biggest area of future development using the custom 3D printing technology.<ref name="AutoSQ-49" /> In March 2014, surgeons in Swansea used 3D printed parts to rebuild the face of a motorcyclist who had been seriously injured in a road accident.<ref name="AutoSQ-50" /> Research is also being conducted on methods to bio-print replacements for lost tissue due to arthritis and cancer {{citation needed|date=November 2015}}. |
|||
====Drawing==== |
|||
The preform, however constructed, is placed in a device known as a [[drawing tower]], where the preform tip is heated and the optical fiber is pulled out as a string. By measuring the resultant fiber width, the tension on the fiber can be controlled to maintain the fiber thickness. |
|||
The things we are printing are becoming more personal and intimate overtime. This is especially true even in medicine: increasingly, what we are printing is ourselves. 3D printing is a great technological advancement in the medical field. 3D printing technology can now be used to make exact replicas of organs. Doctors can use these to plan out surgeries a lot more accurately. While, medical students may even be able to perform practice operations. The printer uses images from patients' MRI or CT scan images as a template and lays down layers of rubber or plastic. These types of procedures are becoming more and more common among doctors and medical researchers everywhere. Many have begun to use 3D printing in order to accurately undergo surgical procedures. This helps that may have been considered inoperable before have the opportunity to have a successful surgery. The hope is that these printers will eventually be able to produce actual organs that can be transplanted into patients by replacing the rubber and plastic printer "ink" with human cells. "The printer and software usually cost in the range of $100,000." Which happens to be less than a CT scan or MRI setup. Rader said "He predicts that interest in the technology will continue to grow as research shows how using simulated organs leads to better surgical outcomes and shorter operating times." This will also help the patients feel at ease as they can be provided with a live model to better explain surgical procedures and put the patient at ease.<ref name="AutoSQ-51" /> |
|||
===Coatings=== |
|||
The light is guided down the core of the fiber by an optical cladding with a lower refractive index that traps light in the core through total internal reflection. |
|||
==== Medical devices ==== |
|||
The cladding is coated by a buffer that protects it from moisture and physical damage.<ref name=Kurkjian/> The buffer coating is what gets stripped off the fiber for termination or splicing. These coatings are UV-cured urethane acrylate composite or polyimide materials applied to the outside of the fiber during the drawing process. The coatings protect the very delicate strands of glass fiber—about the size of a human hair—and allow it to survive the rigors of manufacturing, proof testing, cabling and installation. |
|||
In October 24, 2014, a five-year-old girl born without fully formed fingers on her left hand became the first child in the UK to have a prosthetic hand made with 3D printing technology. Her hand was designed by US-based E-nable, an [[Open design#Open-design organizations|open source design organisation]] which uses a network of volunteers to design and make prosthetics mainly for children. The prosthetic hand was based on a plaster cast made by her parents.<ref>BBC News (October 2014). [http://www.bbc.co.uk/news/uk-scotland-highlands-islands-29441115 "Inverness girl Hayley Fraser gets 3D-printed hand"], BBC News, 01 October 2014. Retrieved 02 October 2014.</ref> A boy named Alex was also born with a missing arm from just above the elbow. The team was able to use 3D printing to upload an e-NABLE Myoelectric arm that runs off of servos and batteries that are actuated by the electromyography muscle. With the use of 3D printers, E-NABLE has so far distributed more than 400 plastic hands to children. |
|||
Printed [[prosthetic]]s have been used in rehabilitation of crippled animals. In 2013, a 3D printed foot let a crippled duckling walk again.<ref name="AutoSQ-42" /> In 2014 a chihuahua born without front legs was fitted with a harness and wheels created with a 3D printer.<ref name="Help Animals">{{cite web |url=http://www.dailymail.co.uk/news/article-2727947/Puppy-power-Chihuahua-born-without-legs-given-turbo-charged-makeover-fitted-3D-printed-body-harness-set-skateboard-wheels.html |title=Puppy power: Chihuahua born without front legs is given turbo-charged makeover after being fitted with 3D printed body harness and a set of skateboard wheels |work=The Daily Mail |date=18 August 2014 |first=Chris |last=Pleasance |accessdate=2014-08-21}}</ref> 3D printed hermit crab shells let [[hermit crab]]s inhabit a new style home.<ref name="AutoSQ-43" /> A prosthetic beak was another tool developed by the use of 3D printing to help aid a bald eagle named Beauty, whose beak was severely mutilated from a shot in the face. Since 2014, commercially available titanium knee implants made with 3D printer for dogs have been used to restore the animal mobility. Over 10,000 dogs in Europe and United States have been treated after only one year.<ref>{{cite web |url=http://www.fierceanimalhealth.com/story/3d-systems-preps-global-launch-printed-knee-implants-dogs/2015-03-20 |title=3D Systems preps for global launch of 'printed' knee implants for dogs |author=Weintraub, Arlene |publisher={{url|fierceanimalhealth.com}} |date=20 March 2015 |accessdate=13 April 2015}}</ref> |
|||
Today’s glass optical fiber draw processes employ a dual-layer coating approach. An inner primary coating is designed to act as a shock absorber to minimize attenuation caused by microbending. An outer secondary coating protects the primary coating against mechanical damage and acts as a barrier to lateral forces. Sometimes a metallic armor layer is added to provide extra protection. |
|||
In February 2015, FDA approved the marketing of a surgical bolt which facilitates less-invasive foot surgery and eliminates the need to drill through bone. The 3D printed titanium device, 'FastForward Bone Tether Plate' is approved to use in correction surgery to treat [[bunion]].<ref>{{cite web |url=http://www.fiercemedicaldevices.com/story/fda-clears-3-d-printed-device-minimally-invasive-foot-surgery/2015-02-02 |title=FDA clears 3-D printed device for minimally invasive foot surgery |author=Saxena, Varun |publisher={{url|fiercemedicaldevices.com}} |date=2 February 2015 |accessdate=14 April 2015}}</ref> |
|||
These fiber optic coating layers are applied during the fiber draw, at speeds approaching {{convert|100|km/h|mph|-1|sp=us}}. Fiber optic coatings are applied using one of two methods: ''wet-on-dry'' and ''wet-on-wet''. In wet-on-dry, the fiber passes through a primary coating application, which is then UV cured—then through the secondary coating application, which is subsequently cured. In wet-on-wet, the fiber passes through both the primary and secondary coating applications, then goes to UV curing. |
|||
==== Bio-printing ==== |
|||
Fiber optic coatings are applied in concentric layers to prevent damage to the fiber during the drawing application and to maximize fiber strength and microbend resistance. Unevenly coated fiber will experience non-uniform forces when the coating expands or contracts, and is susceptible to greater signal attenuation. Under proper drawing and coating processes, the coatings are concentric around the fiber, continuous over the length of the application and have constant thickness. |
|||
{{As of|2012}}, 3D [[bio-printing]] technology has been studied by [[biotechnology]] firms and academia for possible use in tissue engineering applications in which organs and body parts are built using inkjet techniques. In this process, layers of living cells are deposited onto a gel medium or sugar matrix and slowly built up to form three-dimensional structures including vascular systems.<ref name="Auto3D-38" /> The first production system for 3D tissue printing was delivered in 2009, based on [[NovoGen]] bioprinting technology.<ref name="AutoSQ-46" /> Several terms have been used to refer to this field of research: organ printing, bio-printing, body part printing,<ref name="Auto3D-39" /> and computer-aided [[tissue engineering]], among others.<ref name="Auto3D-40" /> The possibility of using 3D tissue printing to create soft tissue architectures for reconstructive surgery is also being explored.<ref>[http://www.engineering.com/3DPrinting/3DPrintingArticles/ArticleID/7379/Engineering-Ourselves-The-Future-Potential-Power-of-3D-Bioprinting.aspx Dan Thomas, Engineering Ourselves – The Future Potential Power of 3D-Bioprinting?, engineering.com, March 25, 2014]</ref> |
|||
In 2013, Chinese scientists began printing ears, livers and kidneys, with living tissue. Researchers in China have been able to successfully print [[human organs]] using specialized 3D bio printers that use living cells instead of plastic {{citation needed|date=November 2015}}. Researchers at [[Hangzhou Dianzi University]] designed the "3D bio printer" dubbed the "Regenovo". Xu Mingen, Regenovo's developer, said that it takes the printer under an hour to produce either a mini liver sample or a four to five inch ear cartilage sample. Xu also predicted that fully functional printed organs may be possible within the next ten to twenty years.<ref name="AutoSQ-76" /><ref name="AutoSQ-77" /> In the same year, researchers at the [[University of Hasselt]], in Belgium had successfully printed a new [[jawbone]] for an 83-year-old Belgian woman.<ref name="AutoSQ-78" /> |
|||
Fiber optic coatings protect the glass fibers from scratches that could lead to strength degradation. The combination of moisture and scratches accelerates the aging and deterioration of fiber strength. When fiber is subjected to low stresses over a long period, fiber fatigue can occur. Over time or in extreme conditions, these factors combine to cause microscopic flaws in the glass fiber to propagate, which can ultimately result in fiber failure. |
|||
==== Pills ==== |
|||
Three key characteristics of fiber optic waveguides can be affected by environmental conditions: strength, attenuation and resistance to losses caused by microbending. External fiber optic coatings protect glass optical fiber from environmental conditions that can affect the fiber’s performance and long-term durability. On the inside, coatings ensure the reliability of the signal being carried and help minimize attenuation due to microbending. |
|||
The first pill manufactured by 3D printing was approved by FDA in August 2015. [[Powder bed and inkjet head 3D printing|Binder-jetting]] into a powder bed of the drug allows very porous pills to be produced, which enables high drug doses in a single pill which dissolves quickly and can be ingested easily.<ref>{{cite web|url=http://www.fiercepharmamanufacturing.com/story/fda-approves-seizure-drug-made-3-d-technology/2015-08-03|title=Company builds plant for 3DP pill making as it nails first FDA approval|author=Palmer, Eric|publisher=fiercepharmamanufacturing.com|date=3 August 2015|accessdate=4 August 2015}}</ref> This has been demonstrated for Spritam, a reformulation of [[levetiracetam]] for the treatment of [[epilepsy]]. |
|||
=== Computers and robots === |
|||
==Practical issues== |
|||
{{See also|Modular design|Open-source robotics}} |
|||
3D printing can also be used to make laptops and other computers and cases. For example, [[Novena (computing platform)|Novena]] and [[VIA OpenBook]] standard [[laptop case]]s. I.e. a [[Novena (computing platform)|Novena]] motherboard can be bought and be used in a printed VIA OpenBook case.<ref>[http://www.wired.com/2014/04/novena/ The Almost Completely Open Source Laptop Goes on Sale]</ref> |
|||
[[Open-source robotics|Open-source robots]] are built using 3D printers. [[Double Robotics]] grant access to their technology (an open [[Software development kit|SDK]]).<ref>[http://www.forbes.com/sites/tjmccue/2014/10/22/robots-and-3d-printing/ Robots And 3D Printing]</ref><ref>[http://www.intorobotics.com/use-3d-printers-best-3d-printers-build-robot/ Why to Use 3D Printers and the Best 3D Printers To Build Your Own Robot]</ref><ref>[http://www.intorobotics.com/printoo-giving-life-everyday-objects/ Printoo: Giving Life to Everyday Objects] (paper-thin, flexible [[Arduino]]-compatible modules)</ref> On the other hand, [[3&DBot]] is an [[Arduino]] 3D printer-robot with wheels<ref>[http://www.intorobotics.com/3dbot-arduino-3d-printer-robot-wheels/ 3&DBot: An Arduino 3D printer-robot with wheels]</ref> and [[ODOI]] is a 3D printed [[humanoid robot]].<ref>[http://www.intorobotics.com/lesson-building-custom-3d-printed-humanoid-robot/ A lesson in building a custom 3D printed humanoid robot]</ref> |
|||
===Cable construction=== |
|||
[[File:Optical fiber cable.jpg|thumb|An [[optical fiber cable]]]] |
|||
{{Main|Optical fiber cable}} |
|||
In practical fibers, the cladding is usually coated with a tough [[resin]] ''[[buffer (optical fiber)|buffer]]'' layer, which may be further surrounded by a ''jacket'' layer, usually glass. These layers add strength to the fiber but do not contribute to its optical wave guide properties. Rigid fiber assemblies sometimes put light-absorbing ("dark") glass between the fibers, to prevent light that leaks out of one fiber from entering another. This reduces [[crosstalk (electronics)|cross-talk]] between the fibers, or reduces [[Lens flare|flare]] in fiber bundle imaging applications.<ref>{{cite web| url=http://zone.ni.com/devzone/cda/ph/p/id/129#toc2| title=Light collection and propagation| work=National Instruments' Developer Zone|publisher=National Instruments Corporation |accessdate=2007-03-19}}</ref><ref name=hecht2002> |
|||
{{cite book| first=Jeff| last=Hecht| title=Understanding Fiber Optics| year=2002| edition=4th| isbn=0-13-027828-9|publisher= Prentice Hall}}</ref> |
|||
=== Space === |
|||
Modern cables come in a wide variety of sheathings and armor, designed for applications such as direct burial in trenches, high voltage isolation, dual use as power lines,<ref>{{cite web |url=http://www.dced.state.ak.us/dca/AEIS/PDF_Files/AIDEA_Energy_Screening.pdf |title=Screening report for Alaska rural energy plan |format=PDF |work=Alaska Division of Community and Regional Affairs|accessdate=April 11, 2006|archiveurl=http://web.archive.org/web/20060508191931/http://www.dced.state.ak.us/dca/AEIS/PDF_Files/AIDEA_Energy_Screening.pdf|archivedate=May 8, 2006}}</ref>{{Failed verification|date=November 2008}} installation in conduit, lashing to aerial telephone poles, submarine installation, and insertion in paved streets. The cost of small fiber-count pole-mounted cables has greatly decreased due to the high demand for [[fiber to the home]] (FTTH) installations in Japan and South Korea. |
|||
{{See also|3D-printed spacecraft|3D printing#Construction}} |
|||
The [[Zero-G Printer]], the first 3D printer designed to operate in zero gravity, was built under a joint partnership between NASA Marshall Space Flight Center (MSFC) and [[Made In Space, Inc.]]<ref>{{cite web|title=New horizons open with space-based 3D printing|url=http://spie.org/newsroom/technical-articles/videos/0331-madeinspace-video|publisher=SPIE Newsroom|accessdate=1 April 2015}}</ref> |
|||
In September 2014, [[SpaceX]] delivered the zero-gravity 3D printer to the [[International Space Station]] (ISS). On December 19, 2014, [[NASA]] emailed CAD drawings for a socket wrench to astronauts aboard the ISS, who then printed the tool using its 3D printer. Applications for space offer the ability to print parts or tools on-site, as opposed to using rockets to bring along pre-manufactured items for space missions to human colonies on the moon, Mars, or elsewhere.<ref>{{cite web|url=http://www.upi.com/Science_News/2014/12/19/NASA-just-emailed-the-space-station-a-new-socket-wrench/1111419017637/ |last=Hays |first=Brooks |title=NASA just emailed the space station a new socket wrench |date=2014-12-19|accessdate=2014-12-20}}</ref> The European Space Agency plans to deliver its new Portable On-Board 3D Printer (POP3D for short) to the International Space Station by June 2015, making it the second 3D printer in space.<ref>{{cite web|url=http://www.space.com/28418-european-3d-printer-in-space.html |last=Brabaw |first=Kasandra |title=Europe's 1st Zero-Gravity 3D Printer Headed for Space |date=2015-01-30|accessdate=2015-02-01}}</ref><ref>{{cite web|url=http://www.gizmag.com/pop3d-3d-printer-esa-iss/34764/ |last=Wood|first=Anthony |title=POP3D to be Europe's first 3D printer in space |date=2014-11-17|accessdate=2015-02-01}}</ref> |
|||
Furthermore, the Sinterhab project is researching a lunar base constructed by 3D printing using [[Lunar soil|lunar regolith]] as a base material. Instead of adding a binding agent to the regolith, researchers are experimenting with microwave [[sintering]] to create solid blocks from the raw material.<ref name="AutoSQ-73" /> |
|||
Fiber cable can be very flexible, but traditional fiber's loss increases greatly if the fiber is bent with a radius smaller than around 30 mm. This creates a problem when the cable is bent around corners or wound around a spool, making [[FTTX]] installations more complicated. "Bendable fibers", targeted towards easier installation in home environments, have been standardized as ITU-T G.657. This type of fiber can be bent with a radius as low as 7.5 mm without adverse impact. Even more bendable fibers have been developed.<ref>{{cite press release |
|||
|title = Corning announces breakthrough optical fiber technology |
|||
|publisher = [[Corning Incorporated]] |
|||
|date = 2007-07-23 |
|||
|url = http://www.corning.com/news_center/news_releases/2007/2007072301.aspx |
|||
|accessdate = 2013-09-09}}</ref> |
|||
Bendable fiber may also be resistant to fiber hacking, in which the signal in a fiber is surreptitiously monitored by bending the fiber and detecting the leakage.<ref>{{cite web |url=http://blogs.techrepublic.com.com/security/?p=222 |title=Protect your network against fiber hacks |accessdate=2007-12-10 |last=Olzak |first=Tom |date=2007-05-03 |work=Techrepublic|publisher=CNET|archiveurl=http://archive.is/1Suj|archivedate=2012-07-07}}</ref> |
|||
Similar researches and projects like these could allow faster construction for lower costs, and has been investigated for construction of off-Earth habitats.<ref name="tc20120120" /><ref name="gizmodo20130131" /> |
|||
Another important feature of cable is cable's ability to withstand horizontally applied force. It is technically called max tensile strength defining how much force can be applied to the cable during the installation period. |
|||
== Sociocultural applications == |
|||
Some fiber optic cable versions are reinforced with [[aramid]] yarns or glass yarns as intermediary [[strength member]]. In commercial terms, usage of the glass yarns are more cost effective while no loss in mechanical durability of the cable. Glass yarns also protect the cable core against rodents and termites. |
|||
[[File:Ted Noten Fashionista in green 2009.jpg|thumb|An example of 3D printed limited edition [[jewellery]]. This necklace is made of glassfiber-filled dyed nylon. It has rotating linkages that were produced in the same manufacturing step as the other parts]] |
|||
[[File:Guardians of Time sculpture Manfred Kielnhofer 3d printing.JPG|thumb|Guardians of Time by [[Manfred Kielnhofer]], 3D printing polished nickel steel by Shapeways 2014]] |
|||
[[File:Ceramic 3D Printing by Studio Under4.jpg|thumb|220px|right|3D printed ceramic pot]] |
|||
In 2005, a rapidly expanding hobbyist and home-use market was established with the inauguration of the [[open-source]] [[RepRap]] and [[Fab@Home]] projects. Virtually all home-use 3D printers released to-date have their technical roots in the ongoing RepRap Project and associated open-source software initiatives.<ref name="reprap heritage" /> In distributed manufacturing, one study has found<ref name="AutoSQ-3" /> that 3D printing could become a mass market product enabling consumers to save money associated with purchasing common household objects.<ref name="wittbrodt" /> For example, instead of going to a store to buy an object made in a factory by [[injection molding]] (such as a [[measuring cup]] or a [[funnel]]), a person might instead print it at home from a downloaded 3D model. |
|||
===Termination and splicing=== |
|||
[[File:ST-optical-fiber-connector-hdr-0a.jpg|thumb|upright|[[optical fiber connector|ST connectors]] on [[multi-mode fiber]].]] |
|||
=== Art === |
|||
Optical fibers are connected to terminal equipment by [[optical fiber connector]]s. These connectors are usually of a standard type such as ''FC'', ''SC'', ''ST'', ''LC'', ''MTRJ'', or ''SMA,'' which is designated for higher power transmission. All of these standardised connectors are a "physical contact" (PC) type where the mating surfaces touch each other to achieve the lowest possible attenuation, and all but the ST have a keyed ferrule that allows and angled (APC) mating surface to reduce reflections that can cause problems with laser transmitters. |
|||
In 2005, academic journals had begun to report on the possible artistic applications of 3D printing technology.<ref name="Auto3D-34" /> By 2007 the mass media followed with an article in the Wall Street Journal<ref name="Auto3D-35" /> and Time Magazine, listing a 3D printed design among their 100 most influential designs of the year.<ref name="Auto3D-36" /> During the 2011 London Design Festival, an installation, curated by Murray Moss and focused on 3D Printing, was held in the Victoria and Albert Museum (the V&A). The installation was called ''Industrial Revolution 2.0: How the Material World will Newly Materialize''.<ref name="Auto3D-37" /> |
|||
[[File:3-d printed flower model.jpg|thumb|flower model made with a 3D printer]] |
|||
Some of the recent developments in 3D printing were revealed at the 3DPrintshow in London, which took place in November 2013 and 2014. The art section had in exposition artworks made with 3D printed plastic and metal. Several artists such as Joshua Harker, Davide Prete, Sophie Kahn, Helena Lukasova, Foteini Setaki showed how 3D printing can modify aesthetic and art processes. One part of the show focused on ways in which 3D printing can advance the medical field. The underlying theme of these advances was that these printers can be used to create parts that are printed with specifications to meet each individual. This makes the process safer and more efficient. One of these advances is the use of 3D printers to produce casts that are created to mimic the bones that they are supporting. These custom-fitted casts are open, which allow the wearer to scratch any itches and also wash the damaged area. Being open also allows for open ventilation. One of the best features is that they can be recycled to create more casts.<ref name="AutoSQ-80" /> |
|||
Optical fibers may be connected to each other by connectors or by ''splicing'', that is, joining two fibers together to form a continuous optical waveguide. The generally accepted splicing method is [[fusion splicing|arc fusion splicing]], which melts the fiber ends together with an [[electric arc]]. For quicker fastening jobs, a “mechanical splice” is used. |
|||
[[File:Ceramic 3D Printing by Studio Under2.jpg|thumb|Ceramic 3D Printing by Studio Under]] |
|||
3D printing is becoming more popular in the customisable gifts industry, with products such as personalized mobile phone cases and dolls,<ref>{{cite web|title=Custom Bobbleheads|url=http://www.headbobble.com/|accessdate=13 January 2015}}</ref> as well as 3D printed chocolate.<ref>{{cite news|title=3D-print your face in chocolate for that special Valentine's Day gift|url=http://www.theguardian.com/artanddesign/architecture-design-blog/2013/jan/25/3d-print-chocolate-face-valentines-day|publisher=The Guardian|date=25 January 2013}}</ref> |
|||
The use of [[3D scanning]] technologies allows the replication of real objects without the use of [[molding (process)|moulding]] techniques that in many cases can be more expensive, more difficult, or too invasive to be performed, particularly for precious or delicate cultural heritage artifacts<ref name="Auto3D-42" /> where direct contact with the moulding substances could harm the original object's surface. |
|||
Fusion splicing is done with a specialized instrument that typically operates as follows: The two cable ends are fastened inside a splice enclosure that will protect the splices, and the fiber ends are stripped of their protective polymer coating (as well as the more sturdy outer jacket, if present). The ends are ''cleaved'' (cut) with a precision cleaver to make them perpendicular, and are placed into special holders in the splicer. The splice is usually inspected via a magnified viewing screen to check the cleaves before and after the splice. The splicer uses small motors to align the end faces together, and emits a small spark between [[electrodes]] at the gap to burn off dust and moisture. Then the splicer generates a larger spark that raises the temperature above the [[melting point]] of the glass, fusing the ends together permanently. The location and energy of the spark is carefully controlled so that the molten core and cladding do not mix, and this minimizes optical loss. A splice loss estimate is measured by the splicer, by directing light through the cladding on one side and measuring the light leaking from the cladding on the other side. A splice loss under 0.1 dB is typical. The complexity of this process makes fiber splicing much more difficult than splicing copper wire. |
|||
[[Critical making]] refers to the hands on productive activities that link digital technologies to society. It is invented to bridge the gap between creative physical and conceptual exploration.<ref name="AutoSQ-63" /> The term was popularized by Matt Ratto, an Assistant Professor and director of the Critical Making lab in the Faculty of Information at the University of Toronto. Ratto describes one of the main goals of critical as "to use material forms of engagement with technologies to supplement and extend critical reflection and, in doing so, to reconnect our lived experiences with technologies to social and conceptual critique".<ref name="AutoSQ-64" /> The main focus of critical making is open design,<ref name="AutoSQ-65" /> which includes, in addition to 3D printing technologies, also other digital software and hardware. People usually reference spectacular design when explaining critical making.<ref name="AutoSQ-66" /> |
|||
Mechanical fiber splices are designed to be quicker and easier to install, but there is still the need for stripping, careful cleaning and precision cleaving. The fiber ends are aligned and held together by a precision-made sleeve, often using a clear [[index-matching gel]] that enhances the transmission of light across the joint. Such joints typically have higher optical loss and are less robust than fusion splices, especially if the gel is used. All splicing techniques involve installing an enclosure that protects the splice. |
|||
=== Communication === |
|||
Fibers are terminated in connectors that hold the fiber end precisely and securely. A fiber-optic connector is basically a rigid cylindrical barrel surrounded by a sleeve that holds the barrel in its mating socket. The mating mechanism can be ''push and click'', ''turn and latch'' (''[[bayonet mount]]''), or ''screw-in'' (''threaded''). A typical connector is installed by preparing the fiber end and inserting it into the rear of the connector body. Quick-set adhesive is usually used to hold the fiber securely, and a [[strain relief]] is secured to the rear. Once the adhesive sets, the fiber's end is polished to a mirror finish. Various polish profiles are used, depending on the type of fiber and the application. For single-mode fiber, fiber ends are typically polished with a slight curvature that makes the mated connectors touch only at their cores. This is called a ''physical contact'' (PC) polish. The curved surface may be polished at an angle, to make an ''angled physical contact (APC)'' connection. Such connections have higher loss than PC connections, but greatly reduced back reflection, because light that reflects from the angled surface leaks out of the fiber core. The resulting signal strength loss is called ''[[gap loss]]''. APC fiber ends have low back reflection even when disconnected. |
|||
Employing additive layer technology offered by 3D printing, Terahertz devices which act as waveguides, couplers and bends have been created. The complex shape of these devices could not be achieved using conventional fabrication techniques. Commercially available professional grade printer EDEN 260V was used to create structures with minimum feature size of 100 µm. The printed structures were later DC sputter coated with gold (or any other metal) to create a Terahertz Plasmonic Device.<ref name="AutoSQ-74" /> |
|||
=== Domestic use === |
|||
In the 1990s, terminating fiber optic cables was labor-intensive. The number of parts per connector, polishing of the fibers, and the need to oven-bake the epoxy in each connector made terminating fiber optic cables difficult. Today, many connectors types are on the market that offer easier, less labor-intensive ways of terminating cables. Some of the most popular connectors are pre-polished at the factory, and include a gel inside the connector. Those two steps help save money on labor, especially on large projects. A [[cleave (fiber)|cleave]] is made at a required length, to get as close to the polished piece already inside the connector. The gel surrounds the point where the two pieces meet inside the connector for very little light loss.{{Citation needed|date=May 2010}} |
|||
As of 2012, domestic 3D printing was mainly practiced by hobbyists and enthusiasts. However, little was used for practical household applications, for example, ornamental objects. Some practical examples include a working clock<ref name="Auto3D-21" /> and [[gear]]s printed for home woodworking machines among other purposes.<ref name="Auto3D-23" /> Web sites associated with home 3D printing tended to include backscratchers, coat hooks, door knobs, etc.<ref>[http://www.yeggi.com/q/backscratcher/?s=tt Search engine for 3D printable models, "backscratcher", etc.]</ref> |
|||
The open source Fab@Home project<ref name="fabathome" /> has developed printers for general use. They have been used in research environments to produce chemical compounds with 3D printing technology, including new ones, initially without immediate application as proof of principle.<ref name="chem" /> The printer can print with anything that can be dispensed from a syringe as liquid or paste. The developers of the chemical application envisage both industrial and domestic use for this technology, including enabling users in remote locations to be able to produce their own medicine or household chemicals.<ref name="Auto3D-25" /><ref name="BBC_2012-04-17" /> |
|||
===Free-space coupling=== |
|||
It is often necessary to align an optical fiber with another optical fiber, or with an [[optoelectronic device]] such as a [[light-emitting diode]], a [[laser diode]], or a [[modulator]]. This can involve either carefully aligning the fiber and placing it in contact with the device, or can use a [[lens (optics)|lens]] to allow coupling over an air gap. In some cases the end of the fiber is polished into a curved form that makes it act as a lens. Some companies can even shape the fiber into lenses by cutting them with lasers.<ref>{{cite web|title=Laser Lensing |work=OpTek Systems Inc.|url=http://www.opteksystems.com/laser-lens}}</ref> |
|||
3D printing is now working its way into households, and more and more children are being introduced to the concept of 3D printing at earlier ages. The prospects of 3D printing are growing, and as more people have access to this new innovation, new uses in households will emerge.<ref>{{cite web|last1=D'Aveni|first1=Richard|title=3-D Printing Will Change the World|url=http://hbr.org/2013/03/3-d-printing-will-change-the-world/|website=Harvard Business Review|accessdate=October 8, 2014}}</ref> |
|||
In a laboratory environment, a bare fiber end is coupled using a fiber launch system, which uses a [[microscope objective lens]] to focus the light down to a fine point. A precision [[translation stage]] (micro-positioning table) is used to move the lens, fiber, or device to allow the coupling efficiency to be optimized. Fibers with a connector on the end make this process much simpler: the connector is simply plugged into a pre-aligned fiberoptic collimator, which contains a lens that is either accurately positioned with respect to the fiber, or is adjustable. To achieve the best injection efficiency into single-mode fiber, the direction, position, size and divergence of the beam must all be optimized. With good beams, 70 to 90% coupling efficiency can be achieved. |
|||
The OpenReflex [[Single-lens reflex camera|SLR]] [[Photographic film|film]] camera was developed for 3D printing as an open-source student project.<ref name="AutoSQ-44" /> |
|||
With properly polished single-mode fibers, the emitted beam has an almost perfect Gaussian shape—even in the far field—if a good lens is used. The lens needs to be large enough to support the full numerical aperture of the fiber, and must not introduce [[optical aberration|aberrations]] in the beam. [[Aspheric lens]]es are typically used. |
|||
=== Education and research === |
|||
===Fiber fuse=== |
|||
3D printing, and open source RepRap 3D printers in particular, are the latest technology making inroads into the classroom.<ref>Schelly, C., Anzalone, G., Wijnen, B., & Pearce, J. M. (2015). "Open-source 3-D printing Technologies for education: Bringing Additive Manufacturing to the Classroom." ''Journal of Visual Languages & Computing''.</ref><ref>Grujović, N., Radović, M., Kanjevac, V., Borota, J., Grujović, G., & Divac, D. (2011, September). "3D printing technology in education environment." In ''34th International Conference on Production Engineering'' (pp. 29–30).</ref><ref>Mercuri, R., & Meredith, K. (2014, March). "An educational venture into 3D Printing." In Integrated STEM Education Conference (ISEC), 2014 IEEE (pp. 1–6). IEEE.</ref> 3D printing allows students to create prototypes of items without the use of expensive tooling required in subtractive methods. Students design and produce actual models they can hold. The classroom environment allows students to learn and employ new applications for 3D printing.<ref name="AutoSQ-60" /> RepRaps, for example, have already been used for an educational mobile robotics platform.<ref>Gonzalez-Gomez, J., Valero-Gomez, A., Prieto-Moreno, A., & Abderrahim, M. (2012). "A new open source 3d-printable mobile robotic platform for education." In ''Advances in Autonomous Mini Robots'' (pp. 49–62). Springer Berlin Heidelberg.</ref> |
|||
At high optical intensities, above 2 [[megawatt]]s per square centimeter, when a fiber is subjected to a shock or is otherwise suddenly damaged, a ''fiber fuse'' can occur. The reflection from the damage vaporizes the fiber immediately before the break, and this new defect remains reflective so that the damage propagates back toward the transmitter at 1–3 meters per second (4–11 km/h, 2–8 mph).<ref>{{cite journal |first=R. M. |last=Atkins |author2=Simpkins, P. G. |author3=Yablon, A. D. | title=Track of a fiber fuse: a Rayleigh instability in optical waveguides |journal=Optics Letters|url=http://ol.osa.org/abstract.cfm?id=72607|year=2003 |volume=28 |issue=12 |pages=974–976 |doi=10.1364/OL.28.000974 |pmid=12836750|bibcode = 2003OptL...28..974A }}</ref><ref>{{cite journal |first=Breck |last=Hitz |title=Origin of 'fiber fuse' is revealed |journal=Photonics Spectra |date=August 2003| url=http://www.photonics.com/Article.aspx?AID=16745|accessdate=2011-01-23}}</ref> The [[open fiber control]] system, which ensures [[laser safety|laser eye safety]] in the event of a broken fiber, can also effectively halt propagation of the fiber fuse.<ref>{{cite journal |first=Koji |last=Seo |title=Evaluation of high-power endurance in optical fiber links |journal=Furukawa Review |issue= 24 |date=October 2003 |pages=17–22 |issn=1348-1797|url=http://www.furukawa.co.jp/review/fr024/fr24_04.pdf|accessdate=2008-07-05|display-authors=etal}}</ref> In situations, such as undersea cables, where high power levels might be used without the need for open fiber control, a "fiber fuse" protection device at the transmitter can break the circuit to keep damage to a minimum. |
|||
Some authors have claimed that RepRap 3D printers offer an unprecedented "revolution" in [[STEM]] education.<ref>J. Irwin, J.M. Pearce, D. Opplinger, and G. Anzalone. [https://www.academia.edu/9647945/The_RepRap_3-D_Printer_Revolution_in_STEM_Education The RepRap 3-D Printer Revolution in STEM Education], ''121st ASEE Annual Conference and Exposition, Indianapolis, IN''. Paper ID #8696 (2014).</ref> The evidence for such claims comes from both the low cost ability for [[rapid prototyping]] in the classroom by students, but also the fabrication of low-cost high-quality scientific equipment from [[open hardware]] designs forming [[open-source labs]].<ref name="AutoSQ-68" /> Engineering and design principles are explored as well as architectural planning. Students recreate duplicates of museum items such as fossils and historical artifacts for study in the classroom without possibly damaging sensitive collections. Other students interested in graphic designing can construct models with complex working parts. 3D printing gives students a new perspective with topographic maps. Science students can study cross-sections of internal organs of the human body and other biological specimens. And chemistry students can explore 3D models of molecules and the relationship within chemical compounds.<ref name="AutoSQ-61" /> |
|||
===Chromatic dispersion=== |
|||
{{main|Dispersion (optics)}} |
|||
The refractive index of fibers varies slightly with the frequency of light, and light sources are not perfectly monochromatic. Modulation of the light source to transmit a signal also slightly widens the frequency band of the transmitted light. This has the effect that, over long distances and at high modulation speeds, the different frequencies of light can take different times to arrive at the receiver, ultimately making the signal impossible to discern, and requiring extra repeaters.<ref>G. P. Agrawal, Fiber Optic Communication Systems, Wiley-Interscience, 1997.</ref> This problem can be overcome in a number of ways, including the use of a relatively short length of fiber that has the opposite refractive index gradient. |
|||
According to a recent paper by Kostakis et al.,<ref name="AutoSQ-62" /> 3D printing and design can electrify various literacies and creative capacities of children in accordance with the spirit of the interconnected, information-based world. |
|||
==See also== |
|||
Future applications for 3D printing might include creating open-source scientific equipment.<ref name="AutoSQ-68" /><ref name="AutoQK-4" /> |
|||
{{Portal|Electronics}} |
|||
{{div col||20em}} |
|||
=== Environmental use === |
|||
* [[Borescope]] |
|||
In [[Bahrain]], large-scale 3D printing using a [[sandstone]]-like material has been used to create unique [[coral]]-shaped structures, which encourage coral [[polyp]]s to colonize and regenerate damaged [[reef]]s. These structures have a much more natural shape than other structures used to create [[artificial reef]]s, and, unlike concrete, are neither acid nor alkaline with neutral [[pH]].<ref name="AutoSQ-79" /> |
|||
* [[Cable jetting]] |
|||
* [[Data cable]] |
|||
=== Archaeology and museums use === |
|||
* [[Distributed acoustic sensing]] |
|||
In the last several years 3D printing has been intensively used by museums and archaeologists for restoration of damaged artifacts. Many Europeans and North American Museums have purchased 3D printers and actively recreate missing pieceс of their of relics.<ref>[http://www.3ders.org/articles/20150714-museum-uses-3d-printing-to-take-fragile-maquette-by-thomas-hart-benton-on-tour.html Museum uses 3D printing to take fragile maquette by Thomas Hart Benton on tour through the States]</ref> |
|||
* [[Endoscopy]] |
|||
* [[Fiber amplifier]] |
|||
The [[Metropolitan Museum of Art]] and the [[British Museum]] have started using their 3D printers to create museum souvenirs that are available in the museum shops.<ref>[http://www.independent.co.uk/life-style/gadgets-and-tech/british-museum-releases-scans-of-artefacts-to-let-you-3d-print-your-own-museum-at-home-9837654.html British Museum releases scans of artefacts to let you 3D print your own museum at home]</ref> Other museums, like the National Museum of Military History and Varna Historical Museum, have gone further and sell through the online platform [[Threeding]] digital models of their artifacts in 3D printing friendly file format, which everyone can 3D print at home.<ref>[http://3dprint.com/45699/threeding-artec-museum/ Threeding Uses Artec 3D Scanning Technology to Catalog 3D Models for Bulgaria’s National Museum of Military History]</ref> |
|||
* [[Fiber Bragg grating]] |
|||
* [[Fiber laser]] |
|||
[[File:3D Printed Ancient Egyptian Figurine.png|thumb|3D printed sculpture of the Egyptian Pharaoh [[Merankhre Mentuhotep]] shown at [[Threeding]]]] |
|||
* [[Fiber pigtail]] |
|||
* [[Fiberscope]] |
|||
=== Specialty materials === |
|||
* [[Fibre Channel]] |
|||
Consumer grade 3D printing has resulted in new materials that have been developed specifically for 3D printers. For example, filament materials have been developed to imitate wood, in its appearance as well as its texture. Furthermore, new technologies, such as infusing carbon fiber<ref name="carbon-fiber" /> into printable plastics, allowing for a stronger, lighter material. In addition to new structural materials that have been developed due to 3D printing, new technologies have allowed for patterns to be applied directly to 3D printed parts. Iron oxide-free [[Portland cement]] powder has been used to create architectural structures up to 9 feet in height.<ref name=sanfranciscocbs>{{cite news|url=http://sanfrancisco.cbslocal.com/2015/03/06/researchers-at-uc-berkeley-create-bloom-first-ever-3-d-printed-cement-structure-that-stands-9-feet-tall/|title=Researchers at UC Berkeley Create Bloom First Ever 3D-printed Cement Structure That Stands 9 Feet Tall|accessdate=23 April 2015|date=6 March 2015|publisher=cbs sanfrancisco}}</ref><ref name=inhabitat>{{cite web|url=http://inhabitat.com/worlds-largest-3d-printed-building-made-from-powdered-cement-unveiled-at-uc-berkeley/|title=UC Berkeley unveils 3D-printed "Bloom" building made of powdered cement|date=9 March 2015|accessdate=23 April 2015|first=Mike|last=Chino}}</ref><ref name=archrecord>{{cite news|url=http://archrecord.construction.com/news/2015/03/150306-Print-it-Real-Good-First-powder-based-3D-Print-Cement-Structure-unveiled-at-UC-Berkeley.asp|date=6 March 2015|accessdate=23 April 2015|title=Print it Real Good: First Powder-Based 3D Printed Cement Structure Unveiled|first=Anna|last=Fixsen}}</ref> |
|||
* [[Gradient-index optics]] |
|||
* [[Interconnect bottleneck]] |
|||
== Legal aspects == |
|||
* [[Leaky mode]] |
|||
* [[Li-Fi]] |
|||
=== Intellectual property === |
|||
* [[Light Peak]] |
|||
{{See also|Free hardware}} |
|||
* [[Modal bandwidth]] |
|||
3D printing has existed for decades within certain manufacturing industries where many legal regimes, including [[patent]]s, [[industrial design right]]s, [[copyright]], and [[trademark]] may apply. However, there is not much [[jurisprudence]] to say how these laws will apply if 3D printers become mainstream and individuals and hobbyist communities begin manufacturing items for personal use, for non-profit distribution, or for sale. |
|||
* [[Optical amplifier]] |
|||
* [[Optical communication]] |
|||
Any of the mentioned legal regimes may prohibit the distribution of the designs used in 3D printing, or the distribution or sale of the printed item. To be allowed to do these things, where an active intellectual property was involved, a person would have to contact the owner and ask for a licence, which may come with conditions and a price. However, many patent, design and copyright laws contain a standard limitation or exception for 'private', 'non-commercial' use of inventions, designs or works of art protected under intellectual property (IP). That standard limitation or exception may leave such private, non-commercial uses outside the scope of IP rights. |
|||
* [[Optical fiber cable]] |
|||
* [[Optical fiber connector]] |
|||
Patents cover inventions including processes, machines, manufactures, and compositions of matter and have a finite duration which varies between countries, but generally 20 years from the date of application. Therefore, if a type of wheel is patented, printing, using, or selling such a wheel could be an infringement of the patent.<ref name="AutoSQ-82" /> |
|||
* [[Optical interconnect]] |
|||
* [[Optical mesh network]] |
|||
Copyright covers an expression<ref name="wired" /> in a tangible, fixed medium and often lasts for the life of the author plus 70 years thereafter.<ref name="deal" /> If someone makes a statue, they may have copyright on the look of that statue, so if someone sees that statue, they cannot then distribute designs to print an identical or similar statue. |
|||
* [[Optical power meter]] |
|||
* [[Optical time-domain reflectometer]] |
|||
When a feature has both artistic (copyrightable) and functional (patentable) merits, when the question has appeared in US court, the courts have often held the feature is not copyrightable unless it can be separated from the functional aspects of the item.<ref name="deal" /> In other countries the law and the courts may apply a different approach allowing, for example, the design of a useful device to be registered (as a whole) as an industrial design on the understanding that, in case of unauthorized copying, only the non-functional features may be claimed under design law whereas any technical features could only be claimed if covered by a valid patent. |
|||
* [[Optoelectronics]] |
|||
* [[Parallel optical interface]] |
|||
=== Gun legislation and administration === |
|||
* [[Photonic-crystal fiber]] |
|||
The US [[Department of Homeland Security]] and the [[Joint Regional Intelligence Center]] released a memo stating that "significant advances in three-dimensional (3D) printing capabilities, availability of free digital 3D printable files for firearms components, and difficulty regulating file sharing may present public safety risks from unqualified gun seekers who obtain or manufacture 3D printed guns," and that "proposed legislation to ban 3D printing of weapons may deter, but cannot completely prevent their production. Even if the practice is prohibited by new legislation, online distribution of these 3D printable files will be as difficult to control as any other illegally traded music, movie or software files."<ref name="AutoSQ-83" /> |
|||
* [[Return loss]] |
|||
* [[Small form-factor pluggable transceiver]] |
|||
Internationally, where gun controls are generally stricter than in the United States, some commentators have said the impact may be more strongly felt, as alternative firearms are not as easily obtainable.<ref name="AutoSQ-84" /> European officials have noted that producing a 3D printed gun would be illegal under their gun control laws,<ref name="AutoSQ-85" /> and that criminals have access to other sources of weapons, but noted that as the technology improved the risks of an effect would increase.<ref name="AutoSQ-86" /><ref name="AutoSQ-87" /> Downloads of the plans from the UK, Germany, Spain, and Brazil were heavy.<ref name="AutoSQ-88" /><ref name="AutoSQ-89" /> |
|||
* [[Soliton]], [[Vector soliton]] |
|||
* [[Submarine communications cable]]s |
|||
Attempting to restrict the distribution over the Internet of gun plans has been likened to the futility of preventing the widespread distribution of [[DeCSS]] which enabled DVD [[ripping]].<ref name="AutoSQ-90" /><ref name="AutoSQ-91" /><ref name="AutoSQ-92" /><ref name="AutoSQ-93" /> After the US government had Defense Distributed take down the plans, they were still widely available via [[The Pirate Bay]] and other file sharing sites.<ref name="AutoSQ-94" /> Some US legislators have proposed regulations on 3D printers, to prevent them being used for printing guns.<ref name="AutoSQ-95" /><ref name="AutoSQ-96" /> 3D printing advocates have suggested that such regulations would be futile, could cripple the 3D printing industry, and could infringe on free speech rights, with early pioneer of 3D printing Professor [[Hod Lipson]] suggesting that gunpowder could be controlled instead.<ref name="AutoSQ-97" /><ref name="AutoSQ-98" /><ref name="AutoSQ-99" /><ref name="AutoSQ-100" /><ref name="AutoSQ-101" /><ref name="AutoSQ-102" /><ref name="AutoSQ-103" /> |
|||
* [[Subwavelength-diameter optical fibre]] |
|||
* [[Surround Optical Fiber Immunoassay (SOFIA)]] |
|||
== Impact == |
|||
* [[XENPAK]] |
|||
Additive manufacturing, starting with today's infancy period, requires manufacturing firms to be flexible, [[continuous improvement process|ever-improving]] users of all available technologies to remain competitive. Advocates of additive manufacturing also predict that this arc of technological development will counter [[globalization]], as end users will do much of their own manufacturing rather than engage in trade to buy products from other people and corporations.<ref name="3D opp" /> The real integration of the newer additive technologies into commercial production, however, is more a matter of complementing traditional subtractive methods rather than displacing them entirely.<ref name="Albert_2011-02_MMS_column" /> |
|||
=== Social change === |
|||
Since the 1950s, a number of writers and social commentators have speculated in some depth about the social and cultural changes that might result from the advent of commercially affordable additive manufacturing technology.<ref name="AutoSQ-104" /> Amongst the more notable ideas to have emerged from these inquiries has been the suggestion that, as more and more 3D printers start to enter people's homes, so the conventional relationship between the home and the workplace might get further eroded.<ref name="AutoSQ-105" /> Likewise, it has also been suggested that, as it becomes easier for businesses to transmit designs for new objects around the globe, so the need for high-speed freight services might also become less.<ref name="AutoSQ-106" /> Finally, given the ease with which certain objects can now be replicated, it remains to be seen whether changes will be made to current copyright legislation so as to protect intellectual property rights with the new technology widely available. |
|||
As 3D printers became more accessible to consumers, online social platforms have developed to support the community.<ref name="AutoSQ-107" /> This includes websites that allow users to access information such as how to build a 3D printer, as well as social forums that discuss how to improve 3D print quality and discuss 3D printing news, as well as social media websites that are dedicated to share 3D models.<ref name="AutoSQ-108" /><ref name="AutoSQ-109" /><ref name="AutoSQ-110" /> RepRap is a wiki based website that was created to hold all information on 3d printing, and has developed into a community that aims to bring 3D printing to everyone. Furthermore, there are other sites such as [[Pinshape]], Thingiverse and MyMiniFactory, which was created initially to allow users to post 3D files for anyone to print, allowing for decreased transaction cost of sharing 3D files. These websites have allowed for greater social interaction between users, creating communities dedicated around 3D printing. |
|||
Some <ref name="triple-c.at" /><ref name="AutoSQ-111" /><ref name="AutoSQ-112" /> call attention to the conjunction of [[Commons-based peer production]] with 3D printing and other low-cost manufacturing techniques. The self-reinforced fantasy of a system of eternal growth can be overcome with the development of economies of scope, and here, the civil society can play an important role contributing to the raising of the whole productive structure to a higher plateau of more sustainable and customized productivity.<ref name="triple-c.at" /> Further, it is true that many issues, problems and threats rise due to the large democratization of the means of production, and especially regarding the physical ones.<ref name="triple-c.at" /> For instance, the recyclability of advanced nanomaterials is still questioned; weapons manufacturing could become easier; not to mention the implications on counterfeiting <ref name="AutoSQ-113" /> and on IP.<ref name="AutoSQ-114" /> It might be maintained that in contrast to the industrial paradigm whose competitive dynamics were about economies of scale, [[Commons-based peer production]] and 3D printing could develop economies of scope. While the advantages of scale rest on cheap global transportation, the economies of scope share infrastructure costs (intangible and tangible productive resources), taking advantage of the capabilities of the fabrication tools.<ref name="triple-c.at" /> And following Neil Gershenfeld <ref name="AutoSQ-115" /> in that "some of the least developed parts of the world need some of the most advanced technologies", Commons-based peer production and 3D printing may offer the necessary tools for thinking globally but act locally in response to certain problems and needs. |
|||
[[Larry Summers]] wrote about the "devastating consequences" of 3D printing and other technologies (robots, artificial intelligence, etc.) for those who perform routine tasks. In his view, "already there are more American men on disability insurance than doing production work in manufacturing. And the trends are all in the wrong direction, particularly for the less skilled, as the capacity of capital embodying artificial intelligence to replace white-collar as well as blue-collar work will increase rapidly in the years ahead." Summers recommends more vigorous cooperative efforts to address the "myriad devices" (e.g., tax havens, bank secrecy, money laundering, and regulatory arbitrage) enabling the holders of great wealth to "avoid paying" income and estate taxes, and to make it more difficult to accumulate great fortunes without requiring "great social contributions" in return, including: more vigorous enforcement of anti-monopoly laws, reductions in "excessive" protection for intellectual property, greater encouragement of profit-sharing schemes that may benefit workers and give them a stake in wealth accumulation, strengthening of collective bargaining arrangements, improvements in corporate governance, strengthening of financial regulation to eliminate subsidies to financial activity, easing of land-use restrictions that may cause the real estate of the rich to keep rising in value, better training for young people and retraining for displaced workers, and increased public and private investment in infrastructure development—e.g., in energy production and transportation.<ref name="AutoSQ-116" /> |
|||
[[Michael Spence]] wrote that "Now comes a … powerful, wave of digital technology that is replacing labor in increasingly complex tasks. This process of labor substitution and [[disintermediation]] has been underway for some time in service sectors—think of ATMs, online banking, enterprise resource planning, customer relationship management, mobile payment systems, and much more. This revolution is spreading to the production of goods, where robots and 3D printing are displacing labor." In his view, the vast majority of the cost of digital technologies comes at the start, in the design of hardware (e.g. 3D printers) and, more important, in creating the software that enables machines to carry out various tasks. "Once this is achieved, the marginal cost of the hardware is relatively low (and declines as scale rises), and the marginal cost of replicating the software is essentially zero. With a huge potential global market to amortize the upfront fixed costs of design and testing, the incentives to invest [in digital technologies] are compelling." Spence believes that, unlike prior digital technologies, which drove firms to deploy underutilized pools of valuable labor around the world, the motivating force in the current wave of digital technologies "is cost reduction via the replacement of labor." For example, as the cost of 3D printing technology declines, it is "easy to imagine" that production may become "extremely" local and customized. Moreover, production may occur in response to actual demand, not anticipated or forecast demand. Spence believes that labor, no matter how inexpensive, will become a less important asset for growth and employment expansion, with labor-intensive, process-oriented manufacturing becoming less effective, and that re-localization will appear in both developed and developing countries. In his view, production will not disappear, but it will be less labor-intensive, and all countries will eventually need to rebuild their growth models around digital technologies and the human capital supporting their deployment and expansion. Spence writes that "the world we are entering is one in which the most powerful global flows will be ideas and digital capital, not goods, services, and traditional capital. Adapting to this will require shifts in mindsets, policies, investments (especially in human capital), and quite possibly models of employment and distribution."<ref name="AutoSQ-117" /> |
|||
[[Forbes]] investment pundits have predicted that 3D printing may lead to a resurgence of American Manufacturing, citing the small, creative companies that comprise the current industry landscape, and the lack of the necessary complex infrastructure in typical outsource markets.<ref name="AutoSQ-118" /> |
|||
== See also == |
|||
{{Portal|Design}} |
|||
{{div col}} |
|||
* [[List of 3D printer manufacturers]] |
|||
* [[List of notable 3D printed weapons and parts]] |
|||
* [[List of common 3D test models]] |
|||
* [[List of emerging technologies]] |
|||
* [[AstroPrint]] |
|||
* [[3D printing marketplace]] |
|||
* [[Additive Manufacturing File Format]] |
|||
* [[Cloud manufacturing]] |
|||
* [[Computer numeric control]] |
|||
* [[Continuous Liquid Interface Production]] |
|||
* [[Digital modeling and fabrication]] |
|||
* [[3D printer extruder]] |
|||
* [[Fusion3]] |
|||
* [[Laser cutting]] |
|||
* [[MakerBot Industries]] |
|||
* [[Magnetically assisted slip casting]] |
|||
* [[Mass customization]] |
|||
* [[Milling center]] |
|||
* [[Modular design]] |
|||
* [[Molecular assembler]] |
|||
* [[Open design]] |
|||
* [[Open source hardware]] |
|||
* [[Organ-on-a-chip]] |
|||
* [[Self-replicating machine]] |
|||
* [[Shapeways]] |
|||
* [[Thingiverse]] |
|||
* [[Volumetric printing]] |
|||
{{div col end}} |
{{div col end}} |
||
==References== |
== References == |
||
{{ |
{{reflist|30em|refs= |
||
<ref name="reprap heritage">{{cite web |title=The RepRap's Heritage|url=http://www.fabbaloo.com/blog/2011/6/15/the-repraps-heritage.html}}</ref> |
|||
<ref name="engineer">{{cite web |last=Excell |first=Jon |url=http://www.theengineer.co.uk/in-depth/the-big-story/the-rise-of-additive-manufacturing/1002560.article |title=The rise of additive manufacturing |work=The Engineer |accessdate=2013-10-30}}</ref> |
|||
<ref name="Auto3D-1">{{cite web |url=http://www.createitreal.com/index.php/en/3d-printer/48 |title=3D Printer Technology – Animation of layering |publisher=Create It Real |accessdate=2012-01-31}}</ref> |
|||
<ref name="3D opp">{{cite news |url=http://www.ft.com/cms/s/0/6dc11070-d763-11e1-a378-00144feabdc0.html#axzz24gFn5Cal |title=Exploring the 3D printing opportunity |work=[[The Financial Times]] |author=Jane Bird |date=2012-08-08 |accessdate=2012-08-30}}</ref> |
|||
<ref name="AutoSQ-1">{{cite news |url=http://www.pcmag.com/slideshow_viewer/0,3253,l=293816&a=289174&po=1,00.asp |title=3D Printing: What You Need to Know |publisher=PCMag.com |accessdate=2013-10-30}}</ref> |
|||
<ref name="Zelinski_2014-06-25">{{cite news |last=Zelinski |first=Peter |date=2014-06-25 |title=Video: World's largest additive metal manufacturing plant |journal=[[Modern Machine Shop]] |url=http://www.mmsonline.com/blog/post/video-worlds-largest-additive-metal-manufacturing-plant}}</ref> |
|||
<ref name="Auto3D-2">{{cite web |url=http://www.ptonline.com/articles/200408cu3.html |title=3D Printers Lead Growth of Rapid Prototyping (Plastics Technology, August 2004) |first=Lilli Manolis |last=Sherman |accessdate=2012-01-31}}</ref> |
|||
<ref name="AutoSQ-2">{{cite news |url=http://www.economist.com/news/technology-quarterly/21584447-digital-manufacturing-there-lot-hype-around-3d-printing-it-fast |title=3D printing: 3D printing scales up |work=The Economist |date=2013-09-07 |accessdate=2013-10-30}}</ref> |
|||
<ref name="AutoSQ-3">{{cite news |first=Heather |last=Kelly |title=Study: At-home 3D printing could save consumers "thousands" |publisher=CNN |url=http://whatsnext.blogs.cnn.com/2013/07/31/study-at-home-3-d-printing-could-save-consumers-thousands/ |date=July 31, 2013}}</ref> |
|||
<ref name="wittbrodt">{{cite journal |last1=Wittbrodt |first1=B. T. |last2=Glover |first2=A. G. |last3=Laureto |first3=J. |last4=Anzalone |first4=G. C. |last5=Oppliger |first5=D. |last6=Irwin |first6=J. L. |last7=Pearce |first7=J. M. |doi=10.1016/j.mechatronics.2013.06.002 |title=Life-cycle economic analysis of distributed manufacturing with open-source 3-D printers |journal=Mechatronics |volume=23 |issue=6 |pages=713 |year=2013}}</ref> |
|||
<ref name="AutoSQ-4">{{US patent|4575330|Apparatus for Production of Three-Dimensional Objects by Stereolithography (8 August 1984)}}</ref> |
|||
<ref name="AutoSQ-5">Freedman, David H. "Layer By Layer." ''Technology Review'' 115.1 (2012): 50–53. Academic Search Premier. Web. 26 July 2013.</ref> |
|||
<ref name="Ngram_additive_manufacturing">{{cite web |title=Google Ngram of the term additive manufacturing |url=https://books.google.com/ngrams/graph?content=additive+manufacturing&year_start=1940&year_end=2014&corpus=15&smoothing=3&share=&direct_url=t1%3B%2Cadditive%20manufacturing%3B%2Cc0}}</ref> |
|||
<ref name="GrabCAD_GE_bracket">{{ citation |author=GrabCAD |title=GE jet engine bracket challenge |url=http://grabcad.com/challenges/ge-jet-engine-bracket-challenge}}</ref> |
|||
<ref name="AutoSQ-6">{{ citation |last=Zelinski |first=Peter |date=2014-06-02 |title=How do you make a howitzer less heavy? |journal=[[Modern Machine Shop]] |url=http://www.mmsonline.com/blog/post/how-do-you-make-a-howitzer-less-heavy}}</ref> |
|||
<ref name="Auto3D-17">{{cite web |url=http://www.ops-uk.com/3d-printers/objet-connex |title=Objet Connex 3D Printers |publisher=Objet Printer Solutions |accessdate=2012-01-31}}</ref> |
|||
<ref name=smooth>[http://machinedesign.com/3d-printing/how-smooth-3d-printed-parts Frick, Lindsey. How to Smooth 3D-Printed Parts. Machine Design Magazine, 29 April 2014]</ref> |
|||
<!-- |
|||
<ref name="Auto3D-6">D. T. Pham, S. S. Dimov, Rapid manufacturing, Springer-Verlag, 2001, ISBN 978-1-85233-360-7, page 6</ref> |
|||
--> |
|||
<ref name="Auto3D-7">{{cite news |url=http://www.economist.com/theworldin/displaystory.cfm?story_id=10105016 |title=A whole new dimension – Rich homes can afford 3D printers |work=The Economist |date=November 15, 2007 |first=Lilli Manolis |last=Sherman}}</ref> |
|||
<ref name="Auto3D-8">{{cite web |url=http://wohlersassociates.com/NovDec05TCT3dp.htm |title=Factors to Consider When Choosing a 3D Printer (WohlersAssociates.com, Nov/Dec 2005) |first=Terry |last=Wohlers}}</ref> |
|||
<ref name="Auto3D-9">{{cite web |author=www.3ders.org |url=http://www.3ders.org/articles/20120925-casting-aluminum-parts-directly-from-3d-printed-pla-parts.html |title=Casting aluminum parts directly from 3D printed PLA parts |publisher=3ders.org |date=2012-09-25 |accessdate=2013-10-30}}</ref> |
|||
<ref name="AutoSQ-14">{{cite web |url=http://www.blueprinter.dk/shs.html |title=Affordable 3D Printing with new Selective Heat Sintering (SHS™) technology |publisher=blueprinter}}</ref> |
|||
<ref name="Auto3D-10">{{cite book |title=Rapid Prototyping |author=Chee Kai Chua |author2=Kah Fai Leong |author3=Chu Sing Lim |date=2003 |publisher=World Scientific |isbn=978-981-238-117-0 |page=124 |url=https://books.google.com/?id=hpNT01xw4EEC&pg=PA124&dq=Stratasys}}</ref> |
|||
<ref name=DMLS>[http://machinedesign.com/metals/aluminum-powder-3d-printed-part-finishes-race-first Frick, Lindsey. Aluminum-powder DMLS-printed part finishes race first. Machine Design Magazine, 3 March 2014]</ref> |
|||
<ref name="Auto3D-3">Deckard, C., "Method and apparatus for producing parts by selective sintering", {{US patent|4863538}}, filed October 17, 1986, published September 5, 1989.</ref> |
|||
<ref name="Auto3D-4">Housholder, R., "Molding Process", {{US patent|4247508}}, filed December 3, 1979, published January 27, 1981.</ref> |
|||
<ref name="Auto3D-12">{{cite web |url = http://www.eetimes.com/design/industrial-control/4013703/Rapid-prototypes-move-to-metal-components |title = Rapid prototypes move to metal components (EE Times, 3/9/2007) |first = Joe |last = Hiemenz |accessdate =}}</ref> |
|||
<ref name="Auto3D-13">{{cite web |url=http://www.smu.edu/Lyle/Departments/ME/Research/CLAM/Research/Rapid_Manufacturing |title=Rapid Manufacturing by Electron Beam Melting |publisher=SMU.edu |accessdate=}}</ref> |
|||
<ref name="AutoSQ-15">[http://www.rapidtoday.com/mcor.html "3D Printer Uses Standard Paper", "Rapid Today", May, 2008]</ref> |
|||
<ref name="AutoSQ-16">{{US patent|4575330}}</ref> |
|||
<ref name="AutoSQ-17">[http://image.eastmanhouse.org/files/GEH_1958_07_05.pdf Beaumont Newhall (May 1958) "Photosculpture," ''Image'', '''7''' (5) : 100–105]</ref> |
|||
<ref name="AutoSQ-18">François Willème, "Photo-sculpture," U.S. Patent no. 43,822 (August 9, 1864). Available on-line at: {{US patent|43822}}</ref> |
|||
<ref name="AutoSQ-19">François Willème (May 15, 1861) "La sculpture photographique", ''Le Moniteur de la photographie'', p. 34.</ref> |
|||
<ref name="NSF RP Report">[http://www.wtec.org/pdf/rp_vi.pdf NSF JTEC/WTEC Panel Report-RPA]</ref> |
|||
<ref name="Auto3D-14">{{cite web |url=http://envisiontec.com/products/perfactory-4-standard-with-erm/ |title=EnvisionTEC Perfactory |publisher=EnvisionTEC}}</ref> |
|||
<ref name="Auto3D-15">{{cite web |url=http://www.eetimes.com/news/semi/showArticle.jhtml?articleID=198701422 |title=Cheaper avenue to 65 nm? (EE Times, 3/30/2007) |first=R. Colin |last=Johnson}}</ref> |
|||
<ref name="Auto3D-16">{{cite web |url=http://amt.tuwien.ac.at/projekte/micro_printer |title=The World's Smallest 3D Printer |date=12 September 2011 |publisher=[[Vienna University of Technology|TU Wien]]}}</ref> |
|||
<ref name="k1113">{{cite web |url=http://www.kurzweilai.net/3d-printing-multi-material-objects-in-minutes-instead-of-hours-to-minutes |title=3D-printing multi-material objects in minutes instead of hours |date=November 22, 2013 |publisher=Kurzweil Accelerating Intelligence}}</ref> |
|||
<ref name="cfr2013">{{cite web|url= http://www.cfr.org/technology-and-science/3d-printing-challenges-opportunities-international-relations/p31709 |title= 3D Printing: Challenges and Opportunities for International Relations |date=October 23, 2013 |publisher=[[Council on Foreign Relations]] |accessdate= 2013-10-30}}</ref> |
|||
<ref name="Auto3D-26">{{cite web |url=http://www.npr.org/templates/story/story.php?storyId=131644649 |title=A Space For DIY People To Do Their Business (NPR.org, November 28, 2010) |first=Jon |last=Kalish |accessdate=2012-01-31}}</ref> |
|||
<ref name="AutoSQ-20">Jones, R., Haufe, P., Sells, E., Iravani, P., Olliver, V., Palmer, C., & Bowyer, A. (2011). Reprap-- the replicating rapid prototyper. Robotica, 29(1), 177-191.</ref> |
|||
<ref name="AutoSQ-21">{{cite web |url=http://www.computerworld.co.nz/article/495672/open_source_3d_printer_copies_itself/ |title=Open source 3D printer copies itself |publisher=Computerworld New Zealand |date=2008-04-07 |accessdate=2013-10-30}}</ref> |
|||
<ref name="AutoSQ-22">[http://blog.reprap.org/2009/04/first-reprapped-circuit.html RepRap blog 2009 visited 2/26/2014]</ref> |
|||
<ref name="AutoSQ-23">[http://www.nytimes.com/2013/12/10/science/an-inexpensive-way-to-print-out-metal-parts.html?_r=0 An Inexpensive Way to Print Out Metal Parts – The New York Times]</ref> |
|||
<ref name="AutoSQ-24">[http://ieeexplore.ieee.org/xpl/articleDetails.jsp?tp=&arnumber=6678531&queryText%3Dopen+source+3d+metal+printer Gerald C. Anzalone, Chenlong Zhang, Bas Wijnen, Paul G. Sanders and Joshua M. Pearce, " Low-Cost Open-Source 3-D Metal Printing" ''IEEE Access'', 1, pp.803–810, (2013). doi: 10.1109/ACCESS.2013.2293018]</ref> |
|||
<ref name="Auto3D-27">{{cite journal |url=http://www.ccsenet.org/journal/index.php/jsd/article/view/6984 |title=3-D Printing of Open Source Appropriate Technologies for Self-Directed Sustainable Development |journal=Journal of Sustainable Development |volume=3 |number=4 |date=2010 |pages=17–29 |first=Joshua M. |last=Pearce |accessdate=2012-01-31 |doi=10.5539/jsd.v3n4p17|display-authors=etal}}</ref> |
|||
<ref name="AutoSQ-25">[http://techfortrade.org/our-initiatives/3d4d-challenge/ Tech for Trade, 3D4D Challenge]</ref> |
|||
<ref name="AutoSQ-26">[http://bits.blogs.nytimes.com/2013/02/17/disruptions-3-d-printing-is-on-the-fast-track/?nl=todaysheadlines&emc=edit_th_20130218 Disruptions: 3-D Printing Is on the Fast Track – NYTimes.com]</ref> |
|||
<ref name="printerlist">{{cite web |author=www.3ders.org |url=http://www.3ders.org/pricecompare/3dprinters/ |title=3D printers list with prices |publisher=3ders.org |accessdate=2013-10-30}}</ref> |
|||
<ref name="fabathome">[http://www.newscientist.com/article/dn10922-desktop-fabricator-may-kickstart-home-revolution.html New Scientist magazine: Desktop fabricator may kick-start home revolution, 9 January 2007]</ref> |
|||
<ref name="AutoSQ-27">{{cite news |title=3D printer by Saskatchewan man gets record crowdsourced cash |date=6 November 2013 |url=http://www.cbc.ca/news/canada/saskatchewan/3d-printer-by-sask-man-gets-record-crowdsourced-cash-1.2417416 |location=Saskatchewan |publisher=CBC News |accessdate=8 November 2013}}</ref> |
|||
<ref name="AutoSQ-28">{{cite news |title=Rapide One – Affordable Professional Desktop 3D Printer by Rapide 3D |date=December 2, 2013 |url=http://www.indiegogo.com/projects/rapide-one-affordable-professional-desktop-3d-printer-by-rapide-3d/x/2711116 |publisher=Indiegogo |accessdate=20 January 2014}}</ref> |
|||
<ref name="AutoSQ-29">[https://www.yahoo.com/tech/kickstarter-review-the-3doodler-pen-70978316285.html Pogue, David. "A Review Of The 3Doodler Pen, Which Raised Over $2 Million On Kickstarter". Yahoo Tech. Retrieved 13 March 2014]</ref> |
|||
<ref name="AutoSQ-30">[http://singularityhub.com/2013/02/27/kickstarter-3doodler-3d-printing-pen-nothing-of-the-sort-but-somehow-raises-2-million/ Dorrier, Jason. "Kickstarter 3Doodler 3D Printing Pen Nothing of the Sort – But Somehow Raises $2 Million". Singularity Hub. Retrieved 13 March 2014]</ref> |
|||
<ref name="AutoSQ-31">{{cite journal |last1=Kreiger |first1=M. |last2=Pearce |first2=J. M. |doi=10.1021/sc400093k |title=Environmental Life Cycle Analysis of Distributed Three-Dimensional Printing and Conventional Manufacturing of Polymer Products |journal=ACS Sustainable Chemistry & Engineering |pages=131002082320002 |year=2013}}</ref> |
|||
<ref name="RPJ">[http://www.academia.edu/2643418/Distributed_Recycling_of_Waste_Polymer_into_RepRap_Feedstock Christian Baechler, Matthew DeVuono, and Joshua M. Pearce, "Distributed Recycling of Waste Polymer into RepRap Feedstock". ''Rapid Prototyping Journal,'' '''19''' (2), pp. 118-125 (2013). DOI:10.1108/13552541311302978]</ref> |
|||
<ref name="AutoSQ-32">[http://www.academia.edu/2921972/Distributed_Recycling_of_Post-Consumer_Plastic_Waste_in_Rural_Areas Kreiger, M., Anzalone, G. C., Mulder, M. L., Glover, A., & Pearce, J. M. (2013). Distributed Recycling of Post-Consumer Plastic Waste in Rural Areas. MRS Online Proceedings Library, 1492, mrsf12-1492]</ref> |
|||
<ref name="AutoQK-1">[http://www.thingiverse.com/thing:17175 See for example the Rostock]</ref> |
|||
<ref name="AutoSQ-33">{{cite web |last=Vandendriessche |first=Pieter-Jan |title=delta 3D printer accuracy |url=http://www.tripodmaker.com/tripodmaker-3d-printing-accuracy/}}</ref> |
|||
<ref name="MatterHackers Opens 3D Printing Store and Releases MatterControl 0.7.6">{{cite web |url=http://www.3dprinterworld.com/article/matterhackers-opens-3d-printing-store-and-releases-mattercontrol-076 |title=MatterHackers Opens 3D Printing Store and Releases MatterControl 0.7.6 |date=July 11, 2013 |accessdate=November 30, 2013 |author=Titsch, Mike}}</ref> |
|||
<ref name="Auto3D-18">{{cite news |url=http://www.economist.com/node/18114327?story_id=18114327 |title=Print me a Stradivarius – How a new manufacturing technology will change the world |publisher=Economist Technology |accessdate=2012-01-31 |date=2011-02-10}}</ref> |
|||
<ref name="TMW_2011-02_Origins">{{harvnb|Vincent|Earls|2011}}</ref> |
|||
<ref name="Auto3D-19">Wohlers Report 2009, State of the Industry Annual Worldwide Progress Report on Additive Manufacturing, Wohlers Associates, ISBN 978-0-9754429-5-1</ref> |
|||
<ref name="Auto3D-20">Hopkinson, N & Dickens, P 2006, 'Emerging Rapid Manufacturing Processes', in Rapid Manufacturing; An industrial revolution for the digital age, Wiley & Sons Ltd, Chichester, W. Sussex</ref> |
|||
<ref name="AutoQK-2">{{cite web |url=http://makie.me |title=The action doll you designed, made real |publisher=makie.me |accessdate=January 18, 2013}}</ref> |
|||
<ref name="AutoQK-3">{{cite web |url=http://www.myrobotnation.com/ |title=Cubify — Express Yourself in 3D |publisher=myrobotnation.com |accessdate=2014-01-25}}</ref> |
|||
<ref name="AutoSQ-34">{{cite journal|title=Turn Your Baby's Cry Into an iPhone Case |url=http://www.businessweek.com/articles/2012-03-10/turn-your-babys-cry-into-an-iphone-case |work=[[Bloomberg Businessweek]] |date=2012-03-10 |accessdate=2013-02-20 |ref=harv}}</ref> |
|||
<ref name="AutoSQ-35">{{cite journal|title=Nokia backs 3D printing for mobile phone cases |url=http://www.bbc.co.uk/news/technology-21084430 |publisher=[[BBC News Online]] |date=2013-02-18 |accessdate=2013-02-20 |ref=harv}}</ref> |
|||
<ref name="AutoSQ-36">{{cite web |url=http://www.businessinsider.com/koenigsegg-one1-comes-with-3d-printed-parts-2014-2 |title=Koenigsegg One:1 Comes With 3D Printed Parts |work=[[Business Insider]] |accessdate=2014-05-14}}</ref> |
|||
<ref name="Auto3D-21">{{cite web |author=ewilhelm |url=http://www.instructables.com/id/3D-Printed-Clock-and-Gears/ |title=3D printed clock and gears |publisher=Instructables.com |accessdate=2013-10-30}}</ref> |
|||
==Further reading== |
|||
<ref name="Auto3D-23">{{cite web |author=23/01/2012 |url=http://3d-printer-kit.com/?p=565 |title=Successful Sumpod 3D printing of a herringbone gear |publisher=3d-printer-kit.com |date=2012-01-23 |accessdate=2013-10-30}}</ref> |
|||
{{Refbegin}} |
|||
<ref name="AutoSQ-42">{{cite web |url=http://mashable.com/2013/07/02/3d-printed-duck-foot |title=3D-Printed Foot Lets Crippled Duck Walk Again}}</ref> |
|||
* {{cite book|first=Govind|last=Agrawal|title=Fiber-Optic Communication Systems|publisher=Wiley|year=2010|isbn=9780470505113|doi=10.1002/9780470918524|edition=4}} |
|||
<ref name="AutoSQ-43">{{cite news |url=http://www.wired.com/design/2013/07/3-d-printed-hermit-crab-shells-based-on-city-skylines |title=So Cute: Hermit Crabs Strut in Stylish 3-D Printed Shells |work=Wired |first=Joseph |last=Flaherty |date=2013-07-30}}</ref> |
|||
* {{cite journal | last1 = Gambling | first1 = W. A. | year = 2000 | title = The Rise and Rise of Optical Fibers | url = | journal = IEEE Journal on Selected Topics in Quantum Electronics | volume = 6 | issue = 6| pages = 1084–1093 | doi = 10.1109/2944.902157 }} |
|||
<ref name="chem">{{cite journal |last1=Symes |first1=M. D. |last2=Kitson |first2=P. J. |last3=Yan |first3=J. |last4=Richmond |first4=C. J. |last5=Cooper |first5=G. J. T. |last6=Bowman |first6=R. W. |last7=Vilbrandt |first7=T. |last8=Cronin |first8=L. |doi=10.1038/nchem.1313 |title=Integrated 3D-printed reactionware for chemical synthesis and analysis |journal=Nature Chemistry |volume=4 |issue=5 |pages=349–354 |year=2012 |pmid= 22522253|pmc=}}</ref> |
|||
* Mirabito, Michael M.A; and Morgenstern, Barbara L., ''The New Communications Technologies: Applications, Policy, and Impact'', 5th. Edition. Focal Press, 2004. (ISBN 0-24-080586-0). |
|||
<ref name="Auto3D-25">[http://www.newscientist.com/article/dn21709-make-your-own-drugs-with-a-3d-printer.html?full=true New Scientist magazine: Make your own drugs with a 3D printer, 17 April 2012]</ref> |
|||
* Mitschke F., ''Fiber Optics – Physics and Technology'', Springer, 2009 (ISBN 978-3-642-03702-3) |
|||
<ref name="BBC_2012-04-17">{{cite news |last=Cronin |first=Lee |title=3D printer developed for drugs |url=http://www.bbc.co.uk/news/uk-scotland-17744314 |publisher=[[BBC News Online]] |format=video interview [5:21] |location=[[Glasgow University]] |date=2012-04-17 |accessdate=2013-03-06}}</ref> |
|||
* Nagel S. R., MacChesney J. B., Walker K. L., "An Overview of the Modified Chemical Vapor Deposition (MCVD) Process and Performance", ''IEEE Journal of Quantum Electronics'', Vol. QE-18, No. 4, p. 459, April 1982. {{doi|10.1109/TMTT.1982.1131071}} |
|||
<ref name="AutoSQ-44">{{cite web |url=http://www.gizmag.com/openreflex-3d-printed-slr/28208/ |title=3D printable SLR brings whole new meaning to "digital camera" |publisher=Gizmag.com |accessdate=2013-10-30}}</ref> |
|||
* {{cite book|author1=Rajiv Ramaswami|author2=Kumar Sivarajan|author3=Galen Sasaki|title=Optical Networks: A Practical Perspective|url={{google books|plainurl=yes|id=WpByp4Ip0z8C}}|date=27 November 2009|publisher=Morgan Kaufmann|isbn=978-0-08-092072-6}} |
|||
<ref name="resins-online.com">{{cite web |url=http://www.resins-online.com/blog/3d-printed-clothing/ |title=3D Printed Clothing Becoming a Reality |publisher=Resins Online |date=2013-06-17 |accessdate=2013-10-30}}</ref> |
|||
* VDV Works LLC [http://www.vdvworks.com/LennieLw ''Lennie Lightwave's Guide To Fiber Optics''], © 2002-6. |
|||
<ref name="AutoSQ-45">{{cite web |author=Michael Fitzgerald |url=http://sloanreview.mit.edu/article/with-3-d-printing-the-shoe-really-fits/ |title=With 3-D Printing, the Shoe Really Fits |publisher=MIT Sloan Management Review |date=2013-05-28 |accessdate=2013-10-30}}</ref> |
|||
* {{cite book |first=Thomas L. |last=Friedman |title=The World is Flat |publisher=Picador |year=2007 |isbn=978-0-312-42507-4}} The book discusses how fiberoptics has contributed to [[globalization]], and has revolutionized communications, business, and even the distribution of capital among countries. |
|||
<ref name="Forbes.com">{{cite web |url=http://www.forbes.com/sites/rakeshsharma/2013/09/10/custom-eyewear-the-next-focal-point-for-3d-printing/ |title=3D Custom Eyewear The Next Focal Point For 3D Printing |publisher=Rakesh Sharma |date=2013-09-10 |accessdate=2013-09-10}}</ref> |
|||
* [http://telecom-info.telcordia.com/site-cgi/ido/docs.cgi?ID=SEARCH&DOCUMENT=GR-771& GR-771, ''Generic Requirements for Fiber Optic Splice Closures''], Telcordia Technologies, Issue 2, July 2008. Discusses fiber optic splice closures and the associated hardware intended to restore the mechanical and environmental integrity of one or more fiber cables entering the enclosure. |
|||
<ref name="Auto3D-38">[http://www.bbc.com/news/technology-18677627 "3D-printed sugar network to help grow artificial liver", BBC, 2 July 2012]</ref> |
|||
* {{cite web|last=Paschotta|first=Rüdiger| title=Tutorial on Passive Fiber optics| url=https://www.rp-photonics.com/passive_fiber_optics.html| publisher=RP Photonics| accessdate=17 October 2013}} |
|||
<ref name="AutoSQ-46">{{cite web |url=http://lifescientist.com.au/content/biotechnology/news/invetech-helps-bring-bio-printers-to-life-413047968 |title=Invetech helps bring bio-printers to life |date=December 11, 2009 |accessdate=December 31, 2013|website=Australian Life Scientist |publisher=[[Westwick-Farrow Media]]}}</ref> |
|||
{{Refend}} |
|||
<ref name="Auto3D-39">[http://www.theengineer.co.uk/in-depth/analysis/building-body-parts-with-3d-printing/1002542.article "Building body parts with 3D printing", The Engineer, 24 May 2010]</ref> |
|||
<ref name="Auto3D-40">{{cite web |url=http://abcnews.go.com/Technology/story?id=1603783&page=1 |title='Organ Printing' Could Drastically Change Medicine (ABC News, 2006) |first=Jonathan |last=Silverstein |accessdate=2012-01-31}}</ref> |
|||
<ref name="AutoSQ-47">{{cite news |url=http://www.bbc.com/news/technology-16907104|agency=BBC |date=2012-02-06 |title=Transplant jaw made by 3D printer claimed as first}}</ref> |
|||
<ref name="AutoSQ-48">{{cite news |url=http://www.npr.org/blogs/health/2014/03/17/289042381/doctors-use-3-d-printing-to-help-a-baby-breathe|agency=NPR |date=2013-03-17 |author=Rob Stein |title=Doctors Use 3-D Printing To Help A Baby Breathe}}</ref> |
|||
<ref name="AutoSQ-49">{{cite web |url=http://www.fiercemedicaldevices.com/story/surgeons-have-implanted-3-d-printed-pelvis-uk-cancer-patient/2014-02-11 |title=Surgeons have implanted a 3-D-printed pelvis into a U.K. cancer patient |author=Moore, Calen |publisher=fiercemedicaldevices.com |date=11 February 2014 |accessdate=4 March 2014}}</ref> |
|||
<ref name="AutoSQ-50">{{cite news |url=http://www.telegraph.co.uk/health/10691753/Man-makes-surgical-history-after-having-his-shattered-face-rebuilt-using-3D-printed-parts.html/2014-03-12 |title=Man makes surgical history after having his shattered face rebuilt using 3D printed parts |author=Keith Perry |publisher=The Daily Telegraph |date=12 March 2014 |accessdate=12 March 2014|location=London}}</ref> |
|||
<ref name="AutoSQ-51">[http://www.3ders.org/articles/20140306-research-into-3d-bioprinting-may-soon-produce-transplantable-human-tissues.html Research into Julie Williams, 3D-Bioprinting may soon produce transplantable human tissues Mar.6, 2014]</ref> |
|||
<ref name="f20120823">{{cite news |last=Greenberg |first=Andy |title='Wiki Weapon Project' Aims To Create A Gun Anyone Can 3D-Print At Home |url=http://www.forbes.com/sites/andygreenberg/2012/08/23/wiki-weapon-project-aims-to-create-a-gun-anyone-can-3d-print-at-home/ |publisher=[[Forbes]] |date=2012-08-23 |accessdate=2012-08-27}}</ref> |
|||
<ref name="pcm20120824">{{cite news |last=Poeter |first=Damon |title=Could a 'Printable Gun' Change the World? |url=http://www.pcmag.com/article2/0,2817,2408899,00.asp |publisher=[[PC Magazine]] |date=2012-08-24 |accessdate=2012-08-27}}</ref> |
|||
<ref name="AutoSQ-53">{{cite web |url=http://www.statesman.com/news/news/blueprints-for-3-d-printer-gun-pulled-off-website/nXnbG/ |title=Blueprints for 3-D printer gun pulled off website |publisher=statesman.com |date=May 2013 |accessdate=2013-10-30}}</ref> |
|||
<ref name="AutoSQ-54">{{cite web |first=Aaron |last=Samsel |url=http://www.guns.com/2013/05/23/3d-printers-meet-othermill-a-cnc-machine-for-your-home-office/ |title=3D Printers, Meet Othermill: A CNC machine for your home office (VIDEO) |publisher=Guns.com |accessdate=2013-10-30}}</ref> |
|||
<ref name="AutoSQ-55">{{cite web |url=http://www.popehat.com/2011/10/06/the-third-wave-cnc-stereolithography-and-the-end-of-gun-control/ |title=The Third Wave, CNC, Stereolithography, and the end of gun control |publisher=Popehat |accessdate=2013-10-30}}</ref> |
|||
<ref name="AutoSQ-56">{{cite news |title=Weapons made with 3-D printers could test gun-control efforts |url=http://www.washingtonpost.com/local/weapons-made-with-3-d-printers-could-test-gun-control-efforts/2013/02/18/9ad8b45e-779b-11e2-95e4-6148e45d7adb_story.html?hpid=z1|newspaper=Washington Post |first=Michael S. |last=Rosenwald |date=2013-02-25}}</ref> |
|||
<ref name="AutoSQ-57">{{cite news |url=http://www.economist.com/news/united-states/21571910-regulatory-and-legal-challenges-posed-3d-printing-gun-parts-ready-print-fire |title=Making guns at home: Ready, print, fire |publisher=The Economist |date=2013-02-16 |accessdate=2013-10-30}}</ref> |
|||
<ref name="AutoSQ-58">{{cite news |last=Rayner |first=Alex |title=3D-printable guns are just the start, says Cody Wilson |url=http://www.guardian.co.uk/world/shortcuts/2013/may/06/3d-printable-guns-cody-wilson|newspaper=The Guardian |date=6 May 2013|location=London}}</ref> |
|||
<ref name="AutoSQ-59">{{cite web |last=Manjoo |first=Farhad |url=http://www.slate.com/articles/technology/technology/2013/05/_3_d_printed_gun_yes_it_will_be_possible_to_make_weapons_with_3_d_printers.single.html |title=3-D-printed gun: Yes, it will be possible to make weapons with 3-D printers. No, that doesn't make gun control futile |publisher=Slate.com |date=2013-05-08 |accessdate=2013-10-30}}</ref> |
|||
<ref name="AutoSQ-60">[https://www.youtube.com/watch?v=_KBxG1_WO8k Students Use 3D Printing to Reconstruct Dinosaurs – YouTube]</ref> |
|||
<ref name="AutoSQ-61">[http://on3dprinting.com/2013/02/20/3d-printing-in-the-classroom-to-accelerate-adoption-of-technology/ 3D Printing in the Classroom to Accelerate Adoption of Technology. On 3D Printing]</ref> |
|||
<ref name="AutoSQ-62">[http://www.sciencedirect.com/science/article/pii/S0736585314000343 Kostakis, V.; Niaros, V.; Giotitsas, C. (2014): ''Open source 3D printing as a means of learning: An educational experiment in two high schools in Greece''. In: Telematics and Informatics]</ref> |
|||
<ref name="AutoSQ-63">{{cite journal |last=DiSalvo, C |title=Design and the Construction of Publics|journal=Design Issues |date=2009|volume=25|series=1 |page=48 |doi=10.1162/desi.2009.25.1.48}}</ref> |
|||
<ref name="AutoSQ-64">{{cite journal |author=Ratto, M.|author2=Ree, R.|last-author-amp=yes |title=Materializing information: 3D printing and social change.|journal=First Monday |date=2012|volume=17|issue=7}}</ref> |
|||
<ref name="AutoSQ-65">{{cite journal |last=Ratto |first=Matt |title=Open Design and Critical Making|journal=Open Design Now: Why Design Cannot Remain Exclusive |date=2011}}</ref> |
|||
<ref name="AutoSQ-66">{{cite journal |last=Lukens |first=Jonathan |title=Speculative Design and Technological Fluency |journal=International Journal of Learning and Media|volume=3|pages=23–39 |doi=10.1162/ijlm_a_00080}}</ref> |
|||
<!-- |
|||
<ref name="AutoSQ-67">{{cite news |title=New VLT component created using 3D printing |url=http://www.eso.org/public/images/ann14011a/ |accessdate=11 February 2014|newspaper=ESO Announcement}}</ref> |
|||
--> |
|||
<ref name="AutoQK-4">Pearce, Joshua M. 2012. "[http://www.sciencemag.org/content/337/6100/1303.summary Building Research Equipment with Free, Open-Source Hardware." ''Science'' '''337''' (6100): 1303–1304]</ref> |
|||
<ref name="AutoSQ-68">{{cite journal |last1=Zhang |first1=C. |last2=Anzalone |first2=N. C. |last3=Faria |first3=R. P. |last4=Pearce |first4=J. M. |editor1-last=De Brevern |editor1-first=Alexandre G |title=Open-Source 3D-Printable Optics Equipment |doi=10.1371/journal.pone.0059840 |journal=PLoS ONE |volume=8 |issue=3 |pages=e59840 |year=2013 |pmid= 23544104|pmc =3609802}}</ref> |
|||
<ref name="tc20120120">{{cite news |title=The World's First 3D-Printed Building Will Arrive In 2014 |url=http://techcrunch.com/2013/01/20/the-worlds-first-3d-printed-building-will-arrive-in-2014-and-it-looks-awesome/ |accessdate=2013-02-08|newspaper=TechCrunch |date=2012-01-20}}</ref> |
|||
<ref name="Auto3D-34">{{cite journal |last1=Séquin |first1=C. H. |title=Rapid prototyping |doi=10.1145/1064830.1064860 |journal=Communications of the ACM |volume=48 |issue=6 |pages=66 |year=2005 |pmid= |pmc=}}</ref> |
|||
<ref name="Auto3D-35">{{cite web |url=http://www.zcorp.com/documents/194_2007-1212-Wall%20Street%20Journal-3DP%20Turns%20Web%20World%20Real.pdf |title=How 3-D Printing Figures To Turn Web Worlds Real (The Wall Street Journal, December 12, 2007) |first=Robert A. |last=Guth |accessdate=2012-01-31}}</ref> |
|||
<ref name="Auto3D-36">{{cite news |author=iPad iPhone Android TIME TV Populist The Page |url=http://www.time.com/time/specials/2007/article/0,28804,1727737_1727680_1727556,00.html |title=''Bathsheba Grossman's Quin.MGX for Materialise'' listed in Time Magazine's Design 100 |publisher=Time.com |date=2008-04-03 |accessdate=2013-10-30}}</ref> |
|||
<ref name="Auto3D-37">{{cite news |url=http://www.independent.co.uk/arts-entertainment/art/features/object-lesson-how-the-world-of-decorative-art-is-being-revolutionised-by-3d-printing-2342500.html |title=Object lesson: How the world of decorative art is being revolutionised by 3D printing (The Independent, 28 August 2011) |last=Williams |first=Holly |accessdate=2012-01-31 |location=London |date=2011-08-28}}</ref> |
|||
<ref name="Auto3D-41">[http://creativemachines.cornell.edu/sites/default/files/SFF09_Cohen1_0.pdf "Hydrocolloid Printing", Cornell Creative, 2012]</ref> |
|||
<ref name="AutoSQ-71">[http://www.ted.com/talks/lee_cronin_print_your_own_medicine.html ted.com, Lee Cronin: Print your own medicine]</ref> |
|||
<ref name="AutoSQ-72">[http://www.businessweek.com/articles/2014-01-28/all-the-food-thats-fit-to-3d-print-from-chocolates-to-pizza A Guide to All the Food That's Fit to 3D Print (So Far)]</ref> |
|||
<ref name="Auto3D-42">{{cite journal |last1=Cignoni |first1=P. |last2=Scopigno |first2=R. |doi=10.1145/1367080.1367082 |title=Sampled 3D models for CH applications |journal=Journal on Computing and Cultural Heritage |volume=1 |pages=1 |year=2008 |pmid= |pmc=}}</ref> |
|||
<ref name="gizmodo20130131">{{cite news |last=Diaz |first=Jesus |title=This Is What the First Lunar Base Could Really Look Like |url=http://gizmodo.com/moon-base/ |accessdate=2013-02-01 |newspaper=Gizmodo |date=2013-01-31}}</ref> |
|||
<ref name="AutoSQ-73">{{cite news |last=Raval |first=Siddharth |title=SinterHab: A Moon Base Concept from Sintered 3D-Printed Lunar Dust |url=http://www.spacesafetymagazine.com/2013/03/29/sinterhab-3d-printed-moon-base-concept-lunar-dust/ |accessdate=2013-10-15 |newspaper=Space Safety Magazine |date=2013-03-29}}</ref> |
|||
<ref name="AutoSQ-74">{{Cite book |
|||
| last1 = Pandey | first1 = S. |
|||
| last2 = Gupta | first2 = B. |
|||
| last3 = Nahata | first3 = A. |
|||
| doi = 10.1364/CLEO_SI.2013.CTh1K.2 |
|||
| chapter = Complex Geometry Plasmonic Terahertz Waveguides Created via 3D Printing |
|||
| title = Cleo: 2013 |
|||
| pages = CTh1K.CTh12 |
|||
| year = 2013 |
|||
| isbn = 978-1-55752-972-5 |
|||
| pmid = |
|||
| pmc = |
|||
}}</ref> |
|||
<ref name="AutoSQ-76">{{cite web |author=The Diplomat |url=http://thediplomat.com/tech-biz/2013/08/15/chinese-scientists-are-3d-printing-ears-and-livers-with-living-tissue/ |title=Chinese Scientists Are 3D Printing Ears and Livers – With Living Tissue |work=Tech Biz |publisher=The Diplomat |date=2013-08-15 |accessdate=2013-10-30}}</ref> |
|||
<ref name="AutoSQ-77">{{cite web |url=http://www.3ders.org/articles/20130815-how-do-they-3d-print-kidney-in-china.html |title=How do they 3D print kidney in China |accessdate=2013-10-30}}</ref> |
|||
<ref name="AutoSQ-78">{{cite web |url=http://globaleconomicanalysis.blogspot.co.uk/2013/08/3d-printing-spare-human-parts-ears-and.html |title=Mish's Global Economic Trend Analysis: 3D-Printing Spare Human Parts; Ears and Jaws Already, Livers Coming Up ; Need an Organ? Just Print It |publisher=Globaleconomicanalysis.blogspot.co.uk |date=2013-08-18 |accessdate=2013-10-30}}</ref> |
|||
<ref name="AutoSQ-79">{{cite web |url=http://blogs.ptc.com/2013/08/01/underwater-city-3d-printed-reef-restores-bahrains-marine-life/ |title=Underwater City: 3D Printed Reef Restores Bahrain's Marine Life |publisher=ptc.com |date=2013-08-01 |accessdate=2013-10-30}}</ref> |
|||
<ref name="AutoSQ-80">{{cite web |url=http://www.techadvisor.co.uk/features/printing/how-3d-printing-is-helping-doctors-mend-you-better/ |title=How 3D printing is helping doctors mend you better |date=November 13, 2013 |last=Bennett |first=Neil |publisher=TechAdvisor}}</ref> |
|||
<ref name="AutoSQ-82">[http://www.patentinsightpro.com/techreports/0214/Tech%20Insight%20Report%20-%203D%20Printing.pdf 3D Printing Technology Insight Report, 2014, patent activity involving 3D-Printing from 1990–2013, accessed 2014-06-10]</ref> |
|||
<ref name="wired">[http://www.wired.com/design/2012/05/3-d-printing-patent-law/ Clive Thompson on 3-D Printing's Legal Morass. Wired, Clive Thompson 05.30.12 1:43 PM]</ref> |
|||
<ref name="deal">{{cite web |url=http://www.publicknowledge.org/files/What%27s%20the%20Deal%20with%20Copyright_%20Final%20version2.pdf |title=What's the Deal with copyright and 3D printing?|first= Michael |last=Weinberg |date=January 2013 |publisher=Institute for Emerging Innovation |format=PDF |accessdate=2013-10-30}}</ref> |
|||
<ref name="AutoSQ-83">{{cite news |url=http://www.foxnews.com/us/2013/05/23/govt-memo-warns-3d-printed-guns-may-be-impossible-to-stop/ |title=Homeland Security bulletin warns 3D-printed guns may be 'impossible' to stop |publisher=Fox News |date=2013-05-23 |accessdate=2013-10-30}}</ref> |
|||
<ref name="AutoSQ-84">{{cite web |last=Cochrane |first=Peter |url=http://www.techrepublic.com/blog/european-technology/peter-cochranes-blog-beyond-3d-printed-guns/1728 |title=Peter Cochrane's Blog: Beyond 3D Printed Guns |publisher=TechRepublic |date=2013-05-21 |accessdate=2013-10-30}}</ref> |
|||
<ref name="AutoSQ-85">{{cite web |last=Gilani |first=Nadia |url=http://metro.co.uk/2013/05/06/gun-factory-fears-as-3d-blueprints-available-online-3714514/ |title=Gun factory fears as 3D blueprints put online by Defense Distributed | Metro News |publisher=Metro.co.uk |date=2013-05-06 |accessdate=2013-10-30}}</ref> |
|||
<ref name="AutoSQ-86">{{cite web |url=http://digitaljournal.com/article/349588 |title=Liberator: First 3D-printed gun sparks gun control controversy |publisher=Digitaljournal.com |accessdate=2013-10-30}}</ref> |
|||
<ref name="AutoSQ-87">{{cite web |url=http://www.ibtimes.co.uk/articles/465236/20130507/3d-printed-gun-test-fire-defense-distributed.htm |title=First 3D Printed Gun 'The Liberator' Successfully Fired |publisher=IBTimes UK |date=2013-05-07 |accessdate=2013-10-30}}</ref> |
|||
<ref name="AutoSQ-88">{{cite web |url=http://www.neurope.eu/article/us-demands-removal-3d-printed-gun-blueprints |title=US demands removal of 3D printed gun blueprints |publisher=neurope.eu |accessdate=2013-10-30}}</ref> |
|||
<ref name="AutoSQ-89">{{cite web |url=http://economia.elpais.com/economia/2013/05/09/agencias/1368130430_552019.html |title=España y EE.UU. lideran las descargas de los planos de la pistola de impresión casera |publisher=ElPais.com |date=2013-05-09 |accessdate=2013-10-30}}</ref> |
|||
<ref name="AutoSQ-90">{{cite web |url=http://quietbabylon.com/2013/controlled-by-guns/ |title=Controlled by Guns |publisher=Quiet Babylon |date=2013-05-07 |accessdate=2013-10-30}}</ref> |
|||
<ref name="AutoSQ-91">{{cite web |url=http://www.joncamfield.com/tags/3dprinting |title=3dprinting |publisher=Joncamfield.com |accessdate=2013-10-30}}</ref> |
|||
<ref name="AutoSQ-92">{{cite web |url=http://news.antiwar.com/2013/05/10/state-dept-censors-3d-gun-plans-citing-national-security/ |title=State Dept Censors 3D Gun Plans, Citing 'National Security' |publisher=News.antiwar.com |date=2013-05-10 |accessdate=2013-10-30}}</ref> |
|||
<ref name="AutoSQ-93">{{cite web |url=http://reason.com/blog/2013/05/08/wishful-thinking-is-control-freaks-last |title=Wishful Thinking Is Control Freaks' Last Defense Against 3D-Printed Guns |publisher=Reason.com |date=2013-05-08 |accessdate=2013-10-30}}</ref> |
|||
<ref name="AutoSQ-94">{{cite web |last=Lennard |first=Natasha |url=http://www.salon.com/2013/05/10/the_pirate_bay_steps_in_to_distribute_3d_gun_designs/ |title=The Pirate Bay steps in to distribute 3-D gun designs |publisher=Salon.com |date=2013-05-10 |accessdate=2013-10-30 |archiveurl=http://www.webcitation.org/6GjdyItbX |archivedate=2013-05-19}}</ref> |
|||
<ref name="AutoSQ-95">{{cite web |url=http://sacramento.cbslocal.com/2013/05/08/sen-leland-yee-proposes-regulations-on-3-d-printers-after-gun-test/ |title=Sen. Leland Yee Proposes Regulating Guns From 3-D Printers |publisher=CBS Sacramento |date=2013-05-08 |accessdate=2013-10-30}}</ref> |
|||
<ref name="AutoSQ-96">[http://newyork.cbslocal.com/2013/05/05/schumer-announces-support-for-measure-to-make-3d-printed-guns-illegal Schumer Announces Support For Measure To Make 3D Printed Guns Illegal]</ref> |
|||
<ref name="AutoSQ-97">{{cite web |url=http://makezine.com/27/doctorow/ |title=Four Horsemen of the 3D Printing Apocalypse |publisher=Makezine.com |date=2011-06-30 |accessdate=2013-10-30}}</ref> |
|||
<ref name="AutoSQ-98">{{cite news |last=Ball |first=James |title=US government attempts to stifle 3D-printer gun designs will ultimately fail |url=http://www.guardian.co.uk/commentisfree/2013/may/10/3d-printing-gun-blueprint-state-department-ban |newspaper=The Guardian |date=10 May 2013 |location=London}}</ref> |
|||
<ref name="AutoSQ-99">{{cite web |author=Gadgets |url=http://techcrunch.com/2013/01/18/like-it-or-not-i-think-3d-printing-is-about-to-get-legislated/ |title=Like It Or Not, 3D Printing Will Probably Be Legislated |publisher=TechCrunch |date=2013-01-18 |accessdate=2013-10-30}}</ref> |
|||
<ref name="AutoSQ-100">{{cite web |first=Liz |last=Klimas |url=http://www.theblaze.com/stories/2013/02/19/engineer-dont-regulate-3d-printed-guns-regulate-explosive-gun-powder-instead/ |title=Engineer: Don't Regulate 3D Printed Guns, Regulate Explosive Gun Powder Instead |publisher=TheBlaze.com |date=2013-02-19 |accessdate=2013-10-30}}</ref> |
|||
<ref name="AutoSQ-101">{{cite news |last=Beckhusen |first=Robert |url=http://www.wired.com/dangerroom/2013/02/gunpowder-regulation/ |title=3-D Printing Pioneer Wants Government to Restrict Gunpowder, Not Printable Guns | Danger Room |publisher=Wired.com |date=2013-02-15 |accessdate=2013-10-30}}</ref> |
|||
<ref name="AutoSQ-102">{{cite web |url=http://www.theatlanticwire.com/technology/2013/05/how-defense-distributed-already-upended-world/65126/ |title=How Defense Distributed Already Upended the World |first=Philip |last=Bump |publisher=The Atlantic Wire |date=2013-05-10 |accessdate=2013-10-30 |archiveurl=http://www.webcitation.org/6GjdvfiAQ |archivedate=2013-05-19}}</ref> |
|||
<ref name="AutoSQ-103">{{cite web |url=http://www.europeanplasticsnews.com/subscriber/headlines2.html?cat=1&id=2961 |title=News |publisher=European Plastics News |accessdate=2013-10-30}}</ref> |
|||
<ref name="Albert_2011-02_MMS_column">{{harvnb|Albert|2011}}</ref> |
|||
<ref name="AutoSQ-104">{{cite web |title=Confronting a New 'Era of Duplication'? 3D Printing, Replicating Technology and the Search for Authenticity in George O. Smith's Venus Equilateral Series |url=http://www.academia.edu/4071685/Confronting_a_new_Era_of_Duplication_3D_Printing_Replicating_Technology_and_the_Search_for_Authenticity_in_George_O._Smiths_Venus_Equilateral_Series |publisher=Durham University |accessdate=July 21, 2013}}</ref> |
|||
<ref name="AutoSQ-105">{{cite web |title=Materializing information: 3D printing and social change |url=http://journals.uic.edu/ojs/index.php/fm/article/view/3968 |accessdate=January 13, 2014}}</ref> |
|||
<ref name="AutoSQ-106">{{cite web |title=Additive Manufacturing: A supply chain wide response to economic uncertainty and environmental sustainability |url=http://www.econolyst.co.uk/resources/documents/files/Paper%20-%20Oct%202008-%20AM%20a%20supply%20chain%20wide%20response.pdf |accessdate=January 11, 2014}}</ref> |
|||
<ref name="AutoSQ-107">{{cite web |title=Materializing information: 3D printing and social change |url=http://firstmonday.org/ojs/index.php/fm/article/view/3968/3273 |accessdate=March 30, 2014}}</ref> |
|||
<ref name="AutoSQ-108">{{cite web |title=RepRap Options |url=http://reprap.org/wiki/RepRap_Options |accessdate=March 30, 2014}}</ref> |
|||
<ref name="AutoSQ-109">{{cite web |title=3D Printing |url=http://www.reddit.com/r/3Dprinting/ |accessdate=March 30, 2014}}</ref> |
|||
<ref name="AutoSQ-110">{{cite web |title=Thingiverse |url=http://www.thingiverse.com/ |accessdate=March 30, 2014}}</ref> |
|||
<ref name="triple-c.at">Kostakis, V. (2013): ''[http://triple-c.at/index.php/tripleC/article/view/463 At the Turning Point of the Current Techno-Economic Paradigm: Commons-Based Peer Production, Desktop Manufacturing and the Role of Civil Society in the Perezian Framework.]''. In: TripleC, 11(1), 173–190.</ref> |
|||
<ref name="AutoSQ-111">Kostakis, V.; Papachristou, M. (2014): ''[http://www.sciencedirect.com/science/article/pii/S0736585313000609 Commons-based peer production and digital fabrication: The case of a RepRap-based, Lego-built 3D printing-milling machine]''. In: Telematics and Informatics, 31(3), 434–443</ref> |
|||
<ref name="AutoSQ-112">Kostakis, V; Fountouklis, M; Drechsler, W. (2013): ''[http://sth.sagepub.com/content/38/6/773 Peer Production and Desktop Manufacturing: The Case of the Helix-T Wind Turbine Project.]''. In: Science, Technology & Human Values, 38(6), 773 – 800.</ref> |
|||
<ref name="AutoSQ-113">Campbell, Thomas, Christopher Williams, Olga Ivanova, and Banning Garrett. (2011): ''[http://www.acus.org/publication/could-3d-printing-change-world Could 3D Printing Change the World? Technologies, Potential, and Implications of Additive Manufacturing]''. Washington: Atlantic Council of the United States</ref> |
|||
<ref name="AutoSQ-114">Bradshaw, Simon, Adrian Bowyer, and Patrick Haufe (2010): ''[http://opus.bath.ac.uk/18661/ The Intellectual Property Implications of Low-Cost 3D Printing]''. In: SCRIPTed 7</ref> |
|||
<ref name="AutoSQ-115">Gershenfeld, Neil (2007): ''FAB: The Coming Revolution on your Desktop: From Personal Computers to Personal Fabrication''. Cambridge: Basic Books, p. 13–14</ref> |
|||
<ref name="AutoSQ-116">[http://www.democracyjournal.org/32/the-inequality-puzzle.php?page=all Larry Summers, The Inequality Puzzle, ''Democracy: A Journal of Ideas'', Issue #32, Spring 2014]</ref> |
|||
<ref name="AutoSQ-117">[http://www.project-syndicate.org/commentary/michael-spence-describes-an-era-in-which-developing-countries-can-no-longer-rely-on-vast-numbers-of-cheap-workers Michael Spence, Labor's Digital Displacement (2014-05-22), ''Project Syndicate'']</ref> |
|||
<ref name="AutoSQ-118">[http://www.forbes.com/sites/rakeshsharma/2014/06/17/can-3d-printing-reshape-manufacturing-in-america/ Can 3D Printing Reshape Manufacturing In America?, Forbes.com 17 June 2014, retrieved 11 Aug 2014]</ref> |
|||
<ref name="Bopp2010">{{cite book |author=Felix Bopp |title=Future Business Models by Additive Manufacturing |url=https://books.google.com/books?id=M_SmmUOwsWwC |accessdate=4 July 2014 |date=2010 |publisher=Verlag |isbn=3-8366-8508-6}}</ref> |
|||
<ref name="AutoSQ-119">{{cite web |url=http://gizmodo.com/3d-hubs-like-airbnb-for-3d-printers-1207496555 |title=3D Hubs: Like Airbnb For 3D Printers |publisher=[[gizmodo]] |accessdate=2014-07-05}}</ref> |
|||
<ref name="Auto3D-28">{{cite news |url=http://www.wired.com/beyond_the_beyond/2011/06/spime-watch-dassault-systemes%E2%80%99-3dvia-and-sculpteo/ |title=Spime Watch: Dassault Systèmes' 3DVIA and Sculpteo (Reuters, June 27, 2011) |first=Bruce |last=Sterling |accessdate=2012-01-31 |date=2011-06-27 |archiveurl=http://uk.reuters.article.archive.joblink-inc.org/oldfiles/2011/06/spime-watch-dassault-systemes |archivedate=15 April 2014 |work=Wired}}</ref> |
|||
==External links== |
|||
<ref name="Auto3D-29">{{cite news |url=http://www.nytimes.com/2011/01/13/technology/personaltech/13basics.html?_r=1 |title=The Wow Factor of 3-D Printing (The New York Times, January 12, 2011) |first=Ashlee |last=Vance |accessdate=2012-01-31 |date=2011-01-12}}</ref> |
|||
{{Commons category|Optical fibers}} |
|||
<ref name=carbon-fiber>[http://machinedesign.com/3d-printing/markforged-5000-3d-printer-prints-carbon-fiber-parts Eitel, Elisabeth. MarkForged: $5,000 3D printer prints carbon-fiber parts. Machine Design Magazine, 7 March 2014]</ref> |
|||
* [http://www.thefoa.org/ The Fiber Optic Association] |
|||
* [http://www.thefoa.org/tech/connID.htm FOA color code for connectors] |
|||
* [http://www.jimhayes.com/lennielw/ Lennie Lightwave's Guide To Fiber Optics] |
|||
* "[https://www.rp-photonics.com/fibers.html Fibers]", article in RP Photonics' ''Encyclopedia of Laser Physics and Technology'' |
|||
* [http://www.fabila.com/proyectos/ftth/tecnologia.asp How Fiber Optics are made] In video |
|||
* "[http://www.gare.co.uk/technology_watch/fibre.htm Fibre optic technologies]", Mercury Communications Ltd, August 1992. |
|||
* "[http://www.gare.co.uk/technology_watch/photo.htm Photonics & the future of fibre]", Mercury Communications Ltd, March 1993. |
|||
* "[http://www.arcelect.com/fibercable.htm Fiber Optic Tutorial]" Educational site from Arc Electronics |
|||
* "[https://sites.google.com/a/electronicbricks.it/electronicbricks/technolgies-and-competitive-advantages/competitive-advantages-of-pof Plastic Optical Fiber]", Technologies and competitive advantages of POF – Plastic Optical Fiber |
|||
* [http://ocw.mit.edu/resources/res-6-005-understanding-lasers-and-fiberoptics-spring-2008/laser-fundamentals-i/ MIT Video Lecture: Understanding Lasers and Fiberoptics] |
|||
* [http://spie.org/Documents/Publications/00%20STEP%20Module%2007.pdf Fundamentals of Photonics: Module on Optical Waveguides and Fibers] |
|||
* [http://webdemo.inue.uni-stuttgart.de/webdemos/02_lectures/uebertragungstechnik_2/chromatic_dispersion webdemo for chromatic dispersion] Institute of Telecommunicatons, University of Stuttgart |
|||
}} |
|||
{{Optical communication}} |
|||
{{Glass science}} |
|||
{{Telecommunications}} |
|||
== Further reading == |
|||
{{Authority control}} |
|||
* {{cite web|title=Results of Make Magazine's 2015 3D Printer Shootout|url=https://docs.google.com/spreadsheets/d/1EKsDga2PVD_H9HI2MJbPXCey6bYFEIWErOsAHKHZ3GU/edit#gid=1210667708|publisher=docs.google.com|accessdate=1 June 2015}} |
|||
* {{cite web|title=Evaluation Protocol for Make Magazine's 2015 3D Printer Shootout|url=http://makezine.com/2014/11/07/how-to-evaluate-the-2015-make-3dp-test-probes/|publisher=makezine.com|accessdate=1 June 2015}} |
|||
* {{cite journal |last=Vincent |last2=Earls |first2=Alan R. |date=February 2011 |title=Origins: A 3D Vision Spawns Stratasys, Inc. |journal=Today's Machining World |volume=7 |issue=1 |publisher=Screw Machine World Inc. |location=Oak Forest, Illinois, USA |pages=24–25 |url=http://www.todaysmachiningworld.com/origins-a-3d-vision-spawns-stratasys-inc/ |ref=harv}} |
|||
* {{cite web|title=Heat Beds in 3D Printing – Advantages and Equipment|url=http://bootsindustries.com/portfolio-item/heat-bed-3d-printing/|website=Boots Industries|accessdate=7 September 2015}} |
|||
* {{cite journal |last=Albert |first=Mark [Editor in Chief] |date=17 January 2011 |title=Subtractive plus additive equals more than ( <nowiki>− + +=></nowiki> ): subtractive and additive processes can be combined to develop innovative manufacturing methods that are superior to conventional methods ['Mark: My Word' column – Editor's Commentary] |journal=Modern Machine Shop |volume=83 |issue=9 |publisher=Gardner Publications Inc. |location=Cincinnati, Ohio, USA |page=14 |url=http://www.mmsonline.com/columns/subtractive-plus-additive-equals-more-than |ref=harv}} |
|||
* {{cite journal |last1=Stephens |first1=B. |last2=Azimi |first2=P. |last3=El Orch |first3=Z. |last4=Ramos |first4=T. |title=Ultrafine particle emissions from desktop 3D printers |doi=10.1016/j.atmosenv.2013.06.050 |journal=Atmospheric Environment |volume=79 |pages=334 |year=2013}} |
|||
* {{cite journal |last=Easton |first=Thomas A. |date=November 2008|title=The 3D Trainwreck: How 3D Printing Will Shake Up Manufacturing |journal=[[Analog Science Fiction and Fact|Analog]] |volume=128 |issue=11 |pages=50–63 |ref=harv}} |
|||
* Wright, Paul K. (2001). ''21st Century Manufacturing''. New Jersey: Prentice-Hall Inc. |
|||
== External links == |
|||
{{DEFAULTSORT:Optical Fiber}} |
|||
{{Commons category|3D printing}}{{Z148}} |
|||
[[Category:Optical fiber| ]] |
|||
* {{dmoz|Science/Technology/Manufacturing/Prototyping/Rapid_Prototyping|Rapid prototyping websites}} |
|||
[[Category:Fiber optics| ]] |
|||
{{Prone to spam|date=May 2015}} |
|||
[[Category:Telecommunications equipment]] |
|||
{{Z148}}<!-- {{No more links}} |
|||
[[Category:Glass production]] |
|||
[[Category:Glass engineering and science]] |
|||
[[Category:Articles containing video clips]] |
|||
Please be cautious adding more external links. |
|||
[[pl:Światłowód włóknisty]] |
|||
Wikipedia is not a collection of links and should not be used for advertising. |
|||
Excessive or inappropriate links will be removed. |
|||
See [[Wikipedia:External links]] and [[Wikipedia:Spam]] for details. |
|||
If there are already suitable links, propose additions or replacements on |
|||
the article's talk page, or submit your link to the relevant category at |
|||
DMOZ (dmoz.org) and link there using {{Dmoz}}. |
|||
--> |
|||
{{3d printing}} |
|||
{{Emerging technologies}} |
|||
{{EngvarB|date=May 2014}} |
|||
[[Category:Japanese inventions]] |
|||
[[Category:1981 introductions]] |
|||
[[Category:Industrial design]] |
|||
[[Category:Emerging technologies]] |
|||
[[Category:Manufacturing]] |
|||
[[Category:3D printing| ]] |
|||
[[Category:Articles containing video clips]] |
|||
[[Category:Industrial Revolution]] |
|||
[[Category:DIY culture]] |
Revision as of 18:56, 7 December 2015

Part of a series on the |
History of printing |
---|
![]() |
3D printing, also known as additive manufacturing (AM), refers to various processes used to synthesize a three-dimensional object.[1] In 3D printing, successive layers of material are formed under computer control to create an object.[2] These objects can be of almost any shape or geometry, and are produced from a 3D model or other electronic data source. A 3D printer is a type of industrial robot.
Futurologists such as Jeremy Rifkin [3] believe that 3D printing signals the beginning of a third industrial revolution,[4] succeeding the production line assembly that dominated manufacturing starting in the late 19th century. Using the power of the Internet, it may eventually be possible to send a blueprint of any product to any place in the world to be replicated by a 3D printer with "elemental inks" capable of being combined into any material substance of any desired form.
3D printing in the term's original sense refers to processes that sequentially deposit material onto a powder bed with inkjet printer heads. More recently, the meaning of the term has expanded to encompass a wider variety of techniques such as extrusion and sintering-based processes. Technical standards generally use the term additive manufacturing for this broader sense.
History
Terminology and methods
Early Additive Manufacturing equipment and materials were developed in the 1980s.[5] In 1981, Hideo Kodama of Nagoya Municipal Industrial Research Institute invented two AM fabricating methods of a three-dimensional plastic model with photo-hardening polymer, where the UV exposure area is controlled by a mask pattern or the scanning fiber transmitter.[6][7] Then in 1984, Chuck Hull of 3D Systems Corporation[8] developed a prototype system based on a process known as stereolithography, in which layers are added by curing photopolymers with ultraviolet light lasers. Hull defined the process as a "system for generating three-dimensional objects by creating a cross-sectional pattern of the object to be formed,"[9][10] but this had been already invented by Kodama. Hull's contribution is the design of the STL (STereoLithography) file format widely accepted by 3D printing software as well as the digital slicing and infill strategies common to many processes today. The term 3D printing originally referred to a process employing standard and custom inkjet print heads. The technology used by most 3D printers to date—especially hobbyist and consumer-oriented models—is fused deposition modeling, a special application of plastic extrusion.
AM processes for metal sintering or melting (such as selective laser sintering, direct metal laser sintering, and selective laser melting) usually went by their own individual names in the 1980s and 1990s. At the time, nearly all metal working was produced by casting, fabrication, stamping, and machining; although plenty of automation was applied to those technologies (such as by robot welding and CNC), the idea of a tool or head moving through a 3D work envelope transforming a mass of raw material into a desired shape layer by layer was associated by most people only with processes that removed metal (rather than adding it), such as CNC milling, CNC EDM, and many others. But AM-type sintering was beginning to challenge that assumption. By the mid 1990s, new techniques for material deposition were developed at Stanford and Carnegie Mellon University, including microcasting[11] and sprayed materials.[12] Sacrificial and support materials had also become more common, enabling new object geometries.[13]
The umbrella term additive manufacturing gained wider currency in the decade of the 2000s.[14] As the various additive processes matured, it became clear that soon metal removal would no longer be the only metalworking process done under that type of control (a tool or head moving through a 3D work envelope transforming a mass of raw material into a desired shape layer by layer). It was during this decade that the term subtractive manufacturing appeared as a retronym for the large family of machining processes with metal removal as their common theme. However, at the time, the term 3D printing still referred only to the polymer technologies in most minds, and the term AM was likelier to be used in metalworking contexts than among polymer/inkjet/stereolithography enthusiasts. The term subtractive has not replaced the term machining, instead complementing it when a term that covers any removal method is needed.
By the early 2010s, the terms 3D printing and additive manufacturing developed senses in which they were synonymous umbrella terms for all AM technologies. Although this was a departure from their earlier technically narrower senses, it reflects the simple fact that the technologies all share the common theme of sequential-layer material addition/joining throughout a 3D work envelope under automated control. (Other terms that have appeared, which are usually used as AM synonyms (although sometimes as hypernyms), have been desktop manufacturing, rapid manufacturing [as the logical production-level successor to rapid prototyping], and on-demand manufacturing [which echoes on-demand printing in the 2D sense of printing].) The 2010s were the first decade in which metal parts such as engine brackets[15] and large nuts[16] would be grown (either before or instead of machining) in job production rather than obligately being machined from bar stock or plate.
As technology matured, several authors had begun to speculate that 3D printing could aid in sustainable development in the developing world.[17][18][19]
General principles
Modeling
3D printable models may be created with a computer aided design (CAD) package, via a 3D scanner or by a plain digital camera and photogrammetry software. 3D printed models created with CAD results in reduced errors and can be corrected before printing, allowing verification in the design of the object before it is printed.[20]

The manual modeling process of preparing geometric data for 3D computer graphics is similar to plastic arts such as sculpting. 3D scanning is a process of collecting digital data on the shape and appearance of a real object, creating a digital model based on it.
Printing
Before printing a 3D model from an STL file, it must first be examined for "manifold errors". This step being called the "fixup."[citation needed] Generally STLs that have been produced from a model obtained through 3D scanning often have many manifold errors in them that need to be rectified. Examples of these errors are surfaces that do not connect, or gaps in the models.[citation needed]
Once completed, the STL file needs to be processed by a piece of software called a "slicer," which converts the model into a series of thin layers and produces a G-code file containing instructions tailored to a specific type of 3D printer (FDM printers).[citation needed] This G-code file can then be printed with 3D printing client software (which loads the G-code, and uses it to instruct the 3D printer during the 3D printing process).[citation needed]
Printer resolution describes layer thickness and X-Y resolution in dots per inch (dpi) or micrometers (µm). Typical layer thickness is around 100 μm (250 DPI), although some machines can print layers as thin as 16 μm (1,600 DPI).[21] X-Y resolution is comparable to that of laser printers. The particles (3D dots) are around 50 to 100 μm (510 to 250 DPI) in diameter.[citation needed]
Construction of a model with contemporary methods can take anywhere from several hours to several days, depending on the method used and the size and complexity of the model. Additive systems can typically reduce this time to a few hours, although it varies widely depending on the type of machine used and the size and number of models being produced simultaneously.[citation needed]
Traditional techniques like injection moulding can be less expensive for manufacturing polymer products in high quantities, but additive manufacturing can be faster, more flexible and less expensive when producing relatively small quantities of parts. 3D printers give designers and concept development teams the ability to produce parts and concept models using a desktop size printer.[citation needed]
Finishing
Though the printer-produced resolution is sufficient for many applications, printing a slightly oversized version of the desired object in standard resolution and then removing material[22] with a higher-resolution subtractive process can achieve greater precision.
Some printable polymers allow the surface finish to be smoothed and improved using chemical vapor processes.[citation needed]
Some additive manufacturing techniques are capable of using multiple materials in the course of constructing parts. These techniques are able to print in multiple colors and color combinations simultaneously, and would not necessarily require painting.
Some printing techniques require internal supports to be built for overhanging features during construction. These supports must be mechanically removed or dissolved upon completion of the print.
All of the commercialized metal 3D printers involve cutting the metal component off of the metal substrate after deposition. A new process for the GMAW 3D printing allows for substrate surface modifications to remove aluminum[23] or steel.[24]
Processes
Several 3D printing processes have been invented since the late 1970s. The printers were originally large, expensive, and highly limited in what they could produce.[5]
A large number of additive processes are now available. The main differences between processes are in the way layers are deposited to create parts and in the materials that are used. Some methods melt or soften the material to produce the layers, for example. selective laser melting (SLM) or direct metal laser sintering (DMLS), selective laser sintering (SLS), fused deposition modeling (FDM),[25] or fused filament fabrication (FFF), while others cure liquid materials using different sophisticated technologies, such as stereolithography (SLA). With laminated object manufacturing (LOM), thin layers are cut to shape and joined together (e.g., paper, polymer, metal). Each method has its own advantages and drawbacks, which is why some companies offer a choice of powder and polymer for the material used to build the object.[26] Others sometimes use standard, off-the-shelf business paper as the build material to produce a durable prototype. The main considerations in choosing a machine are generally speed, costs of the 3D printer, of the printed prototype, choice and cost of the materials, and color capabilities.[27]
Printers that work directly with metals are generally expensive. However less expensive printers can be used to make a mold, which is then used to make metal parts.[28]
Extrusion deposition

Fused deposition modeling (FDM), derives from automatic polymeric foil hot air welding system, hot-melt glueing and automatic gasket deposition. Such principle has been further developed by S. Scott Crump in the late 1980s and was commercialized in 1990 by Stratasys.[30] After the patent on this technology expired, a large open-source development community developed and both commercial and DIY variants utilizing this type of 3D printer appeared. As a result, the price of this technology has dropped by two orders of magnitude since its creation.
In fused deposition modeling the model or part is produced by extruding small beads of material which harden immediately to form layers. A thermoplastic filament or metal wire that is wound on a coil is unreeled to supply material to an extrusion nozzle head (3D printer extruder). The nozzle head heats the material and turns the flow on and off. Typically stepper motors or servo motors are employed to move the extrusion head and adjust the flow. The printer usually has 3 axes of motion. A computer-aided manufacturing (CAM) software package is used to generate the G-Code that is sent to a microcontroller which controls the motors.
Extrusion in 3D printing using material extrusion involves a cold end and a hot end.
Various polymers are used, including acrylonitrile butadiene styrene (ABS), polycarbonate (PC), polylactic acid (PLA), high density polyethylene (HDPE), PC/ABS, polyphenylsulfone (PPSU) and high impact polystyrene (HIPS). In general, the polymer is in the form of a filament fabricated from virgin resins. There are multiple projects in the open-sourced community aimed at processing post-consumer plastic waste into filament. These involve machines used to shred and extrude the plastic material into filament.
FDM is somewhat restricted in the variation of shapes that may be fabricated. For example, FDM usually cannot produce stalactite-like structures, since they would be unsupported during the build. Otherwise, a thin support must be designed into the structure which can be broken away during finishing. Fused deposition modeling is also referred to as fused filament fabrication (FFF) by companies who do not hold the original patents like Stratasys does.
Binding of granular materials
Another 3D printing approach is the selective fusing of materials in a granular bed. The technique fuses parts of the layer and then moves downward in the working area, adding another layer of granules and repeating the process until the piece has built up. This process uses the unfused media to support overhangs and thin walls in the part being produced, which reduces the need for temporary auxiliary supports for the piece. A laser is typically used to sinter the media into a solid. Examples include selective laser sintering (SLS), with both metals and polymers (e.g., PA, PA-GF, Rigid GF, PEEK, PS, Alumide, Carbonmide, elastomers), and direct metal laser sintering (DMLS).[31]
Selective Laser Sintering (SLS) was developed and patented by Dr. Carl Deckard and Dr. Joseph Beaman at the University of Texas at Austin in the mid-1980s, under sponsorship of DARPA.[32] A similar process was patented without being commercialized by R. F. Housholder in 1979.[33]
Selective laser melting (SLM) does not use sintering for the fusion of powder granules but will completely melt the powder using a high-energy laser to create fully dense materials in a layer-wise method that has mechanical properties similar to those of conventional manufactured metals.
Electron beam melting (EBM) is a similar type of additive manufacturing technology for metal parts (e.g. titanium alloys). EBM manufactures parts by melting metal powder layer by layer with an electron beam in a high vacuum. Unlike metal sintering techniques that operate below melting point, EBM parts void-freeR.[34][35]
Another method consists of an inkjet 3D printing system. The printer creates the model one layer at a time by spreading a layer of powder (plaster, or resins) and printing a binder in the cross-section of the part using an inkjet-like process. This is repeated until every layer has been printed. This technology allows the printing of full color prototypes, overhangs, and elastomer parts. The strength of bonded powder prints can be enhanced with wax or thermoset polymer impregnation.
Lamination
In some printers, paper can be used as the build material, resulting in a lower cost to print. During the 1990s some companies marketed printers that cut cross sections out of special adhesive coated paper using a carbon dioxide laser and then laminated them together.
In 2005 Mcor Technologies Ltd developed a different process using ordinary sheets of office paper, a tungsten carbide blade to cut the shape, and selective deposition of adhesive and pressure to bond the prototype.[36]
There are also a number of companies selling printers that print laminated objects using thin plastic and metal sheets.
Photopolymerization

Stereolithography was patented in 1986 by Chuck Hull.[37] Photopolymerization is primarily used in stereolithography (SLA) to produce a solid part from a liquid. This process was a dramatic departure from the "photosculpture" method of François Willème (1830–1905) developed in 1860 and the photopolymerization of Mitsubishi's Matsubara in 1974.[38]
The "photosculpture" method consisted of photographing a subject from a variety of equidistant angles and projecting each photograph onto a screen, where a pantograph was used to trace the outline onto modeling clay.[39][40][41] In photo-polymerization, a vat of liquid polymer is exposed to controlled lighting under safelight conditions. The exposed liquid polymer hardens. Polymerization occurs when photopolymers are exposed to light when photopolymers contain chromophores, otherwise, the addition of molecules that are photosensitive are utilized to react with the solution to begin polymerization. Polymerization of monomers lead to cross-linking, which creates a polymer. Through these covalent bonds, the property of the solution is changed.[20] The build plate then moves down in small increments and the liquid polymer is again exposed to light. The process repeats until the model has been built. The liquid polymer is then drained from the vat, leaving the solid model. The EnvisionTEC Perfactory[42] is an example of a DLP rapid prototyping system.
Inkjet printer systems like the Objet PolyJet system spray photopolymer materials onto a build tray in ultra-thin layers (between 16 and 30 µm) until the part is completed. Each photopolymer layer is cured with UV light after it is jetted, producing fully cured models that can be handled and used immediately, without post-curing. The gel-like support material, which is designed to support complicated geometries, is removed by hand and water jetting. It is also suitable for elastomers.
Ultra-small features can be made with the 3D micro-fabrication technique used in multiphoton photopolymerisation. This approach uses a focused laser to trace the desired 3D object into a block of gel. Due to the nonlinear nature of photo excitation, the gel is cured to a solid only in the places where the laser was focused while the remaining gel is then washed away. Feature sizes of under 100 nm are easily produced, as well as complex structures with moving and interlocked parts.[43]
Yet another approach uses a synthetic resin that is solidified using LEDs.[44]
In Mask-image-projection-based stereolithography a 3D digital model is sliced by a set of horizontal planes. Each slice is converted into a two-dimensional mask image. The mask image is then projected onto a photocurable liquid resin surface and light is projected onto the resin to cure it in the shape of the layer.[45] The technique has been used to create objects composed of multiple materials that cure at different rates.[45] In research systems, the light is projected from below, allowing the resin to be quickly spread into uniform thin layers, reducing production time from hours to minutes.[45] Commercially available devices such as Objet Connex apply the resin via small nozzles.[45]
Metal wire processes
Laser-based wirefeed systems, such as Laser Metal Deposition-wire (LMD-w), feed wire through a nozzle that is melted by a laser using inert gas shielding in either an open environment (gas surrounding the laser), or in a sealed chamber. Electron beam freeform fabrication uses an electron beam heat source inside a vacuum chamber.
It is also possible to use conventional gas metal arc welding attached to a 3D stage to 3-D print metals such as steel and aluminum.[46] Low-cost open source RepRap-style 3-D printers have been outfitted with Arduino-based sensors and demonstrated reasonable metallurgical properties from conventional welding wire as feedstock.[47]
Printers
Industry use
![]() | This section needs expansion. You can help by adding to it. (August 2015) |
As October 2012, additive manufacturing systems were on the market that ranged from $2,000 to $500,000 in price and were employed in industries including aerospace, architecture, automotive, defense, and medical replacements, among many others. For example, General Electric uses the high-end model to build parts for turbines.[48] Many of these systems are used for rapid prototyping, before mass production methods are employed.
Consumer use



Several projects and companies are making efforts to develop affordable 3D printers for home desktop use. Much of this work has been driven by and targeted at DIY/Maker/enthusiast/early adopter communities, with additional ties to the academic and hacker communities.[49]
RepRap Project is one of the longest running projects in the desktop category. The RepRap project aims to produce a free and open source hardware (FOSH) 3D printer, whose full specifications are released under the GNU General Public License, and which is capable of replicating itself by printing many of its own (plastic) parts to create more machines.[50][51] RepRaps have already been shown to be able to print circuit boards[52] and metal parts.[53][54]
Because of the FOSH aims of RepRap, many related projects have used their design for inspiration, creating an ecosystem of related or derivative 3D printers, most of which are also open source designs. The availability of these open source designs means that variants of 3D printers are easy to invent. The quality and complexity of printer designs, however, as well as the quality of kit or finished products, varies greatly from project to project. This rapid development of open source 3D printers is gaining interest in many spheres as it enables hyper-customization and the use of public domain designs to fabricate open source appropriate technology. This technology can also assist initiatives in sustainable development since technologies are easily and economically made from resources available to local communities.[17][18]
The cost of 3D printers has decreased dramatically since about 2010, with machines that used to cost $20,000 now costing less than $1,000.[55] For instance, as of 2013, several companies and individuals are selling parts to build various RepRap designs, with prices starting at about €400 / US$500.[56] The open source Fab@Home project[57] has developed printers for general use with anything that can be squirted through a nozzle, from chocolate to silicone sealant and chemical reactants. Printers following the project's designs have been available from suppliers in kits or in pre-assembled form since 2012 at prices in the US$2000 range.[56] The Kickstarter funded Peachy Printer is designed to cost $100[58] and several other new 3D printers are aimed at the small, inexpensive market including the mUVe3D and Lumifold. Rapide 3D has designed a professional grade crowdsourced 3D-printer costing $1499 which has no fumes nor constant rattle during use.[59] The 3Doodler, "3D printing pen", raised $2.3 million on Kickstarter with the pens selling at $99,[60] though the 3D Doodler has been criticized for being more of a crafting pen than a 3D printer.[61]
As the costs of 3D printers have come down they are becoming more appealing financially to use for self-manufacturing of personal products.[62] In addition, 3D printing products at home may reduce the environmental impacts of manufacturing by reducing material use and distribution impacts.[63]
In addition, several RecycleBots such as the commercialized Filastruder have been designed and fabricated to convert waste plastic, such as shampoo containers and milk jugs, into inexpensive RepRap filament.[64] There is some evidence that using this approach of distributed recycling is better for the environment.[65]
The development and hyper-customization of the RepRap-based 3D printers has produced a new category of printers suitable for small business and consumer use. Manufacturers such as Solidoodle,[48] Robo 3D, RepRapPro and Pirx 3D have introduced models and kits priced at less than $1,000, thousands less than they were in September 2012.[48] Depending on the application, the print resolution and speed of manufacturing lies somewhere between a personal printer and an industrial printer. A list of printers with pricing and other information is maintained.[56] Most recently delta robots, like the TripodMaker, have been utilized for 3D printing to increase fabrication speed further.[66] For delta 3D printers, due to its geometry and differentiation movements, the accuracy of the print depends on the position of the printer head.[67]
Some companies are also offering software for 3D printing, as a support for hardware manufactured by other companies.[68]
Large 3D printers
Large 3D printers have been developed for industrial, education, and demonstrative uses. A large delta-style 3D printer was built in 2014 by SeeMeCNC. The printer is capable of making an object with diameter of up to 4 feet (1.2 m) and up to 10 feet (3.0 m) in height. It also uses plastic pellets as the raw material instead of the typical plastic filaments used in other 3D printers.[69]

Another type of large printer is Big Area Additive Manufacturing (BAAM). The goal is to develop printers that can produce a large object in high speed. A BAAM machine of Cincinnati Incorporated can produce an object at the speeds 200-500 times faster than typical 3D printers available in 2014. Another BAAM machine is being developed by Lockheed Martin with an aim to print long objects of up to 100 feet (30 m) to be used in aerospace industries.[70]
Microscale and nanoscale 3D printing
Microelectronic device fabrication methods can be employed to perform the 3D printing of nanoscale-size objects. Such printed objects are typically grown on a solid substrate, e.g. silicon wafer, to which they adhere after printing as they're too small and fragile to be manipulated post-construction.
In one technique, 3D nanostructures can be printed by physically moving a dynamic stencil mask during the material deposition process, somewhat analogous to the extrusion method of traditional 3D printers. Programmable-height nanostructures with resolutions as small as 10 nm have been produced in this fashion, by metallic physical vapor deposition through a piezo-actuator controlled stencil mask having a milled nanopore in a silicon nitride membrane.[71]
Another method enhances the photopolymerization process on a much smaller scale, using finely-focused lasers controlled by adjustable mirrors. This method has produced objects with feature resolutions of 100 nm.[72]
Manufacturing applications
Three-dimensional printing makes it as cheap to create single items as it is to produce thousands and thus undermines economies of scale. It may have as profound an impact on the world as the coming of the factory did....Just as nobody could have predicted the impact of the steam engine in 1750—or the printing press in 1450, or the transistor in 1950—it is impossible to foresee the long-term impact of 3D printing. But the technology is coming, and it is likely to disrupt every field it touches.
— The Economist, in a February 10, 2011 leader[73]
AM technologies found applications starting in the 1980s in product development, data visualization, rapid prototyping, and specialized manufacturing. Their expansion into production (job production, mass production, and distributed manufacturing) has been under development in the decades since. Industrial production roles within the metalworking industries[74] achieved significant scale for the first time in the early 2010s. Since the start of the 21st century there has been a large growth in the sales of AM machines, and their price has dropped substantially.[75] According to Wohlers Associates, a consultancy, the market for 3D printers and services was worth $2.2 billion worldwide in 2012, up 29% from 2011.[76] There are many applications for AM technologies, including architecture, construction (AEC), industrial design, automotive, aerospace,[77] military, engineering, dental and medical industries, biotech (human tissue replacement), fashion, footwear, jewelry, eyewear, education, geographic information systems, food, and many other fields.
Additive manufacturing's earliest applications have been on the toolroom end of the manufacturing spectrum. For example, rapid prototyping was one of the earliest additive variants, and its mission was to reduce the lead time and cost of developing prototypes of new parts and devices, which was earlier only done with subtractive toolroom methods such as cnc milling and turning, and precision grinding, far more accurate than 3d printing with accuracy down to 0.00005" and creating better quality parts faster, but sometimes too expensive for low accuracy prototype parts.[78] With technological advances in additive manufacturing, however, and the dissemination of those advances into the business world, additive methods are moving ever further into the production end of manufacturing in creative and sometimes unexpected ways.[78] Parts that were formerly the sole province of subtractive methods can now in some cases be made more profitably via additive ones. In addition, new developments in RepRap technology allow the same device to perform both additive and subtractive manufacturing by swapping magnetic-mounted tool heads.[79]
Cloud-based additive manufacturing
Additive manufacturing in combination with cloud computing technologies allows decentralized and geographically independent distributed production.[80] Cloud-based additive manufacturing refers to a service-oriented networked manufacturing model in which service consumers are able to build parts through Infrastructure-as-a-Service (IaaS), Platform-as-a-Service (PaaS), Hardware-as-a-Service (HaaS), and Software-as-a-Service (SaaS).[81][82][83] Distributed manufacturing as such is carried out by some enterprises; there is also a services like 3D Hubs that put people needing 3D printing in contact with owners of printers.[84]
Some companies offer on-line 3D printing services to both commercial and private customers,[85] working from 3D designs uploaded to the company website. 3D-printed designs are either shipped to the customer or picked up from the service provider.[86]
Mass customization

Companies have created services where consumers can customize objects using simplified web based customisation software, and order the resulting items as 3D printed unique objects.[87][88] This now allows consumers to create custom cases for their mobile phones.[89] Nokia has released the 3D designs for its case so that owners can customize their own case and have it 3D printed.[90]
Rapid manufacturing
Advances in RP technology have introduced materials that are appropriate for final manufacture, which has in turn introduced the possibility of directly manufacturing finished components. One advantage of 3D printing for rapid manufacturing lies in the relatively inexpensive production of small numbers of parts.
Rapid manufacturing is a new method of manufacturing and many of its processes remain unproven. 3D printing is now entering the field of rapid manufacturing and was identified as a "next level" technology by many experts in a 2009 report.[91] One of the most promising processes looks to be the adaptation of selective laser sintering (SLS), or direct metal laser sintering (DMLS) some of the better-established rapid prototyping methods. As of 2006[update], however, these techniques were still very much in their infancy, with many obstacles to be overcome before RM could be considered a realistic manufacturing method.[92] ,l,/.
Rapid prototyping
Industrial 3D printers have existed since the early 1980s and have been used extensively for rapid prototyping and research purposes. These are generally larger machines that use proprietary powdered metals, casting media (e.g. sand), plastics, paper or cartridges, and are used for rapid prototyping by universities and commercial companies.
Research
3D printing can be particularly useful in research labs due to its ability to make specialized, bespoke geometries. In 2012 a proof of principle project at the University of Glasgow, UK, showed that it is possible to use 3D printing techniques to assist in the production of chemical compounds. They first printed chemical reaction vessels, then used the printer to deposit reactants into them.[93] They have produced new compounds to verify the validity of the process, but have not pursued anything with a particular application.[93]
Food
Cornell Creative Machines Lab announced in 2012 that it was possible to produce customized food with 3D Hydrocolloid Printing.[94] Additive manufacturing of food is currently being developed by squeezing out food, layer by layer, into three-dimensional objects. A large variety of foods are appropriate candidates, such as chocolate and candy, and flat foods such as crackers, pasta,[95] and pizza.[96] NASA has considered the versatility of the concept, awarding a contract to the Systems and Materials Research Consultancy to study the feasibility of printing food in space.[97] One of the problem with food printing is the nature of the texture of a food. For example, foods that are not strong enough to be filed are not appropriate for 3D printing for now.
Medical applications
Professor Leroy Cronin of Glasgow University proposed in a 2012 TED Talk that it was possible to use chemical inks to print medicine.[98] Similarly, 3D printing has been considered as a method of implanting stem cells capable of generating new tissues and organs in living humans.[99]
Industrial applications
Apparel
3D printing has entered the world of clothing with fashion designers experimenting with 3D-printed bikinis, shoes, and dresses.[100] In commercial production Nike is using 3D printing to prototype and manufacture the 2012 Vapor Laser Talon football shoe for players of American football, and New Balance is 3D manufacturing custom-fit shoes for athletes.[100][101]
3D printing has come to the point where companies are printing consumer grade eyewear with on-demand custom fit and styling (although they cannot print the lenses). On-demand customization of glasses is possible with rapid prototyping.[102]
Vehicle

In early 2014, the Swedish supercar manufacturer, Koenigsegg, announced the One:1, a supercar that utilizes many components that were 3D printed. In the limited run of vehicles Koenigsegg produces, the One:1 has side-mirror internals, air ducts, titanium exhaust components, and even complete turbocharger assemblies that have been 3D printed as part of the manufacturing process.[103]
Urbee is the name of the first car in the world car mounted using the technology 3D printing (his bodywork and his car windows were "printed"). Created in 2010 through the partnership between the US engineering group Kor Ecologic and the company Stratasys (manufacturer of printers Stratasys 3D), it is a hybrid vehicle with futuristic look.[104][105][106]
In May 2015 Airbus announced that its new Airbus A350 XWB included over 1000 components manufactured by 3D printing.[107]
3D printing is also being utilized by air forces to print spare parts for planes. In 2015, a Royal Air Force Eurofighter Typhoon fighter jet flew with printed parts. The United States Air Force has begun to work with 3D printers, and the Israeli Air Force has also purchased a 3D printer to print spare parts.[108]
Construction
Until recent years models were built by hand, often taking a long time. Thus, architects are often forced to show their clients drawings of their projects. According to Erik Kinipper, clients usually need to see the product from all possible viewpoints in space to get a clearer picture of the design and make an informed decision. In order to get these scale models to clients in a small amount of time, architects and architecture firms tend to rely on 3D printing.[109] Using 3D printing, these firms can reduce lead times of production by 50 to 80 percent, producing scale models up to 60 percent lighter than the machined part while being sturdy.[110] Thus, the designs and the models are only limited by a person's imagination.
The improvements on accuracy, speed and quality of materials in 3D printing technology have opened new doors for it to move beyond the use of 3D printing in the modeling process and actually move it to manufacturing strategy. A good example is Dr. Behrokh Khoshnevis’ research at the University of Southern California which resulted in a 3D printer that can build a house in 24 hours .The process is called Contour Crafting. Khoshnevis, Russell, Kwon, & Bukkapatnam, define contour crafting as an additive manufacturing process which uses computer controlled systems to repeatedly lay down layers of materials such as concrete. Bushey also discussed Khoshnevis's robot which comes equipped with a nozzle that spews out concrete and can build a home based on a set computer pattern. Contour Crafting technology has great potential for automating the construction of whole structures as well as sub-components. Using this process, a single house or a colony of houses, each with possibly a different design, may be automatically constructed in a single run, embedded in each house all the conduits for electrical, plumbing and air-conditioning.[111]
Firearms
In 2012, the US-based group Defense Distributed disclosed plans to "[design] a working plastic gun that could be downloaded and reproduced by anybody with a 3D printer."[112][113] Defense Distributed has also designed a 3D printable AR-15 type rifle lower receiver (capable of lasting more than 650 rounds) and a 30 round M16 magazine The AR-15 has multiple receivers (both an upper and lower receiver), but the legally controlled part is the one that is serialized (the lower, in the AR-15's case). Soon after Defense Distributed succeeded in designing the first working blueprint to produce a plastic gun with a 3D printer in May 2013, the United States Department of State demanded that they remove the instructions from their website.[114] After Defense Distributed released their plans, questions were raised regarding the effects that 3D printing and widespread consumer-level CNC machining[115][116] may have on gun control effectiveness.[117][118][119][120]
In 2014, a man from Japan became the first person in the world to be imprisoned for making 3D printed firearms.[121] Yoshitomo Imura posted videos and blueprints of the gun online and was sentenced to jail for two years. Police found at least two guns in his household that were capable of firing bullets.[121]
Medical
![]() | This section needs to be updated.(February 2015) |

3D printing has been used to print patient specific implant and device for medical use. Successful operations include a titanium pelvis implanted into a British patient, titanium lower jaw transplanted to a Belgian patient,[122] and a plastic tracheal splint for an American infant.[123] The hearing aid and dental industries are expected to be the biggest area of future development using the custom 3D printing technology.[124] In March 2014, surgeons in Swansea used 3D printed parts to rebuild the face of a motorcyclist who had been seriously injured in a road accident.[125] Research is also being conducted on methods to bio-print replacements for lost tissue due to arthritis and cancer [citation needed].
The things we are printing are becoming more personal and intimate overtime. This is especially true even in medicine: increasingly, what we are printing is ourselves. 3D printing is a great technological advancement in the medical field. 3D printing technology can now be used to make exact replicas of organs. Doctors can use these to plan out surgeries a lot more accurately. While, medical students may even be able to perform practice operations. The printer uses images from patients' MRI or CT scan images as a template and lays down layers of rubber or plastic. These types of procedures are becoming more and more common among doctors and medical researchers everywhere. Many have begun to use 3D printing in order to accurately undergo surgical procedures. This helps that may have been considered inoperable before have the opportunity to have a successful surgery. The hope is that these printers will eventually be able to produce actual organs that can be transplanted into patients by replacing the rubber and plastic printer "ink" with human cells. "The printer and software usually cost in the range of $100,000." Which happens to be less than a CT scan or MRI setup. Rader said "He predicts that interest in the technology will continue to grow as research shows how using simulated organs leads to better surgical outcomes and shorter operating times." This will also help the patients feel at ease as they can be provided with a live model to better explain surgical procedures and put the patient at ease.[126]
Medical devices
In October 24, 2014, a five-year-old girl born without fully formed fingers on her left hand became the first child in the UK to have a prosthetic hand made with 3D printing technology. Her hand was designed by US-based E-nable, an open source design organisation which uses a network of volunteers to design and make prosthetics mainly for children. The prosthetic hand was based on a plaster cast made by her parents.[127] A boy named Alex was also born with a missing arm from just above the elbow. The team was able to use 3D printing to upload an e-NABLE Myoelectric arm that runs off of servos and batteries that are actuated by the electromyography muscle. With the use of 3D printers, E-NABLE has so far distributed more than 400 plastic hands to children.
Printed prosthetics have been used in rehabilitation of crippled animals. In 2013, a 3D printed foot let a crippled duckling walk again.[128] In 2014 a chihuahua born without front legs was fitted with a harness and wheels created with a 3D printer.[129] 3D printed hermit crab shells let hermit crabs inhabit a new style home.[130] A prosthetic beak was another tool developed by the use of 3D printing to help aid a bald eagle named Beauty, whose beak was severely mutilated from a shot in the face. Since 2014, commercially available titanium knee implants made with 3D printer for dogs have been used to restore the animal mobility. Over 10,000 dogs in Europe and United States have been treated after only one year.[131]
In February 2015, FDA approved the marketing of a surgical bolt which facilitates less-invasive foot surgery and eliminates the need to drill through bone. The 3D printed titanium device, 'FastForward Bone Tether Plate' is approved to use in correction surgery to treat bunion.[132]
Bio-printing
As of 2012[update], 3D bio-printing technology has been studied by biotechnology firms and academia for possible use in tissue engineering applications in which organs and body parts are built using inkjet techniques. In this process, layers of living cells are deposited onto a gel medium or sugar matrix and slowly built up to form three-dimensional structures including vascular systems.[133] The first production system for 3D tissue printing was delivered in 2009, based on NovoGen bioprinting technology.[134] Several terms have been used to refer to this field of research: organ printing, bio-printing, body part printing,[135] and computer-aided tissue engineering, among others.[136] The possibility of using 3D tissue printing to create soft tissue architectures for reconstructive surgery is also being explored.[137]
In 2013, Chinese scientists began printing ears, livers and kidneys, with living tissue. Researchers in China have been able to successfully print human organs using specialized 3D bio printers that use living cells instead of plastic [citation needed]. Researchers at Hangzhou Dianzi University designed the "3D bio printer" dubbed the "Regenovo". Xu Mingen, Regenovo's developer, said that it takes the printer under an hour to produce either a mini liver sample or a four to five inch ear cartilage sample. Xu also predicted that fully functional printed organs may be possible within the next ten to twenty years.[138][139] In the same year, researchers at the University of Hasselt, in Belgium had successfully printed a new jawbone for an 83-year-old Belgian woman.[140]
Pills
The first pill manufactured by 3D printing was approved by FDA in August 2015. Binder-jetting into a powder bed of the drug allows very porous pills to be produced, which enables high drug doses in a single pill which dissolves quickly and can be ingested easily.[141] This has been demonstrated for Spritam, a reformulation of levetiracetam for the treatment of epilepsy.
Computers and robots
3D printing can also be used to make laptops and other computers and cases. For example, Novena and VIA OpenBook standard laptop cases. I.e. a Novena motherboard can be bought and be used in a printed VIA OpenBook case.[142]
Open-source robots are built using 3D printers. Double Robotics grant access to their technology (an open SDK).[143][144][145] On the other hand, 3&DBot is an Arduino 3D printer-robot with wheels[146] and ODOI is a 3D printed humanoid robot.[147]
Space
The Zero-G Printer, the first 3D printer designed to operate in zero gravity, was built under a joint partnership between NASA Marshall Space Flight Center (MSFC) and Made In Space, Inc.[148] In September 2014, SpaceX delivered the zero-gravity 3D printer to the International Space Station (ISS). On December 19, 2014, NASA emailed CAD drawings for a socket wrench to astronauts aboard the ISS, who then printed the tool using its 3D printer. Applications for space offer the ability to print parts or tools on-site, as opposed to using rockets to bring along pre-manufactured items for space missions to human colonies on the moon, Mars, or elsewhere.[149] The European Space Agency plans to deliver its new Portable On-Board 3D Printer (POP3D for short) to the International Space Station by June 2015, making it the second 3D printer in space.[150][151]
Furthermore, the Sinterhab project is researching a lunar base constructed by 3D printing using lunar regolith as a base material. Instead of adding a binding agent to the regolith, researchers are experimenting with microwave sintering to create solid blocks from the raw material.[152]
Similar researches and projects like these could allow faster construction for lower costs, and has been investigated for construction of off-Earth habitats.[153][154]
Sociocultural applications


In 2005, a rapidly expanding hobbyist and home-use market was established with the inauguration of the open-source RepRap and Fab@Home projects. Virtually all home-use 3D printers released to-date have their technical roots in the ongoing RepRap Project and associated open-source software initiatives.[155] In distributed manufacturing, one study has found[156] that 3D printing could become a mass market product enabling consumers to save money associated with purchasing common household objects.[62] For example, instead of going to a store to buy an object made in a factory by injection molding (such as a measuring cup or a funnel), a person might instead print it at home from a downloaded 3D model.
Art
In 2005, academic journals had begun to report on the possible artistic applications of 3D printing technology.[157] By 2007 the mass media followed with an article in the Wall Street Journal[158] and Time Magazine, listing a 3D printed design among their 100 most influential designs of the year.[159] During the 2011 London Design Festival, an installation, curated by Murray Moss and focused on 3D Printing, was held in the Victoria and Albert Museum (the V&A). The installation was called Industrial Revolution 2.0: How the Material World will Newly Materialize.[160]

Some of the recent developments in 3D printing were revealed at the 3DPrintshow in London, which took place in November 2013 and 2014. The art section had in exposition artworks made with 3D printed plastic and metal. Several artists such as Joshua Harker, Davide Prete, Sophie Kahn, Helena Lukasova, Foteini Setaki showed how 3D printing can modify aesthetic and art processes. One part of the show focused on ways in which 3D printing can advance the medical field. The underlying theme of these advances was that these printers can be used to create parts that are printed with specifications to meet each individual. This makes the process safer and more efficient. One of these advances is the use of 3D printers to produce casts that are created to mimic the bones that they are supporting. These custom-fitted casts are open, which allow the wearer to scratch any itches and also wash the damaged area. Being open also allows for open ventilation. One of the best features is that they can be recycled to create more casts.[161]

3D printing is becoming more popular in the customisable gifts industry, with products such as personalized mobile phone cases and dolls,[162] as well as 3D printed chocolate.[163]
The use of 3D scanning technologies allows the replication of real objects without the use of moulding techniques that in many cases can be more expensive, more difficult, or too invasive to be performed, particularly for precious or delicate cultural heritage artifacts[164] where direct contact with the moulding substances could harm the original object's surface.
Critical making refers to the hands on productive activities that link digital technologies to society. It is invented to bridge the gap between creative physical and conceptual exploration.[165] The term was popularized by Matt Ratto, an Assistant Professor and director of the Critical Making lab in the Faculty of Information at the University of Toronto. Ratto describes one of the main goals of critical as "to use material forms of engagement with technologies to supplement and extend critical reflection and, in doing so, to reconnect our lived experiences with technologies to social and conceptual critique".[166] The main focus of critical making is open design,[167] which includes, in addition to 3D printing technologies, also other digital software and hardware. People usually reference spectacular design when explaining critical making.[168]
Communication
Employing additive layer technology offered by 3D printing, Terahertz devices which act as waveguides, couplers and bends have been created. The complex shape of these devices could not be achieved using conventional fabrication techniques. Commercially available professional grade printer EDEN 260V was used to create structures with minimum feature size of 100 µm. The printed structures were later DC sputter coated with gold (or any other metal) to create a Terahertz Plasmonic Device.[169]
Domestic use
As of 2012, domestic 3D printing was mainly practiced by hobbyists and enthusiasts. However, little was used for practical household applications, for example, ornamental objects. Some practical examples include a working clock[170] and gears printed for home woodworking machines among other purposes.[171] Web sites associated with home 3D printing tended to include backscratchers, coat hooks, door knobs, etc.[172]
The open source Fab@Home project[57] has developed printers for general use. They have been used in research environments to produce chemical compounds with 3D printing technology, including new ones, initially without immediate application as proof of principle.[93] The printer can print with anything that can be dispensed from a syringe as liquid or paste. The developers of the chemical application envisage both industrial and domestic use for this technology, including enabling users in remote locations to be able to produce their own medicine or household chemicals.[173][174]
3D printing is now working its way into households, and more and more children are being introduced to the concept of 3D printing at earlier ages. The prospects of 3D printing are growing, and as more people have access to this new innovation, new uses in households will emerge.[175]
The OpenReflex SLR film camera was developed for 3D printing as an open-source student project.[176]
Education and research
3D printing, and open source RepRap 3D printers in particular, are the latest technology making inroads into the classroom.[177][178][179] 3D printing allows students to create prototypes of items without the use of expensive tooling required in subtractive methods. Students design and produce actual models they can hold. The classroom environment allows students to learn and employ new applications for 3D printing.[180] RepRaps, for example, have already been used for an educational mobile robotics platform.[181]
Some authors have claimed that RepRap 3D printers offer an unprecedented "revolution" in STEM education.[182] The evidence for such claims comes from both the low cost ability for rapid prototyping in the classroom by students, but also the fabrication of low-cost high-quality scientific equipment from open hardware designs forming open-source labs.[183] Engineering and design principles are explored as well as architectural planning. Students recreate duplicates of museum items such as fossils and historical artifacts for study in the classroom without possibly damaging sensitive collections. Other students interested in graphic designing can construct models with complex working parts. 3D printing gives students a new perspective with topographic maps. Science students can study cross-sections of internal organs of the human body and other biological specimens. And chemistry students can explore 3D models of molecules and the relationship within chemical compounds.[184]
According to a recent paper by Kostakis et al.,[185] 3D printing and design can electrify various literacies and creative capacities of children in accordance with the spirit of the interconnected, information-based world.
Future applications for 3D printing might include creating open-source scientific equipment.[183][186]
Environmental use
In Bahrain, large-scale 3D printing using a sandstone-like material has been used to create unique coral-shaped structures, which encourage coral polyps to colonize and regenerate damaged reefs. These structures have a much more natural shape than other structures used to create artificial reefs, and, unlike concrete, are neither acid nor alkaline with neutral pH.[187]
Archaeology and museums use
In the last several years 3D printing has been intensively used by museums and archaeologists for restoration of damaged artifacts. Many Europeans and North American Museums have purchased 3D printers and actively recreate missing pieceс of their of relics.[188]
The Metropolitan Museum of Art and the British Museum have started using their 3D printers to create museum souvenirs that are available in the museum shops.[189] Other museums, like the National Museum of Military History and Varna Historical Museum, have gone further and sell through the online platform Threeding digital models of their artifacts in 3D printing friendly file format, which everyone can 3D print at home.[190]
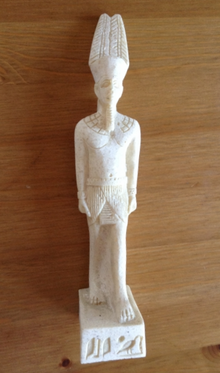
Specialty materials
Consumer grade 3D printing has resulted in new materials that have been developed specifically for 3D printers. For example, filament materials have been developed to imitate wood, in its appearance as well as its texture. Furthermore, new technologies, such as infusing carbon fiber[191] into printable plastics, allowing for a stronger, lighter material. In addition to new structural materials that have been developed due to 3D printing, new technologies have allowed for patterns to be applied directly to 3D printed parts. Iron oxide-free Portland cement powder has been used to create architectural structures up to 9 feet in height.[192][193][194]
Legal aspects
Intellectual property
3D printing has existed for decades within certain manufacturing industries where many legal regimes, including patents, industrial design rights, copyright, and trademark may apply. However, there is not much jurisprudence to say how these laws will apply if 3D printers become mainstream and individuals and hobbyist communities begin manufacturing items for personal use, for non-profit distribution, or for sale.
Any of the mentioned legal regimes may prohibit the distribution of the designs used in 3D printing, or the distribution or sale of the printed item. To be allowed to do these things, where an active intellectual property was involved, a person would have to contact the owner and ask for a licence, which may come with conditions and a price. However, many patent, design and copyright laws contain a standard limitation or exception for 'private', 'non-commercial' use of inventions, designs or works of art protected under intellectual property (IP). That standard limitation or exception may leave such private, non-commercial uses outside the scope of IP rights.
Patents cover inventions including processes, machines, manufactures, and compositions of matter and have a finite duration which varies between countries, but generally 20 years from the date of application. Therefore, if a type of wheel is patented, printing, using, or selling such a wheel could be an infringement of the patent.[195]
Copyright covers an expression[196] in a tangible, fixed medium and often lasts for the life of the author plus 70 years thereafter.[197] If someone makes a statue, they may have copyright on the look of that statue, so if someone sees that statue, they cannot then distribute designs to print an identical or similar statue.
When a feature has both artistic (copyrightable) and functional (patentable) merits, when the question has appeared in US court, the courts have often held the feature is not copyrightable unless it can be separated from the functional aspects of the item.[197] In other countries the law and the courts may apply a different approach allowing, for example, the design of a useful device to be registered (as a whole) as an industrial design on the understanding that, in case of unauthorized copying, only the non-functional features may be claimed under design law whereas any technical features could only be claimed if covered by a valid patent.
Gun legislation and administration
The US Department of Homeland Security and the Joint Regional Intelligence Center released a memo stating that "significant advances in three-dimensional (3D) printing capabilities, availability of free digital 3D printable files for firearms components, and difficulty regulating file sharing may present public safety risks from unqualified gun seekers who obtain or manufacture 3D printed guns," and that "proposed legislation to ban 3D printing of weapons may deter, but cannot completely prevent their production. Even if the practice is prohibited by new legislation, online distribution of these 3D printable files will be as difficult to control as any other illegally traded music, movie or software files."[198]
Internationally, where gun controls are generally stricter than in the United States, some commentators have said the impact may be more strongly felt, as alternative firearms are not as easily obtainable.[199] European officials have noted that producing a 3D printed gun would be illegal under their gun control laws,[200] and that criminals have access to other sources of weapons, but noted that as the technology improved the risks of an effect would increase.[201][202] Downloads of the plans from the UK, Germany, Spain, and Brazil were heavy.[203][204]
Attempting to restrict the distribution over the Internet of gun plans has been likened to the futility of preventing the widespread distribution of DeCSS which enabled DVD ripping.[205][206][207][208] After the US government had Defense Distributed take down the plans, they were still widely available via The Pirate Bay and other file sharing sites.[209] Some US legislators have proposed regulations on 3D printers, to prevent them being used for printing guns.[210][211] 3D printing advocates have suggested that such regulations would be futile, could cripple the 3D printing industry, and could infringe on free speech rights, with early pioneer of 3D printing Professor Hod Lipson suggesting that gunpowder could be controlled instead.[212][213][214][215][216][217][218]
Impact
Additive manufacturing, starting with today's infancy period, requires manufacturing firms to be flexible, ever-improving users of all available technologies to remain competitive. Advocates of additive manufacturing also predict that this arc of technological development will counter globalization, as end users will do much of their own manufacturing rather than engage in trade to buy products from other people and corporations.[5] The real integration of the newer additive technologies into commercial production, however, is more a matter of complementing traditional subtractive methods rather than displacing them entirely.[219]
Social change
Since the 1950s, a number of writers and social commentators have speculated in some depth about the social and cultural changes that might result from the advent of commercially affordable additive manufacturing technology.[220] Amongst the more notable ideas to have emerged from these inquiries has been the suggestion that, as more and more 3D printers start to enter people's homes, so the conventional relationship between the home and the workplace might get further eroded.[221] Likewise, it has also been suggested that, as it becomes easier for businesses to transmit designs for new objects around the globe, so the need for high-speed freight services might also become less.[222] Finally, given the ease with which certain objects can now be replicated, it remains to be seen whether changes will be made to current copyright legislation so as to protect intellectual property rights with the new technology widely available.
As 3D printers became more accessible to consumers, online social platforms have developed to support the community.[223] This includes websites that allow users to access information such as how to build a 3D printer, as well as social forums that discuss how to improve 3D print quality and discuss 3D printing news, as well as social media websites that are dedicated to share 3D models.[224][225][226] RepRap is a wiki based website that was created to hold all information on 3d printing, and has developed into a community that aims to bring 3D printing to everyone. Furthermore, there are other sites such as Pinshape, Thingiverse and MyMiniFactory, which was created initially to allow users to post 3D files for anyone to print, allowing for decreased transaction cost of sharing 3D files. These websites have allowed for greater social interaction between users, creating communities dedicated around 3D printing.
Some [227][228][229] call attention to the conjunction of Commons-based peer production with 3D printing and other low-cost manufacturing techniques. The self-reinforced fantasy of a system of eternal growth can be overcome with the development of economies of scope, and here, the civil society can play an important role contributing to the raising of the whole productive structure to a higher plateau of more sustainable and customized productivity.[227] Further, it is true that many issues, problems and threats rise due to the large democratization of the means of production, and especially regarding the physical ones.[227] For instance, the recyclability of advanced nanomaterials is still questioned; weapons manufacturing could become easier; not to mention the implications on counterfeiting [230] and on IP.[231] It might be maintained that in contrast to the industrial paradigm whose competitive dynamics were about economies of scale, Commons-based peer production and 3D printing could develop economies of scope. While the advantages of scale rest on cheap global transportation, the economies of scope share infrastructure costs (intangible and tangible productive resources), taking advantage of the capabilities of the fabrication tools.[227] And following Neil Gershenfeld [232] in that "some of the least developed parts of the world need some of the most advanced technologies", Commons-based peer production and 3D printing may offer the necessary tools for thinking globally but act locally in response to certain problems and needs.
Larry Summers wrote about the "devastating consequences" of 3D printing and other technologies (robots, artificial intelligence, etc.) for those who perform routine tasks. In his view, "already there are more American men on disability insurance than doing production work in manufacturing. And the trends are all in the wrong direction, particularly for the less skilled, as the capacity of capital embodying artificial intelligence to replace white-collar as well as blue-collar work will increase rapidly in the years ahead." Summers recommends more vigorous cooperative efforts to address the "myriad devices" (e.g., tax havens, bank secrecy, money laundering, and regulatory arbitrage) enabling the holders of great wealth to "avoid paying" income and estate taxes, and to make it more difficult to accumulate great fortunes without requiring "great social contributions" in return, including: more vigorous enforcement of anti-monopoly laws, reductions in "excessive" protection for intellectual property, greater encouragement of profit-sharing schemes that may benefit workers and give them a stake in wealth accumulation, strengthening of collective bargaining arrangements, improvements in corporate governance, strengthening of financial regulation to eliminate subsidies to financial activity, easing of land-use restrictions that may cause the real estate of the rich to keep rising in value, better training for young people and retraining for displaced workers, and increased public and private investment in infrastructure development—e.g., in energy production and transportation.[233]
Michael Spence wrote that "Now comes a … powerful, wave of digital technology that is replacing labor in increasingly complex tasks. This process of labor substitution and disintermediation has been underway for some time in service sectors—think of ATMs, online banking, enterprise resource planning, customer relationship management, mobile payment systems, and much more. This revolution is spreading to the production of goods, where robots and 3D printing are displacing labor." In his view, the vast majority of the cost of digital technologies comes at the start, in the design of hardware (e.g. 3D printers) and, more important, in creating the software that enables machines to carry out various tasks. "Once this is achieved, the marginal cost of the hardware is relatively low (and declines as scale rises), and the marginal cost of replicating the software is essentially zero. With a huge potential global market to amortize the upfront fixed costs of design and testing, the incentives to invest [in digital technologies] are compelling." Spence believes that, unlike prior digital technologies, which drove firms to deploy underutilized pools of valuable labor around the world, the motivating force in the current wave of digital technologies "is cost reduction via the replacement of labor." For example, as the cost of 3D printing technology declines, it is "easy to imagine" that production may become "extremely" local and customized. Moreover, production may occur in response to actual demand, not anticipated or forecast demand. Spence believes that labor, no matter how inexpensive, will become a less important asset for growth and employment expansion, with labor-intensive, process-oriented manufacturing becoming less effective, and that re-localization will appear in both developed and developing countries. In his view, production will not disappear, but it will be less labor-intensive, and all countries will eventually need to rebuild their growth models around digital technologies and the human capital supporting their deployment and expansion. Spence writes that "the world we are entering is one in which the most powerful global flows will be ideas and digital capital, not goods, services, and traditional capital. Adapting to this will require shifts in mindsets, policies, investments (especially in human capital), and quite possibly models of employment and distribution."[234]
Forbes investment pundits have predicted that 3D printing may lead to a resurgence of American Manufacturing, citing the small, creative companies that comprise the current industry landscape, and the lack of the necessary complex infrastructure in typical outsource markets.[235]
See also
- List of 3D printer manufacturers
- List of notable 3D printed weapons and parts
- List of common 3D test models
- List of emerging technologies
- AstroPrint
- 3D printing marketplace
- Additive Manufacturing File Format
- Cloud manufacturing
- Computer numeric control
- Continuous Liquid Interface Production
- Digital modeling and fabrication
- 3D printer extruder
- Fusion3
- Laser cutting
- MakerBot Industries
- Magnetically assisted slip casting
- Mass customization
- Milling center
- Modular design
- Molecular assembler
- Open design
- Open source hardware
- Organ-on-a-chip
- Self-replicating machine
- Shapeways
- Thingiverse
- Volumetric printing
References
- ^ Excell, Jon. "The rise of additive manufacturing". The Engineer. Retrieved 2013-10-30.
- ^ "3D Printer Technology – Animation of layering". Create It Real. Retrieved 2012-01-31.
- ^ http://www.thethirdindustrialrevolution.com/
- ^ http://www.economist.com/node/21552901
- ^ a b c Jane Bird (2012-08-08). "Exploring the 3D printing opportunity". The Financial Times. Retrieved 2012-08-30.
- ^ Hideo Kodama, "A Scheme for Three-Dimensional Display by Automatic Fabrication of Three-Dimensional Model," IEICE TRANSACTIONS on Electronics (Japanese Edition), vol.J64-C, No.4, pp.237–241, April 1981
- ^ Hideo Kodama, "Automatic method for fabricating a three-dimensional plastic model with photo-hardening polymer," Review of Scientific Instruments, Vol. 52, No. 11, pp. 1770–1773, November 1981
- ^ "3D Printing: What You Need to Know". PCMag.com. Retrieved 2013-10-30.
- ^ Apparatus for Production of Three-Dimensional Objects by Stereolithography (8 August 1984)
- ^ Freedman, David H. "Layer By Layer." Technology Review 115.1 (2012): 50–53. Academic Search Premier. Web. 26 July 2013.
- ^ Amon, C. H.; Beuth, J. L.; Weiss, L. E.; Merz, R.; Prinz, F. B. (1998). "Shape Deposition Manufacturing With Microcasting: Processing, Thermal and Mechanical Issues" (PDF). Journal of Manufacturing Science and Engineering. 120 (3). Retrieved 2014-12-20.
- ^ Beck, J.E.; Fritz, B.; Siewiorek, Daniel; Weiss, Lee (1992). "Manufacturing Mechatronics Using Thermal Spray Shape Deposition" (PDF). Proceedings of the 1992 Solid Freeform Fabrication Symposium. Retrieved 2014-12-20.
- ^ Prinz, F. B.; Merz, R.; Weiss, Lee (1997). Ikawa, N. (ed.). Building Parts You Could Not Build Before. Proceedings of the 8th International Conference on Production Engineering. 2-6 Boundary Row, London SE1 8HN, UK: Chapman & Hall. pp. 40–44.
{{cite conference}}
: CS1 maint: location (link) - ^ "Google Ngram of the term additive manufacturing".
- ^ GrabCAD, GE jet engine bracket challenge
- ^ Zelinski, Peter (2014-06-02), "How do you make a howitzer less heavy?", Modern Machine Shop
- ^ a b Pearce, Joshua M.; et al. (2010). "3-D Printing of Open Source Appropriate Technologies for Self-Directed Sustainable Development". Journal of Sustainable Development. 3 (4): 17–29. doi:10.5539/jsd.v3n4p17. Retrieved 2012-01-31.
- ^ a b Tech for Trade, 3D4D Challenge
- ^ Ishengoma, Fredrick R.; Mtaho, Adam B. (2014-10-18). "3D Printing: Developing Countries Perspectives". International Journal of Computer Applications. 104 (11): 30–34. arXiv:1410.5349. doi:10.5120/18249-9329. ISSN 0975-8887.
- ^ a b Jacobs, Paul Francis (1992-01-01). Rapid Prototyping & Manufacturing: Fundamentals of Stereolithography. Society of Manufacturing Engineers. ISBN 9780872634251.
- ^ "Objet Connex 3D Printers". Objet Printer Solutions. Retrieved 2012-01-31.
- ^ Frick, Lindsey. How to Smooth 3D-Printed Parts. Machine Design Magazine, 29 April 2014
- ^ "Substrate Release Mechanisms for Gas Metal Arc 3-D Aluminum Metal Printing". 3D Printing and Additive Manufacturing. 1 (4): 204–209. 2014. doi:10.1089/3dp.2014.0015 (inactive 2015-12-05).
{{cite journal}}
: Unknown parameter|authors=
ignored (help)CS1 maint: DOI inactive as of December 2015 (link) - ^ Amberlee S. Haselhuhn, Bas Wijnen, Gerald C. Anzalone, Paul G. Sanders, Joshua M. Pearce, In Situ Formation of Substrate Release Mechanisms for Gas Metal Arc Weld Metal 3-D Printing. Journal of Materials Processing Technology. 226, pp. 50–59 (2015) DOI: 10.1016/j.jmatprotec.2015.06.038 10.1016/j.jmatprotec.2015.06.038
- ^ FDM is a proprietary term owned by Stratasys. All 3-D printers that are not Stratasys machines and use a fused filament process are referred to as or fused filament fabrication (FFF).
- ^ Sherman, Lilli Manolis (November 15, 2007). "A whole new dimension – Rich homes can afford 3D printers". The Economist.
- ^ Wohlers, Terry. "Factors to Consider When Choosing a 3D Printer (WohlersAssociates.com, Nov/Dec 2005)".
- ^ www.3ders.org (2012-09-25). "Casting aluminum parts directly from 3D printed PLA parts". 3ders.org. Retrieved 2013-10-30.
{{cite web}}
: CS1 maint: numeric names: authors list (link) - ^ "Affordable 3D Printing with new Selective Heat Sintering (SHS™) technology". blueprinter.
- ^ Chee Kai Chua; Kah Fai Leong; Chu Sing Lim (2003). Rapid Prototyping. World Scientific. p. 124. ISBN 978-981-238-117-0.
- ^ Frick, Lindsey. Aluminum-powder DMLS-printed part finishes race first. Machine Design Magazine, 3 March 2014
- ^ Deckard, C., "Method and apparatus for producing parts by selective sintering", U.S. patent 4,863,538, filed October 17, 1986, published September 5, 1989.
- ^ Housholder, R., "Molding Process", U.S. patent 4,247,508, filed December 3, 1979, published January 27, 1981.
- ^ Hiemenz, Joe. "Rapid prototypes move to metal components (EE Times, 3/9/2007)".
- ^ "Rapid Manufacturing by Electron Beam Melting". SMU.edu.
- ^ "3D Printer Uses Standard Paper", "Rapid Today", May, 2008
- ^ U.S. patent 4,575,330
- ^ NSF JTEC/WTEC Panel Report-RPA
- ^ Beaumont Newhall (May 1958) "Photosculpture," Image, 7 (5) : 100–105
- ^ François Willème, "Photo-sculpture," U.S. Patent no. 43,822 (August 9, 1864). Available on-line at: U.S. patent 43,822
- ^ François Willème (May 15, 1861) "La sculpture photographique", Le Moniteur de la photographie, p. 34.
- ^ "EnvisionTEC Perfactory". EnvisionTEC.
- ^ Johnson, R. Colin. "Cheaper avenue to 65 nm? (EE Times, 3/30/2007)".
- ^ "The World's Smallest 3D Printer". TU Wien. 12 September 2011.
- ^ a b c d "3D-printing multi-material objects in minutes instead of hours". Kurzweil Accelerating Intelligence. November 22, 2013.
- ^ Yuenyong Nilsiam, Amberlee Haselhuhn, Bas Wijnen, Paul Sanders, & Joshua M. Pearce. Integrated Voltage - Current Monitoring and Control of Gas Metal Arc Weld Magnetic Ball-Jointed Open Source 3-D Printer.Machines 3(4), 339-351 (2015).
- ^ A. Pinar, B. Wijnen, G. C. Anzalone, T. C. Havens, P. G. Sanders, J. M. Pearce. Low-cost Open-Source Voltage and Current Monitor for Gas Metal Arc Weld 3-D Printing. Journal of Sensors Vol. 2015, Article ID 876714, 8 pages, 2015.
- ^ a b c "3D Printing: Challenges and Opportunities for International Relations". Council on Foreign Relations. October 23, 2013. Retrieved 2013-10-30.
- ^ Kalish, Jon. "A Space For DIY People To Do Their Business (NPR.org, November 28, 2010)". Retrieved 2012-01-31.
- ^ Jones, R., Haufe, P., Sells, E., Iravani, P., Olliver, V., Palmer, C., & Bowyer, A. (2011). Reprap-- the replicating rapid prototyper. Robotica, 29(1), 177-191.
- ^ "Open source 3D printer copies itself". Computerworld New Zealand. 2008-04-07. Retrieved 2013-10-30.
- ^ RepRap blog 2009 visited 2/26/2014
- ^ An Inexpensive Way to Print Out Metal Parts – The New York Times
- ^ Gerald C. Anzalone, Chenlong Zhang, Bas Wijnen, Paul G. Sanders and Joshua M. Pearce, " Low-Cost Open-Source 3-D Metal Printing" IEEE Access, 1, pp.803–810, (2013). doi: 10.1109/ACCESS.2013.2293018
- ^ Disruptions: 3-D Printing Is on the Fast Track – NYTimes.com
- ^ a b c www.3ders.org. "3D printers list with prices". 3ders.org. Retrieved 2013-10-30.
{{cite web}}
: CS1 maint: numeric names: authors list (link) - ^ a b New Scientist magazine: Desktop fabricator may kick-start home revolution, 9 January 2007
- ^ "3D printer by Saskatchewan man gets record crowdsourced cash". Saskatchewan: CBC News. 6 November 2013. Retrieved 8 November 2013.
- ^ "Rapide One – Affordable Professional Desktop 3D Printer by Rapide 3D". Indiegogo. December 2, 2013. Retrieved 20 January 2014.
- ^ Pogue, David. "A Review Of The 3Doodler Pen, Which Raised Over $2 Million On Kickstarter". Yahoo Tech. Retrieved 13 March 2014
- ^ Dorrier, Jason. "Kickstarter 3Doodler 3D Printing Pen Nothing of the Sort – But Somehow Raises $2 Million". Singularity Hub. Retrieved 13 March 2014
- ^ a b Wittbrodt, B. T.; Glover, A. G.; Laureto, J.; Anzalone, G. C.; Oppliger, D.; Irwin, J. L.; Pearce, J. M. (2013). "Life-cycle economic analysis of distributed manufacturing with open-source 3-D printers". Mechatronics. 23 (6): 713. doi:10.1016/j.mechatronics.2013.06.002.
- ^ Kreiger, M.; Pearce, J. M. (2013). "Environmental Life Cycle Analysis of Distributed Three-Dimensional Printing and Conventional Manufacturing of Polymer Products". ACS Sustainable Chemistry & Engineering: 131002082320002. doi:10.1021/sc400093k.
- ^ Christian Baechler, Matthew DeVuono, and Joshua M. Pearce, "Distributed Recycling of Waste Polymer into RepRap Feedstock". Rapid Prototyping Journal, 19 (2), pp. 118-125 (2013). DOI:10.1108/13552541311302978
- ^ Kreiger, M., Anzalone, G. C., Mulder, M. L., Glover, A., & Pearce, J. M. (2013). Distributed Recycling of Post-Consumer Plastic Waste in Rural Areas. MRS Online Proceedings Library, 1492, mrsf12-1492
- ^ See for example the Rostock
- ^ Vandendriessche, Pieter-Jan. "delta 3D printer accuracy".
- ^ Titsch, Mike (July 11, 2013). "MatterHackers Opens 3D Printing Store and Releases MatterControl 0.7.6". Retrieved November 30, 2013.
- ^ "Hoosier Daddy – The Largest Delta 3D Printer In the World". 3D Printer World. Punchbowl Media. 23 July 2014. Retrieved 28 September 2014.
- ^ McKenna, Beth (26 April 2014). "The Next Big Thing in 3-D Printing: Big Area Additive Manufacturing, or BAAM". The Motley Fool. Retrieved 28 September 2014.
- ^ J. L. Wasserman; et al. (2008). "Fabrication of One-Dimensional Programmable-Height Nanostructures via Dynamic Stencil Deposition". Review of Scientific Instruments. 79 (7): 073909. arXiv:0802.1848. doi:10.1063/1.2960573.
- ^ patel, Prachi (5 March 2013). "Micro 3-D Printer Creates Tiny Structures in Seconds". MIT Technology Review.
- ^ "Print me a Stradivarius – How a new manufacturing technology will change the world". Economist Technology. 2011-02-10. Retrieved 2012-01-31.
- ^ Zelinski, Peter (2014-06-25). "Video: World's largest additive metal manufacturing plant". Modern Machine Shop.
- ^ Sherman, Lilli Manolis. "3D Printers Lead Growth of Rapid Prototyping (Plastics Technology, August 2004)". Retrieved 2012-01-31.
- ^ "3D printing: 3D printing scales up". The Economist. 2013-09-07. Retrieved 2013-10-30.
- ^ Development of a Three-Dimensional Printed, Liquid-Cooled Nozzle for a Hybrid Rocket Motor, Nick Quigley and James Evans Lyne, Journal of Propulsion and Power, Vol. 30, No. 6 (2014), pp. 1726–1727.
- ^ a b Vincent & Earls 2011
- ^ G. Anzalone, B. Wijnen, Joshua M. Pearce, (2015) "Multi-material additive and subtractive prosumer digital fabrication with a free and open-source convertible delta RepRap 3-D printer", Rapid Prototyping Journal, 21(5), pp.506–519. doi:10.1108/RPJ-09-2014-0113
- ^ Felix Bopp (2010). Future Business Models by Additive Manufacturing. Verlag. ISBN 3-8366-8508-6. Retrieved 4 July 2014.
- ^ Wu, D., Thames, J.L., Rosen, D.W., & Schaefer, D. (2013). Enhancing the Product Realization Process with Cloud-Based Design and Manufacturing Systems. Transactions of the ASME, Journal of Computing and Information Science in Engineering (JCISE), 13(4): 041004-041004-14. doi:10.1115/1.4025257
- ^ Wu, D., Rosen, D.W., Wang, L., & Schaefer, D. (2015). Cloud-Based Design and Manufacturing: A New Paradigm in Digital Manufacturing and Design Innovation. Computer-Aided Design, 59(1): 1-14, DOI: 10.1016/j.cad.2014.07.006.
- ^ Wu, D., Rosen, D.W., & Schaefer, D. (2015). Scalability Planning for Cloud-Based Manufacturing Systems. Transactions of the ASME, Journal of Manufacturing Science and Engineering, DOI:10.1115/1.4030266.
- ^ "3D Hubs: Like Airbnb For 3D Printers". gizmodo. Retrieved 2014-07-05.
- ^ Sterling, Bruce (2011-06-27). "Spime Watch: Dassault Systèmes' 3DVIA and Sculpteo (Reuters, June 27, 2011)". Wired. Archived from the original on 15 April 2014. Retrieved 2012-01-31.
- ^ Vance, Ashlee (2011-01-12). "The Wow Factor of 3-D Printing (The New York Times, January 12, 2011)". Retrieved 2012-01-31.
- ^ "The action doll you designed, made real". makie.me. Retrieved January 18, 2013.
- ^ "Cubify — Express Yourself in 3D". myrobotnation.com. Retrieved 2014-01-25.
- ^ "Turn Your Baby's Cry Into an iPhone Case". Bloomberg Businessweek. 2012-03-10. Retrieved 2013-02-20.
{{cite journal}}
: Invalid|ref=harv
(help) - ^ "Nokia backs 3D printing for mobile phone cases". BBC News Online. 2013-02-18. Retrieved 2013-02-20.
{{cite journal}}
: Cite journal requires|journal=
(help); Invalid|ref=harv
(help) - ^ Wohlers Report 2009, State of the Industry Annual Worldwide Progress Report on Additive Manufacturing, Wohlers Associates, ISBN 978-0-9754429-5-1
- ^ Hopkinson, N & Dickens, P 2006, 'Emerging Rapid Manufacturing Processes', in Rapid Manufacturing; An industrial revolution for the digital age, Wiley & Sons Ltd, Chichester, W. Sussex
- ^ a b c Symes, M. D.; Kitson, P. J.; Yan, J.; Richmond, C. J.; Cooper, G. J. T.; Bowman, R. W.; Vilbrandt, T.; Cronin, L. (2012). "Integrated 3D-printed reactionware for chemical synthesis and analysis". Nature Chemistry. 4 (5): 349–354. doi:10.1038/nchem.1313. PMID 22522253.
- ^ "Hydrocolloid Printing", Cornell Creative, 2012
- ^ A Guide to All the Food That's Fit to 3D Print (So Far)
- ^ "Foodini 3D Printer Cooks Up Meals Like the Star Trek Food Replicator". Retrieved 27 January 2015.
- ^ "3D Printing: Food in Space". NASA. Retrieved 2015-09-30.
- ^ ted.com, Lee Cronin: Print your own medicine
- ^ "RFA-HD-15-023: Use of 3-D Printers for the Production of Medical Devices (R43/R44)". NIH grants. Retrieved 2015-09-30.
- ^ a b "3D Printed Clothing Becoming a Reality". Resins Online. 2013-06-17. Retrieved 2013-10-30.
- ^ Michael Fitzgerald (2013-05-28). "With 3-D Printing, the Shoe Really Fits". MIT Sloan Management Review. Retrieved 2013-10-30.
- ^ "3D Custom Eyewear The Next Focal Point For 3D Printing". Rakesh Sharma. 2013-09-10. Retrieved 2013-09-10.
- ^ "Koenigsegg One:1 Comes With 3D Printed Parts". Business Insider. Retrieved 2014-05-14.
- ^ tecmundo.com.br/ Conheça o Urbee, primeiro carro a ser fabricado com uma impressora 3D
- ^ The "Urbee" 3D-Printed Car: Coast to Coast on 10 Gallons Truthout
- ^ 3D Printed Car Creator Discusses Future of the Urbee
- ^ Simmons, Dan (May 6, 2015). "Airbus had 1,000 parts 3D printed to meet deadline". BBC. Retrieved 2015-11-27.
- ^ Zitun, Yoav (July 27, 2015). "The 3D printer revolution comes to the IAF". Ynet News. Retrieved 2015-09-29.
- ^ Knippers, E. "Architecture | Leapfrog 3D Printers". www.lpfrg.com. Retrieved 2015-09-29.
- ^ "Concept Modeling, Realistic 3D Printed Models | Stratasys". www.stratasys.com. Retrieved 2015-09-29.
- ^ Khoshnevis, B.; Russell, R.; Kwon, Hongkyu; Bukkapatnam, S. (2001-09-01). "Crafting large prototypes". IEEE Robotics Automation Magazine. 8 (3): 33–42. doi:10.1109/100.956812. ISSN 1070-9932.
- ^ Greenberg, Andy (2012-08-23). "'Wiki Weapon Project' Aims To Create A Gun Anyone Can 3D-Print At Home". Forbes. Retrieved 2012-08-27.
- ^ Poeter, Damon (2012-08-24). "Could a 'Printable Gun' Change the World?". PC Magazine. Retrieved 2012-08-27.
- ^ "Blueprints for 3-D printer gun pulled off website". statesman.com. May 2013. Retrieved 2013-10-30.
- ^ Samsel, Aaron. "3D Printers, Meet Othermill: A CNC machine for your home office (VIDEO)". Guns.com. Retrieved 2013-10-30.
- ^ "The Third Wave, CNC, Stereolithography, and the end of gun control". Popehat. Retrieved 2013-10-30.
- ^ Rosenwald, Michael S. (2013-02-25). "Weapons made with 3-D printers could test gun-control efforts". Washington Post.
- ^ "Making guns at home: Ready, print, fire". The Economist. 2013-02-16. Retrieved 2013-10-30.
- ^ Rayner, Alex (6 May 2013). "3D-printable guns are just the start, says Cody Wilson". The Guardian. London.
- ^ Manjoo, Farhad (2013-05-08). "3-D-printed gun: Yes, it will be possible to make weapons with 3-D printers. No, that doesn't make gun control futile". Slate.com. Retrieved 2013-10-30.
- ^ a b Franzen, Carl. "3D-printed gun maker in Japan sentenced to two years in prison". The Verge.
- ^ "Transplant jaw made by 3D printer claimed as first". BBC. 2012-02-06.
- ^ Rob Stein (2013-03-17). "Doctors Use 3-D Printing To Help A Baby Breathe". NPR.
- ^ Moore, Calen (11 February 2014). "Surgeons have implanted a 3-D-printed pelvis into a U.K. cancer patient". fiercemedicaldevices.com. Retrieved 4 March 2014.
- ^ Keith Perry (12 March 2014). "Man makes surgical history after having his shattered face rebuilt using 3D printed parts". London: The Daily Telegraph. Retrieved 12 March 2014.
- ^ Research into Julie Williams, 3D-Bioprinting may soon produce transplantable human tissues Mar.6, 2014
- ^ BBC News (October 2014). "Inverness girl Hayley Fraser gets 3D-printed hand", BBC News, 01 October 2014. Retrieved 02 October 2014.
- ^ "3D-Printed Foot Lets Crippled Duck Walk Again".
- ^ Pleasance, Chris (18 August 2014). "Puppy power: Chihuahua born without front legs is given turbo-charged makeover after being fitted with 3D printed body harness and a set of skateboard wheels". The Daily Mail. Retrieved 2014-08-21.
- ^ Flaherty, Joseph (2013-07-30). "So Cute: Hermit Crabs Strut in Stylish 3-D Printed Shells". Wired.
- ^ Weintraub, Arlene (20 March 2015). "3D Systems preps for global launch of 'printed' knee implants for dogs". fierceanimalhealth
.com. Retrieved 13 April 2015. {{cite web}}
: External link in
(help)|publisher=
- ^ Saxena, Varun (2 February 2015). "FDA clears 3-D printed device for minimally invasive foot surgery". fiercemedicaldevices
.com. Retrieved 14 April 2015. {{cite web}}
: External link in
(help)|publisher=
- ^ "3D-printed sugar network to help grow artificial liver", BBC, 2 July 2012
- ^ "Invetech helps bring bio-printers to life". Australian Life Scientist. Westwick-Farrow Media. December 11, 2009. Retrieved December 31, 2013.
- ^ "Building body parts with 3D printing", The Engineer, 24 May 2010
- ^ Silverstein, Jonathan. "'Organ Printing' Could Drastically Change Medicine (ABC News, 2006)". Retrieved 2012-01-31.
- ^ Dan Thomas, Engineering Ourselves – The Future Potential Power of 3D-Bioprinting?, engineering.com, March 25, 2014
- ^ The Diplomat (2013-08-15). "Chinese Scientists Are 3D Printing Ears and Livers – With Living Tissue". Tech Biz. The Diplomat. Retrieved 2013-10-30.
- ^ "How do they 3D print kidney in China". Retrieved 2013-10-30.
- ^ "Mish's Global Economic Trend Analysis: 3D-Printing Spare Human Parts; Ears and Jaws Already, Livers Coming Up ; Need an Organ? Just Print It". Globaleconomicanalysis.blogspot.co.uk. 2013-08-18. Retrieved 2013-10-30.
- ^ Palmer, Eric (3 August 2015). "Company builds plant for 3DP pill making as it nails first FDA approval". fiercepharmamanufacturing.com. Retrieved 4 August 2015.
- ^ The Almost Completely Open Source Laptop Goes on Sale
- ^ Robots And 3D Printing
- ^ Why to Use 3D Printers and the Best 3D Printers To Build Your Own Robot
- ^ Printoo: Giving Life to Everyday Objects (paper-thin, flexible Arduino-compatible modules)
- ^ 3&DBot: An Arduino 3D printer-robot with wheels
- ^ A lesson in building a custom 3D printed humanoid robot
- ^ "New horizons open with space-based 3D printing". SPIE Newsroom. Retrieved 1 April 2015.
- ^ Hays, Brooks (2014-12-19). "NASA just emailed the space station a new socket wrench". Retrieved 2014-12-20.
- ^ Brabaw, Kasandra (2015-01-30). "Europe's 1st Zero-Gravity 3D Printer Headed for Space". Retrieved 2015-02-01.
- ^ Wood, Anthony (2014-11-17). "POP3D to be Europe's first 3D printer in space". Retrieved 2015-02-01.
- ^ Raval, Siddharth (2013-03-29). "SinterHab: A Moon Base Concept from Sintered 3D-Printed Lunar Dust". Space Safety Magazine. Retrieved 2013-10-15.
- ^ "The World's First 3D-Printed Building Will Arrive In 2014". TechCrunch. 2012-01-20. Retrieved 2013-02-08.
- ^ Diaz, Jesus (2013-01-31). "This Is What the First Lunar Base Could Really Look Like". Gizmodo. Retrieved 2013-02-01.
- ^ "The RepRap's Heritage".
- ^ Kelly, Heather (July 31, 2013). "Study: At-home 3D printing could save consumers "thousands"". CNN.
- ^ Séquin, C. H. (2005). "Rapid prototyping". Communications of the ACM. 48 (6): 66. doi:10.1145/1064830.1064860.
- ^ Guth, Robert A. "How 3-D Printing Figures To Turn Web Worlds Real (The Wall Street Journal, December 12, 2007)" (PDF). Retrieved 2012-01-31.
- ^ iPad iPhone Android TIME TV Populist The Page (2008-04-03). "Bathsheba Grossman's Quin.MGX for Materialise listed in Time Magazine's Design 100". Time.com. Retrieved 2013-10-30.
- ^ Williams, Holly (2011-08-28). "Object lesson: How the world of decorative art is being revolutionised by 3D printing (The Independent, 28 August 2011)". London. Retrieved 2012-01-31.
- ^ Bennett, Neil (November 13, 2013). "How 3D printing is helping doctors mend you better". TechAdvisor.
- ^ "Custom Bobbleheads". Retrieved 13 January 2015.
- ^ "3D-print your face in chocolate for that special Valentine's Day gift". The Guardian. 25 January 2013.
- ^ Cignoni, P.; Scopigno, R. (2008). "Sampled 3D models for CH applications". Journal on Computing and Cultural Heritage. 1: 1. doi:10.1145/1367080.1367082.
- ^ DiSalvo, C (2009). "Design and the Construction of Publics". Design Issues. 1. 25: 48. doi:10.1162/desi.2009.25.1.48.
- ^ Ratto, M.; Ree, R. (2012). "Materializing information: 3D printing and social change". First Monday. 17 (7).
{{cite journal}}
: Unknown parameter|last-author-amp=
ignored (|name-list-style=
suggested) (help) - ^ Ratto, Matt (2011). "Open Design and Critical Making". Open Design Now: Why Design Cannot Remain Exclusive.
- ^ Lukens, Jonathan. "Speculative Design and Technological Fluency". International Journal of Learning and Media. 3: 23–39. doi:10.1162/ijlm_a_00080.
- ^ Pandey, S.; Gupta, B.; Nahata, A. (2013). "Complex Geometry Plasmonic Terahertz Waveguides Created via 3D Printing". Cleo: 2013. pp. CTh1K.CTh12. doi:10.1364/CLEO_SI.2013.CTh1K.2. ISBN 978-1-55752-972-5.
- ^ ewilhelm. "3D printed clock and gears". Instructables.com. Retrieved 2013-10-30.
- ^ 23/01/2012 (2012-01-23). "Successful Sumpod 3D printing of a herringbone gear". 3d-printer-kit.com. Retrieved 2013-10-30.
{{cite web}}
:|author=
has numeric name (help) - ^ Search engine for 3D printable models, "backscratcher", etc.
- ^ New Scientist magazine: Make your own drugs with a 3D printer, 17 April 2012
- ^ Cronin, Lee (2012-04-17). "3D printer developed for drugs" (video interview [5:21]). Glasgow University: BBC News Online. Retrieved 2013-03-06.
- ^ D'Aveni, Richard. "3-D Printing Will Change the World". Harvard Business Review. Retrieved October 8, 2014.
- ^ "3D printable SLR brings whole new meaning to "digital camera"". Gizmag.com. Retrieved 2013-10-30.
- ^ Schelly, C., Anzalone, G., Wijnen, B., & Pearce, J. M. (2015). "Open-source 3-D printing Technologies for education: Bringing Additive Manufacturing to the Classroom." Journal of Visual Languages & Computing.
- ^ Grujović, N., Radović, M., Kanjevac, V., Borota, J., Grujović, G., & Divac, D. (2011, September). "3D printing technology in education environment." In 34th International Conference on Production Engineering (pp. 29–30).
- ^ Mercuri, R., & Meredith, K. (2014, March). "An educational venture into 3D Printing." In Integrated STEM Education Conference (ISEC), 2014 IEEE (pp. 1–6). IEEE.
- ^ Students Use 3D Printing to Reconstruct Dinosaurs – YouTube
- ^ Gonzalez-Gomez, J., Valero-Gomez, A., Prieto-Moreno, A., & Abderrahim, M. (2012). "A new open source 3d-printable mobile robotic platform for education." In Advances in Autonomous Mini Robots (pp. 49–62). Springer Berlin Heidelberg.
- ^ J. Irwin, J.M. Pearce, D. Opplinger, and G. Anzalone. The RepRap 3-D Printer Revolution in STEM Education, 121st ASEE Annual Conference and Exposition, Indianapolis, IN. Paper ID #8696 (2014).
- ^ a b Zhang, C.; Anzalone, N. C.; Faria, R. P.; Pearce, J. M. (2013). De Brevern, Alexandre G (ed.). "Open-Source 3D-Printable Optics Equipment". PLoS ONE. 8 (3): e59840. doi:10.1371/journal.pone.0059840. PMC 3609802. PMID 23544104.
{{cite journal}}
: CS1 maint: unflagged free DOI (link) - ^ 3D Printing in the Classroom to Accelerate Adoption of Technology. On 3D Printing
- ^ Kostakis, V.; Niaros, V.; Giotitsas, C. (2014): Open source 3D printing as a means of learning: An educational experiment in two high schools in Greece. In: Telematics and Informatics
- ^ Pearce, Joshua M. 2012. "Building Research Equipment with Free, Open-Source Hardware." Science 337 (6100): 1303–1304
- ^ "Underwater City: 3D Printed Reef Restores Bahrain's Marine Life". ptc.com. 2013-08-01. Retrieved 2013-10-30.
- ^ Museum uses 3D printing to take fragile maquette by Thomas Hart Benton on tour through the States
- ^ British Museum releases scans of artefacts to let you 3D print your own museum at home
- ^ Threeding Uses Artec 3D Scanning Technology to Catalog 3D Models for Bulgaria’s National Museum of Military History
- ^ Eitel, Elisabeth. MarkForged: $5,000 3D printer prints carbon-fiber parts. Machine Design Magazine, 7 March 2014
- ^ "Researchers at UC Berkeley Create Bloom First Ever 3D-printed Cement Structure That Stands 9 Feet Tall". cbs sanfrancisco. 6 March 2015. Retrieved 23 April 2015.
- ^ Chino, Mike (9 March 2015). "UC Berkeley unveils 3D-printed "Bloom" building made of powdered cement". Retrieved 23 April 2015.
- ^ Fixsen, Anna (6 March 2015). "Print it Real Good: First Powder-Based 3D Printed Cement Structure Unveiled". Retrieved 23 April 2015.
- ^ 3D Printing Technology Insight Report, 2014, patent activity involving 3D-Printing from 1990–2013, accessed 2014-06-10
- ^ Clive Thompson on 3-D Printing's Legal Morass. Wired, Clive Thompson 05.30.12 1:43 PM
- ^ a b Weinberg, Michael (January 2013). "What's the Deal with copyright and 3D printing?" (PDF). Institute for Emerging Innovation. Retrieved 2013-10-30.
- ^ "Homeland Security bulletin warns 3D-printed guns may be 'impossible' to stop". Fox News. 2013-05-23. Retrieved 2013-10-30.
- ^ Cochrane, Peter (2013-05-21). "Peter Cochrane's Blog: Beyond 3D Printed Guns". TechRepublic. Retrieved 2013-10-30.
- ^ Gilani, Nadia (2013-05-06). "Gun factory fears as 3D blueprints put online by Defense Distributed | Metro News". Metro.co.uk. Retrieved 2013-10-30.
- ^ "Liberator: First 3D-printed gun sparks gun control controversy". Digitaljournal.com. Retrieved 2013-10-30.
- ^ "First 3D Printed Gun 'The Liberator' Successfully Fired". IBTimes UK. 2013-05-07. Retrieved 2013-10-30.
- ^ "US demands removal of 3D printed gun blueprints". neurope.eu. Retrieved 2013-10-30.
- ^ "España y EE.UU. lideran las descargas de los planos de la pistola de impresión casera". ElPais.com. 2013-05-09. Retrieved 2013-10-30.
- ^ "Controlled by Guns". Quiet Babylon. 2013-05-07. Retrieved 2013-10-30.
- ^ "3dprinting". Joncamfield.com. Retrieved 2013-10-30.
- ^ "State Dept Censors 3D Gun Plans, Citing 'National Security'". News.antiwar.com. 2013-05-10. Retrieved 2013-10-30.
- ^ "Wishful Thinking Is Control Freaks' Last Defense Against 3D-Printed Guns". Reason.com. 2013-05-08. Retrieved 2013-10-30.
- ^ Lennard, Natasha (2013-05-10). "The Pirate Bay steps in to distribute 3-D gun designs". Salon.com. Archived from the original on 2013-05-19. Retrieved 2013-10-30.
- ^ "Sen. Leland Yee Proposes Regulating Guns From 3-D Printers". CBS Sacramento. 2013-05-08. Retrieved 2013-10-30.
- ^ Schumer Announces Support For Measure To Make 3D Printed Guns Illegal
- ^ "Four Horsemen of the 3D Printing Apocalypse". Makezine.com. 2011-06-30. Retrieved 2013-10-30.
- ^ Ball, James (10 May 2013). "US government attempts to stifle 3D-printer gun designs will ultimately fail". The Guardian. London.
- ^ Gadgets (2013-01-18). "Like It Or Not, 3D Printing Will Probably Be Legislated". TechCrunch. Retrieved 2013-10-30.
- ^ Klimas, Liz (2013-02-19). "Engineer: Don't Regulate 3D Printed Guns, Regulate Explosive Gun Powder Instead". TheBlaze.com. Retrieved 2013-10-30.
- ^ Beckhusen, Robert (2013-02-15). "3-D Printing Pioneer Wants Government to Restrict Gunpowder, Not Printable Guns | Danger Room". Wired.com. Retrieved 2013-10-30.
- ^ Bump, Philip (2013-05-10). "How Defense Distributed Already Upended the World". The Atlantic Wire. Archived from the original on 2013-05-19. Retrieved 2013-10-30.
- ^ "News". European Plastics News. Retrieved 2013-10-30.
- ^ Albert 2011
- ^ "Confronting a New 'Era of Duplication'? 3D Printing, Replicating Technology and the Search for Authenticity in George O. Smith's Venus Equilateral Series". Durham University. Retrieved July 21, 2013.
- ^ "Materializing information: 3D printing and social change". Retrieved January 13, 2014.
- ^ "Additive Manufacturing: A supply chain wide response to economic uncertainty and environmental sustainability" (PDF). Retrieved January 11, 2014.
- ^ "Materializing information: 3D printing and social change". Retrieved March 30, 2014.
- ^ "RepRap Options". Retrieved March 30, 2014.
- ^ "3D Printing". Retrieved March 30, 2014.
- ^ "Thingiverse". Retrieved March 30, 2014.
- ^ a b c d Kostakis, V. (2013): At the Turning Point of the Current Techno-Economic Paradigm: Commons-Based Peer Production, Desktop Manufacturing and the Role of Civil Society in the Perezian Framework.. In: TripleC, 11(1), 173–190.
- ^ Kostakis, V.; Papachristou, M. (2014): Commons-based peer production and digital fabrication: The case of a RepRap-based, Lego-built 3D printing-milling machine. In: Telematics and Informatics, 31(3), 434–443
- ^ Kostakis, V; Fountouklis, M; Drechsler, W. (2013): Peer Production and Desktop Manufacturing: The Case of the Helix-T Wind Turbine Project.. In: Science, Technology & Human Values, 38(6), 773 – 800.
- ^ Campbell, Thomas, Christopher Williams, Olga Ivanova, and Banning Garrett. (2011): Could 3D Printing Change the World? Technologies, Potential, and Implications of Additive Manufacturing. Washington: Atlantic Council of the United States
- ^ Bradshaw, Simon, Adrian Bowyer, and Patrick Haufe (2010): The Intellectual Property Implications of Low-Cost 3D Printing. In: SCRIPTed 7
- ^ Gershenfeld, Neil (2007): FAB: The Coming Revolution on your Desktop: From Personal Computers to Personal Fabrication. Cambridge: Basic Books, p. 13–14
- ^ Larry Summers, The Inequality Puzzle, Democracy: A Journal of Ideas, Issue #32, Spring 2014
- ^ Michael Spence, Labor's Digital Displacement (2014-05-22), Project Syndicate
- ^ Can 3D Printing Reshape Manufacturing In America?, Forbes.com 17 June 2014, retrieved 11 Aug 2014
Further reading
- "Results of Make Magazine's 2015 3D Printer Shootout". docs.google.com. Retrieved 1 June 2015.
- "Evaluation Protocol for Make Magazine's 2015 3D Printer Shootout". makezine.com. Retrieved 1 June 2015.
- Vincent; Earls, Alan R. (February 2011). "Origins: A 3D Vision Spawns Stratasys, Inc". Today's Machining World. 7 (1). Oak Forest, Illinois, USA: Screw Machine World Inc.: 24–25.
{{cite journal}}
: Invalid|ref=harv
(help) - "Heat Beds in 3D Printing – Advantages and Equipment". Boots Industries. Retrieved 7 September 2015.
- Albert, Mark [Editor in Chief] (17 January 2011). "Subtractive plus additive equals more than ( − + +=> ): subtractive and additive processes can be combined to develop innovative manufacturing methods that are superior to conventional methods ['Mark: My Word' column – Editor's Commentary]". Modern Machine Shop. 83 (9). Cincinnati, Ohio, USA: Gardner Publications Inc.: 14.
{{cite journal}}
:|first=
has generic name (help); Invalid|ref=harv
(help) - Stephens, B.; Azimi, P.; El Orch, Z.; Ramos, T. (2013). "Ultrafine particle emissions from desktop 3D printers". Atmospheric Environment. 79: 334. doi:10.1016/j.atmosenv.2013.06.050.
- Easton, Thomas A. (November 2008). "The 3D Trainwreck: How 3D Printing Will Shake Up Manufacturing". Analog. 128 (11): 50–63.
{{cite journal}}
: Invalid|ref=harv
(help) - Wright, Paul K. (2001). 21st Century Manufacturing. New Jersey: Prentice-Hall Inc.
External links
