Astronomy in the medieval Islamic world: Difference between revisions
→Armillary spheres: added image |
→Famous Muslim astronomy books: added Shams al-Din al-Khafri |
||
Line 302: | Line 302: | ||
**''Risala fi'l-alat'' (''Treatise on Instruments'') |
**''Risala fi'l-alat'' (''Treatise on Instruments'') |
||
*[[Nasīr al-Dīn al-Tūsī]] (1201-1274) |
*[[Nasīr al-Dīn al-Tūsī]] (1201-1274) |
||
**''Al-Tadhkirah fi'ilm al-hay'ah'' |
**''Al-Tadhkirah fi'ilm al-hay'ah'' (''Momento in astronomy'') |
||
**''[[Zij-i Ilkhani]]'' (''Ilkhanic Tables'') (1272) |
**''[[Zij-i Ilkhani]]'' (''Ilkhanic Tables'') (1272) |
||
*'Umar al-Katibi al-[[Qazwini]] (d. 1277) |
*'Umar al-Katibi al-[[Qazwini]] (d. 1277) |
||
Line 312: | Line 312: | ||
*[[Ulugh Beg]] (1394-1449) |
*[[Ulugh Beg]] (1394-1449) |
||
**''[[Zij-i-Sultani]]'' (1437) |
**''[[Zij-i-Sultani]]'' (1437) |
||
*Shams al-Din al-Khafri (d. 1525) |
|||
**''The complement to the explanation of the momento'' |
|||
==See also== |
==See also== |
Revision as of 19:12, 19 September 2007
- This is a sub-article of Islamic science and Astronomy.
In the history of astronomy, Islamic astronomy or Arabic astronomy refers to the astronomical developments made by the Islamic civilization between the 8th and 17th centuries and written in Arabic. It closely parallels the genesis of other Islamic sciences in its assimilation of foreign material and the amalgamation of the disparate elements of that material to create a science that was essentially Islamic. These included Indian, Sassanid and Hellenistic works in particular, which were translated and built upon.
Some stars in the sky, such as Aldebaran, are still today recognized with their Arabic names.
History
Pre-Islamic Arabs had no scientific astronomy. Their knowledge of stars was only empirical, limited to what they observed regarding the rising and setting of stars. The rise of Islam provoked increased Arab thought in this field.[1]
The foundations of Islamic astronomy closely parallels the genesis of other Islamic sciences in its assimilation of foreign material and the amalgamation of the disparate elements of that material to create a science that was essentially Islamic. These include Indian, Sassanid and Hellenistic works which were translated and built upon.
The science historian Donald Routledge Hill has divided the history of Islamic astronomy into the four following distinct time periods in its history.
700-825
The period of assimilation and syncretisation of earlier Hellenistic, Indian and Sassanid astronomy.
During this period, a number of Sanskrit and Persian texts were translated into Arabic. The most notable of the texts was Zij al-Sindhind,[2] based on the Surya Siddhanta and the works of Brahmagupta, and translated by Muhammad al-Fazari and Yaqūb ibn Tāriq in 777. Sources indicate that the text was translated after, in 770, an Indian astronomer visited the court of Caliph Al-Mansur. Another text translated was the Zij al-Shah, a collection of astronomical tables compiled in Persia over two centuries.
Fragments of text during this period indicate that Arabs adopted the sine function (inherited from Indian trigonometry) instead of the chords of arc used in Hellenistic mathematics.[1]
Islamic interest in astronomy ran parallel to the interest in mathematics. Noteworthy in this regard was the Almagest of Egyptian astronomer Ptolemy (c. 100-178). The Almagest was a landmark work in its field, assembling, as Euclid's Elements had previously done with geometrical works, all extant knowledge in the field of astromony that was known to the author. This work was originally known as The Mathematical Composition, but after it had come to be used as a text in astronomy, it was called The Great Astronomer. The Islamic world called it The Greatest prefixing the Greek work megiste (greatest) with the article al- and it has since been known to the world as Al-megiste or, after popular use in Western translation, Almagest. Ptolemy also produced other works, such as Optics, Harmonica, and some suggest he also wrote Tetrabiblon.
The Almagest was a particularly unifying work for its exhaustive lists of sidereal phenomena. He drew up a list of chronological tables of Assyrian, Persian, Greek, and Roman kings for use in reckoning the lapse of time between known astronomical events and fixed dates. In addition to its relevance to calculating accurate calendars, it linked far and foreign cultures together by a common interest in the stars and astrology. The work of Ptolemy was replicated and refined over the years under Arab, Persian and other Muslim astronomers and astrologers.
Early cosmology
There are several cosmological verses in the Qur'an which some modern writers have interpreted as foreshadowing the Big Bang theory:[3]
[21:30] Don't those who reject faith see that the heavens and the earth were a single entity then We ripped them apart?
[51:47] And the heavens We did create with Our Hands, and We do cause it to expand.
In the 8th century, Ja'far al-Sadiq refuted the geocentric model of the universe common at the time, in which the Earth is not moving and the Sun, Moon and the planets are orbiting around it. He was the first to refute Ptolemy's theory of the sun having two movements, one going round the Earth in one year and the other going round the earth in 24 hours causing day and night. Al-Sadiq argued that if the Sun is moving round the Earth for one year, it cannot suddenly change its course and go round the Earth for one day. He suggested that this could be explained with a heliocentric theory in which the Earth rotates on its axis and around the Sun. Al-Sadiq also wrote a theory on how the universe is expanding and contracting. He also stated that every object in the universe is always in motion, including objects which appear to be inanimate.[4]
Al-Sadiq's student, Geber (Jabir ibn Hayyan), asked him the following question on the stars:
"How does the movement of the stars keep them from falling?"[4]
Al-Sadiq replied:
"Put a stone in a sling and swing it round your head. The stone will stay in the sling so long as you are rotating it. But as soon as you stop the rotation, the stone will fall down on the ground. In the same way the perpetual motion of stars keeps them from falling down."[4]
825-1025
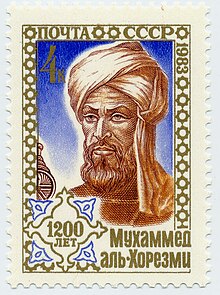
This period of vigorous investigation, in which the superiority of the Ptolemaic system of astronomy was accepted and significant contributions made to it. Astronomical research was greatly supported by the Abbasid caliph al-Mamun. Baghdad and Damascus became the centers of such activity. The caliphs not only supported this work financially, but endowed the work with formal prestige.
The first major Muslim work of astronomy was Zij al-Sindh by al-Khwarizimi in 830. The work contains tables for the movements of the sun, the moon and the five planets known at the time. The work is significant as it introduced Ptolemaic concepts into Islamic sciences. This work also marks the turning point in Islamic astronomy. Hitherto, Muslim astronomers had adopted a primarily research approach to the field, translating works of others and learning already discovered knowledge. Al-Khwarizmi's work marked the beginning of non-traditional methods of study and calculations.[5]
In 850, al-Farghani wrote Kitab fi Jawani ("A compendium of the science of stars"). The book primarily gave a summary of Ptolemic cosmography. However, it also corrected Ptolemy based on findings of earlier Arab astronomers. Al-Farghani gave revised values for the obliquity of the ecliptic, the precessional movement of the apogees of the sun and the moon, and the circumference of the earth. The books were widely circulated through the Muslim world, and even translated into Latin.[6]
Early heliocentric model
In the 9th century, Ja'far ibn Muhammad Abu Ma'shar al-Balkhi developed a planetary model which some have interpreted as a heliocentric model. This is due to his orbital revolutions of the planets being given as heliocentric revolutions rather than geocentric revolutions, and the only known planetary theory in which this occurs is in the heliocentric theory. His work on planetary theory has not survived, but his astronomical data was later recorded by al-Hashimi and Abū Rayhān al-Bīrūnī.[7]
Beginning of celestial mechanics
Celestial mechanics began with the eldest Banū Mūsā brother, Ja'far Muhammad ibn Mūsā ibn Shākir (9th century), who was the first to discover that the heavenly bodies and celestial spheres were subject to the same laws of physics as Earth, unlike the ancients who believed that the celestial spheres followed their own set of physical laws different from that of Earth.[8]
In his Astral Motion and The Force of Attraction, Muhammad ibn Musa discovered that there was a force of attraction between heavenly bodies,[9] foreshadowing Newton's law of universal gravitation.[10]
Beginning of experimental astronomy
In the 11th century, Abū Rayhān al-Bīrūnī introduced the experimental method into astronomy and was the first to conduct elaborate experiments related to astronomical phenomena. He discovered the Milky Way galaxy to be a collection of numerous nebulous stars. In Afghanistan, he observed and described the solar eclipse on April 8, 1019, and the lunar eclipse on September 17, 1019, in detail, and gave the exact latitudes of the stars during the lunar eclipse.[11]
1025-1450
During this period a distinctive Islamic system of astronomy flourished. Within the Greek tradition and its successors it was traditional to separate mathematical astronomy (as typified by Ptolemy) from philosophical cosmology (as typified by Aristotle). Muslim scholars developed a program of seeking a physically real configuration (hay'a) of the universe, that would be consistent with both mathematical and physical principles. Within the context of this hay'a tradition, Muslim astronomers began questioning technical details of the Ptolemaic system of astronomy.[12] These criticisms, however, remained within the geocentric framework and most continued to follow Ptolemy's astronomical paradigm.[13] As the historian of astronomy, A. I. Sabra, noted:
"All Islamic astronomers from Thabit ibn Qurra in the ninth century to Ibn al-Shatir in the fourteenth, and all natural philosophers from al-Kindi to Averroes and later, are known to have accepted what Kuhn has called the "two-sphere universe" ...--the Greek picture of the world as consisting of two spheres of which one, the celestial sphere made up of a special element called aether, concentrically envelops the other, where the four elements of earth, water, air, and fire reside."[14]
Some Muslim scholars, including al-Biruni, discussed whether the Earth moved and considered how this might be consistent with astronomical computations and physical systems.[15] Several Muslim astronomers, most notably Nasīr al-Dīn al-Tūsī and his succesors at the Maragheh school, developed non-Ptolemaic computational models within a geocentric context that were later adapted in the Copernican model in a heliocentric context.
Celestial mechanics
In celestial mechanics, Abū Rayhān al-Bīrūnī described the Earth's gravitation as:[16]
"The attraction of all things towards the centre of the earth."
Al-Biruni also discovered that gravity exists within the heavenly bodies and celestial spheres, and he criticized Aristotle's views of them not having any levity or gravity and of circular motion being an innate property of the heavenly bodies.[17]
In 1121, al-Khazini, in his treatise The Book of the Balance of Wisdom, states:[18]
"For each heavy body of a known weight positioned at a certain distance from the centre of the universe, its gravity depends on the remoteness from the centre of the universe. For that reason, the gravities of bodies relate as their distances from the centre of the universe."
Al-Khazini was thus the first to propose the theory that the gravities of bodies vary depending on their distances from the centre of the Earth. This phenomenon was not proven until Newton's law of universal gravitation in the 18th century.[18]
Beginning of hay'a tradition
Between 1025 and 1028, Ibn al-Haytham (Latinized as Alhacen), began the hay'a tradition of Islamic astronomy with his Al-Shuku ala Batlamyus (Doubts on Ptolemy). While maintaining the physical reality of the geocentric model, he was the first to criticize Ptolemy's astronomical system, for relating actual physical motions to imaginary mathematical points, lines, and circles:
"Ptolemy assumed an arrangement that cannot exist, and the fact that this arrangement produces in his imagination the motions that belong to the planets does not free him from the error he committed in his assumed arrangement, for the existing motions of the planets cannot be the result of an arrangement that is impossible to exist."[19]
Ibn al-Haytham developed a physical structure of the Ptolemaic system in his Treatise on the configuration of the World, or Maqâlah fî hay'at al-‛âlam, which became an influential work in the hay'a tradition.[20] In his Epitome of Astronomy, he insisted that the heavenly bodies "were accountable to the laws of physics".[21] The foundations of telescopic astronomy can also be traced back to Ibn al-Haytham, due to the influence of his optical studies on the later development of the modern telescope.[22]
In 1038, Ibn al-Haytham described the first non-Ptolemaic configuration in The Model of the Motions. His reform excluded cosmology, as he developed a systematic study of celestial kinematics that was completely geometric. This in turn led to innovative developments in infinitesimal geometry.[23] His reformed model was the first to reject the equant[24] and eccentrics,[25] free celestial kinematics from cosmology, and reduce physical entities to geometrical entities. The model also propounded the Earth's rotation about its axis,[26] and the centres of motion were geometrical points without any physical significance, like Johannes Kepler's model centuries later.[27] Ibn al-Haytham also describes an early version of Occam's razor, where he employs only minimal hypotheses regarding the properties that characterize astronomical motions, as he attempts to eliminate from his planetary model the cosmological hypotheses that cannot be observed from Earth.[28]
Early alternative models
In 1030, Abū al-Rayhān al-Bīrūnī discussed the Indian planetary theories of Aryabhata, Brahmagupta and Varahamihira in his Ta'rikh al-Hind (Latinized as Indica). Biruni stated that Brahmagupta and others consider that the earth rotates on its axis and Biruni noted that this does not create any mathematical problems.[29]
Abu Said Sinjari, a contemporary of al-Biruni, suggested the possible heliocentric movement of the Earth around the Sun, which al-Biruni did not reject.[30] Al-Biruni agreed with the Earth's rotation about its own axis, and while he was initially neutral regarding the heliocentric and geocentric models,[31] he considered heliocentrism to be a philosophical problem.[32] He remarked that if the Earth rotates on its axis and moves around the Sun, it would remain consistent with his astronomical parameters:[16][33]
"Rotation of the earth would in no way invalidate astronomical calculations, for all the astronomical data are as explicable in terms of the one theory as of the other. The problem is thus difficult of solution."
In 1031, al-Biruni completed his extensive astronomical encyclopaedia Kitab al-Qanun al-Mas'udi (Latinized as Canon Mas’udicus),[34] in which he recorded his astronomical findings and formulated astronomical tables. In it he presented a geocentric model, tabulating the distance of all the celestial spheres from the central Earth, computed according to the principles of Ptolemy's Almagest.[35] The book introduces the mathematical technique of analysing the acceleration of the planets, and first states that the motions of the solar apogee and the precession are not identical. Al-Biruni also discovered that the distance between the Earth and the Sun is larger than Ptolemy's estimate, on the basis that Ptolemy disregarded the annual solar eclipses.[16][36]
In 1070, Abu Ubayd al-Juzjani, a pupil of Avicenna, proposed a non-Ptolemaic configuration in his Tarik al-Aflak. In his work, he indicated the so-called "equant" problem of the Ptolemic model, and proposed a solution for the problem. He claimed that his teacher Avicenna had also worked out the equant problem.[37]
Andalusian school

In the 11th-12th centuries, astronomers in al-Andalus took up the challenge earlier posed by Ibn al-Haytham, namely to develop an alternate non-Ptolemaic configuration that evaded the errors found in the Ptolemaic model.[38] Like Ibn al-Haytham's critique, the anonymous Andalusian work, al-Istidrak ala Batlamyus (Recapitulation regarding Ptolemy), included a list of objections to Ptolemic astronomy.
In the late 11th century, al-Zarqali (Latinized as Arzachel) discovered that the orbits of the planets are elliptic orbits and not circular orbits,[39] though he still followed the Ptolemaic model.
In the 12th century, Averroes rejected the eccentric deferents introduced by Ptolemy. He rejected the Ptolemaic model and instead argued for a strictly concentric model of the universe. He wrote the following criticism on the Ptolemaic model of planetary motion:[40]
"To assert the existence of an eccentric sphere or an epicyclic sphere is contrary to nature. [...] The astronomy of our time offers no truth, but only agrees with the calculations and not with what exists."
Averroes' contemporary, Maimonides, wrote the following on the planetary model proposed by Ibn Bajjah (Avempace):
"I have heard that Abu Bakr [Ibn Bajja] discovered a system in which no epicycles occur, but eccentric spheres are not excluded by him. I have not heard it from his pupils; and even if it be correct that he discovered such a system, he has not gained much by it, for eccentricity is likewise contrary to the principles laid down by Aristotle.... I have explained to you that these difficulties do not concern the astronomer, for he does not profess to tell us the existing properties of the spheres, but to suggest, whether correctly or not, a theory in which the motion of the stars and planets is uniform and circular, and in agreement with observation."[41]
Later in the 12th century, Ibn Bajjah's successors, Ibn Tufail (Abubacer) and al-Betrugi (Alpetragius), were the first to propose planetary models without any equant, epicycles or eccentrics. Al-Betrugi was also the first to discover that the planets are self-luminous.[42] Their configurations, however, were not accepted due to the numerical predictions of the planetary positions in their models being less accurate than that of the Ptolemaic model,[43] mainly because they followed Aristotle's notion of perfect circular motion.
Maragheh school

From the 13th century, astronomers of the Maragheh school, like the Andalusian astronomers, attempted to solve the equant problem and produce alternative configurations to the Ptolemaic model. They were more successful than their Andalusian predecessors in producing non-Ptolemaic configurations, which eliminated the equant and eccentrics, that were just as accurate as the Ptolemaic model in numerically predicting planetary positions. The most important of the Maragheh astronomers included Mo'ayyeduddin Urdi (d. 1266), Nasir al-Din al-Tusi (1201-1274), 'Umar al-Katibi al-Qazwini (d. 1277), Qutb al-Din al-Shirazi (1236-1311), Sadr al-Sharia al-Bukhari (c. 1347), Ibn al-Shatir (1304-1375), Ala al-Qushji (c. 1474), and Shams al-Din al-Khafri (d. 1550).[44]
Mo'ayyeduddin Urdi (d. 1266) was the first of the Maragheh astronomers to develop a non-Ptolemaic model, and he proposed a new theorem, the "Urdi lemma".[45] Nasir al-Din al-Tusi (1201-1274) resolved significant problems in the Ptolemaic system by developing the Tusi-couple as an alternative to the physically problematic equant introduced by Ptolemy,[46] and conceived a plausible model for elliptical orbits.[34] Tusi's student Qutb al-Din al-Shirazi (1236-1311), in his The Limit of Accomplishment concerning Knowledge of the Heavens, discussed the possibility of heliocentrism. 'Umar al-Katibi al-Qazwini (d. 1277), who also worked at the Maragheh observatory, in his Hikmat al-'Ain, wrote an argument for a heliocentric model, though he later abandoned the idea.[30]
Ibn al-Shatir (1304–1375), in A Final Inquiry Concerning the Rectification of Planetary Theory, incorporated the Urdi lemma, and eliminated the need for an equant by introducing an extra epicycle (the Tusi-couple), departing from the Ptolemaic system in a way that was mathematically identical to what Nicolaus Copernicus did in the 16th century. Ibn al-Shatir's system was also only approximately geocentric, rather than exactly so, having demonstrated trigonometrically that the Earth was not the exact center of the universe.
Y. M. Faruqi wrote:[47]
"Ibn al-Shatir’s theory of lunar motion was very similar to that attributed to Copernicus some 150 years later".
"Whereas Ibn al-Shatir’s concept of planetary motion was conceived in order to play an important role in an earth-centred planetary model, Copernicus used the same concept of motion to present his sun-centred planetary model. Thus the development of alternative models took place that permitted an empirical testing of the models."
Ibn al-Shatir’s rectified model, which included the Tusi-couple and Urdi lemma, was later adapted into a heliocentric model by Copernicus,[46] which was mathematically achieved by reversing the direction of the last vector connecting the Earth to the Sun in Ibn al-Shatir's model.[32] In the published version of his masterwork, De revolutionibus orbium coelestium, Copernicus also cites the theories of al-Battani, Arzachel and Averroes as influences,[34] while the works of Ibn al-Haytham (Alhacen) and al-Biruni were also known in Europe at the time.
1450-1900
The period of stagnation, when the traditional system of astronomy continued to be practised with enthusiasm, but with rapidly decreasing innovation of any major significance. This view, however, has been questioned by George Saliba after studying the works of the 16th century astronomer Shams al-Din al-Khafri (d. 1550), a commentator on earlier Maragheh astronomers. Saliba wrote the following on al-Khafri's work:
"By his sheer insight into the role of mathematics in describing natural phenomena, this astronomer managed to bring the hay'a tradition to such unparalleled heights that could not be matched anywhere else in the world at that time neither mathematically nor astronomically. By working on the alternative mathematical models that could replace those of Ptolemy, and by scrutinizing the works of his predecessors who were all searching for unique mathematical models that could describe the physical phenomena consistently, this astronomer finally realized that all mathematical modeling had no physical truth by itself and was simply another language with which one could describe the physical observed reality. He also realized that the specific phenomena that were being described by the Ptolemaic models did not have unique mathematical solutions that were subject to the same restraints. Rather there were several mathematical models that could account for the Ptolemaic observations, yield identical predictive results at the same critical points used by Ptolemy to construct his own models (thus accounting for the observations as perfectly as Ptolemy could) and still meet the consistency requirement that was imposed by the Aristotelian cosmology which was adopted by the writers in the hay'a tradition."[48]
A large corpus of literature from Islamic astronomy remains today, numbering around at least 10,000 manuscript volumes scattered throughout the world, much of which has not been read or even catalogued. Even so, a reasonably accurate picture of Islamic activity in the field of astronomy can be reconstructed.
Observatories

The first systematic observations in Islam are reported to have taken place under the patronage of al-Ma'mun, and the first Islamic observatories were built in 9th century Iraq under his patronage.
Early observatories
In many private observatories from Damascus to Baghdad, meridian degrees were measured, solar parameters were established, and detailed observations of the Sun, Moon, and planets were undertaken.
In the 10th century, the Buwayhid dynasty encouraged the undertaking of extensive works in Astronomy, such as the construction of a large scale instrument with which observations were made in the year 950CE. We know of this by recordings made in the zij of astronomers such as Ibn al-Alam. The great astronomer Abd Al-Rahman Al Sufi was patronised by prince Adud o-dowleh, who systematically revised Ptolemy's catalogue of stars. Sharaf al-Daula also established a similar observatory in Baghdad. And reports by Ibn Yunus and al-Zarqall in Toledo and Cordoba indicate the use of sophisticated instruments for their time.
It was Malik Shah I who established the first large observatory, probably in Isfahan. It was here where Omar Khayyám with many other collaborators constructed a zij and formulated the Persian Solar Calendar a.k.a. the jalali calendar. A modern version of this calendar is still in official use in Iran today.
Maragheh observatory
The most influential observatory, however, was the Maragheh observatory founded by Nasīr al-Dīn al-Tūsī under the patronage of Hulegu Khan in the 13th century. Here, al-Tusi supervised its technical construction at Maragheh. The facility contained resting quarters for Hulagu Khan, as well as a library and mosque. Some of the top astronomers of the day gathered there, and from their collaboration resulted important modifications to the Ptolemaic system over a period of 50 years.
Samarkand and Istanbul observatories
In 1420, prince Ulugh Beg, himself an astronomer and mathematician, founded another large observatory in Samarkand, the remains of which were excavated in 1908 by Russian teams.
And finally, Taqi al-din bin Ma'ruf founded a large observatory in Istanbul in 1575, which was on the same scale as those in Maragha and Samarkand.
Modern observatories
In modern times, Turkey [1][2]has many well equipped observatories, while Jordan [3], Palestine [4], Lebanon [5], UAE [6], Tunisia [7], and other Arab states are also active as well. Iran has modern facilities at Shiraz University and Tabriz University. In Dec 2005, Physics Today reported of Iranian plans to construct a "world class" facility with a 2.0 m telescope observatory in the near future.[8]
Instruments

Modern knowledge of the instruments used by Muslim astronomers primarily comes from two sources. First the remaining instruments in private and museum collections today, and second the treatises and manuscripts preserved from the Middle Ages.
Muslims made many improvements to instruments already in use before their time, such as adding new scales or details, and invented many of their own new instruments. Their contributions to astronomical instrumentation are abundant. Many of these instruments were often invented or designed for Islamic purposes, such as the determination of the direction of Qibla or the times of Salah.
Astrolabes
Brass astrolabe
Brass astrolabes were developed in much of the Islamic world, chiefly as an aid to finding the qibla. The earliest known example is dated 315 (in the Islamic calendar, corresponding to 927-8CE). The first person credited for building the Astrolabe in the Islamic world is reportedly Fazari (Richard Nelson Frye: Golden Age of Persia. p163). He only improved it though, the Greeks had already invented astrolabes to chart the stars. The Arabs then took it during the Abbasid Dynasty and perfected it to be used to find the beginning of Ramadan, the hours of prayer, and the direction of Mecca.
Mechanical geared astrolabe
The first mechanical astrolabes with gears were invented in the Muslim world, and were perfected by Ibn Samh (c. 1020). One such device with eight gear-wheels was also constructed by Abū Rayhān al-Bīrūnī in 996. These can be considered as an ancestor of the mechanical clocks developed by later Muslim engineers.[49]
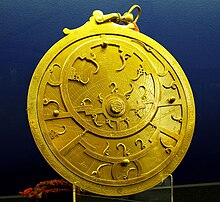
Navigational astrolabe
The first navigational astrolabe was invented in the Islamic world. It employed the use of a polar projection system.[50]
Orthographical astrolabe
Abu Rayhan al-Biruni invented and wrote the earliest treatise on the orthographical astrolabe in the 1000s.[16]
Saphaea
The first astrolabe instruments were used to read the rise of the time of rise of the Sun and fixed stars. Arzachel (al-Zarqali) of Al-Andalus constructed one such instrument in which, unlike its predecessors, did not depend on the latitude of the observer, and could be used anywhere. This universal astrolabe instrument became known in Europe as the "Saphaea".
Linear astrolabe
A famous work by Sharaf al-Dīn al-Tūsī is one in which he describes the linear astrolabe, sometimes called the "staff of al-Tusi", which he invented.[51]
Analog computers

Equatorium
The Equatorium was invented by Abū Ishāq Ibrāhīm al-Zarqālī (Arzachel) in al-Andalus, probably around 1015 CE. It is a mechanical device for finding the positions of the Moon, Sun, and planets, without calculation using a geometrical model to represent the celestial body's mean and anomalistic position.
Planisphere
Abu Rayhan al-Biruni invented and wrote the earliest treatise on the planisphere in the 1000s.[16]
Mechanical geared calendar computer
Abū Rayhān al-Bīrūnī invented the first mechanical lunisolar calendar computer which employed a gear train and eight gear-wheels.[52] This was an early example of a fixed-wired knowledge processing machine.[53]
Mechanical astrolabe with geared calendar computer
In 1235, Abi Bakr of Isfahan invented a brass astrolabe astrolabe with a geared calendar movement based on the design of Abū Rayhān al-Bīrūnī.[54] Abi Bakr's geared astrolabe uses a set of gear-wheels and is the oldest surviving complete mechanical geared machine in existence.[55][56]
Armillary spheres

An armillary sphere had similar applications to a celestial globe. No early Islamic armillary spheres survive, but several treatises on “the instrument with the rings” were written.
Spherical astrolabes
The spherical astrolabe was first produced in the Islamic world.[57] It was an Islamic variation of the astrolabe and the armillary sphere, of which only one complete instrument, from the 14th century, has survived.
Celestial globes
Celestial globes were used primarily for solving problems in celestial astronomy. Today, 126 such instruments remain worldwide, the oldest from the 11th century. The altitude of the sun, or the Right Ascension and Declination of stars could be calculated with these by inputting the location of the observer on the meridian ring of the globe.
Dials
Muslim astronomers and engineers invented a variety of dials for timekeeping.
Sundials
Muslims made several important improvements to the theory and construction of sundials, which they inherited from their Indian and Hellenistic predecessors. Al-Khwarizmi made tables for these instruments which considerably shortened the time needed to make specific calculations. Muslim sundials could also be observed from anywhere on the Earth.
Sundials were frequently placed on mosques to determine the time of prayer. One of the most striking examples was built in the 14th century by the muwaqqit (timekeeper) of the Umayyad Mosque in Damascus, Ibn al-Shatir.[58]
Navicula de Venetiis
This was a universal horary dial invented in 9th century Baghdad. It was used for accurate timekeeping by the Sun and Stars, and could be observed from any latitude.[59] This was later known in Europe as the "Navicula de Venetiis",[60] which was considered the most sophisticated timekeeping instrument of the Renaissance.[61]
Mural instruments
A number of mural instruments were invented by Muslim astronomers and engineers.
Sine quadrant
Several different quadrants were invented by Muslims. Among them was the sine quadrant used for astronomical calculations, invented by Muhammad ibn Mūsā al-Khwārizmī in 9th century Baghdad.[62]
Horary quadrant
The first horary quadrant for specific latitudes was invented by Muhammad ibn Mūsā al-Khwārizmī in 9th century Baghdad.[62] It was used to determine time (especially the times of prayer) by observations of the Sun or stars. A center of the development of quadrants was ninth-century Baghdad.[63]
Quadrans Vetus
This was a universal horary quadrant, an ingeniuous mathematical device invented in 9th century Baghdad and later known as the "Quadrans Vetus" in medieval Europe from the 13th century. It could be used for any latitude on Earth and at any time of the year to determine the time in hours from the altitude of the Sun. This was the second most widely used astronomical instrument during the Middle Ages after the astrolabe. One of its main purposes in the Islamic world was to determine the times of Salah.[62]
Sextant
The first sextant was constructed in Ray, Iran, by Abu-Mahmud al-Khujandi in 994. It was a very large sextant that achieved a high level of accuracy for astronomical measurements, which he described his in his treatise, On the obliquity of the ecliptic and the latitudes of the cities.[64]
In the 15th century, Ulugh Beg constructed the "Fakhri Sextant", which had a radius of approximately 36 meters. Contructed in Samarkand, Uzbekistan, the arc was finely constructed with a staircase on either side to provide access for the assistants who performed the measurements.
Other instruments
In 14th century Egypt, Najm al-Din al-Misri (c. 1325) wrote a treatise describing over 100 different types of scientific and astronomical instruments, many of which he invented himself.[61]
Alhidade
The Alhidade was invented in the Islamic world, while the term "alhidade" is itself derived from Arabic.
Astronomical clocks
The Muslims constructed a variety of highly accurate astronomical clocks for use in their observatories.[65]
Cartographic Qibla instruments
In 17th century Safavid Persia, two unique brass instruments with Mecca-centred world maps engraved on them were produced primarily for the purpose of finding the Qibla. These instruments were engraved with cartographic grids to make it more convenient to find the direction and distance to Mecca at the centre from anywhere on the Earth, which may be based on cartographic grids dating back to 10th century Baghdad.[61]
One of the two instruments, produced by Muhammad Husayn,[66] also had a sundial and compass attached to it.[67]
Shadow square
The shadow square was an instrument used to determine the linear height of an object, in conjunction with the alidade, for angular observations.[68] It was invented by Muhammad ibn Mūsā al-Khwārizmī in 9th century Baghdad.[62]
Famous Muslim astronomy books
- Ibrahim al-Fazari (d. 777) and Muhammad al-Fazari (d. 796/806)
- Az-Zij ‛alā Sinī al-‛Arab (c. 750)
- Yaqūb ibn Tāriq (d. 796)
- Az-Zij al-Mahlul min as-Sindhind li-Darajat Daraja
- Muhammad ibn Mūsā al-Khwārizmī (Latinized as Algorismi) (c. 780-850)
- Zij al-Sindhind (c. 830)
- Ja'far Muhammad ibn Mūsā ibn Shākir (Latinized as Mohammed Ben Musa) (800-873)
- Book on the motion of the orbs
- Astral Motion
- The Force of Attraction
- Ahmad ibn Muhammad ibn Kathīr al-Farghānī (Latinized as Alfraganus) (d. 850)
- Elements of astronomy on the celestial motions (c. 833)
- Kitab fi Jawami Ilm al-Nujum
- Muhammad ibn Jābir al-Harrānī al-Battānī (Latinized as Albategni) (853-929)
- Az-Zij as-Sabi
- Ibn al-Haytham (Latinized as Alhacen) (965-1039)
- On the Configuration of the World
- Doubts concerning Ptolemy (c. 1028)
- The Resolution of Doubts (c. 1029)
- The Model of the Motions of Each of the Seven Planets (1029-1039)
- Abū Rayhān al-Bīrūnī (973-1048)
- Kitab al-Qanun al-Mas'udi (Latinized as Canon Mas’udicus) (1031)
- Al-Zarqali (Latinized as Arzachel) (1028-1087)
- Abu Ubayd al-Juzjani (c. 1070)
- Tarik al-Aflak (1070)
- Al-Istidrak ala Batlamyus (Recapitulation regarding Ptolemy) (11th century)
- Al-Khazini (fl. 1115-1130)
- Az-Zij As-Sanjarī (Sinjaric Tables) (1115-1116)
- Risala fi'l-alat (Treatise on Instruments)
- Nasīr al-Dīn al-Tūsī (1201-1274)
- Al-Tadhkirah fi'ilm al-hay'ah (Momento in astronomy)
- Zij-i Ilkhani (Ilkhanic Tables) (1272)
- 'Umar al-Katibi al-Qazwini (d. 1277)
- Hikmat al-'Ain
- Qutb al-Din al-Shirazi (1236-1311)
- The Limit of Accomplishment concerning Knowledge of the Heavens
- Ibn al-Shatir (1304–1375)
- A Final Inquiry Concerning the Rectification of Planetary Theory
- Ulugh Beg (1394-1449)
- Zij-i-Sultani (1437)
- Shams al-Din al-Khafri (d. 1525)
- The complement to the explanation of the momento
See also
- Islamic science
- Islamic Golden Age
- Inventions in the Muslim world
- List of Muslim astronomers
- List of Muslim scientists
- List of Iranian scientists
- Islamic astrology
- Arab and Persian astrology
- History of astronomy
- Hebrew astronomy
- Zij
Notes
- ^ a b Dallal (1999), p. 162
- ^ This book is not related to al-Khwarizmi's Zij al-Sindh. On zijes see E. S. Kennedy, "A Survey of Islamic Astronomical Tables".
- ^ A. Abd-Allah, The Qur'an, Knowledge, and Science, University of Southern California.
- ^ a b c Reseach Committee of Strasburg University, Imam Jafar Ibn Muhammad As-Sadiq A.S. The Great Muslim Scientist and Philosopher, translated by Kaukab Ali Mirza, 2000. Willowdale Ont. ISBN 0969949014.
- ^ Dallal (1999), pg. 163
- ^ Dallal (1999), pg. 164
- ^ Bartel Leendert van der Waerden (1987). "The Heliocentric System in Greek, Persian and Hindu Astronomy", Annals of the New York Academy of Sciences 500 (1), 525–545 [534-537].
- ^ George Saliba (1994). "Early Arabic Critique of Ptolemaic Cosmology: A Ninth-Century Text on the Motion of the Celestial Spheres", Journal for the History of Astronomy 25, p. 115-141 [116].
- ^ K. A. Waheed (1978). Islam and The Origins of Modern Science, p. 27. Islamic Publication Ltd., Lahore.
- ^ Robert Briffault (1938). The Making of Humanity, p. 191.
- ^ Dr. A. Zahoor (1997), Abu Raihan Muhammad al-Biruni, Hasanuddin University.
- ^ A. I. Sabra (1998), pp. 293-8
- ^ Dennis Duke, Arabic Models for outer Planets and Venus
- ^ A. I. Sabra (1998), pp. 317-18
- ^ Teresi, et al., (2002)
- ^ a b c d e Khwarizm, Foundation for Science Technology and Civilisation.
- ^ Rafik Berjak and Muzaffar Iqbal, "Ibn Sina--Al-Biruni correspondence", Islam & Science, June 2003.
- ^ a b Salah Zaimeche PhD (2005). Merv, p. 7. Foundation for Science Technology and Civilization.
- ^ Nicolaus Copernicus. Stanford Encyclopedia of Philosophy (2004).
- ^ Y. Tzvi Langermann, ed. and trans., Ibn al-Haytham's On the Configuration of the World, Harvard Dissertations in the History of Science, (New York: Garland, 1990), pp. 25-34
- ^ Duhem, Pierre (1908, 1969). To Save the Phenomena: An Essay on the Idea of Physical theory from Plato to Galileo, p. 28. University of Chicago Press, Chicago.
- ^ O. S. Marshall (1950). "Alhazen and the Telescope", Astronomical Society of the Pacific Leaflets 6, p. 4.
- ^ Rashed (2007).
- ^ Rashed (2007), p. 20, 53.
- ^ Rashed (2007), p. 33-34.
- ^ Rashed (2007), p. 20, 32-33.
- ^ Rashed (2007), p. 51-52.
- ^ Rashed (2007), p. 35-36.
- ^ S. H. Nasr, Islamic Cosmological Doctrines, p. 135, n. 13
- ^ a b A. Baker, L. Chapter (2002)
- ^ Michael E. Marmura (1965). "An Introduction to Islamic Cosmological Doctrines. Conceptions of Nature and Methods Used for Its Study by the Ikhwan Al-Safa'an, Al-Biruni, and Ibn Sina by Seyyed Hossein Nasr", Speculum 40 (4), p. 744-746.
- ^ a b Saliba (1999).
- ^ G. Wiet, V. Elisseeff, P. Wolff, J. Naudu (1975). History of Mankind, Vol 3: The Great medieval Civilisations, p. 649. George Allen & Unwin Ltd, UNESCO.
- ^ a b c Richard Covington (2007).
- ^ S. H. Nasr, Islamic Cosmological Doctrines, p. 134
- ^ George Saliba (1980), "Al-Biruni", in Joseph Strayer, Dictionary of the Middle Ages, Vol. 2, p. 249. Charles Scribner's Sons, New York.
- ^ A. I. Sabra (1998). "Configuring the Universe: Aporetic, Problem Solving, and Kinematic Modeling as Themes of Arabic Astronomy", Perspectives on Science 6 (3), p. 288-330 [305-306].
- ^ George Saliba (1981). "Geschichte des arabischen Schriftiums. Band VI: Astronomie bis ca. 430 H by F. Sezgin", Journal of the American Oriental Society 101 (2), p. 219-221 [219].
- ^ Robert Briffault (1938). The Making of Humanity, p. 190.
- ^ Owen Gingerich (April 1986). "Islamic astronomy", Scientific American 254 (10), p. 74.
- ^ Bernard R. Goldstein (March 1972). "Theory and Observation in Medieval Astronomy", Isis 63 (1), p. 39-47 [40-41].
- ^ Bernard R. Goldstein (March 1972). "Theory and Observation in Medieval Astronomy", Isis 63 (1), p. 39-47 [41].
- ^ Ptolemaic Astronomy, Islamic Planetary Theory, and Copernicus's Debt to the Maragha School, Science and Its Times, Thomson Gale.
- ^ Dallal (1999), pg. 171
- ^ George Saliba (1979). "The First Non-Ptolemaic Astronomy at the Maraghah School", Isis 70 (4), p. 571-576.
- ^ a b M. Gill (2005). Was Muslim Astronomy the Harbinger of Copernicanism?
- ^ Y. M. Faruqi (2006). "Contributions of Islamic scholars to the scientific enterprise", International Education Journal 7 (4), p. 395-396.
- ^ Saliba (2000).
- ^ Islam, Knowledge, and Science. University of Southern California.
- ^ Robert Hannah (1997). "The Mapping of the Heavens by Peter Whitfield", Imago Mundi 49, p. 161-162.
- ^ Linear astrolabe, Encyclopædia Britannica.
- ^ Donald Routledge Hill (1985). "Al-Biruni's mechanical calendar", Annals of Science 42, p. 139-163.
- ^ Tuncer Oren (2001). "Advances in Computer and Information Sciences: From Abacus to Holonic Agents", Turk J Elec Engin 9 (1), p. 63-70 [64].
- ^ Silvio A. Bedini, Francis R. Maddison (1966). "Mechanical Universe: The Astrarium of Giovanni de' Dondi", Transactions of the American Philosophical Society 56 (5), p. 1-69.
- ^ Astrolabe gearing, Museum of the History of Science, Oxford.
- ^ History of the Astrolabe, Museum of the History of Science, Oxford.
- ^ Emilie Savage-Smith (1993). "Book Reviews", Journal of Islamic Studies 4 (2), p. 296-299.
"There is no evidence for the Hellenistic origin of the spherical astrolabe, but rather evidence so far available suggests that it may have been an early but distinctly Islamic development with no Greek antecedents."
- ^ David A. King, "Islamic Astronomy," pp. 168-9.
- ^ David A. King, Studies in Astronomical Timekeeping in Medieval Islamic Civilization, E. J. Brill.
- ^ David A. King (December 2003). "14th-Century England or 9th-Century Baghdad? New Insights on the Elusive Astronomical Instrument Called Navicula de Venetiis", Centaurus 45 (1-4), p. 204-226.
- ^ a b c David A. King, "Reflections on some new studies on applied science in Islamic societies (8th-19th centuries)", Islam & Science, June 2004.
- ^ a b c d David A. King (2002). "A Vetustissimus Arabic Text on the Quadrans Vetus", Journal for the History of Astronomy 33, p. 237-255 [237-238].
- ^ David A. King, "Islamic Astronomy", in Christopher Walker (1999), ed., Astronomy before the telescope, p. 167-168. British Museum Press. ISBN 0-7141-2733-7.
- ^ O'Connor, John J.; Robertson, Edmund F., "Al-Khujandi", MacTutor History of Mathematics Archive, University of St Andrews
- ^ Ajram (1992).
- ^ Muzaffar Iqbal, "David A. King, World-Maps for Finding the Direction and Distance to Mecca: Innovation and Tradition in Islamic Science", Islam & Science, June 2003.
- ^ David A. King (1997). "Two Iranian World Maps for Finding the Direction and Distance to Mecca", Imago Mundi 49, p. 62-82 [62].
- ^ Shadow square, National Maritime Museum.
References
- Abdulhak Adnan, La science chez les Turcs ottomans, Paris, 1939.
- K. Ajram (1992). Miracle of Islamic Science, Appendix B. Knowledge House Publishers. ISBN 0911119434.
- A. Baker and L. Chapter (2002), "Part 4: The Sciences". In M. M. Sharif, "A History of Muslim Philosophy", Philosophia Islamica.
- Richard Covington (May-June 2007). "Rediscovering Arabic science", Saudi Aramco World, p. 2-16.
- Ahmad Dallal, "Science, Medicine and Technology.", in The Oxford History of Islam, ed. John Esposito, New York: Oxford University Press, (1999).
- Antoine Gautier, L'âge d'or de l'astronomie ottomane, in L'Astronomie, (Monthly magazine created by Camille Flammarion in 1882), December 2005, volume 119.
- M. Gill (2005). Was Muslim Astronomy the Harbinger of Copernicanism?
- Donald R. Hill, Islamic Science And Engineering, Edinburgh University Press (1993), ISBN 0-7486-0455-3
- E. S. Kennedy, "A Survey of Islamic Astronomical Tables," Transactions of the American Philosophical Society, 46, 2 (1956).
- Edward S. Kennedy (1998), Astronomy and Astrology in the Medieval Islamic World. Brookfield, VT: Ashgate.
- David A. King (1986). Islamic mathematical astronomy. London.
- David A. King, "Islamic Astronomy", in Astronomy before the telescope, ed. Christopher Walker. British Museum Press, (1999), pp. 143-174. ISBN 0-7141-2733-7
- Seyyed H. Nasr, (1964) An Introduction to Islamic Cosmological Doctrines,, Cambridge: Belknap Press of the Harvard University Press.
- Roshdi Rashed (2007). "The Celestial Kinematics of Ibn al-Haytham", Arabic Sciences and Philosophy 17, p. 7-55. Cambridge University Press.
- A. I. Sabra (1998). "Configuring the Universe: Aporetic, Problem Solving, and Kinematic Modeling as Themes of Arabic Astronomy," Perspectives on Science 6, p. 288-330.
- George Saliba (1999). Whose Science is Arabic Science in Renaissance Europe? Columbia University.
- George Saliba (2000). "Arabic versus Greek Astronomy: A Debate over the Foundations of Science", Perspectives on Science 8, p. 328-341.
- H. Suter (1902). Mathematiker und Astronomen der Araber.
- Dick Teresi, Jamil Ragep, and Roger Hart (2002). "Ancient Roots of Modern Science", Talk of the Nation (NPR discussion of intercultural scientific contacts; astronomy is discussed in the first fifteen-minute segment).
External links
- "Tubitak Turkish National Observatory Antalya"
- "Scientific American" article on Islamic Astronomy
- The Arab Union for Astronomy and Space Sciences (AUASS)
- King Abdul Aziz Observatory
- History of Islamic Astrolabes
- Arabic models for replacing the equant for the outer planets and Venus
- Ibn ash-Shatir model for Mercury
- Ibn ash-Shatir model for the Moon
- Arabian astronomy