Marine life: Difference between revisions
Epipelagic (talk | contribs) →Marine vertebrates: marine tetrapods |
Epipelagic (talk | contribs) |
||
Line 399: | Line 399: | ||
{{Main article|Marine reptile}} |
{{Main article|Marine reptile}} |
||
⚫ | [[Reptiles]] (Late Latin for ''creeping'' or ''crawling'') form a [[Class (biology)|class]] Reptilia of [[tetrapod]]s, [[vertebrates]] that have either four limbs or have four-limbed ancestors. Some reptiles are more closely related to [[bird]]s than other reptiles, and many scientists prefer to make Reptilia a monophyletic group which includes the birds.<ref name=modestoanderson2004>{{cite journal | last1=Modesto | first1=S.P. | last2=Anderson | first2=J.S. | year=2004 | title=The phylogenetic definition of Reptilia | url=http://sysbio.oxfordjournals.org/content/53/5/815.full | journal=Systematic Biology | pmid=15545258 | volume=53 | issue=5| pages=815–821 | doi=10.1080/10635150490503026 }}{{open access}}</ref><ref name=GKT88>{{cite book |last=Gauthier |first=J.A. |author2=Kluge, A.G. |author3= Rowe, T. |year=1988 |chapter=The early evolution of the Amniota |title=The Phylogeny and Classification of the Tetrapods |volume=1 |editors=Benton, M.J. |publisher=Clarendon Press |location=Oxford |isbn=978-0-19-857705-8 |pages=103–155}}</ref><ref name="Laurin 95">{{Cite journal |last=Laurin |first=M. |author2=Reisz, R. R. |year=1995 |title=A reevaluation of early amniote phylogeny |journal=[[Zoological Journal of the Linnean Society]] |volume=113 |issue=2 |pages=165–223 |doi=10.1111/j.1096-3642.1995.tb00932.x |url=http://www.iucn-tftsg.org/wp-content/uploads/file/Articles/Laurin_and_Reisz_1995.pdf}}{{open access}}</ref><ref name=MSP99>{{cite journal |last=Modesto |first=S.P. |year=1999 |title=Observations of the structure of the Early Permian reptile ''Stereosternum tumidum'' Cope |journal=Palaeontologia Africana |volume=35 |pages=7–19 }}</ref> [[Extant taxon|Extant]] non-avian reptiles which inhabit or frequent the sea include [[sea turtle]]s, [[sea snake]]s, [[terrapin]]s, the [[marine iguana]], and the [[saltwater crocodile]]. Currently, of the approximately 12,000 extant [[reptile]] species and sub-species, only about 100 of are classed as marine reptiles.<ref name=":0">{{Cite journal|title = Marine Reptiles|url = http://dx.doi.org/10.1371/journal.pone.0027373|journal = PLoS ONE|date = 2011-11-08|pmc = 3210815|pmid = 22087300|pages = e27373|volume = 6|issue = 11|doi = 10.1371/journal.pone.0027373|first = Arne Redsted|last = Rasmussen|first2 = John C.|last2 = Murphy|first3 = Medy|last3 = Ompi|first4 = J. Whitfield|last4 = Gibbons|first5 = Peter|last5 = Uetz}}</ref> |
||
⚫ | |||
⚫ | Unlike [[amphibian]]s, reptiles do not have an aquatic larval stage. Most reptiles are oviparous, although several species of squamates are [[vivipary|viviparous]], as were some extinct aquatic clades<ref name=S12>{{cite journal |last=Sander |first=P. Martin. |year=2012 |title=Reproduction in early amniotes |journal=Science |volume=337|issue=6096 |pages=806–808 |doi=10.1126/science.1224301}}</ref> — the fetus develops within the mother, contained in a [[placenta]] rather than an [[eggshell]]. As [[amniote]]s, reptile eggs are surrounded by membranes for protection and transport, which adapt them to reproduction on dry land. Many of the viviparous species feed their [[fetus]]es through various forms of placenta analogous to those of [[mammal]]s, with some providing initial care for their hatchlings. |
||
⚫ | Some reptiles are more closely related to [[bird]]s than other reptiles, and many scientists prefer to make Reptilia a monophyletic group which includes the birds.<ref name=modestoanderson2004>{{cite journal | last1=Modesto | first1=S.P. | last2=Anderson | first2=J.S. | year=2004 | title=The phylogenetic definition of Reptilia | url=http://sysbio.oxfordjournals.org/content/53/5/815.full | journal=Systematic Biology | pmid=15545258 | volume=53 | issue=5| pages=815–821 | doi=10.1080/10635150490503026 }}{{open access}}</ref><ref name=GKT88>{{cite book |last=Gauthier |first=J.A. |author2=Kluge, A.G. |author3= Rowe, T. |year=1988 |chapter=The early evolution of the Amniota |title=The Phylogeny and Classification of the Tetrapods |volume=1 |editors=Benton, M.J. |publisher=Clarendon Press |location=Oxford |isbn=978-0-19-857705-8 |pages=103–155}}</ref><ref name="Laurin 95">{{Cite journal |last=Laurin |first=M. |author2=Reisz, R. R. |year=1995 |title=A reevaluation of early amniote phylogeny |journal=[[Zoological Journal of the Linnean Society]] |volume=113 |issue=2 |pages=165–223 |doi=10.1111/j.1096-3642.1995.tb00932.x |url=http://www.iucn-tftsg.org/wp-content/uploads/file/Articles/Laurin_and_Reisz_1995.pdf}}{{open access}}</ref><ref name=MSP99>{{cite journal |last=Modesto |first=S.P. |year=1999 |title=Observations of the structure of the Early Permian reptile ''Stereosternum tumidum'' Cope |journal=Palaeontologia Africana |volume=35 |pages=7–19 }}</ref> |
||
Most extant marine reptiles, except for some sea snakes, are [[oviparity|oviparous]] and need to return to land to lay their eggs. Thus most species, excepting sea turtles, spend most of their lives on or near land rather than in the ocean. Despite their marine adaptations, most sea snakes prefer shallow waters nearby land, around islands, especially waters that are somewhat sheltered, as well as near estuaries.<ref name="Sti74">Stidworthy J. 1974. Snakes of the World. Grosset & Dunlap Inc. 160 pp. ISBN 0-448-11856-4.</ref><ref name="RAS-FAO">[ftp://ftp.fao.org/docrep/fao/009/y0870e/y0870e65.pdf Sea snakes] at [http://www.fao.org/ Food and Agriculture Organization of the United Nations]. Accessed 7 August 2007.</ref> Some [[extinction|extinct]] marine reptiles, such as [[ichthyosaur]]s, evolved to be [[viviparity|viviparous]] and had no requirement to return to land. |
|||
<gallery mode=packed heights=140px style=float:left;> |
<gallery mode=packed heights=140px style=float:left;> |
||
Line 409: | Line 411: | ||
{{clear}} |
{{clear}} |
||
The earliest marine reptiles arose in the [[Permian]]. During the [[Mesozoic]] many groups of reptiles became adapted to life in the seas, including [[ichthyosaur]]s, [[plesiosaur]]s, [[mosasaur]]s, [[nothosaur]]s, [[placodont]]s, [[sea turtle]]s, [[thalattosaur]]s and [[thalattosuchia]]ns. Marine reptiles were less numerous after [[mass extinction]] at the end of the [[Cretaceous]]. |
|||
The earliest known proto-reptiles originated around 312 million years ago during the [[Carboniferous]] period, having evolved from advanced [[Reptiliomorpha|reptiliomorph]] tetrapods that became increasingly adapted to life on dry land. Some early examples include the lizard-like ''[[Hylonomus]]'' and ''[[Casineria]]''. In addition to the living reptiles, there are many diverse groups that are now [[Extinction|extinct]], in some cases due to [[mass extinction]] events. In particular, the [[Cretaceous–Paleogene extinction event|K–Pg extinction]] wiped out the [[Pterosauria|pterosaurs]], [[Plesiosauria|plesiosaurs]], [[ornithischia]]ns, and [[Sauropoda|sauropods]], as well as many species of [[Theropoda|theropods]] (e.g. [[Tyrannosauridae|tyrannosaurids]] and [[Dromaeosauridae|dromaeosaurids]]), [[crocodyliform]]s, and [[Squamata|squamates]] (e.g. [[mosasaur]]ids). |
|||
====Marine birds==== |
====Marine birds==== |
Revision as of 05:22, 14 August 2016


Marine life refers to the plants, animals and other organisms that live in the ocean. At a fundamental level, marine life helps determine the very nature of our planet. Marine organisms produce much of the oxygen we breathe and probably help regulate the earth's climate. Shorelines are in part shaped and protected by marine life, and some marine organisms even help create new land.
Most life forms evolved initially in marine habitats. The earliest vertebrates appeared in the form of fish, which live exclusively in water. Some of these evolved into amphibians which spend portions of their lives in water and portions on land. Others fish evolved into land mammals and subsequently returned to the ocean as seals, dolphins or whales. Plant forms such as kelp and algae grow in the water and are the basis for some underwater ecosystems. Plankton is generally the foundation of the ocean food chain.
Marine vertebrates must obtain oxygen to survive, and they do so in various ways. Fish have gills instead of lungs, although some species of fish, such as the lungfish, have both. Marine mammals, such as dolphins, whales, otters, and seals need to surface periodically to breathe air. Some amphibians are able to absorb oxygen through their skin. Invertebrates exhibit a wide range of modifications to survive in poorly oxygenated waters including breathing tubes (see insect and mollusc siphons) and gills (Carcinus). However as invertebrate life evolved in an aquatic habitat most have little or no specialisation for respiration in water.
Altogether there are 230,000 documented marine species, and it has been estimated that nearly two million marine species are yet to be documented.[1] Marine species range in size from the microscopic, including plankton and phytoplankton which can be as small as 0.02 micrometres and are both important as key primary producers of the sea, to huge cetaceans (whales, dolphins and porpoises) which in the case of the blue whale reach up to 33 metres (109 feet) in length.
Water
There is no life without water, which has been characterised as the "solvent of life".[2] The Nobel prize winner Albert Szent-Györgyi referred to water as the mater und matrix, the mater and matrix of life.[3]
Evolution
−4500 — – — – −4000 — – — – −3500 — – — – −3000 — – — – −2500 — – — – −2000 — – — – −1500 — – — – −1000 — – — – −500 — – — – 0 — |
| |||||||||||||||||||||||||||||||||||||||||||||
The Earth is about 4.54 billion years old.[4][5][6] The earliest undisputed evidence of life on Earth dates from at least 3.5 billion years ago,[7][8] during the Eoarchean Era after a geological crust started to solidify following the earlier molten Hadean Eon. Microbial mat fossils have been found in 3.48 billion-year-old sandstone in Western Australia.[9][10][11] Other early physical evidence of a biogenic substance is graphite in 3.7 billion-year-old metasedimentary rocks discovered in Western Greenland[12] as well as "remains of biotic life" found in 4.1 billion-year-old rocks in Western Australia.[13][14] According to one of the researchers, "If life arose relatively quickly on Earth … then it could be common in the universe."[13]
All organisms on Earth are descended from a common ancestor or ancestral gene pool.[15][16] Highly energetic chemistry is thought to have produced a self-replicating molecule around 4 billion years ago, and half a billion years later the last common ancestor of all life existed.[17] The current scientific consensus is that the complex biochemistry that makes up life came from simpler chemical reactions.[18] The beginning of life may have included self-replicating molecules such as RNA[19] and the assembly of simple cells.[20]
Current species are a stage in the process of evolution, with their diversity the product of a long series of speciation and extinction events.[21] The common descent of organisms was first deduced from four simple facts about organisms: First, they have geographic distributions that cannot be explained by local adaptation. Second, the diversity of life is not a set of completely unique organisms, but organisms that share morphological similarities. Third, vestigial traits with no clear purpose resemble functional ancestral traits and finally, that organisms can be classified using these similarities into a hierarchy of nested groups—similar to a family tree.[22] However, modern research has suggested that, due to horizontal gene transfer, this "tree of life" may be more complicated than a simple branching tree since some genes have spread independently between distantly related species.[23][24]
Past species have also left records of their evolutionary history. Fossils, along with the comparative anatomy of present-day organisms, constitute the morphological, or anatomical, record.[25] By comparing the anatomies of both modern and extinct species, paleontologists can infer the lineages of those species. However, this approach is most successful for organisms that had hard body parts, such as shells, bones or teeth. Further, as prokaryotes such as bacteria and archaea share a limited set of common morphologies, their fossils do not provide information on their ancestry.
More recently, evidence for common descent has come from the study of biochemical similarities between organisms. For example, all living cells use the same basic set of nucleotides and amino acids.[26] The development of molecular genetics has revealed the record of evolution left in organisms' genomes: dating when species diverged through the molecular clock produced by mutations.[27] For example, these DNA sequence comparisons have revealed that humans and chimpanzees share 98% of their genomes and analysing the few areas where they differ helps shed light on when the common ancestor of these species existed.[28]

Prokaryotes inhabited the Earth from approximately 3–4 billion years ago.[30][31] No obvious changes in morphology or cellular organisation occurred in these organisms over the next few billion years.[32] The eukaryotic cells emerged between 1.6–2.7 billion years ago. The next major change in cell structure came when bacteria were engulfed by eukaryotic cells, in a cooperative association called endosymbiosis.[33][34] The engulfed bacteria and the host cell then underwent coevolution, with the bacteria evolving into either mitochondria or hydrogenosomes.[35] Another engulfment of cyanobacterial-like organisms led to the formation of chloroplasts in algae and plants.[36]
The history of life was that of the unicellular eukaryotes, prokaryotes and archaea until about 610 million years ago when multicellular organisms began to appear in the oceans in the Ediacaran period.[30][37] The evolution of multicellularity occurred in multiple independent events, in organisms as diverse as sponges, brown algae, cyanobacteria, slime moulds and myxobacteria.[38] In January 2016, scientists reported that, about 800 million years ago, a minor genetic change in a single molecule called GK-PID may have allowed organisms to go from a single cell organism to one of many cells.[39]
Soon after the emergence of these first multicellular organisms, a remarkable amount of biological diversity appeared over approximately 10 million years, in an event called the Cambrian explosion. Here, the majority of types of modern animals appeared in the fossil record, as well as unique lineages that subsequently became extinct.[40] Various triggers for the Cambrian explosion have been proposed, including the accumulation of oxygen in the atmosphere from photosynthesis.[41]
About 500 million years ago, plants and fungi colonised the land and were soon followed by arthropods and other animals.[42] Insects were particularly successful and even today make up the majority of animal species.[43] Amphibians first appeared around 364 million years ago, followed by early amniotes and birds around 155 million years ago (both from "reptile"-like lineages), mammals around 129 million years ago, homininae around 10 million years ago and modern humans around 250,000 years ago.[44][45][46] However, despite the evolution of these large animals, smaller organisms similar to the types that evolved early in this process continue to be highly successful and dominate the Earth, with the majority of both biomass and species being prokaryotes.[47]
Estimates on the number of Earth's current species range from 10 million to 14 million,[48] of which about 1.2 million have been documented and over 86 percent have not yet been described.[49]
Marine microorganisms
A microorganism (or microbe) is a microscopic living organism, which may be single-celled[50] or multicellular. Microorganisms are very diverse and include all bacteria, archaea and most protozoa. This group also contains some species of fungi, algae, and certain microscopic animals, such as rotifers.
Many macroscopic animals and plants have microscopic juvenile stages. Some microbiologists also classify viruses (and viroids) as microorganisms, but others consider these as nonliving.[51][52] In July 2016, scientists reported identifying a set of 355 genes from the last universal common ancestor (LUCA) of all life, including microorganisms, living on Earth.[53]
Microorganisms live in every part of the biosphere, including soil, hot springs, "seven miles deep" in the ocean, "40 miles high" in the atmosphere and inside rocks far down within the Earth's crust (see also endolith).[54] Microorganisms, under certain test conditions, have been observed to thrive in the vacuum of outer space.[55][56] According to some estimates, microorganisms outweigh "all other living things combined thousands of times over".[57] The mass of prokaryote microorganisms — which includes bacteria and archaea, but not the nucleated eukaryote microorganisms — may be as much as 0.8 trillion tons of carbon (of the total biosphere mass, estimated at between 1 and 4 trillion tons).[58] On 17 March 2013, researchers reported data that suggested microbial life forms thrive in the Mariana Trench. the deepest spot in the Earth's oceans.[59][60] Other researchers reported related studies that microorganisms thrive inside rocks up to 580 m (1,900 ft; 0.36 mi) below the sea floor under 2,590 m (8,500 ft; 1.61 mi) of ocean off the coast of the northwestern United States,[59][61] as well as 2,400 m (7,900 ft; 1.5 mi) beneath the seabed off Japan.[62] On 20 August 2014, scientists confirmed the existence of microorganisms living 800 m (2,600 ft; 0.50 mi) below the ice of Antarctica.[63][64] According to one researcher, "You can find microbes everywhere — they're extremely adaptable to conditions, and survive wherever they are."[59]
Microorganisms are crucial to nutrient recycling in ecosystems as they act as decomposers. A small proportion of microorganisms are pathogenic, causing disease and even death in plants and animals.[65] As inhabitants of the largest environment on Earth, microbial marine systems drive changes in every global system. Microbes are responsible for virtually all the photosynthesis that occurs in the ocean, as well as the cycling of carbon, nitrogen, phosphorus and other nutrients and trace elements.[66]
Microscopic life undersea is incredibly diverse and still poorly understood. For example, the role of viruses in marine ecosystems is barely being explored even in the beginning of the 21st century.[67]
A teaspoon of seawater contains about one million viruses.[68] Most of these are bacteriophages, which are harmless to plants and animals, and are in fact essential to the regulation of saltwater and freshwater ecosystems.[69] They infect and destroy bacteria in aquatic microbial communities, and are the most important mechanism of recycling carbon in the marine environment. The organic molecules released from the dead bacterial cells stimulate fresh bacterial and algal growth.[70] Viral activity may also contribute to the biological pump, the process whereby carbon is sequestered in the deep ocean.[71]
Marine bacteriophages are viruses that live as obligate parasitic agents in marine bacteria such as cyanobacteria.[72] Their existence was discovered through electron microscopy and epifluorescence microscopy of ecological water samples, and later through metagenomic sampling of uncultured viral samples.[72][73] The tailed bacteriophages appear to dominate marine ecosystems in number and diversity of organisms.[72] However, viruses belonging to families Corticoviridae,[74] Inoviridae[75] and Microviridae[76] are also known to infect diverse marine bacteria. Metagenomic evidence suggests that microviruses (icosahedral ssDNA phages) are particularly prevalent in marine habitats.[76]
Bacteriophages, viruses that are parasitic on bacteria, were first discovered in the early twentieth century. Scientists today consider that their importance in ecosystems, particularly marine ecosystems, has been underestimated, leading to these infectious agents being poorly investigated and their numbers and species biodiversity being greatly under reported.[77]
Microorganisms constitute more than 90% of the biomass in the sea. It is estimated that viruses kill approximately 20% of this biomass each day and that there are 15 times as many viruses in the oceans as there are bacteria and archaea. Viruses are the main agents responsible for the rapid destruction of harmful algal blooms,[78] which often kill other marine life.[79] The number of viruses in the oceans decreases further offshore and deeper into the water, where there are fewer host organisms.[71]
Marine viruses

A virus is a small infectious agent that replicates only inside the living cells of other organisms. Viruses can infect all types of life forms, from animals and plants to microorganisms, including bacteria and archaea.[80]
When not inside an infected cell or in the process of infecting a cell, viruses exist in the form of independent particles. These viral particles, also known as virions, consist of two or three parts: (i) the genetic material made from either DNA or RNA, long molecules that carry genetic information; (ii) a protein coat, called the capsid, which surrounds and protects the genetic material; and in some cases (iii) an envelope of lipids that surrounds the protein coat when they are outside a cell. The shapes of these virus particles range from simple helical and icosahedral forms for some virus species to more complex structures for others. Most virus species have virions that are too small to be seen with an optical microscope. The average virion is about one one-hundredth the size of the average bacterium.
The origins of viruses in the evolutionary history of life are unclear: some may have evolved from plasmids—pieces of DNA that can move between cells—while others may have evolved from bacteria. In evolution, viruses are an important means of horizontal gene transfer, which increases genetic diversity.[81] Viruses are considered by some to be a life form, because they carry genetic material, reproduce, and evolve through natural selection. However they lack key characteristics (such as cell structure) that are generally considered necessary to count as life. Because they possess some but not all such qualities, viruses have been described as "organisms at the edge of life"[82] and as replicators.[83]
Viruses are found wherever there is life and have probably existed since living cells first evolved.[84] The origin of viruses is unclear because they do not form fossils, so molecular techniques have been used to compare the DNA or RNA of viruses and are a useful means of investigating how they arose.[85]
Viruses are now recognised as ancient and as having origins that pre-date the divergence of life into the three domains.[86]
Opinions differ on whether viruses are a form of life, or organic structures that interact with living organisms.[87] They have been described as "organisms at the edge of life",[82] since they resemble organisms in that they possess genes, evolve by natural selection,[88] and reproduce by creating multiple copies of themselves through self-assembly. Although they have genes, they do not have a cellular structure, which is often seen as the basic unit of life. Viruses do not have their own metabolism, and require a host cell to make new products. They therefore cannot naturally reproduce outside a host cell.[89]
Bacterial viruses, called bacteriophages, are a common and diverse group of viruses and are the most abundant form of biological entity in aquatic environments – there are up to ten times more of these viruses in the oceans than there are bacteria,[90] reaching levels of 250,000,000 bacteriophages per millilitre of seawater.[91]
There are also archaean viruses which replicate within archaea: these are double-stranded DNA viruses with unusual and sometimes unique shapes.[92][93] These viruses have been studied in most detail in the thermophilic archaea, particularly the orders Sulfolobales and Thermoproteales.[94]
A teaspoon of seawater contains about one million viruses.[68] Most of these are bacteriophages, which are harmless to plants and animals, and are in fact essential to the regulation of saltwater and freshwater ecosystems.[69] They infect and destroy bacteria in aquatic microbial communities, and are the most important mechanism of recycling carbon in the marine environment. The organic molecules released from the dead bacterial cells stimulate fresh bacterial and algal growth.[70] Viral activity may also contribute to the biological pump, the process whereby carbon is sequestered in the deep ocean.[71]
Microorganisms constitute more than 90% of the biomass in the sea. It is estimated that viruses kill approximately 20% of this biomass each day and that there are 15 times as many viruses in the oceans as there are bacteria and archaea. Viruses are the main agents responsible for the rapid destruction of harmful algal blooms,[78] which often kill other marine life.[79] The number of viruses in the oceans decreases further offshore and deeper into the water, where there are fewer host organisms.[71]
Viruses are an important natural means of transferring genes between different species, which increases genetic diversity and drives evolution.[81] It is thought that viruses played a central role in the early evolution, before the diversification of bacteria, archaea and eukaryotes, at the time of the last universal common ancestor of life on Earth.[95] Viruses are still one of the largest reservoirs of unexplored genetic diversity on Earth.[71]
Marine bacteria


Bacteria constitute a large domain of prokaryotic microorganisms. Typically a few micrometres in length, bacteria have a number of shapes, ranging from spheres to rods and spirals. Bacteria were among the first life forms to appear on Earth, and are present in most of its habitats. Bacteria inhabit soil, water, acidic hot springs, radioactive waste,[97] and the deep portions of Earth's crust. Bacteria also live in symbiotic and parasitic relationships with plants and animals.
Once regarded as plants constituting the class Schizomycetes, bacteria are now classified as prokaryotes. Unlike cells of animals and other eukaryotes, bacterial cells do not contain a nucleus and rarely harbour membrane-bound organelles. Although the term bacteria traditionally included all prokaryotes, the scientific classification changed after the discovery in the 1990s that prokaryotes consist of two very different groups of organisms that evolved from an ancient common ancestor. These evolutionary domains are called Bacteria and Archaea.[98]
The ancestors of modern bacteria were unicellular microorganisms that were the first forms of life to appear on Earth, about 4 billion years ago. For about 3 billion years, most organisms were microscopic, and bacteria and archaea were the dominant forms of life.[99][100] Although bacterial fossils exist, such as stromatolites, their lack of distinctive morphology prevents them from being used to examine the history of bacterial evolution, or to date the time of origin of a particular bacterial species. However, gene sequences can be used to reconstruct the bacterial phylogeny, and these studies indicate that bacteria diverged first from the archaeal/eukaryotic lineage.[101] Bacteria were also involved in the second great evolutionary divergence, that of the archaea and eukaryotes. Here, eukaryotes resulted from the entering of ancient bacteria into endosymbiotic associations with the ancestors of eukaryotic cells, which were themselves possibly related to the Archaea.[34][102] This involved the engulfment by proto-eukaryotic cells of alphaproteobacterial symbionts to form either mitochondria or hydrogenosomes, which are still found in all known Eukarya. Later on, some eukaryotes that already contained mitochondria also engulfed cyanobacterial-like organisms. This led to the formation of chloroplasts in algae and plants. There are also some algae that originated from even later endosymbiotic events. Here, eukaryotes engulfed a eukaryotic algae that developed into a "second-generation" plastid.[103][104] This is known as secondary endosymbiosis.
In 2000 a team of microbiologists led by Edward DeLong made a crucial discovery in the understanding of the marine carbon and energy cycles. They discovered a gene in several species of bacteria[105][106] responsible for production of the protein rhodopsin, previously unheard of in the domain Bacteria. These proteins found in the cell membranes are capable of converting light energy to biochemical energy due to a change in configuration of the rhodopsin molecule as sunlight strikes it, causing the pumping of a proton from inside out and a subsequent inflow that generates the energy.[107]
Marine archaea

The archaea constitute a domain and kingdom of single-celled microorganisms. These microbes are prokaryotes, meaning that they have no cell nucleus or any other membrane-bound organelles in their cells.
Archaea were initially classified as bacteria, but this classification is outdated.[108] Archaeal cells have unique properties separating them from the other two domains of life, Bacteria and Eukaryota. The Archaea are further divided into multiple recognized phyla. Classification is difficult because the majority have not been isolated in the laboratory and have only been detected by analysis of their nucleic acids in samples from their environment.
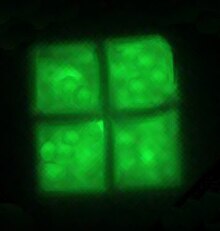
Archaea and bacteria are generally similar in size and shape, although a few archaea have very strange shapes, such as the flat and square-shaped cells of Haloquadratum walsbyi.[109] Despite this morphological similarity to bacteria, archaea possess genes and several metabolic pathways that are more closely related to those of eukaryotes, notably the enzymes involved in transcription and translation. Other aspects of archaeal biochemistry are unique, such as their reliance on ether lipids in their cell membranes, such as archaeols. Archaea use more energy sources than eukaryotes: these range from organic compounds, such as sugars, to ammonia, metal ions or even hydrogen gas. Salt-tolerant archaea (the Haloarchaea) use sunlight as an energy source, and other species of archaea fix carbon; however, unlike plants and cyanobacteria, no known species of archaea does both. Archaea reproduce asexually by binary fission, fragmentation, or budding; unlike bacteria and eukaryotes, no known species forms spores.
Archaea were initially viewed as extremophiles living in harsh environments, such as hot springs and salt lakes, but they have since been found in a broad range of habitats.[110] Archaea are particularly numerous in the oceans, and the archaea in plankton may be one of the most abundant groups of organisms on the planet. Archaea are a major part of Earth's life and may play roles in both the carbon cycle and the nitrogen cycle.
Marine algae and plants
Microscopic algae and plants provide important habitats for life, sometimes acting as hiding and foraging places for larval forms of larger fish and invertebrates.
Algal life is widespread and very diverse under the ocean. Microscopic photosynthetic algae contribute a larger proportion of the world's photosynthetic output than all the terrestrial forests combined. Most of the niche occupied by sub plants on land is actually occupied by macroscopic algae in the ocean, such as Sargassum and kelp, which are commonly known as seaweeds that create kelp forests.
Plants that survive in the sea are often found in shallow waters, such as the seagrasses (examples of which are eelgrass, Zostera, and turtle grass, Thalassia). These plants have adapted to the high salinity of the ocean environment. The intertidal zone is also a good place to find plant life in the sea, where mangroves or cordgrass or beach grass might grow. Microscopic algae and plants provide important habitats for life, sometimes acting as hiding and foraging places for larval forms of larger fish and invertebrates.
Marine fungi


Over 1500 species of fungi are known from marine environments.[111] These are parasitic on marine algae or animals, or are saprobes on algae, corals, protozoan cysts, sea grasses, wood and other substrata, and can also be found in sea foam.[112] Spores of many species have special appendages which facilitate attachment to the substratum.[113] A very diverse range of unusual secondary metabolites is produced by marine fungi.[114]
Mycoplankton are saprotropic members of the plankton communities of marine and freshwater ecosystems.[115][116] They are composed of filamentous free-living fungi and yeasts that are associated with planktonic particles or phytoplankton.[117] Similar to bacterioplankton, these aquatic fungi play a significant role in heterotrophic mineralization and nutrient cycling.[118] Mycoplankton can be up to 20 mm in diameter and over 50 mm in length.[119]
In a typical milliliter of seawater, there are approximately 103 to 104 fungal cells.[120] This number is greater in coastal ecosystems and estuaries due to nutritional runoff from terrestrial communities. The greatest diversity and number of species of mycoplankton is found in surface waters (< 1000 m), and the vertical profile depends on the abundance of phytoplankton.[121][122] Furthermore, this difference in distribution may vary between seasons due to nutrient availability.[123] Marine fungi survive in a constant oxygen deficient environment, and therefore depend on oxygen diffusion by turbulence and oxygen generated by photosynthetic organisms.[124]
Marine fungi can be classified as:[124]
- Lower fungi - adapted to marine habitats (zoosporic fungi, including mastigomycetes: oomycetes and chytridiomycetes)
- Higher fungi - filamentous, modified to planktonic lifestyle (hyphomycetes, ascomycetes, basidiomycetes)
- Terrestrial fungi - contain appendages of marine fungi (trichomycetes)
Most mycoplankton species are higher fungi.[121]
Lichens are mutualistic associations between a fungus, usually an ascomycete, and an alga or a cyanobacterium. Several lichens are found in marine environments.[125] Many more occur in the splash zone, where they occupy different vertical zones depending on how tolerant they are to submersion.[126] Fossil marine lichens 600 million years old have been discovered in the late Neoproterozoic marine phosphate rocks in the sedimentary, fossil-rich Doushantuo Formation in China.[127]
According to fossil records, fungi date back to the late Proterozoic era 900-570 million years ago. It has been hypothesized that mycoplankton evolved from terrestrial fungi, likely in the Paleozoic era (390 million years ago).[128]
Marine invertebrates
Marine invertebrates are all multicellular animals that inhabit a marine environment apart from the vertebrate members of the chordate phylum; invertebrates, lack a vertebral column. Some have evolved a shell or a hard exoskeleton.
Arthropods total about 1,113,000 described extant species, molluscs about 85,000 and chordates about 52,000.[129][130]
Marine sponges

Sponges are multicellular organisms that have bodies full of pores and channels allowing water to circulate through them, consisting of jelly-like mesohyl sandwiched between two thin layers of cells. They have unspecialized cells that can transform into other types and that often migrate between the main cell layers and the mesohyl in the process. Sponges do not have nervous, digestive or circulatory systems. Instead, most rely on maintaining a constant water flow through their bodies to obtain food and oxygen and to remove wastes.
Sponges are similar to other animals in that they are multicellular, heterotrophic, lack cell walls and produce sperm cells. Unlike other animals, they lack true tissues and organs, and have no body symmetry. The shapes of their bodies are adapted for maximal efficiency of water flow through the central cavity, where it deposits nutrients, and leaves through a hole called the osculum. Many sponges have internal skeletons of spongin and/or spicules of calcium carbonate or silicon dioxide. All sponges are sessile aquatic animals. Although there are freshwater species, the great majority are marine (salt water) species, ranging from tidal zones to depths exceeding 8,800 m (5.5 mi).
While most of the approximately 5,000–10,000 known species feed on bacteria and other food particles in the water, some host photosynthesizing micro-organisms as endosymbionts and these alliances often produce more food and oxygen than they consume. A few species of sponge that live in food-poor environments have become carnivores that prey mainly on small crustaceans.[131]
Marine cnidarians
Cnidarians (Greek for nettle) are distinguished by the presence of stinging cells, specialized cells that they use mainly for capturing prey. Cnidarians include corals, sea anemones, jellyfish and hydrozoans. They form a phylum containing over 10,000[132] species of animals found exclusively in aquatic (mainly marine) environments. Their bodies consist of mesoglea, a non-living jelly-like substance, sandwiched between two layers of epithelium that are mostly one cell thick. They have two basic body forms: swimming medusae and sessile polyps, both of which are radially symmetrical with mouths surrounded by tentacles that bear cnidocytes. Both forms have a single orifice and body cavity that are used for digestion and respiration.
Fossil cnidarians have been found in rocks formed about 580 million years ago. Fossils of cnidarians that do not build mineralized structures are rare. Scientists currently think cnidarians, ctenophores and bilaterians are more closely related to calcareous sponges than these are to other sponges, and that anthozoans are the evolutionary "aunts" or "sisters" of other cnidarians, and the most closely related to bilaterians.
Cnidarians are the simplest animals in which the cells are organised into tissues.[133] The starlet sea anemone is used as a model organism in research.[134] It is easy to care for in the laboratory and a protocol has been developed which can yield large numbers of embryos on a daily basis.[135] There is a remarkable degree of similarity in the gene sequence conservation and complexity between the sea anemone and vertebrates.[135]In particular, genes concerned in the formation of the head in vertebrates are also present in the anemone.[136][137]
Marine worms

Worms (Old English for serpent) typically have long cylindrical tube-like bodies and no limbs. Marine worms vary in size from microscopic to over 1 metre (3.3 ft) in length for some marine polychaete worms (bristle worms)[138] and up to 58 metres (190 ft) for the marine nemertean worm (bootlace worm).[139] Some marine worms occupy a small variety of parasitic niches, living inside the bodies of other animals, while others live more freely in the marine environment or by burrowing underground.
Different groups of marine worms are related only distantly, so they are found in several different phyla such as the Platyhelminthes, Annelida (segmented worms), Chaetognatha, Hemichordata, and Phoronida. Many of these worms have specialized tentacles used for exchanging oxygen and carbon dioxide and also may be used for reproduction. Some marine worms are tube worms, such as the giant tube worm which lives in waters near underwater volcanoes and can withstand temperatures up to 90 degrees Celsius.
Nematodes (roundworms) constitute a further phylum which has tubular digestive systems with openings at both ends.[140][141] Over 25,000 nematode species have been described,[142][143] and it has been estimated another million remain undescribed.[144] They are ubiquitous in marine, freshwater and terrestrial environments, where they often outnumber other animals in both individual and species counts. They are found in locations as diverse as mountains, deserts and oceanic trenches, as well as every part of the earth's lithosphere.[145] By count they represent 90% of all animals on the ocean floor.[146] Their numerical dominance, often exceeding a million individuals per square meter and accounting for about 80% of all individual animals on earth, their diversity of life cycles, and their presence at various trophic levels point at an important role in many ecosystems.[147]
-
The bootlace worm can grow to 58 metres (190 ft).
-
Giant tube worms cluster around hydrothermal vents
-
Nematodes are ubiquitous pseudocoelomates which can parasite marine plants and animals.
-
Bloodworms are typically found on the bottom of shallow marine waters
Echinoderms

Echinoderms (Greek for spiny skin) is a phylum which contains only marine invertebrates. The adults are recognizable by their radial symmetry (usually five-point) and include starfish, sea urchins, sand dollars, and sea cucumbers, as well as the sea lilies.[148] Echinoderms are found at every ocean depth, from the intertidal zone to the abyssal zone. The phylum contains about 7000 living species,[149] making it the second-largest grouping of deuterostomes (a superphylum), after the chordates (which include the vertebrates, such as birds, fishes, mammals, and reptiles).
Echinoderms are unique among animals in having bilateral symmetry at the larval stage, but fivefold symmetry (pentamerism, a special type of radial symmetry) as adults.[150]
The echinoderms are important both biologically and geologically. Biologically, there are few other groupings so abundant in the biotic desert of the deep sea, as well as shallower oceans. The more notably distinct trait, which most echinoderms have, is their remarkable powers of regeneration of tissue, organs, limbs, and of asexual reproduction, and in some cases, complete regeneration from a single limb. Geologically, the value of echinoderms is in their ossified skeletons, which are major contributors to many limestone formations, and can provide valuable clues as to the geological environment. They were the most used species in regenerative research in the 19th and 20th centuries. Further, it is held by some scientists that the radiation of echinoderms was responsible for the Mesozoic Marine Revolution.
-
Echinoderm literally means "spiny skin", as this water melon sea urchin illustrates
-
Sea cucumbers filter feed on plankton and suspended solids
-
Benthopelagic sea cucumbers can lift off the seafloor and journey as much as 1,000 m (3,300 ft) up the water column
-
The ochre sea star was the first keystone predator to be studied. They limit mussels which can overwhelm intertidal communities.[151]
-
Colorful sea lilies in shallow waters
Aside from the hard-to-classify Arkarua (a Precambrian animal with echinoderm-like pentamerous radial symmetry), the first definitive members of the phylum appeared near the start of the Cambrian.
Marine molluscs

Molluscs (Latin for soft) form a phylum with about 85,000 extant recognized species.[152] They are the largest marine phylum, comprising about 23% of all the named marine organisms. Molluscs have more varied forms than other animal phylums. They are highly diverse, not just in size and in anatomical structure, but also in behaviour and in habitat. The majority of species still live in the oceans, from the seashores to the abyssal zone, but some form a significant part of the freshwater fauna and the terrestrial ecosystems. The phylum is divided into 9 or 10 taxonomic classes, two of which are extinct. They include snails, slugs and other gastropods; clams and other bivalves; squids and other cephalopods; and other lesser-known but similarly distinctive subgroups. Gastropods (snails and slugs) are by far the most numerous molluscs in terms of classified species, and account for 80% of the total.[129] Cephalopoda such as squid, cuttlefish, and octopuses are among the neurologically most advanced of all invertebrates.[153]
Molluscs have such diverse shapes that many textbooks base their descriptions of molluscan anatomy on a generalized or hypothetical ancestral mollusc. This generalized mollusc is unsegmented and bilaterally symmetrical with an underside consisting of a single muscular foot.[154][155] Beyond that it has three further key features. Firstly, it has a muscular cloak called a mantle covering its viscera and containing a significant cavity used for breathing and excretion. A shell secreted by the mantle covers the upper surface.[155] Secondly (apart from bivalves) it has a rasping tongue called a radula used for feeding. Thirdly, it has a nervous system including a complex digestive system using microscopic, muscle-powered hairs called cilia to exude mucus. The generalized mollusc has two paired nerve cords (three in bivalves). The brain, in species that have one, encircles the esophagus. Most molluscs have eyes and all have sensors detecting chemicals, vibrations, and touch. The simplest type of molluscan reproductive system relies on external fertilization, but more complex variations occur. All produce eggs, from which may emerge trochophore larvae, more complex veliger larvae, or miniature adults. The depiction is rather similar to modern monoplacophorans, and some suggest it may resemble very early molluscs.[156][154][157][158]
In 1969 David Nicol estimated the probable total number of living molluscs at 107,000 of which about were 80,000 marine.[159] In 2009, Chapman estimated the number of described living species at 85,000.[152] Haszprunar in 2001 estimated about 93,000 named species,[160] which include 23% of all named marine organisms.[161] About 200,000 living species in total are estimated,[152][162] and 70,000 fossil species,[163] although the total number of mollusc species ever to have existed, whether or not preserved, must be many times greater than the number alive today.[164]
-
Colossal squid, largest of all invertebrates [165]
-
The nautilus is a living fossil little changed since it evolved 500 million years ago as one of the first cephalopods.[166][167][168]
-
The sea snail Syrinx aruanus has the largest shell of any living gastropod
-
Molluscs usually have eyes. Bordering the edge of the mantle of a scallop, a bivalve mollusc, can be over 100 simple eyes.
-
Common mussel, another bivalve
Good evidence exists for the appearance of marine gastropods, cephalopods and bivalves in the Cambrian period 538.8 to 485.4 million years ago. However, the evolutionary history both of molluscs' emergence from the ancestral Lophotrochozoa and of their diversification into the well-known living and fossil forms are still subjects of vigorous debate among scientists.
Marine arthropods
Arthropods (Greek for jointed feet) have an exoskeleton (external skeleton), a segmented body, and jointed appendages (paired appendages). They form a phylum which includes insects, arachnids, myriapods, and crustaceans. Arthropods are characterized by their jointed limbs and cuticle made of chitin, often mineralised with calcium carbonate. The arthropod body plan consists of segments, each with a pair of appendages. The rigid cuticle inhibits growth, so arthropods replace it periodically by moulting. Their versatility has enabled them to become the most species-rich members of all ecological guilds in most environments.
Marine arthropods range in size from the microscopic crustacean Stygotantulus to the Japanese spider crab. Arthropods' primary internal cavity is a hemocoel, which accommodates their internal organs, and through which their haemolymph - analogue of blood - circulates; they have open circulatory systems. Like their exteriors, the internal organs of arthropods are generally built of repeated segments. Their nervous system is "ladder-like", with paired ventral nerve cords running through all segments and forming paired ganglia in each segment. Their heads are formed by fusion of varying numbers of segments, and their brains are formed by fusion of the ganglia of these segments and encircle the esophagus. The respiratory and excretory systems of arthropods vary, depending as much on their environment as on the subphylum to which they belong.
Their vision relies on various combinations of compound eyes and pigment-pit ocelli: in most species the ocelli can only detect the direction from which light is coming, and the compound eyes are the main source of information, but the main eyes of spiders are ocelli that can form images and, in a few cases, can swivel to track prey. Arthropods also have a wide range of chemical and mechanical sensors, mostly based on modifications of the many setae (bristles) that project through their cuticles. Arthropods' methods of reproduction and development are diverse; all terrestrial species use internal fertilization, but this is often by indirect transfer of the sperm via an appendage or the ground, rather than by direct injection. Marine species all lay eggs and use either internal or external fertilization. Arthropod hatchlings vary from miniature adults to grubs that lack jointed limbs and eventually undergo a total metamorphosis to produce the adult form.
-
Trilobites, now extinct, roamed oceans for 270 million years.[170]
-
Horseshoe crab, a living fossil arthropod from 450 million years ago
-
The banded cleaner shrimp is a crustacean common in the tropics.
-
Many crustaceans are very small, like this tiny amphipod, and make up a significant part of the ocean's zooplankton
-
The Japanese spider crab has the longest leg span of any arthropod.
The evolutionary ancestry of arthropods dates back to the Cambrian period. The group is generally regarded as monophyletic, and many analyses support the placement of arthropods with cycloneuralians (or their constituent clades) in a superphylum Ecdysozoa. Overall however, the basal relationships of Metazoa are not yet well resolved. Likewise, the relationships between various arthropod groups are still actively debated.
Others
- Tardigrade, Lobopodia, (Onychophora)
- Non-craniate chordates: Cephalochordate, Tunicata and Haikouella
-
The lancelet, a small translucent fish-like Cephalochordate, is the closest living invertebrate relative of the vertebrates.[171][172]
Marine vertebrates
Amphibians mostly require fresh water to reproduce. A few inhabit brackish water, but there are no true marine amphibians.[173] There have been reports, however, of amphibians invading marine waters, such as a Black Sea invasion by the natural hybrid Pelophylax esculentus reported in 2010.[174]
Marine fish
Fish anatomy includes a two-chambered heart, operculum, swim bladder, scales, eyes adapted to seeing underwater, and secretory cells that produce mucous. Fish breathe by extracting oxygen from water through gills. Fins propel and stabilize the fish in the water. Fish fall into two main groups: fish with bony skeletons and fish with cartilaginous skeletons.
A reported 32,700 species of fish have been described (as of December 2013),[175] more than the combined total of all other vertebrates. About 60% of fish species are saltwater fish.[176]
Marine tetrapods
Marine reptiles
Reptiles (Late Latin for creeping or crawling) form a class Reptilia of tetrapods, vertebrates that have either four limbs or have four-limbed ancestors. Some reptiles are more closely related to birds than other reptiles, and many scientists prefer to make Reptilia a monophyletic group which includes the birds.[177][178][179][180] Extant non-avian reptiles which inhabit or frequent the sea include sea turtles, sea snakes, terrapins, the marine iguana, and the saltwater crocodile. Currently, of the approximately 12,000 extant reptile species and sub-species, only about 100 of are classed as marine reptiles.[124]
Unlike amphibians, reptiles do not have an aquatic larval stage. Most reptiles are oviparous, although several species of squamates are viviparous, as were some extinct aquatic clades[181] — the fetus develops within the mother, contained in a placenta rather than an eggshell. As amniotes, reptile eggs are surrounded by membranes for protection and transport, which adapt them to reproduction on dry land. Many of the viviparous species feed their fetuses through various forms of placenta analogous to those of mammals, with some providing initial care for their hatchlings.
Most extant marine reptiles, except for some sea snakes, are oviparous and need to return to land to lay their eggs. Thus most species, excepting sea turtles, spend most of their lives on or near land rather than in the ocean. Despite their marine adaptations, most sea snakes prefer shallow waters nearby land, around islands, especially waters that are somewhat sheltered, as well as near estuaries.[182][183] Some extinct marine reptiles, such as ichthyosaurs, evolved to be viviparous and had no requirement to return to land.
The earliest marine reptiles arose in the Permian. During the Mesozoic many groups of reptiles became adapted to life in the seas, including ichthyosaurs, plesiosaurs, mosasaurs, nothosaurs, placodonts, sea turtles, thalattosaurs and thalattosuchians. Marine reptiles were less numerous after mass extinction at the end of the Cretaceous.
Marine birds
Birds adapted to living in the marine environment are often called seabirds. Examples include albatross, penguins, gannets, and auks. Although they spend most of their lives in the ocean, species such as gulls can often be found thousands of miles inland.
Marine mammals

There are five main types of marine mammals.
- Cetaceans include toothed whales (suborder Odontoceti), such as the sperm whale, dolphins, and porpoises such as the Dall's porpoise. Cetaceans also include baleen whales (suborder Mysticeti), such as the gray whale, humpback whale, and blue whale.
- Sirenians include manatees, the dugong, and the extinct Steller's sea cow.
- Seals (family Phocidae), sea lions (family Otariidae - which also include the fur seals), and the walrus (family Odobenidae) are all considered pinnipeds.
- The sea otter is a member of the family Mustelidae, which includes weasels and badgers.
- The polar bear is a member of the family Ursidae.
Plankton

Plankton are a diverse group of organisms that live in the water column of large bodies of water and that cannot swim against a current.[184] They provide a crucial source of food to many large aquatic organisms, such as fish and whales.
These organisms include drifting or floating bacteria, archaea, algae, protozoa and animals that inhabit, for example, the pelagic zone of oceans, seas, or bodies of fresh water. Essentially, plankton are defined by their ecological niche rather than any phylogenetic or taxonomic classification.
Though many planktonic species are microscopic in size, plankton includes organisms covering a wide range of sizes, including large organisms such as jellyfish.[185]
The role of phytoplankton is better understood due to their critical position as the most numerous primary producers on Earth. Phytoplankton are categorized into cyanobacteria (also called blue-green algae/bacteria), various types of algae (red, green, brown, and yellow-green), diatoms, dinoflagellates, euglenoids, coccolithophorids, cryptomonads, chrysophytes, chlorophytes, prasinophytes, and silicoflagellates.
Zooplankton tend to be somewhat larger, and not all are microscopic. Many Protozoa are zooplankton, including dinoflagellates, zooflagellates, foraminiferans, and radiolarians. Some of these (such as dinoflagellates) are also phytoplankton; the distinction between plants and animals often breaks down in very small organisms. Other zooplankton include cnidarians, ctenophores, chaetognaths, molluscs, arthropods, urochordates, and annelids such as polychaetes. Many larger animals begin their life as zooplankton before they become large enough to take their familiar forms. Two examples are fish larvae and sea stars (also called starfish).
Interactions
With freshwater
With land
Extinctions and threats
More than 99 percent of all species, amounting to over five billion species,[186] that ever lived on Earth are estimated to be extinct.[187][188] Extinction events occur when life undergoes precipitous global declines. Most diversity and biomass on earth is found among the microorganisms, which are difficult to measure. Recorded extinction events are therefore based on the more easily observed changes in the diversity and abundance of larger multicellular organisms, rather than the total diversity and abundance of life.[189]
Extinction occurs at an uneven rate. Based on the fossil record, the background rate of extinctions on Earth is about two to five taxonomic families of marine animals every million years. Marine fossils are mostly used to measure extinction rates because of their superior fossil record and stratigraphic range compared to land organisms.
The Great Oxygenation Event was perhaps the first major extinction event. Since the Cambrian explosion five further major mass extinctions have significantly exceeded the background extinction rate.[190] In addition to these major mass extinctions there are numerous minor ones, as well as the current ongoing mass-extinction caused by human activity, the Holocene extinction sometimes called the "sixth extinction".
As a field of study

During the sixth century BC, the Greek philosopher Xenophanes (570-475 BC) recognised that some fossil shells were remains of shellfish. He used this to argue that what was at the time dry land was once under the sea.[191] This was an important step in advancing from simply stating an idea to backing it with evidence and observation.[192]
Later, during the fourth century BC, another Greek philosopher Aristotle (384–322 BC) attempted a comprehensive classification of animals which included systematic descriptions of many marine species,[193][194] and particularly species found in the Mediterranean Sea.[195] These pioneering works include History of Animals, a general biology of animals, Parts of Animals, a comparative anatomy and physiology of animals, and Generation of Animals, on developmental biology. The most striking passages are about the sea-life visible from observation on Lesbos and available from the catches of fishermen. His observations on catfish, electric fish (Torpedo) and angler-fish are detailed, as is his writing on cephalopods, namely, Octopus, Sepia (cuttlefish) and the paper nautilus (Argonauta argo). His description of the hectocotyl arm, used in sexual reproduction, was widely disbelieved until its rediscovery in the 19th century. He separated aquatic mammals from fish, and knew that sharks and rays were part of a group he called Selachē (selachians).[196] He gave accurate descriptions of the ovoviviparous embryological development of the hound shark Mustelus mustelus.[197] His classification of living things contains elements which were still in use in the 19th century. What the modern zoologist would call vertebrates and invertebrates, Aristotle called "animals with blood" and "animals without blood" (he did not know that complex invertebrates do make use of hemoglobin, but of a different kind from vertebrates). He divided animals with blood into live-bearing (mammals), and egg-bearing (birds and fish). Invertebrates ("animals without blood") he divided into insects, crustacea (further divided into non-shelled – cephalopods – and shelled) and testacea (molluscs).[198][199]
In contemporary times, marine life is a field of study both in marine biology and in biological oceanography. In biology many phyla, families and genera have some species that live in the sea and others that live on land. Marine biology classifies species based on the environment rather than on taxonomy. For this reason marine biology encompasses not only organisms that live only in a marine environment, but also other organisms whose lives revolve around the sea. Biological oceanography is the study of how organisms affect and are affected by the physics, chemistry, and geology of the oceanographic system. Biological oceanography mostly focuses on the microorganisms within the ocean; looking at how they are affected by their environment and how that affects larger marine creatures and their ecosystem.[200] Biological oceanography is similar to marine biology, but is different because of the perspective used to study the ocean. Biological oceanography takes a bottom up approach (in terms of the food web), while marine biology studies the ocean from a top down perspective. Biological oceanography mainly focuses on the ecosystem of the ocean with an emphasis on plankton: their diversity (morphology, nutritional sources, motility, and metabolism); their productivity and how that plays a role in the global carbon cycle; and their distribution (predation and life cycle).[200][201][202] Biological oceanography also investigates the role of microbes in food webs, and how humans impact the ecosystems in the oceans.[200]
See also
References
- ^ Drogin, Bob (August 2, 2009). "Mapping an ocean of species". Los Angeles Times. Retrieved August 18, 2009.
- ^ Agre, P. (2006) "The aquaporin water channels". Proceedings of the American Thoracic Society, 3 (1): 5–13.
- ^ Collins J. C. (1991) The Matrix of Life: A View of Natural Molecules from the Perspective of Environmental Water Molecular Presentations. ISBN 9780962971907.
- ^ "Age of the Earth". United States Geological Survey. July 9, 2007. Retrieved 2015-05-31.
- ^ Dalrymple 2001, pp. 205–221
- ^ Manhesa, Gérard; Allègre, Claude J.; Dupréa, Bernard; Hamelin, Bruno (May 1980). "Lead isotope study of basic-ultrabasic layered complexes: Speculations about the age of the earth and primitive mantle characteristics". Earth and Planetary Science Letters. 47 (3). Amsterdam, the Netherlands: Elsevier: 370–382. Bibcode:1980E&PSL..47..370M. doi:10.1016/0012-821X(80)90024-2. ISSN 0012-821X.
- ^ Schopf, J. William; Kudryavtsev, Anatoliy B.; Czaja, Andrew D.; Tripathi, Abhishek B. (October 5, 2007). "Evidence of Archean life: Stromatolites and microfossils". Precambrian Research. 158 (3–4). Amsterdam, the Netherlands: Elsevier: 141–155. doi:10.1016/j.precamres.2007.04.009. ISSN 0301-9268.
- ^ Raven & Johnson 2002, p. 68
- ^ Borenstein, Seth (November 13, 2013). "Oldest fossil found: Meet your microbial mom". Excite. Yonkers, NY: Mindspark Interactive Network. Associated Press. Retrieved 2015-05-31.
- ^ Pearlman, Jonathan (November 13, 2013). "'Oldest signs of life on Earth found'". The Daily Telegraph. London: Telegraph Media Group. Retrieved 2014-12-15.
- ^ Noffke, Nora; Christian, Daniel; Wacey, David; Hazen, Robert M. (November 16, 2013). "Microbially Induced Sedimentary Structures Recording an Ancient Ecosystem in the ca. 3.48 Billion-Year-Old Dresser Formation, Pilbara, Western Australia". Astrobiology. 13 (12). New Rochelle, NY: Mary Ann Liebert, Inc.: 1103–1124. Bibcode:2013AsBio..13.1103N. doi:10.1089/ast.2013.1030. ISSN 1531-1074. PMC 3870916. PMID 24205812.
- ^ Ohtomo, Yoko; Kakegawa, Takeshi; Ishida, Akizumi; et al. (January 2014). "Evidence for biogenic graphite in early Archaean Isua metasedimentary rocks". Nature Geoscience. 7 (1). London: Nature Publishing Group: 25–28. Bibcode:2014NatGe...7...25O. doi:10.1038/ngeo2025. ISSN 1752-0894.
- ^ a b Borenstein, Seth (October 19, 2015). "Hints of life on what was thought to be desolate early Earth". Excite. Yonkers, NY: Mindspark Interactive Network. Associated Press. Retrieved 2015-10-20.
- ^ Bell, Elizabeth A.; Boehnike, Patrick; Harrison, T. Mark; et al. (November 24, 2015). "Potentially biogenic carbon preserved in a 4.1 billion-year-old zircon" (PDF). Proc. Natl. Acad. Sci. U.S.A. 112 (47). Washington, D.C.: National Academy of Sciences: 14518–14521. doi:10.1073/pnas.1517557112. ISSN 0027-8424. PMC 4664351. PMID 26483481. Retrieved 2015-12-30.
- ^ Penny, David; Poole, Anthony (December 1999). "The nature of the last universal common ancestor". Current Opinion in Genetics & Development. 9 (6). Amsterdam, the Netherlands: Elsevier: 672–677. doi:10.1016/S0959-437X(99)00020-9. ISSN 0959-437X. PMID 10607605.
- ^ Theobald, Douglas L. (May 13, 2010). "A formal test of the theory of universal common ancestry". Nature. 465 (7295). London: Nature Publishing Group: 219–222. Bibcode:2010Natur.465..219T. doi:10.1038/nature09014. ISSN 0028-0836. PMID 20463738.
- ^ Doolittle, W. Ford (February 2000). "Uprooting the Tree of Life" (PDF). Scientific American. 282 (2). Stuttgart: Georg von Holtzbrinck Publishing Group: 90–95. doi:10.1038/scientificamerican0200-90. ISSN 0036-8733. PMID 10710791. Archived from the original (PDF) on 2006-09-07. Retrieved 2015-04-05.
- ^ Peretó, Juli (March 2005). "Controversies on the origin of life" (PDF). International Microbiology. 8 (1). Barcelona: Spanish Society for Microbiology: 23–31. ISSN 1139-6709. PMID 15906258.
- ^ Joyce, Gerald F. (July 11, 2002). "The antiquity of RNA-based evolution". Nature. 418 (6894). London: Nature Publishing Group: 214–221. Bibcode:2002Natur.418..214J. doi:10.1038/418214a. ISSN 0028-0836. PMID 12110897.
- ^ Trevors, Jack T.; Psenner, Roland (December 2001). "From self-assembly of life to present-day bacteria: a possible role for nanocells". FEMS Microbiology Reviews. 25 (5). Amsterdam, the Netherlands: Elsevier on behalf of the Federation of European Microbiological Societies: 573–582. doi:10.1111/j.1574-6976.2001.tb00592.x. ISSN 1574-6976. PMID 11742692.
- ^ Bapteste, Eric; Walsh, David A. (June 2005). "Does the 'Ring of Life' ring true?". Trends in Microbiology. 13 (6). Cambridge, MA: Cell Press: 256–261. doi:10.1016/j.tim.2005.03.012. ISSN 0966-842X. PMID 15936656.
- ^ Darwin 1859, p. 1
- ^ Doolittle, W. Ford; Bapteste, Eric (February 13, 2007). "Pattern pluralism and the Tree of Life hypothesis". Proc. Natl. Acad. Sci. U.S.A. 104 (7). Washington, D.C.: National Academy of Sciences: 2043–2049. Bibcode:2007PNAS..104.2043D. doi:10.1073/pnas.0610699104. ISSN 0027-8424. PMC 1892968. PMID 17261804.
- ^ Kunin, Victor; Goldovsky, Leon; Darzentas, Nikos; Ouzounis, Christos A. (July 2005). "The net of life: Reconstructing the microbial phylogenetic network". Genome Research. 15 (7). Cold Spring Harbor, NY: Cold Spring Harbor Laboratory Press: 954–959. doi:10.1101/gr.3666505. ISSN 1088-9051. PMC 1172039. PMID 15965028.
- ^ Jablonski, David (June 25, 1999). "The Future of the Fossil Record". Science. 284 (5423). Washington, D.C.: American Association for the Advancement of Science: 2114–2116. doi:10.1126/science.284.5423.2114. ISSN 0036-8075. PMID 10381868.
- ^ Mason, Stephen F. (September 6, 1984). "Origins of biomolecular handedness". Nature. 311 (5981). London: Nature Publishing Group: 19–23. Bibcode:1984Natur.311...19M. doi:10.1038/311019a0. ISSN 0028-0836. PMID 6472461.
- ^ Wolf, Yuri I.; Rogozin, Igor B.; Grishin, Nick V.; Koonin, Eugene V. (September 1, 2002). "Genome trees and the tree of life". Trends in Genetics. 18 (9). Cambridge, MA: Cell Press: 472–479. doi:10.1016/S0168-9525(02)02744-0. ISSN 0168-9525. PMID 12175808.
- ^ Varki, Ajit; Altheide, Tasha K. (December 2005). "Comparing the human and chimpanzee genomes: searching for needles in a haystack". Genome Research. 15 (12). Cold Spring Harbor, NY: Cold Spring Harbor Laboratory Press: 1746–1758. doi:10.1101/gr.3737405. ISSN 1088-9051. PMID 16339373.
- ^ Ciccarelli, Francesca D.; Doerks, Tobias; von Mering, Christian; et al. (March 3, 2006). "Toward Automatic Reconstruction of a Highly Resolved Tree of Life". Science. 311 (5765). Washington, D.C.: American Association for the Advancement of Science: 1283–1287. Bibcode:2006Sci...311.1283C. doi:10.1126/science.1123061. ISSN 0036-8075. PMID 16513982.
- ^ a b Cavalier-Smith, Thomas (June 29, 2006). "Cell evolution and Earth history: stasis and revolution". Philosophical Transactions of the Royal Society B: Biological Sciences. 361 (1470). London: Royal Society: 969–1006. doi:10.1098/rstb.2006.1842. ISSN 0962-8436. PMC 1578732. PMID 16754610.
- ^ Schopf, J. William (June 29, 2006). "Fossil evidence of Archaean life". Philosophical Transactions of the Royal Society B: Biological Sciences. 361 (1470). London: Royal Society: 869–885. doi:10.1098/rstb.2006.1834. ISSN 0962-8436. PMC 1578735. PMID 16754604.
- Altermann, Wladyslaw; Kazmierczak, Józef (November 2003). "Archean microfossils: a reappraisal of early life on Earth". Research in Microbiology. 154 (9). Amsterdam, the Netherlands: Elsevier for the Pasteur Institute: 611–617. doi:10.1016/j.resmic.2003.08.006. ISSN 0923-2508. PMID 14596897.
- ^ Schopf, J. William (July 19, 1994). "Disparate rates, differing fates: tempo and mode of evolution changed from the Precambrian to the Phanerozoic". Proc. Natl. Acad. Sci. U.S.A. 91 (15). Washington, D.C.: National Academy of Sciences: 6735–6742. Bibcode:1994PNAS...91.6735S. doi:10.1073/pnas.91.15.6735. ISSN 0027-8424. PMC 44277. PMID 8041691.
- ^ Poole, Anthony M.; Penny, David (January 2007). "Evaluating hypotheses for the origin of eukaryotes". BioEssays. 29 (1). Hoboken, NJ: John Wiley & Sons: 74–84. doi:10.1002/bies.20516. ISSN 0265-9247. PMID 17187354.
- ^ a b Dyall, Sabrina D.; Brown, Mark T.; Johnson, Patricia J. (April 9, 2004). "Ancient Invasions: From Endosymbionts to Organelles". Science. 304 (5668). Washington, D.C.: American Association for the Advancement of Science: 253–257. Bibcode:2004Sci...304..253D. doi:10.1126/science.1094884. ISSN 0036-8075. PMID 15073369.
- ^ Martin, William (October 2005). "The missing link between hydrogenosomes and mitochondria". Trends in Microbiology. 13 (10). Cambridge, MA: Cell Press: 457–459. doi:10.1016/j.tim.2005.08.005. ISSN 0966-842X. PMID 16109488.
- ^ Lang, B. Franz; Gray, Michael W.; Burger, Gertraud (December 1999). "Mitochondrial genome evolution and the origin of eukaryotes". Annual Review of Genetics. 33. Palo Alto, CA: Annual Reviews: 351–397. doi:10.1146/annurev.genet.33.1.351. ISSN 0066-4197. PMID 10690412.
- ^ DeLong, Edward F.; Pace, Norman R. (August 1, 2001). "Environmental Diversity of Bacteria and Archaea". Systematic Biology. 50 (4). Oxford: Oxford University Press on behalf of the Society of Systematic Biologists: 470–478. doi:10.1080/106351501750435040. ISSN 1063-5157. PMID 12116647.
- ^ Kaiser, Dale (December 2001). "Building a multicellular organism". Annual Review of Genetics. 35. Palo Alto, CA: Annual Reviews: 103–123. doi:10.1146/annurev.genet.35.102401.090145. ISSN 0066-4197. PMID 11700279.
- ^ Zimmer, Carl (January 7, 2016). "Genetic Flip Helped Organisms Go From One Cell to Many". The New York Times. Retrieved January 7, 2016.
- ^ Valentine, James W.; Jablonski, David; Erwin, Douglas H. (March 1, 1999). "Fossils, molecules and embryos: new perspectives on the Cambrian explosion". Development. 126 (5). Cambridge: The Company of Biologists: 851–859. ISSN 0950-1991. PMID 9927587. Retrieved 2014-12-30.
- ^ Ohno, Susumu (January 1997). "The reason for as well as the consequence of the Cambrian explosion in animal evolution". Journal of Molecular Evolution. 44 (Suppl. 1). New York: Springer-Verlag New York: S23–S27. doi:10.1007/PL00000055. ISSN 0022-2844. PMID 9071008.
- Valentine, James W.; Jablonski, David (2003). "Morphological and developmental macroevolution: a paleontological perspective". The International Journal of Developmental Biology. 47 (7–8). Bilbao, Spain: University of the Basque Country Press: 517–522. ISSN 0214-6282. PMID 14756327. Retrieved 2014-12-30.
- ^ Waters, Elizabeth R. (December 2003). "Molecular adaptation and the origin of land plants". Molecular Phylogenetics and Evolution. 29 (3). Academic Press: 456–463. doi:10.1016/j.ympev.2003.07.018. ISSN 1055-7903. PMID 14615186.
- ^ Mayhew, Peter J. (August 2007). "Why are there so many insect species? Perspectives from fossils and phylogenies". Biological Reviews. 82 (3). Cambridge: Cambridge University Press on behalf of the Cambridge Philosophical Society: 425–454. doi:10.1111/j.1469-185X.2007.00018.x. ISSN 1464-7931. PMID 17624962.
- ^ Carroll, Robert L. (May 2007). "The Palaeozoic Ancestry of Salamanders, Frogs and Caecilians". Zoological Journal of the Linnean Society. 150 (Supplement s1). Hoboken, NJ: Wiley-Blackwell: 1–140. doi:10.1111/j.1096-3642.2007.00246.x. ISSN 1096-3642. PMID 12752770.
- ^ Wible, John R.; Rougier, Guillermo W.; Novacek, Michael J.; Asher, Robert J. (June 21, 2007). "Cretaceous eutherians and Laurasian origin for placental mammals near the K/T boundary". Nature. 447 (7147). London: Nature Publishing Group: 1003–1006. Bibcode:2007Natur.447.1003W. doi:10.1038/nature05854. ISSN 0028-0836. PMID 17581585.
- ^ Witmer, Lawrence M. (July 28, 2011). "Palaeontology: An icon knocked from its perch". Nature. 475 (7357). London: Nature Publishing Group: 458–459. doi:10.1038/475458a. ISSN 0028-0836. PMID 21796198.
- ^ Schloss, Patrick D.; Handelsman, Jo (December 2004). "Status of the Microbial Census". Microbiology and Molecular Biology Reviews. 68 (4). Washington, D.C.: American Society for Microbiology: 686–691. doi:10.1128/MMBR.68.4.686-691.2004. ISSN 1092-2172. PMC 539005. PMID 15590780.
- ^ Miller & Spoolman 2012, p. 62
- ^ Mora, Camilo; Tittensor, Derek P.; Adl, Sina; et al. (August 23, 2011). "How Many Species Are There on Earth and in the Ocean?". PLOS Biology. 9 (8). San Francisco, CA: Public Library of Science: e1001127. doi:10.1371/journal.pbio.1001127. ISSN 1545-7885. PMC 3160336. PMID 21886479.
{{cite journal}}
: CS1 maint: unflagged free DOI (link) - ^ Madigan M; Martinko J, eds. (2006). Brock Biology of Microorganisms (13th ed.). Pearson Education. p. 1096. ISBN 0-321-73551-X.
- ^ Rybicki EP (1990). "The classification of organisms at the edge of life, or problems with virus systematics". S Aft J Sci. 86: 182–6. ISSN 0038-2353.
- ^ Lwoff A (1956). "The concept of virus". J. Gen. Microbiol. 17 (2): 239–53. doi:10.1099/00221287-17-2-239. PMID 13481308.
- ^ Wade, Nicholas (25 July 2016). "Meet Luca, the Ancestor of All Living Things". New York Times. Retrieved 25 July 2016.
- ^ University of Georgia (25 August 1998). "First-Ever Scientific Estimate Of Total Bacteria On Earth Shows Far Greater Numbers Than Ever Known Before". Science Daily. Retrieved 10 November 2014.
- ^ Dose, K; Bieger-Dose, A; Dillmann, R; Gill, M; Kerz, O; Klein, A; Meinert, H; Nawroth, T; Risi, S; Stridde, C (1995). "ERA-experiment "space biochemistry"". Advances in Space Research. 16 (8): 119–129. doi:10.1016/0273-1177(95)00280-R. PMID 11542696.
- ^ Vaisberg, Horneck G; Eschweiler U; Reitz G; Wehner J; Willimek R; Strauch K (1995). "Biological responses to space: results of the experiment "Exobiological Unit" of ERA on EURECA I". Adv Space Res. 16 (8): 105–18. Bibcode:1995AdSpR..16..105V. doi:10.1016/0273-1177(95)00279-N. PMID 11542695.
- ^ Staff (2012). "The JTM Effect". jtmnutrients.com. Archived from the original on 2012-11-14. Retrieved 10 November 2014.
- ^ Staff (2014). "The Biosphere". Aspen Global Change Institute. Retrieved 10 November 2014.
- ^ a b c Choi, Charles Q. (17 March 2013). "Microbes Thrive in Deepest Spot on Earth". LiveScience. Retrieved 17 March 2013.
- ^ Glud, Ronnie; Wenzhöfer, Frank; Middelboe, Mathias; Oguri, Kazumasa; Turnewitsch, Robert; Canfield, Donald E.; Kitazato, Hiroshi (17 March 2013). "High rates of microbial carbon turnover in sediments in the deepest oceanic trench on Earth". Nature Geoscience. 6 (4): 284–288. Bibcode:2013NatGe...6..284G. doi:10.1038/ngeo1773. Retrieved 17 March 2013.
- ^ Oskin, Becky (14 March 2013). "Intraterrestrials: Life Thrives in Ocean Floor". LiveScience. Retrieved 17 March 2013.
- ^ Morelle, Rebecca (15 December 2014). "Microbes discovered by deepest marine drill analysed". BBC News. Retrieved 15 December 2014.
- ^ Fox, Douglas (20 August 2014). "Lakes under the ice: Antarctica's secret garden". Nature. 512 (7514): 244–246. Bibcode:2014Natur.512..244F. doi:10.1038/512244a. Retrieved 21 August 2014.
- ^ Mack, Eric (20 August 2014). "Life Confirmed Under Antarctic Ice; Is Space Next?". Forbes. Retrieved 21 August 2014.
- ^ 2002 WHO mortality data Accessed 20 January 2007
- ^ "Functions of global ocean microbiome key to understanding environmental changes". www.sciencedaily.com. University of Georgia. December 10, 2015. Retrieved December 11, 2015.
- ^ Suttle, C.A. (2005). "Viruses in the Sea". Nature. 437 (9): 356–361. doi:10.1038/nature04160. PMID 16163346.
- ^ a b Shors p. 4
- ^ a b Shors p. 5
- ^ a b Shors p. 593
- ^ a b c d e Suttle CA. Marine viruses—major players in the global ecosystem. Nature Reviews Microbiology. 2007;5(10):801–12. doi:10.1038/nrmicro1750. PMID 17853907.
- ^ a b c Mann, NH (2005-05-17). "The Third Age of Phage". PloS Biol. 3 (5). United States: Public Library of Science: 753–755. doi:10.1371/journal.pbio.0030182. PMC 1110918. PMID 15884981.
{{cite journal}}
: CS1 maint: unflagged free DOI (link) - ^ Wommack, K. Eric; Russell T. Hill; Terri A. Muller; Rita R. Colwell (April 1996). "Effects of sunlight on bacteriophage viability and structure". Applied and Environmental Microbiology. 62 (4). United States of America: American Society for Microbiology: 1336–1341. PMC 167899. PMID 8919794.
- ^ Krupovic M, Bamford DH (2007). "Putative prophages related to lytic tailless marine dsDNA phage PM2 are widespread in the genomes of aquatic bacteria". BMC Genomics. 8: 236. doi:10.1186/1471-2164-8-236. PMC 1950889. PMID 17634101.
{{cite journal}}
: CS1 maint: unflagged free DOI (link) - ^ Xue H, Xu Y, Boucher Y, Polz MF (2012). "High frequency of a novel filamentous phage, VCY φ, within an environmental Vibrio cholerae population". Appl Environ Microbiol. 78 (1): 28–33. doi:10.1128/AEM.06297-11. PMC 3255608. PMID 22020507.
- ^ a b Roux S, Krupovic M, Poulet A, Debroas D, Enault F (2012). "Evolution and diversity of the Microviridae viral family through a collection of 81 new complete genomes assembled from virome reads". PLOS ONE. 7 (7): e40418. doi:10.1371/journal.pone.0040418. PMC 3394797. PMID 22808158.
{{cite journal}}
: CS1 maint: unflagged free DOI (link) - ^ Kellogg, CA; JB Rose; SC Jiang; and JM Thurmond; JH Paul (1995). "Genetic diversity of related vibriophages isolated from marine environments around Florida and Hawaii, USA". Marine Ecology Progress Series. 120 (1–3). Germany: Inter-Research Science Center: 89–98. doi:10.3354/meps120089.
- ^ a b Suttle CA. Viruses in the sea. Nature. 2005;437(7057):356–61. doi:10.1038/nature04160. PMID 16163346. Bibcode:2005Natur.437..356S.
- ^ a b www.cdc.gov. Harmful Algal Blooms: Red Tide: Home [Retrieved 2014-12-19].
- ^ Koonin EV, Senkevich TG, Dolja VV. The ancient Virus World and evolution of cells. Biol. Direct. 2006;1:29. doi:10.1186/1745-6150-1-29. PMID 16984643.
- ^ a b Canchaya C, Fournous G, Chibani-Chennoufi S, Dillmann ML, Brüssow H. Phage as agents of lateral gene transfer. Current Opinion in Microbiology. 2003;6(4):417–24. doi:10.1016/S1369-5274(03)00086-9. PMID 12941415.
- ^ a b Rybicki, EP. The classification of organisms at the edge of life, or problems with virus systematics. S Afr J Sci. 1990;86:182–186.
- ^ Koonin, E. V.; Starokadomskyy, P. (7 March 2016). "Are viruses alive? The replicator paradigm sheds decisive light on an old but misguided question". Stud Hist Philos Biol Biomed Sci. doi:10.1016/j.shpsc.2016.02.016. PMID 26965225.
- ^ Iyer LM, Balaji S, Koonin EV, Aravind L. Evolutionary genomics of nucleo-cytoplasmic large DNA viruses. Virus Res.. 2006;117(1):156–84. doi:10.1016/j.virusres.2006.01.009. PMID 16494962.
- ^ Sanjuán R, Nebot MR, Chirico N, Mansky LM, Belshaw R. Viral mutation rates. Journal of Virology. 2010;84(19):9733–48. doi:10.1128/JVI.00694-10. PMID 20660197.
- ^ Mahy WJ & Van Regenmortel MHV (eds). Desk Encyclopedia of General Virology. Oxford: Academic Press; 2009. ISBN 0-12-375146-2. p. 28.
- ^ Are viruses alive? The replicator paradigm sheds decisive light on an old but misguided question. Studies in History and Philosophy of Biological and Biomedical Sciences. 2016. doi:10.1016/j.shpsc.2016.02.016. PMID 26965225.
- ^ Holmes EC. Viral evolution in the genomic age. PLoS Biol.. 2007;5(10):e278. doi:10.1371/journal.pbio.0050278. PMID 17914905.
- ^ Wimmer E, Mueller S, Tumpey TM, Taubenberger JK. Synthetic viruses: a new opportunity to understand and prevent viral disease. Nature Biotechnology. 2009;27(12):1163–72. doi:10.1038/nbt.1593. PMID 20010599.
- ^ Wommack KE, Colwell RR. Virioplankton: viruses in aquatic ecosystems. Microbiol. Mol. Biol. Rev.. 2000;64(1):69–114. doi:10.1128/MMBR.64.1.69-114.2000. PMID 10704475.
- ^ Bergh O, Børsheim KY, Bratbak G, Heldal M. High abundance of viruses found in aquatic environments. Nature. 1989;340(6233):467–8. doi:10.1038/340467a0. PMID 2755508. Bibcode:1989Natur.340..467B.
- ^ Lawrence CM, Menon S, Eilers BJ, et al.. Structural and functional studies of archaeal viruses. J. Biol. Chem.. 2009;284(19):12599–603. doi:10.1074/jbc.R800078200. PMID 19158076.
- ^ Prangishvili D, Forterre P, Garrett RA. Viruses of the Archaea: a unifying view. Nature Reviews Microbiology. 2006;4(11):837–48. doi:10.1038/nrmicro1527. PMID 17041631.
- ^ Prangishvili D, Garrett RA. Exceptionally diverse morphotypes and genomes of crenarchaeal hyperthermophilic viruses. Biochem. Soc. Trans.. 2004;32(Pt 2):204–8. doi:10.1042/BST0320204. PMID 15046572.
- ^ Forterre P, Philippe H. The last universal common ancestor (LUCA), simple or complex?. The Biological Bulletin. 1999;196(3):373–5; discussion 375–7. doi:10.2307/1542973. PMID 11536914.
- ^ DeLong, E.F. and Beja, O., 2010) "The light-driven proton pump proteorhodopsin enhances bacterial survival during tough times". PLoS Biol, 8 (4): p.e1000359. doi:10.1371/journal.pbio.1000359
- ^ Fredrickson JK, Zachara JM, Balkwill DL, Kennedy D, Li SM, Kostandarithes HM, Daly MJ, Romine MF, Brockman FJ (2004). "Geomicrobiology of high-level nuclear waste-contaminated vadose sediments at the Hanford site, Washington state". Applied and Environmental Microbiology. 70 (7): 4230–41. doi:10.1128/AEM.70.7.4230-4241.2004. PMC 444790. PMID 15240306.
- ^ Woese CR, Kandler O, Wheelis ML (1990). "Towards a natural system of organisms: proposal for the domains Archaea, Bacteria, and Eucarya". Proceedings of the National Academy of Sciences of the United States of America. 87 (12): 4576–9. Bibcode:1990PNAS...87.4576W. doi:10.1073/pnas.87.12.4576. PMC 54159. PMID 2112744.
- ^ Schopf JW (1994). "Disparate rates, differing fates: tempo and mode of evolution changed from the Precambrian to the Phanerozoic". Proceedings of the National Academy of Sciences of the United States of America. 91 (15): 6735–42. Bibcode:1994PNAS...91.6735S. doi:10.1073/pnas.91.15.6735. PMC 44277. PMID 8041691.
- ^ DeLong EF, Pace NR (2001). "Environmental diversity of bacteria and archaea". Syst Biol. 50 (4): 470–8. doi:10.1080/106351501750435040. PMID 12116647.
- ^ Brown JR, Doolittle WF (1997). "Archaea and the prokaryote-to-eukaryote transition". Microbiology and Molecular Biology Reviews. 61 (4): 456–502. PMC 232621. PMID 9409149.
- ^ Poole AM, Penny D (2007). "Evaluating hypotheses for the origin of eukaryotes". BioEssays. 29 (1): 74–84. doi:10.1002/bies.20516. PMID 17187354.
- ^ Lang BF, Gray MW, Burger G (1999). "Mitochondrial genome evolution and the origin of eukaryotes". Annu Rev Genet. 33: 351–97. doi:10.1146/annurev.genet.33.1.351. PMID 10690412.
- ^ McFadden GI (1999). "Endosymbiosis and evolution of the plant cell". Current Opinion in Plant Biology. 2 (6): 513–9. doi:10.1016/S1369-5266(99)00025-4. PMID 10607659.
- ^ Béja, O., Aravind, L., Koonin, E.V., Suzuki, M.T., Hadd, A., Nguyen, L.P., Jovanovich, S.B., Gates, C.M., Feldman, R.A., Spudich, J.L. and Spudich, E.N. (2000) "Bacterial rhodopsin: evidence for a new type of phototrophy in the sea". Science, 289 (5486): 1902-1906. doi:10.1126/science.289.5486.1902
- ^ "Interviews with Fellows: Ed Delong". American Academy of Microbiology. Retrieved 2 July 2016.
- ^ Bacteria with Batteries, Popular Science magazine, January 2001, Page 55.
- ^ Pace NR (May 2006). "Time for a change". Nature. 441 (7091): 289. Bibcode:2006Natur.441..289P. doi:10.1038/441289a. PMID 16710401.
- ^ Stoeckenius W (1 October 1981). "Walsby's square bacterium: fine structure of an orthogonal procaryote". J. Bacteriol. 148 (1): 352–60. PMC 216199. PMID 7287626.
- ^ Bang C, Schmitz RA (2015). "Archaea associated with human surfaces: not to be underestimated". FEMS Microbiology Reviews. 39 (5): 631–48. doi:10.1093/femsre/fuv010. PMID 25907112.
- ^ Hyde, K.D.; E.B.J. Jones; E. Leaño; S.B. Pointing; A.D. Poonyth; L.L.P. Vrijmoed (1998). "Role of fungi in marine ecosystems". Biodiversity and Conservation. 7 (9): 1147–1161. doi:10.1023/A:1008823515157.
- ^ Kirk, P.M., Cannon, P.F., Minter, D.W. and Stalpers, J. "Dictionary of the Fungi". Edn 10. CABI, 2008
- ^ Hyde, K.D.; E.B.J. Jones (1989). "Spore attachment in marine fungi". Botanica Marina. 32: 205–218. doi:10.1515/botm.1989.32.3.205.
- ^ San-Martín, A.; S. Orejanera; C. Gallardo; M. Silva; J. Becerra; R. Reinoso; M.C. Chamy; K. Vergara; J. Rovirosa (2008). "Steroids from the marine fungus Geotrichum sp". Journal of the Chilean Chemical Society. 53 (1): 1377–1378.
- ^ Jones, E.B.G., Hyde, K.D., & Pang, K.-L., eds. (2014). Freshwater fungi: and fungal-like organisms. Berlin/Boston: De Gruyter.
- ^ Jones, E.B.G.; Pang, K.-L., eds. (2012). Marine Fungi, and Fungal-like Organisms. Marine and Freshwater Botany. Berlin, Boston: De Gruyter (published August 2012). ISBN 978-3-11-026406-7. Retrieved September 3, 2015.
{{cite book}}
: Cite has empty unknown parameter:|deadurl=
(help) - ^ "Distribution and Diversity of Planktonic Fungi in the West Pacific Warm Pool". doi:10.1371/journal.pone.0101523.s001.
{{cite journal}}
: Cite journal requires|journal=
(help)CS1 maint: unflagged free DOI (link) - ^ Wang, G.; Wang, X.; Liu, X.; Li, Q. (2012). "Diversity and biogeochemical function of planktonic fungi in the ocean". In Raghukumar, C. (ed.). Biology of marine fungi. Berlin, Heidelberg: Springer-Verlag. pp. 71–88. doi:10.1007/978-3-642-23342-5. ISBN 978-3-642-23341-8. Retrieved September 3, 2015.
- ^ Damare, Samir; Raghukumar, Chandralata (2007-11-11). "Fungi and Macroaggregation in Deep-Sea Sediments". Microbial Ecology. 56 (1): 168–177. doi:10.1007/s00248-007-9334-y. ISSN 0095-3628.
- ^ Kubanek, Julia; Jensen, Paul R.; Keifer, Paul A.; Sullards, M. Cameron; Collins, Dwight O.; Fenical, William (2003-06-10). "Seaweed resistance to microbial attack: A targeted chemical defense against marine fungi". Proceedings of the National Academy of Sciences. 100 (12): 6916–6921. doi:10.1073/pnas.1131855100. ISSN 0027-8424. PMC 165804. PMID 12756301.
- ^ a b Gao, Zheng; Johnson, Zackary I.; Wang, Guangyi (2009-07-30). "Molecular characterization of the spatial diversity and novel lineages of mycoplankton in Hawaiian coastal waters". The ISME Journal. 4 (1): 111–120. doi:10.1038/ismej.2009.87. ISSN 1751-7362.
- ^ Panzer, Katrin; Yilmaz, Pelin; Weiß, Michael; Reich, Lothar; Richter, Michael; Wiese, Jutta; Schmaljohann, Rolf; Labes, Antje; Imhoff, Johannes F. (2015-07-30). "Identification of Habitat-Specific Biomes of Aquatic Fungal Communities Using a Comprehensive Nearly Full-Length 18S rRNA Dataset Enriched with Contextual Data". PLoS ONE. 10 (7): e0134377. doi:10.1371/journal.pone.0134377. PMC 4520555. PMID 26226014.
{{cite journal}}
: CS1 maint: unflagged free DOI (link) - ^ GUTIERREZ, Marcelo H; PANTOJA, Silvio; QUINONES, Renato A and GONZALEZ, Rodrigo R. First record of flamentous fungi in the coastal upwelling ecosystem off central Chile. Gayana (Concepc.) [online]. 2010, vol.74, n.1, pp. 66-73. ISSN 0717-6538.
- ^ a b c Sridhar, K.R. (2009). "10. Aquatic fungi – Are they planktonic?". Plankton Dynamics of Indian Waters. Jaipur, India: Pratiksha Publications. pp. 133–148. Cite error: The named reference ":0" was defined multiple times with different content (see the help page).
- ^ Species of Higher Marine Fungi University of Mississippi. Retrieved 2012-02-05.
- ^ Freshwater and marine lichen-forming fungi Retrieved 2012-02-06.
- ^ Yuan X, Xiao S; Taylor TN. (2005). "Lichen-Like Symbiosis 600 Million Years Ago". Science. 308: 1017–1020. doi:10.1126/science.1111347. PMID 15890881.
{{cite journal}}
: Unknown parameter|lastauthoramp=
ignored (|name-list-style=
suggested) (help) - ^ Jones, E. B. Gareth; Pang, Ka-Lai (2012-08-31). Marine Fungi: and Fungal-like Organisms. Walter de Gruyter. ISBN 9783110264067.
- ^ a b Ponder, W.F.; Lindberg, D.R., eds. (2008). Phylogeny and Evolution of the Mollusca. Berkeley: University of California Press. p. 481. ISBN 978-0-520-25092-5.
- ^ Ruppert, Front endpaper 1
- ^ Vacelet & Duport 2004, pp. 179–190.
- ^ Zhang, Z.-Q. (2011). "Animal biodiversity: An introduction to higher-level classification and taxonomic richness" (PDF). Zootaxa. 3148: 7–12.
- ^ "Nematostella vectensis v1.0". Genome Portal. University of California. Retrieved 2014-01-19.
- ^ "Nematostella". Nematostella.org. Retrieved 2014-01-18.
- ^ a b Genikhovich, G.; U. Technau (2009). "The Starlet sea anemone Nematostella vectensis: An anthozoan model organism for studies in comparative genomics and functional evolutionary developmental biology". Cold Spring Harbor Protocols. 2009. doi:10.1101/pdb.emo129.
{{cite journal}}
: Unknown parameter|lastauthoramp=
ignored (|name-list-style=
suggested) (help) - ^ "Where Does Our Head Come From? Brainless Sea Anemone Sheds New Light on the Evolutionary Origin of the Head". Science Daily. 2013-02-12. Retrieved 2014-01-18.
- ^ Sinigaglia, C.; et al. (2013). "The bilaterian head patterning gene six3/6 controls aboral domain development in a cnidarian". PLoS Biology. 11 (2): e1001488. doi:10.1371/journal.pbio.1001488.
{{cite journal}}
: CS1 maint: unflagged free DOI (link) - ^ "Cornwall – Nature – Superstar Worm". BBC.
- ^ Mark Carwardine (1995) The Guinness Book of Animal Records. Guinness Publishing. p. 232.
- ^ http://plpnemweb.ucdavis.edu/nemaplex/General/animpara.htm
- ^ https://cid.oxfordjournals.org/content/29/4/734.full.pdf
- ^ Hodda, M (2011). "Phylum Nematoda Cobb, 1932. In: Zhang, Z.-Q. (Ed.) Animal biodiversity: An outline of higher-level classification and survey of taxonomic richness". Zootaxa. 3148: 63–95.
- ^ Zhang, Z (2013). "Animal biodiversity: An update of classification and diversity in 2013. In: Zhang, Z.-Q. (Ed.) Animal Biodiversity: An Outline of Higher-level Classification and Survey of Taxonomic Richness (Addenda 2013)". Zootaxa. 3703 (1): 5–11. doi:10.11646/zootaxa.3703.1.3.
- ^ Lambshead PJD (1993). "Recent developments in marine benthic biodiversity research". Oceanis. 19 (6): 5–24.
- ^ Borgonie G, García-Moyano A, Litthauer D, Bert W, Bester A, van Heerden E, Möller C, Erasmus M, Onstott TC (June 2011). "Nematoda from the terrestrial deep subsurface of South Africa". Nature. 474 (7349): 79–82. doi:10.1038/nature09974. PMID 21637257.
- ^ Danovaro R, Gambi C, Dell'Anno A, Corinaldesi C, Fraschetti S, Vanreusel A, Vincx M, Gooday AJ (January 2008). "Exponential decline of deep-sea ecosystem functioning linked to benthic biodiversity loss". Curr. Biol. 18 (1): 1–8. doi:10.1016/j.cub.2007.11.056. PMID 18164201.
{{cite journal}}
: Unknown parameter|laysource=
ignored (help); Unknown parameter|laysummary=
ignored (help) - ^ Platt HM (1994). "foreword". In Lorenzen S, Lorenzen SA (eds.). The phylogenetic systematics of freeliving nematodes. London: The Ray Society. ISBN 0-903874-22-9.
- ^ "Sea Lily". Science Encyclopedia. Retrieved Sep 5, 2014.
- ^ "Animal Diversity Web - Echinodermata". University of Michigan Museum of Zoology. Retrieved 26 August 2012.
- ^ Fox, Richard. "Asterias forbesi". Invertebrate Anatomy OnLine. Lander University. Retrieved 14 June 2014.
- ^ Holsinger, K. (2005). Keystone species. Retrieved May 10, 2010, from http://darwin.eeb.uconn.edu/eeb310/lecture-notes/interactions/node2.html
- ^ a b c Chapman, A.D. (2009). Numbers of Living Species in Australia and the World, 2nd edition. Australian Biological Resources Study, Canberra. Retrieved 12 January 2010. ISBN 978-0-642-56860-1 (printed); ISBN 978-0-642-56861-8 (online).
- ^ Barnes, R.S.K., Calow, P., Olive, P.J.W., Golding, D.W. and Spicer, J.I. (2001). The Invertebrates, A Synthesis (3 ed.). UK: Blackwell Science.
{{cite book}}
: CS1 maint: multiple names: authors list (link) - ^ a b Ruppert, pp. 284–291
- ^ a b Hayward, PJ (1996). Handbook of the Marine Fauna of North-West Europe. Oxford University Press. pp. 484–628. ISBN 0-19-854055-8.
- ^ Giribet, G.; Okusu, A, A.; Lindgren, A.R., A. R.; Huff, S.W., S. W.; Schrödl, M, M.; Nishiguchi, M.K., M. K. (May 2006). "Evidence for a clade composed of molluscs with serially repeated structures: monoplacophorans are related to chitons" (Free full text). Proceedings of the National Academy of Sciences of the United States of America. 103 (20): 7723–7728. Bibcode:2006PNAS..103.7723G. doi:10.1073/pnas.0602578103. PMC 1472512. PMID 16675549.
- ^ Ruppert, pp. 298–300
- ^ Healy, J.M. (2001). "The Mollusca". In Anderson, D.T. (ed.). Invertebrate Zoology (2 ed.). Oxford University Press. pp. 120–171. ISBN 0-19-551368-1.
- ^ Nicol, David (1969). "The Number of Living Species of Molluscs". Systematic Zoology. 18 (2): 251–254. doi:10.2307/2412618.
- ^ Haszprunar, G. (2001). "Mollusca (Molluscs)". Encyclopedia of Life Sciences. John Wiley & Sons, Ltd. doi:10.1038/npg.els.0001598.
- ^ Hancock, Rebecca (2008). "Recognising research on molluscs". Australian Museum. Retrieved 2009-03-09.
- ^ Ponder, Winston F.; Lindberg, David R. (2004). "Phylogeny of the Molluscs". World Congress of Malacology. Retrieved 2009-03-09.
{{cite web}}
: Unknown parameter|lastauthoramp=
ignored (|name-list-style=
suggested) (help) - ^ Brusca, R.C.; Brusca, G.J. (2003). Invertebrates (2 ed.). Sinauer Associates. p. 702. ISBN 0-87893-097-3.
{{cite book}}
: Unknown parameter|lastauthoramp=
ignored (|name-list-style=
suggested) (help) - ^ Raup, David M.; Stanley, Steven M. (1978). Principles of Paleontology (2 ed.). W.H. Freeman and Co. pp. 4–5. ISBN 0716700220.
{{cite book}}
: Unknown parameter|lastauthoramp=
ignored (|name-list-style=
suggested) (help) - ^ Black, Richard (April 26, 2008). "Colossal squid out of the freezer". BBC News.
- ^ Ewen Callaway (2 June 2008). "Simple-Minded Nautilus Shows Flash of Memory". New Scientist. Retrieved 7 March 2012.
- ^ Kathryn Phillips (15 June 2008). "Living Fossil Memories" (PDF). Inside JEB. 211 (12): iii. doi:10.1242/jeb.020370.
- ^ Robyn Crook; Jennifer Basil (2008). "A biphasic memory curve in the chambered nautilus, Nautilus pompilius L. (Cephalopoda: Nautiloidea)" (PDF). The Journal of Experimental Biology. 211 (12): 1992–1998. doi:10.1242/jeb.018531.
{{cite journal}}
: Unknown parameter|lastauthoramp=
ignored (|name-list-style=
suggested) (help) - ^ Ruppert, Fox & Barnes (2004), pp. 518–522
- ^ http://firstlifeseries.com/learn-more/
- ^ Gewin, V. (2005). "Functional genomics thickens the biological plot". PLoS biology, 3 (6), e219. doi:10.1371/journal.pbio.0030219
- ^ Lancelet (amphioxus) genome and the origin of vertebrates Ars Technica, 19 June 2008.
- ^ Hopkins Gareth R.; Brodie Edmund D., Jr (2015). "Occurrence of Amphibians in Saline Habitats: A Review and Evolutionary Perspective". Herpetological Monographs. 29 (1): 1–27. doi:10.1655/HERPMONOGRAPHS-D-14-00006.
- ^ Natchev, Nikolay; Tzankov, Nikolay; Geme, Richard (2011). "Green frog invasion in the Black Sea: habitat ecology of the Pelophylax esculentus complex (Anura, Amphibia) population in the region of Shablenska Тuzla lagoon in Bulgaria" (PDF). Herpetology Notes. 4: 347–351.
- ^ http://www.fishbase.org
- ^ http://www.worldwatch.org/node/784
- ^ Modesto, S.P.; Anderson, J.S. (2004). "The phylogenetic definition of Reptilia". Systematic Biology. 53 (5): 815–821. doi:10.1080/10635150490503026. PMID 15545258.
- ^ Gauthier, J.A.; Kluge, A.G.; Rowe, T. (1988). "The early evolution of the Amniota". The Phylogeny and Classification of the Tetrapods. Vol. 1. Oxford: Clarendon Press. pp. 103–155. ISBN 978-0-19-857705-8.
{{cite book}}
: Unknown parameter|editors=
ignored (|editor=
suggested) (help) - ^ Laurin, M.; Reisz, R. R. (1995). "A reevaluation of early amniote phylogeny" (PDF). Zoological Journal of the Linnean Society. 113 (2): 165–223. doi:10.1111/j.1096-3642.1995.tb00932.x.
- ^ Modesto, S.P. (1999). "Observations of the structure of the Early Permian reptile Stereosternum tumidum Cope". Palaeontologia Africana. 35: 7–19.
- ^ Sander, P. Martin. (2012). "Reproduction in early amniotes". Science. 337 (6096): 806–808. doi:10.1126/science.1224301.
- ^ Stidworthy J. 1974. Snakes of the World. Grosset & Dunlap Inc. 160 pp. ISBN 0-448-11856-4.
- ^ Sea snakes at Food and Agriculture Organization of the United Nations. Accessed 7 August 2007.
- ^ Lalli, C.; Parsons, T. (1993). Biological Oceanography: An Introduction. Butterworth-Heinemann. ISBN 0 7506 3384 0.
- ^ John Dolan (November 2012). "Microzooplankton: the microscopic (micro) animals (zoo) of the plankton" (PDF).
- ^ McKinney 1997, p. 110
- ^ Stearns & Stearns 1999, p. x
- ^ Novacek, Michael J. (November 8, 2014). "Prehistory's Brilliant Future". The New York Times. New York: The New York Times Company. ISSN 0362-4331. Retrieved 2014-12-25.
- ^ Nee, S. (2004). "Extinction, slime, and bottoms". PLoS Biology. 2 (8): E272. doi:10.1371/journal.pbio.0020272. PMC 509315. PMID 15314670.
{{cite journal}}
: CS1 maint: unflagged free DOI (link) - ^ Ward, Peter D (2006). "Impact from the Deep". Scientific American. 295 (4): 64–71. doi:10.1038/scientificamerican1006-64.
- ^ Desmond, Adrian (1975). "The Discovery of Marine Transgressions and the Explanation of Fossils in Antiquity". American Journal of Science 275: 692–697.
- ^ McKirahan, Richard D. "Xenophanes of Colophon. Philosophy Before Socrates. Indianapolis: Hackett Publishing Company, 1994. 66. Print.
- ^ Grene MG, Grene M and Depew D (2004) The Philosophy of Biology: An Episodic History pages 1–34, Cambridge University Press .ISBN 9780521643801.
- ^ Boylan, Michael "Aristotle: Biology" Internet Encyclopedia of Philosophy. Retrieved 5 August 2016.
- ^ Lee, H. D. P. (1948) "Place-Names and the date of Aristotle's Biological Works". Classical Quarterly, 42 (3/4) :61–7
- ^ Singer, Charles. A short history of biology. Oxford 1931.
- ^ Emily Kearns, "Animals, knowledge about," in Oxford Classical Dictionary, 3rd ed., 1996, p. 92.
- ^ Carl T. Bergstrom; Lee Alan Dugatkin (2012). Evolution. Norton. p. 35. ISBN 978-0-393-92592-0.
- ^ Rhodes, Frank Harold Trevor (1 January 1974). Evolution. Golden Press. p. 7. ISBN 978-0-307-64360-5.
- ^ a b c Lalli, Carol M., and Timothy R. Parsons. "Introduction." Biological Oceanography: An Introduction. First Edition ed. Tarrytown, New York: Pergamon, 1993. 7-21. Print.
- ^ Menden-Deuer, Susanne. "Course Info." OCG 561 Biological Oceanography. Web. 22 Oct. 2014. <http://www.gso.uri.edu/ocg561/>.
- ^ Miller, Charles B., and Patricia A. Wheeler. Biological Oceanography. Second ed. Chinchester, West Sussex: John Wiley & Sons, 2012. Print.
Further references
- Ruppert, E.E., Fox, R.S., and Barnes, R.D. (2004). Invertebrate Zoology (7 ed.). Brooks / Cole. ISBN 0-03-025982-7.
{{cite book}}
: CS1 maint: multiple names: authors list (link)