Eye
- This article refers to the sight organ. See Eye (disambiguation) for other usages.
You must add a |reason=
parameter to this Cleanup template – replace it with {{Cleanup|reason=<Fill reason here>}}
, or remove the Cleanup template.
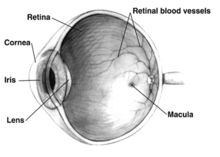
An eye is an organ that detects light. Different kinds of light-sensitive organs are found in a variety of creatures. The simplest eyes do nothing but detect whether the surroundings are light or dark. More complex eyes are used to provide the sense of vision. Many complex organisms including some mammals, birds, reptiles and fish have two eyes which may be placed on the same plane producing a single three-dimensional "image" (binocular vision), as in humans; or on different planes producing two seperate "images" (monocular vision), as in rabbits.
Varieties of eye

Compound eyes are found among the arthropods (insects and kin), and are composed of many simple facets which give a pixelated image (not multiple images as is often believed). Trilobites (now extinct) had a unique form of eye (usually compound) formed from crystals of calcite, incorporating a doublet structure that gave a good field of view despite the rigid lens.
In most vertebrates and some mollusks (such as octopuses) the eye works by projecting images onto a light-sensitive retina, where the light is detected and transmitted to the brain via the optic nerve. The eye is typically roughly spherical, filled with a transparent gel-like substance called the vitreous humour, with a focusing lens and often a muscle called the iris that controls how much light enters. Although they are quite similar in function and appearance once fully developed, vertebrate eyes grow outward from brain cells during embryonic development, while mollusk eyes grow inward from skin cells.
How a complex structure like the eye could have evolved is often said to be a difficult question for the theory of evolution, on the basis that intermediate forms of an eye would presumably have been of little use, and light-sensitive organs are present in a variety of different creatures without any clear evolutionary link. However, eyes in different levels various animals show adaption to their requirements (for example, birds of prey have much greater visual acuity than humans), and the different forms of eye in, for example, vertebrates and mollusks are often cited as examples of parallel evolution.
Structure
The structure of the mammalian eye owes itself completely to the task of focusing light onto the retina. The photosensitive cells of the retina then undergo chemical change in response to the light, the products of this change then trigger an impulse which travels to the brain via the optic nerve. All of the individual components through which light travels within the eye before reaching the retina are transparent, aiming to not dim the light. Several of these components help to converge and focus light rays (as in the cornea and lens respectively).
The pathway of light through the eye begins at the cornea across which it passes from air (or other medium, such as water) into the aqueous humour. The first of the two "humours" connects the cornea with the lens of the eye, it helps maintain the convex shape of the cornea (necessary to the convergence of light at the lens) and provides the corneal endothelium with nutrients. The lens is a convex, flexible disk which focuses light onto the retina. With help from the ciliary body it may change shape, increasing in diametre to grow thinner to focus on far objects and to grow thicker to focus on near objects. In front of the lens is the iris at the centre of which is the pupil. The size of the pupil is adjusted by the circular and radial muscles to maintain a constant level of light entering the eye. Too much light being let in could damage the retina, too light would be blinding. On the other side of the lens is the second humour, the vitreous humour, a jelly-like substance which helps maintain the shape of the eye and suspends the delicate lens. The vitreous humour is bounded on all sides, apart from that with the lens, ciliary body and suspensory ligaments, by the retina.
The eye consists of three layers of tissue surrounding the vitreous humour. The outermost is the sclera which gives most of they eye its white colour. It consists of fibrin connective tissue and both protects the inner components of the eye and maintains its shape. On the inner side of the sclera is the choroid which contains blood vessels which supply the retinal cells with necessary oxygen and removes the waste products of respiration. The choroid gives the inner eye a dark colour, this ensures that all light which hit the bounding layers of the vitreous humour are not reflected within they eye. The inner most layer of the eye is the retina, consisting of the photosensitive rod and cone cells.
To maximise vision and light absorption, the retina is a relatively flat (but curved) and unblemished layer. However, it does have two points at which this is not followed, the fovea and blind spot. The fovea is a dip in the retina directly opposite the lens. It gives humans a fairly central vision enabling them to see specific objects in great detail, such as is necessary in reading and watching television. The fovea only contains the high acuity cone cells which are densely packed and are largely responsible for colour vision in humans. The blind spot is a point on the retina, it is at the point at which the optic nerve connects to the eye. The sensory fibres which bundle there mean that no photosensitive cells exist at this point, it is thus "blind".
Focusing
In order for light rays to be brought to a focus they must be refracted. The amount of refraction required depends on the distance of the object which is being viewed. A distant object will require less bending of light than a nearer one. Most of the refraction occurs at the cornea which has a fixed curvature. The remainder of the required refraction occurs at the lens. The lens can be pulled flatter or rounder by muscles, which adjust the power of the lens. As we age we lose this ability to adjust the focus. Such a condition is known as presbyopia. There are other refraction errors arising from the shape of the cornea and lens, and from the length of the eyeball. These include myopia, hyperopia, and astigmatism.
Cytology
The eye contains two forms of photosensitive cells - rods and cones. Though structurally and metabolically similar, their function is quite different and are equally important to vision. Rod cells are highly sensitive to light allowing them to respond in dim light and dark conditions. These are the cells which allow humans and other animals to see by moonlight, or with very little available light (as in a dark room). However, they are not sensitive to colour, and have low visual acuity (a measure of detail). This is why the darker conditions become, the less colour objects seem to have. Cone cells, conversely, need high light intensities to respond and have high visual acuity. These are the cells which allow an organism to see colour.
The differences are useful, apart from enabling sight in both dim and light condiitons, humans have given them further application. The fovea, direcly behind the lens, consists of almost totally densely-packed cone cells. This gives humans a highly detailed central vision, allowing us to read, bird watch, or complete any other task which primarily requires looking at things. This does cause problems for some - astronomers cannot see dim stars, or other objects, using central vision because the light from these is not enough to stimulate cone cells. Because cone cells are all that exist directly behind the lens, astronmers have to look at stars through the "corner of their eyes" where rods also exist. The light is then sufficient to stimulate cells, allowing the individual to observe distant stars.
These cells are both photosensitive, but different to different frequencies of light because they both contain a different pigmented photoreceptor protein. Rod cells contain the protein rhodopsin and cone cells contain iodopsin. The process through which these proteins go is quite similar - upon being subjected to electromagnetic radiation of a particular wavelength and intensity (ie. a colour visable light) the protein breaks down into two constituent products. Rhodopsin, of rods, breaks down into opsin and retinal; iodopsin of cones breaks down into photopsin and retinal. The opsin in both opens ion channels on the cell membrane which leads to the generation of an action potential (an impulse which will eventually get to the visual cortex in the brain).
This is the reason why cones and rods enable organisms to see in dark and light conditions - each of the photorecptor proteins requires a different light intensity to break down into the constituent products. Further, synaptic convergence means that several rod cells are connected to a single bipolar cell, which then connects to a single ganglion cell and information is relayed to the visual cortex. Whereas, a single cone cell is connected to a single bipolar cell. Thus, action potentials from rods share neurons, where those froms cones are given their own. This results in the high visual acuity, or the high ability to distinguish between detail, of cone cells and not rods. If a ray of light were to reach just one rod cell this may not be enough to stimulate an action potential. Because several "converge" onto a bipolar cell, enough transmitter molecules reach the synapse of the bipolar cell to attain the threshold level to generage an action potential.
Furthermore, colour is distinguishable when breaking down the iodopsin of cone cells because there are three forms of this protein. One form is broken down by the particular EM wavelength that is red light, another green light, and lastly blue light. In simple terms, this allows human beings to see red, green and blue light. If all three forms of cones are stimulated equally, then white is seen. If none are stimulated, black is seen. Most of the time however, the three forms are stimulated to different extents - resulting in different colours being seen. If, for example, the red and green cones are stimulated to the same extent, and no blue cones are stimulated, yellow is seen. For this reason we call red, green and blue primary colours and the products of mixing two secondary colours. The secondary colours can be further complimented with primary colours to see tertiary colours.
Convergence

When a person stares at an object, the two eyeballs rotate sideways to point to the object, so that the object appears at the center of the image formed in each eye's retina. In order to look at a nearby object, the two eyeballs rotate towards each other so that their eyesight can converge on the object. This is generally referred to as cross-eyed viewing. To see a faraway object, the two eyeballs diverge to become almost parallel to each other. This is known as wall-eyed viewing, where the convergence angle is much smaller than that in a cross-eyed viewing.
Normally, focusing operations of the eyes are coupled to the convergence operations. That is, when looking at a nearby object, the brain automatically increases the refraction index of lenses to focus on the object. At the same time, the brain also rotates the two eyeballs towards each other so they converge on the object.
Understanding of the eye
The eye, including its structure and mechanism, has fascinated scientists and the public in general since ancient times. The discovery of the eye went through two cycles of limiting speculation and freeing observation, which led to a dark age between Galen and Versalius.
Arabic scientists are some of the earliest to have written about and drawn the anatomy of the eye—the earliest known diagram being in Hunain ibn Is-hâq's Book of the Ten Treatises on the Eye. Earlier manuscripts exist with references to diagrams which are not extant. The extent to which we are aware of Græco-Roman understanding of the eye is limited, many manuscripts lacked diagrams. Infact, there are very few extant diagrams of the eye. Thus, we remain confused as to what certain names in manuscripts refer to, and what such objects do.
The pre-Hippocratics largely based their anatomical conceptions of the eye on speculation, rather than empiricism. They recognised the sclera and transparent cornea running flushly as the outer coating of the eye, with an inner layer with pupil, and a fluid at the centre. It was believed, by Alcamaeon and others, that this fluid was the medium of vision and flowed from the eye to the brain via a tube. Aristotle advanced such ideas with empiricism, by dissecting the eyes of animals, and discovering three layers (not two). The fluid was seen to be of a constant consistantancy with the lens forming (or congealing) after death, and the surrounding layers were seen to be juxtaposed. He, and his contemporaries, further put forth the existance of three tubes leading from the eye, not one. One tube from each eye met within the skull.
Alexandrian studies extensively contributed to knowledge of the eye. Aetius tells us that Herophilus dedicated an entire study to they eye which no longer exists. In fact, no manuscripts from the region and time are extant, leading us to rely on Celsius' account—a confused account written by a man who did not know the subject. From Celsius we know that the lens had been recognised, though the anterior chamber is not so. The first and second layers are seen to be apposed, apart from a break at the pupil. The retina forms a sac, or other body, in the rear half of the eye and includes the lens (seen to be half-way into the eye) and fluid. In front of the retina is the empty locus vacuus, still enclosed in the top two layers. He no longer saw a fluid flowing to the brain through some hollow fluid, but likely a continuation of the top twomost layers into the brain (rather than the retina).
Rufus recognised a more modern eye, with conjunctiva, extending as a fourth epithelial layer over the eye. Rufus was the first to recognise a two chambered eye - with one chamber from cornea to lens (filled with water), the other from lens to retina (filled with a egg-white-like substance). Galen remedied some mistakes including the curvature of the cornea and lesn, the nature of the optic nerve, and the existance of a posterior chamber. Though this model is close to the modern understanding of the eye, it was not correct, yet was not advanced upon until after Versalius. A ciliary body was then discovered and the sclera, retina, choroid and cornea were seen to meet at the same point. The two chambers were seen to hold the same fluid as well as the lens being attached to the choroid. Galen, though seeing the optic nerve as solid, continued the notion of a central canal. He further wrote of seven muscles, one more than today are known and knew of the tear ducts.
After Galen a period of speculation is again noted by Arab scientists - the lens modified Galens model to place the lens in the middle of the eye, a notion which lasted until Versalius reversed the era of speculation. He, however, was not an opthalmologist and taught of the eye to a more primitive notion than both that of Galen and Arabian scientists - the cornea was not seen as being of greater curvature and the posterior side of the lens wasn't seen to be larger.
Understanding of the eye had been so slow to develop because the lens was percieved to be the seat of vision, not a tool of vision. This mistake was corrected when Fabricius and his successors correctly placed the lens and developed the modern notion of the structure of the eye. They removed the idea of a seventh muscle (the retractor bulbi) and reinstated the correct curvatures of the lens and cornea, as well as stating the ciliary body as a connective strucutre between the lens and the choroid.
The seventeenth and eighteenth century saw the use of hand-lenses (by Malpighi), microscopes (Leeuwenhoek), preparations for fixing the eye for study (Ruysch) and later the freezing of the eye (Petit). This allowed for detailed study of the eye and an advanced model. Some mistakes persisted such as why the pupil changed size (seen to be vessels of the iris filling with blood), the existance of the posterior chamber, and of course the nature of the retina. In 1722 Leeuwenhoek noted the existance of rods and cones though they were not properly discovered until Treviranus in 1834 by use of a microscope.
Ocular anatomy

- Aqueous humour
- Anterior chamber
- Blind spot
- Canal of Schlemm
- Ciliary muscle (or body)
- Cornea
- Conjunctiva
- Choroid
- Fovea
- Iris
- Lens
- Macula
- Optic disc
- Optic nerve
- Ora serrata
- Posterior chamber
- Pupil
- Rectus medialis
- Retina
- Sclera
- Suspensory ligament
- Tapetum lucidum (not in humans)
- Vitreous humour
- Zonular fibers
Eye related problems
- Achromatopsia
- Age-related macular degeneration
- Aniridia
- Amblyopia
- Anisometropia
- Arc eye
- Astigmatism
- Blindness
- Cataracts
- Color blindness
- Conjunctivitis
- Corrective lenses
- Floaters
- Glaucoma
- Hyperopia
- Keratoconus
- Myopia
- Nyctalopia
- Presbyopia
- Retinal detachment
- Retinopathy
- Scotoma
- Snow blindness
- Strabismus
- Uveitis
See also

- Adaptation
- Accommodation
- Binocular vision
- Crystallin
- Evil eye
- Evolution of the Eye
- Eye color
- Eye contact
- Eye tracking
- Eyeglass prescription
- Macropsia
- Micropsia
- Nictating membrane
- Ocular tremor
- Optometry
- Ophthalmology
- Persistence of vision
- Saccade
- Snellen chart
- Staring contest
- Tears
- Visual acuity
- Visual perception
External links
References
"Anatomy". History of Ophthalmology. 23 April. {{cite web}}
: Check date values in: |date=
and |year=
/ |date=
mismatch (help)