Fourth-generation fighter: Difference between revisions
m →Exercise reports: Self Correction |
Removed unsourced text. |
||
Line 202: | Line 202: | ||
The primary sensor for all modern fighters is [[radar]]. The U.S. pioneered the use of solid-state [[AESA]] radars{{Fact|date=February 2007}}, which have no moving parts and are capable of projecting a much tighter beam and quicker scans. It is fitted to [[F-15 Eagle|F-15C]], the [[F/A-18E/F Super Hornet]], and the block 60 (export) F-16, and will be used for all future American fighters. A European coalition [[GTDAR]] is developing an AESA radar for use on the Typhoon and Rafale, Russia has an AESA radar on MIG-35 and the newest SU-27 versions. For the next generation F-22 and F-35, the U.S. will utilize [[Low Probability of Intercept]] (LPI) capacity. This will spread the energy of a radar pulse over several frequencies, so as not to trip the [[radar warning receivers]] that all aircraft carry. |
The primary sensor for all modern fighters is [[radar]]. The U.S. pioneered the use of solid-state [[AESA]] radars{{Fact|date=February 2007}}, which have no moving parts and are capable of projecting a much tighter beam and quicker scans. It is fitted to [[F-15 Eagle|F-15C]], the [[F/A-18E/F Super Hornet]], and the block 60 (export) F-16, and will be used for all future American fighters. A European coalition [[GTDAR]] is developing an AESA radar for use on the Typhoon and Rafale, Russia has an AESA radar on MIG-35 and the newest SU-27 versions. For the next generation F-22 and F-35, the U.S. will utilize [[Low Probability of Intercept]] (LPI) capacity. This will spread the energy of a radar pulse over several frequencies, so as not to trip the [[radar warning receivers]] that all aircraft carry. |
||
In reaction to the increasing American emphasis on radar-evading stealth designs, the Soviet Union turned to alternate sensors. This drove them to emphasize [[infra-red search and track]] (IRST) sensors, first introduced on the American [[F-101 Voodoo]] and [[F-102 Delta Dagger]] fighters in the 1960s, for detection and tracking of airborne targets. These are essentially a TV camera in the IR wavelength, passively measuring the emitted IR radiation from targets. However, as a passive sensor it has limited range, and contains no inherent data about position and direction of targets - these must be inferred from the images captured |
In reaction to the increasing American emphasis on radar-evading stealth designs, the Soviet Union turned to alternate sensors. This drove them to emphasize [[infra-red search and track]] (IRST) sensors, first introduced on the American [[F-101 Voodoo]] and [[F-102 Delta Dagger]] fighters in the 1960s, for detection and tracking of airborne targets. These are essentially a TV camera in the IR wavelength, passively measuring the emitted IR radiation from targets. However, as a passive sensor it has limited range, and contains no inherent data about position and direction of targets - these must be inferred from the images captured. With the exception of the [[F-14 Tomcat|F-14D]] (officially retired as of September 2006), no 4th generation Western fighters carry built-in IRST sensors for air-to-air detection, though the similar [[FLIR]] is often used to acquire ground targets. The next-generation [[Eurofighter|Eurofighter Typhoon]] (beginning with Tranche 1 Block 5 aircraft,<ref>http://www.publicservice.co.uk/pdf/dmj/issue31/DMJ31%200012%20A%20Brookes%20ATL.pdf</ref> while previously build aircraft are being retrofited since spring 2007 <ref>{{cite news | title = Type Acceptance for Block 5 Standard Eurofighter Typhoon | url =http://www.eurofighter.com/news/article263.asp | work = www.eurofighter.com | publisher = Eurofighter GmbH | date = 2007-02-15 | accessdate = 2007-06-20}}</ref>), [[F-22 Raptor|F-22]] and [[F-35 Joint Strike Fighter|F-35]] will all have built-in IRST sensors. Begining in 2012 the Super hornet will also have an IRST.<ref>http://www.flightglobal.com/articles/2007/03/13/212600/ultra-hornet.html</ref> |
||
The tactical implications of the computing and data bus capabilities of aircraft are hard to determine. A more sophisticated computer bus would allow more flexible uses of the existing avionics. For example, it is speculated that the F-22 is able to jam or damage enemy electronics with a focused application of its radar. A computing feature of significant tactical importance is the datalink. All of the modern European and American aircraft are capable of sharing targeting data with allied fighters and from AWACS planes (see [[JTIDS]]). The Russian [[MiG-31]] interceptor also has some datalink capability, so it is reasonable to assume that other Russian planes can also do so. The sharing of targeting and sensor data allows pilots to put radiating, highly visible sensors further from enemy forces, while using that data to vector silent fighters toward the enemy. |
The tactical implications of the computing and data bus capabilities of aircraft are hard to determine. A more sophisticated computer bus would allow more flexible uses of the existing avionics. For example, it is speculated that the F-22 is able to jam or damage enemy electronics with a focused application of its radar. A computing feature of significant tactical importance is the datalink. All of the modern European and American aircraft are capable of sharing targeting data with allied fighters and from AWACS planes (see [[JTIDS]]). The Russian [[MiG-31]] interceptor also has some datalink capability, so it is reasonable to assume that other Russian planes can also do so. The sharing of targeting and sensor data allows pilots to put radiating, highly visible sensors further from enemy forces, while using that data to vector silent fighters toward the enemy. |
Revision as of 02:24, 30 September 2007
![]() |
![]() | This article possibly contains original research. (September 2007) |

Aircraft classified as fourth generation jet fighters are those in service approximately from 1980–2010, representing the design concepts of the 1970s. Fourth generation designs are heavily influenced by lessons learned from the previous generation of combat aircraft. Representative fighters include the "teen" series of American fighters (F-14, F-15, F-16, and F/A-18) and the Soviet MiG-29 and Su-27. The growing costs and the demonstrated success of multi-role aircraft such as the F-4 Phantom II gave rise to the popularity of multi-role fighters. Long-range air-to-air missiles, originally thought to make dogfighting obsolete, proved less influential than expected; designers responded with a renewed emphasis on maneuverability.
The rapid advance of microcomputers in the 1980s and 90s permitted rapid upgrades to the avionics over the lifetimes of these fighters, incorporating system upgrades such as AESA, digital avionics buses, and IRST. Because of the drastic enhancement of capabilities in these upgraded fighters and in new designs of the 1990s that reflected these new capabilities, the designation 4.5th generation is sometimes used to refer to these later designs. It is intended to reflect a class of fighters that are evolutionary upgrades of the 4th generation to incorporate integrated avionics suite, advanced weapons, and elements of stealth technology (but not true stealth) [1][2].
A prime example of this generation is the F/A-18E/F Super Hornet, a growth version of the 1970s Hornet design. While the basic aerodynamic features are largely the same, the Super Hornet features improved avionics in the form of an all-glass cockpit, a solid-state AESA active phased array radar, new engines, the structural use of composite materials to reduce weight, a slightly modified shape to minimize its radar signature, and IRST.
4th generation aircraft
Aircraft that entered service
France: Dassault Mirage 2000 (NATO)
Russia
People's Republic of China:
Republic of China: AIDC F-CK Indigenous Defence Fighter
United Kingdom /
United States: McDonnell Douglas/BAe Harrier II (NATO)
United States: (NATO)
European Union (only
United Kingdom/
Germany/
Italy): Panavia Tornado (NATO)
Cancelled aircraft
Argentina: FMA SAIA 90
People's Republic of China: Chengdu J-9
Israel: IAI Lavi
France: Dassault Mirage 4000
Poland: PZL-230 Skorpion
Romania: IAR 95
Switzerland: ALR Piranha
Yugoslavia: Novi Avion
United States: (NATO)
4.5th generation
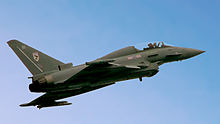
Aircraft that entered service
Russia
- Mikoyan MiG-35
- Sukhoi Su-30/33/35/37(Su-27 Derivatives) [3]
Sweden
Cancelled Aircraft
United States
- F-16XL (cancelled)
Under Development
5th generation aircraft
In Service
Scheduled To Enter Active Service
Test flight by 2009 and enter active service by 2012
Technology Demonstrators
* Built, flown and tested (one unit only) - but design not selected for active service
In Development
In very early development or rumored projects
People's Republic of China
- Shenyang J-XX (under development)
India
- Medium Combat Aircraft (under development)
South Korea
- KFX (under development)
Japan
- Mitsubishi ATD-X (under development)
The American F-22 Raptor, put into service in 2004, is often regarded [citation needed] as the first of a new generation of fighters, termed the fifth generation. One of the key design differences between the F-22 and preceding designs is an emphasis on stealth. The in-development F-35 Lightning II (formerly Joint Strike Fighter) has also been designed for stealth, and the F-22 has influenced [citation needed] the continued development of the 4th generation designs, and the shape of design work for other countries' long-term fighter development projects (for instance, the Russian PAK FA).
Design considerations
Performance
Performance has traditionally been one of the most important design characteristics, as it enables a fighter to gain a favourable position to use its weapons while rendering the enemy unable to use theirs. This can occur at long range (beyond visual range or BVR) or short range (within visual range or WVR). At short range, the ideal position is to the rear of the enemy aircraft, where it is unable to aim or fire weapons and the hot exhaust makes a good target for IR-guided missiles. At long range, while optimal positions are not so clear, it is nonetheless an advantage to intercept enemy aircraft before they reach their targets.
These two scenarios have competing demands — interception requires excellent linear speed, while WVR engagements require excellent turn rate and acceleration. Prior to the 1970s, a popular view in the defence community was that missiles would render WVR combat obsolete and hence maneuverability useless. Combat experience proved this untrue due to the poor quality of missiles and the recurring need to identify targets visually. Though improvements in missile technology may make that vision a reality, experience has indicated that sensors are not foolproof and that fighters will still need to be able to fight and maneuver at close ranges. So whereas the premier third-generation jet fighters (e.g., the F-4 and MiG-23) were designed as interceptors with a secondary emphasis on maneuverability, interceptors have been relegated to a secondary role in the fourth generation, with a renewed emphasis on maneuverability.
There are two primary contributing factors to maneuverability — the amount of power delivered by the engines, and the ability of the aircraft's control surfaces to translate that power into changes in direction. Air combat maneuvering (ACM) involves a great deal of energy management, where energy is roughly the sum of altitude and airspeed. The greater energy a fighter has, the more flexibility it has to move where it wants. An aircraft with little energy is immobile, and a defenceless target. Note that power does not necessarily equal speed; while more power gives greater acceleration, the maximum speed of an aircraft is determined by how much drag it produces. Herein lies the trade-off. Low-drag configurations have small, stubby, highly swept wings that disrupt the airflow as little as possible. However, that also means they have greatly reduced ability to alter the airflow to maneuver the aircraft.
There are two rough indicators of these factors. A plane's turning ability can be roughly measured by its wing loading, defined as the mass of the aircraft divided by the area of its lifting surfaces. A highly loaded wing has little capacity to produce additional lift, and so has limited turning ability, whereas a lightly loaded wing has much greater potential lifting power. A rough measure of acceleration is a plane's thrust-to-weight ratio.
Thrust/ Weight Ratio | Wing loading (kg/m²) | Notes | |
---|---|---|---|
Rafale F2 | 1.13 | 326 | 5300 l fuel internal |
Typhoon | 1.18 | 311 | 4700 l fuel internal |
F-2 | 0.89 | 430 | |
F-15C | 1.30 | 358 | For models equipped with PW-F100-229, 1.12 otherwise |
MiG-29SM | 1.13 | 411 | |
Su-27 | 1.09 | 371 | |
Su-30MKI | 0.77 | 414 | Has thrust vectoring (tridimensional) |
J-10 | 0.89 | 427 | Proposed upgrade will have thrust vectoring |
Gripen | 0.94 | 341 | |
HAL Tejas | 1.07 | 221.4 | 95% composites by surface area. Naval version will LEVCONs. |
F-22A | 1.26 | 314 | 13000 l fuel internal and thrust vectoring (bidimensional) |
F-35A | 1.22 | 309 | 6811 l fuel internal (50%) |
Notes:
- values are at normal take-off weight unless otherwise specified
- some of the take-off weights and thrust values are not officially available
Thrust vectoring
Thrust vectoring is a new technology being introduced to further enhance a fighter's turning ability. By redirecting the jet exhaust, it is possible to directly translate the engine's power into directional changes, more efficiently than via the plane's control surfaces. The technology has been fitted to the Mikoyan MiG-35, Sukhoi Su-30MKI and later derivatives, and F-22 Raptor. The U.S. explored fitting the technology to the F-16 and the F-15, but only introduced it on the F-22 Raptor. There is some indication that the J-10 and Typhoon may eventually be refitted with thrust vectoring.
Supercruise
Supercruise is the ability of aircraft to cruise efficiently at supersonic speeds without the afterburner. Because of parasitic drag effects, fighters carrying external weapons stores encounter excessive drag near the speed of sound, making it impossible or prohibitively fuel-consuming to break the sound barrier. Though fighters easily break Mach 1 and 2 in clean configurations on afterburner, and the English Electric Lightning was able to break Mach 1 without the use of afterburner, these were academic exercises as they were not carrying combat loads.
With improvements to engine power output and careful aeronautical design of weapons stores, it is now possible for fighters to supercruise with combat loads. The Typhoon and the F-22 are the only modern fighters with this ability. Dassault claims the Rafale to be able to supercruise but this claim has yet to be demonstrated. The F-22 can supercruise at Mach 1.5+. The Typhoon is believed to have supercruise ability just above Mach 1.
Avionics
Avionics is a catch-all phrase for the electronic systems aboard an aircraft, which have been growing in complexity and importance. The main elements of an aircraft's avionics are its sensors (Radar and IR sensors), computers and data bus, and user interface. Because they can be readily swapped out as new technologies become available, they are often upgraded over the lifetime of an aircraft. Details about these systems are highly protected. Since many export aircraft have downgraded avionics, many buyers substitute domestically developed avionics, (sometimes considered superior to the original). For example, the Sukhoi Su-30MKI sold to India, the F-15I and F-16I sold to Israel, and the F-15K sold to South Korea.
The primary sensor for all modern fighters is radar. The U.S. pioneered the use of solid-state AESA radars[citation needed], which have no moving parts and are capable of projecting a much tighter beam and quicker scans. It is fitted to F-15C, the F/A-18E/F Super Hornet, and the block 60 (export) F-16, and will be used for all future American fighters. A European coalition GTDAR is developing an AESA radar for use on the Typhoon and Rafale, Russia has an AESA radar on MIG-35 and the newest SU-27 versions. For the next generation F-22 and F-35, the U.S. will utilize Low Probability of Intercept (LPI) capacity. This will spread the energy of a radar pulse over several frequencies, so as not to trip the radar warning receivers that all aircraft carry.
In reaction to the increasing American emphasis on radar-evading stealth designs, the Soviet Union turned to alternate sensors. This drove them to emphasize infra-red search and track (IRST) sensors, first introduced on the American F-101 Voodoo and F-102 Delta Dagger fighters in the 1960s, for detection and tracking of airborne targets. These are essentially a TV camera in the IR wavelength, passively measuring the emitted IR radiation from targets. However, as a passive sensor it has limited range, and contains no inherent data about position and direction of targets - these must be inferred from the images captured. With the exception of the F-14D (officially retired as of September 2006), no 4th generation Western fighters carry built-in IRST sensors for air-to-air detection, though the similar FLIR is often used to acquire ground targets. The next-generation Eurofighter Typhoon (beginning with Tranche 1 Block 5 aircraft,[6] while previously build aircraft are being retrofited since spring 2007 [7]), F-22 and F-35 will all have built-in IRST sensors. Begining in 2012 the Super hornet will also have an IRST.[8]
The tactical implications of the computing and data bus capabilities of aircraft are hard to determine. A more sophisticated computer bus would allow more flexible uses of the existing avionics. For example, it is speculated that the F-22 is able to jam or damage enemy electronics with a focused application of its radar. A computing feature of significant tactical importance is the datalink. All of the modern European and American aircraft are capable of sharing targeting data with allied fighters and from AWACS planes (see JTIDS). The Russian MiG-31 interceptor also has some datalink capability, so it is reasonable to assume that other Russian planes can also do so. The sharing of targeting and sensor data allows pilots to put radiating, highly visible sensors further from enemy forces, while using that data to vector silent fighters toward the enemy.
Cost
The per unit cost is difficult to accurately determine, as the amortization of a large development cost over a varying number of units produced can greatly vary the price. Moreover, the purchase price does not reflect lifetime costs of maintenance, parts, and training. A useful guide to costs come from export prices, which are widely reported, and represent a mix of the marginal cost of production plus some recouperation of development costs.
- Dassault Rafale More than €50m, depending on export sales
- Eurofighter Typhoon : '07 €62m us$121m
- Mitsubishi F-2 US$ 100m
- MiG-29 about '98 US$ 27m
- MiG-35 '07 US$ 70m
- Sukhoi Su-27US$ 24m
- Sukhoi Su-30 US$ ~38m (Several variants)
- Sukhoi Su-30K for Indonesia: '98 US$ 33m
- Sukhoi Su-30MKK/MK2 for China: '98 US$ 38m
- Sukhoi Su-30MKI for India: '98 US$ 45m
- Sukhoi Su-30MKM for Malaysia: '03 US$ 50m
- HAL Tejas about US$23m
- JF-17 Thunder about US$ 20m (estimated)
- JAS 39 Gripen about '98 US$ 25m
- Ching Kuo IDF (Taiwan) initially large order put cost per unit at US$ 24m
- F-14 Tomcat '98 US$ 48m
- F-15 Eagle '98 US$ 43m
- F-16 Fighting Falcon late models about '98 US$ 25m
- F/A-18E/F Super Hornet '98 US$ 60m
- F-22 Raptor Fly away unit cost is about US$ 120m
- F-35 Lightning II:
- F-35A US$ 45m
- F-35B > US$ 100m '06
- F-35C US$ 55m
Maintenance demands
As militaries make growing use of Operations Research and other management techniques from the corporate world to examine the effectiveness of hardware, a growing emphasis is being placed on maintenance and reliability. A prime example of this is the F/A-18 Hornet. While unimpressive on paper compared to its contemporaries, it incorporated a low-maintenance design. As a result, its mean time between failure is three times greater than any other Navy strike aircraft, and requires half the maintenance time. These greatly elevated its sortie rate and made it more effective than aircraft that were superior in a 1 on 1 comparison but which were more often than not unavailable to fight.
Also the Gripen has an incredibly low maintenance cost,[citation needed] partly because 5-10 'maintenance men' can re-arm it in 10 minutes, and it can use virtually anything flat and 400 meters long as a runway. Some have speculated that a Gripen squadron could be sent on a long range mission, and while it is gone a runway could be built where there was wilderness before, which, conceptually, could almost double its range.
Stealth technology
Stealth technology is an extension of the notion of visual camouflage to modern radar and IR detection sensors. While not rendering an aircraft "invisible" as is popularly conceived, stealth makes an aircraft much more difficult to discern from the sky, clouds, or distant aircraft, conferring a significant tactical advantage. While the basic principles of shaping aircraft to avoid detection were known at least since the 1960's, it was not until the availability of supercomputers that shape computations could be performed from every angle, a complex task. The use of computer-aided shaping, combined with radar-absorbent materials, produced aircraft of drastically reduced radar cross section (RCS) and were much more difficult to detect on radar.
During the 1970's, the rudimentary level of stealth shaping (as seen in the faceted design of the F-117 Nighthawk) resulted in too severe a performance penalty to be used on fighters. Faster computers enabled smoother designs such as the B-2 Spirit, and thought was given to applying the basic ideas to decrease, if not drastically reduce, the RCS of fighter aircraft. These techniques are also combined with methods of decreasing the IR, visual, and aural signature of the aircraft.
Recent American fighter aircraft development has focused on stealth, and the recently deployed F-22 is the first fighter designed from the ground up with a consideration for stealth. However, the stealthiness of the F-22 from angles other than head-on is not clear. The F-35 incorporates the same degree of stealth shaping, although its exposed rear turbine blades render it significantly less stealthy from the rear (the thrust vectoring nozzles of the F-22 also serve to conceal the turbine blades).[citation needed] Several late 4.5th generation fighters have been given stealth shaping and other refinements to reduce their RCS, including the Super Hornet, Typhoon, and Rafale.

There are some reports that the Rafale’s avionics, the Thales Spectra, includes "stealthy" radar jamming technology, a radar cancellation systems analogous to the acoustic noise suppression systems on the De Havilland Canada Dash 8. Conventional jammers make locating an aircraft more difficult, but their operation is itself detectable; the French system is hypothesised to interfere with detection without revealing that jamming is in operation. In effect, such a system could potentially offer stealth advantages similar in effect to, but likely less effective than, the F-22 and F-35. However, it is unclear how effective the system is, or even whether it is fully operational yet.
Research continues into other ways of decreasing observability by radar. There are claims that the Russians are working on "plasma stealth".[9] Obviously, such techniques might well remove some of the current advantage of the F-22 and F-35, but American defence research also continues unabated.
There are ways to detect fighters other than radar. For instance, passive infra-red sensors can detect the heat of engines, and even the sound of a sonic boom (which any supersonic aircraft will make) can be tracked with a network of sensors and computers. However, using these to provide precise targeting information for a long-range missile is considerably less straightforward than radar.
Combat performance
- 1991 Gulf War
- On January 17, 1991, the first night of the Gulf War, an Iraqi MiG-25PD shot down a U.S. Navy F/A-18C (piloted by Lt Cdr Scott Speicher), which was lost 29 nautical miles southeast of Baghdad.[10][11]
- During the 1991 Gulf War, USAF F-15 pilots shot down five Iraqi MiG-29s.[12]
- On January 17, 1993, a USAF F-16 pilot shot down a MiG-29 in the Iraqi no-fly zone. Some sources claim it was a MiG-23.[12][13][14]
- 1999 Kosovo War,
- a Dutch F-16 pilot shot down a Yugoslavian MiG-29 and a USAF F-16 pilot also shot down a MiG-29.[15][16]
- USAF F-15 pilots shot down four MiG-29s.[15]
- Eritrean-Ethiopian War. In February 1999, according to some reports, Ethiopian Su-27 pilots shot down four Eritrean MiG-29s. Some of these sources claim that the Ethiopian planes were flown by Russian pilots, and the Eritrean planes by Ukrainians. (It is certainly true that local pilots were trained by instructors from those nations.[17]
Comparative analysis
It's misleading to extrapolate comparisons regarding these fighters from the combat history, as fighters function in a combined arms environment in which many other factors, including C4I (command, control, communications, computers, and intelligence) assets and pilot training determine success. For example, the undefeated records of the F-15 and F-16 should not be taken as unambiguous indicators of their superiority as airframes, as their combat action involved action by American and Israeli pilots with superior training and C4I assets against poorly trained adversaries with much poorer C4I assets.
However, for purchasing considerations, nations often consider comparative analyses of fighters to fill their specific mission requirements. Additionally, joint exercises are often revealing about the performance of fighters in a system, even as their validity is compromised by the inherent assumptions about the systems on either side.
Controversial DERA study
Britain’s Defence Evaluation and Research Agency (now split into QinetiQ and DSTL) did an evaluation in 1994 (simulation based on the available data) comparing the Typhoon with some other modern fighters in how well they performed against an expected adversary aircraft, the Sukhoi Su-35. Due to the lack of information gathered on the 5th generation combat aircraft, the inability to take into account advances in avionics and weapons now fitted to the fighters tested, and lack of knowledge on the capabilities of the Su-35 during the time of this study it is not meant to be considered official and it's results should be taken with a grain of salt.
The study used real pilots flying the JOUST system of networked simulators. Various western aircraft supposed data were put in simulated combat against the Su-35. The results were:
Aircraft | Odds vs. Su-35 |
---|---|
Lockheed Martin/Boeing F-22 Raptor | 10.1:1 |
Eurofighter Typhoon | 4.5:1 |
Sukhoi Su-35 'Flanker' | 1.0:1 |
Dassault Rafale C | 1.0:1 |
McDonnell Douglas F-15C Eagle | 0.8:1 |
Boeing F/A-18+ | 0.4:1 |
McDonnell Douglas F/A-18C | 0.3:1 |
General Dynamics F-16C | 0.3:1 |
These results mean, for example, that in simulated combat, 4.5 Su-35s were shot down for every Typhoon lost. Missiles such as the KS-172 may be intended for large targets and not fighters, but their impact on a long range BVR engagement needs to be factored in.
The "F/A-18+" in the study was apparently not the current F/A-18E/F, but an improved version. All the western aircraft in the simulation were using an older version of the AMRAAM missile, except the Rafale which was using the MICA missile. This does not reflect the likely long-term air-to-air armament of Eurofighters (as well as Rafales), which will ultimately be equipped with the longer-range MBDA Meteor (while carrying the AMRAAM as an interim measure).
Details of the simulation have not been released, making it harder to verify whether it gives an accurate evaluation (for instance, whether they had adequate knowledge of the Sukhoi and Raptor to realistically simulate their combat performance). Another problem with the study is the scenarios under which the combat took place are unclear; it is possible that they were deliberately or accidentally skewed to combat scenarios that favoured certain aircraft over others; For instance, long-range engagements favour planes with stealth, good radar and advanced missiles, whereas the Su-35’s alleged above-average maneuverability may prove advantageous in short-range combat. Nor is it clear whether the Su-35 was modeled with thrust vector control (as the present MKIs, MKMs have).
Additionally, the DERA simulation was made in the mid 90s with limited knowledge about the Radar Cross Section, the ECM and the radar performances of the actual aircraft: indeed, at that time, the 4th/5th generation fighters were all at the prototype stage.
Exercise reports
Friendly air forces regularly practice against each other in exercises, and when these air forces fly different aircraft some indication of the relative capabilities of the aircraft can be gained.
The results of an exercise in 2004 pitting USAF F-15 Eagles against Indian Air Force Su-30MKs, Mirage 2000s, MiG-29s and even the elderly MiG-21 have been widely publicized, with the Indians winning "90% of the mock combat missions"[18]. reports[19]. claims that the Indian Su-30MK obtained its high kill ratio through tactics that exploited the inherent maneuverability of the Flanker design to evade a lock by the Doppler radar used by the F-15 through maneuvers that placed the Su-30 at a low relative forward speed. Once within range the soviet designed Vympel R-73 was used to score the kill. The older second generation Mig-21 and third generation Mig-23 aircraft were able to score favorable kill ratios against the F-15s through superior numbers, and through the use of avionics “BISON” upgrades that gave the Migs fourth generation datalink capability. [20] The Indian aircraft used a combination of the Russian R-77, R-73 and R-27AE missiles all of who possess fire-and-forget BVR capability.
In June 2005, a Royal Air Force Eurofighter pilot was reportedly able, in a mock confrontation, to avoid two pursuing F-15E fighter-bombers and outmanoeuvre them to get into a shooting position.[21]
During Exercise Northern Edge 2006 in Alaska in early June, the F-22 reportedly proved its mettle against as many as 40 "enemy aircraft" during simulated battles. The Raptor is claimed to have achieved a 108-to-zero kill ratio at that exercise[22].
Notes
- ^ "F-22 Tops Japan's Military Wish List".
{{cite web}}
: Text "publisher - Aviation Week and Space Technology" ignored (help) - ^ a b "The Gray Threat".
{{cite web}}
: Text "Air Force Magazine" ignored (help) - ^ a b c d e Devin L. Cate. "The Air Superiority Fighter and Defense Transformation" (PDF). Air University Press.
- ^ http://www.airpower.at/forum/viewtopic.php?t=2629 Austrian Eurofiger committee of inquiry: Knoll about Eurofighter and Stealth
- ^ Russia's fifth generation combat aircraft to fly by late 2008-Ivanov
- ^ http://www.publicservice.co.uk/pdf/dmj/issue31/DMJ31%200012%20A%20Brookes%20ATL.pdf
- ^ "Type Acceptance for Block 5 Standard Eurofighter Typhoon". www.eurofighter.com. Eurofighter GmbH. 2007-02-15. Retrieved 2007-06-20.
- ^ http://www.flightglobal.com/articles/2007/03/13/212600/ultra-hornet.html
- ^ http://www.aeronautics.ru/archive/research_literature/aviation_articles/Aviation%20Week/topics/plasma_stealth/index.htm
- ^ Intelligence Community Assessment of the Lieutenant Commander Speicher Case, Iraq Operation Desert Storm Downed Pilot. Central Intelligence Agency, USA.
- ^ ACIG.
- ^ a b Sci.
- ^ F-16 Airframe.
- ^ F-16.
- ^ a b F-16 Timeline 1999.
- ^ Zap 16.
- ^ ACIG.
- ^ Russian fighters superior, says Pentagon.
- ^ Aviation Week and Space Technology,Su-30MK Beats F-15C 'Every Time' secondary archive
- ^ [1]
- ^ MacLeod, Murdo (19 June 2005). "Eurofighter a shooting star in clash with US jets"; Scotsman. Accessed on 29 September 2007.
- ^ F-22 excels at establishing air dominance.
References
- Kelly, Orr (1990). Hornet: the inside story of the F/A-18. Novato: Presido Press. ISBN 0-89141-344-8.
- Shaw, Robert (1985). Fighter Combat: Tactics and Maneuvering. Annapolis: Naval Institute Press. ISBN 0-87021-059-9.
- Sweetman, Bill (2001). "Fighter Tactics". Jane's International Defense Review. Jane's. Retrieved 2006-04-10.
- Kopp, Carlo (2002). "Lockheed-Martin F-35 Joint Strike Fighter Analysis 2002". Air Power Australia. Retrieved 2006-04-10.