Iron: Difference between revisions
No edit summary |
m Reverting possible vandalism by 134.241.194.63 to version by Plantsurfer. False positive? report it. Thanks, User:ClueBot. (264408) (Bot) |
||
Line 1: | Line 1: | ||
:''For other uses, see [[Iron (disambiguation)]].'' |
|||
:''Fe redirects here. For other uses, see [[FE]].'' |
|||
{{Infobox iron}} |
|||
'''Iron''' ({{pronEng|ˈˈī(-ə)rn}}) is a [[chemical element]] with the symbol '''Fe''' ({{lang-la|ferrum}}) and [[atomic number]] 26. Iron is a [[Group 8 element|group 8]] and [[Period 4 element|period 4]] [[element]]. Iron is a lustrous, silvery soft metal. It is one of the few [[ferromagnet]]ic elements. |
|||
Iron and [[ |
Iron and [[nickel]] are notable for being the final elements produced by [[stellar nucleosynthesis]], and are therefore the heaviest elements which do not require a [[red giant]] or [[supernova]] for formation. Iron and nickel are therefore the most abundant metals in metallic meteorites and in the dense-metal cores of planets such as Earth. Iron and iron [[alloy]]s are also the most common source of [[ferromagnetic]] materials in everyday use. |
||
== Occurrence == |
== Occurrence == |
||
Line 19: | Line 23: | ||
==Allotropes== |
==Allotropes== |
||
{{Main|Allotropes of iron}} |
{{Main|Allotropes of iron}} |
||
Iron represents perhaps the best-known example for [[allotropy]] in a metal. There are four allotropic forms of iron, known as |
Iron represents perhaps the best-known example for [[allotropy]] in a metal. There are four allotropic forms of iron, known as alpha, beta, gamma, and delta. |
||
As molten iron cools down it crystallises at 1535°C into its delta allotrope, which has a [[body-centred cubic]] (BCC) crystal structure. As it cools further its [[crystal structure]] changes to [[face-centred cubic]] (FCC) at 1401°C, when it is known as gamma-iron, or austenite. At 912°C the crystal structure again becomes BCC as beta-iron4 is formed, and at 770°C (the Curie point, Tc ) the iron becomes [[magnetic]] as alpha-iron, also known as [[ferrite]], which is also BCC, is formed. Thus there is no change in crystalline structure, but there is a change in 'domain structure', where each domain contains iron atoms with a particular [[electronic spin]]. In unmagnetised iron, all the electronic spins of the atoms within one domain are in the same direction. However, in neighbouring domains they point in various directions and thus cancel out. In magnetised iron, the electronic spins of all the domains are all aligned, so that the magnetic effects of neighbouring domains reinforce each other. Although each domain contains billions of atoms, they are very small, about one thousandth of a centimetre across. |
As molten iron cools down it crystallises at 1535°C into its delta allotrope, which has a [[body-centred cubic]] (BCC) crystal structure. As it cools further its [[crystal structure]] changes to [[face-centred cubic]] (FCC) at 1401°C, when it is known as gamma-iron, or austenite. At 912°C the crystal structure again becomes BCC as beta-iron4 is formed, and at 770°C (the Curie point, Tc ) the iron becomes [[magnetic]] as alpha-iron, also known as [[ferrite]], which is also BCC, is formed. Thus there is no change in crystalline structure, but there is a change in 'domain structure', where each domain contains iron atoms with a particular [[electronic spin]]. In unmagnetised iron, all the electronic spins of the atoms within one domain are in the same direction. However, in neighbouring domains they point in various directions and thus cancel out. In magnetised iron, the electronic spins of all the domains are all aligned, so that the magnetic effects of neighbouring domains reinforce each other. Although each domain contains billions of atoms, they are very small, about one thousandth of a centimetre across. |
||
Line 33: | Line 37: | ||
* [[Pig iron]] has 3.5 - 4.5% carbon<ref name="msts">{{cite book | last = Camp | first = James McIntyre | coauthor = Francis, Charles Blaine | title = The Making, Shaping and Treating of Steel | publisher = Carnegie Steel Company |date=1920 | location = Pittsburgh | pages = 173 - 174 | url = http://books.google.com/books?id=P9MxAAAAMAAJ}}</ref> and contains varying amounts of contaminants such as [[sulfur]], [[silicon]] and [[phosphorus]]. Its only significance is that of an intermediate step on the way from [[iron ore]] to [[cast iron]] and [[steel]]. |
* [[Pig iron]] has 3.5 - 4.5% carbon<ref name="msts">{{cite book | last = Camp | first = James McIntyre | coauthor = Francis, Charles Blaine | title = The Making, Shaping and Treating of Steel | publisher = Carnegie Steel Company |date=1920 | location = Pittsburgh | pages = 173 - 174 | url = http://books.google.com/books?id=P9MxAAAAMAAJ}}</ref> and contains varying amounts of contaminants such as [[sulfur]], [[silicon]] and [[phosphorus]]. Its only significance is that of an intermediate step on the way from [[iron ore]] to [[cast iron]] and [[steel]]. |
||
* [[Cast iron]] contains 2% – 4.0% [[carbon]], 1% – 6% [[silicon]], and small amounts of [[manganese]]. Contaminants present in pig iron that negatively affect material properties, such as sulfur and phosphorus, have been reduced to an acceptable level. It has a melting point in the range of 1420–1470 K, which is lower than either of its two main components, and makes it the first product to be melted when carbon and iron are heated together. Its mechanical properties vary greatly, dependent upon the form [[carbon]] takes in the alloy. 'White' cast irons contain their carbon in the form of [[cementite]], or iron carbide. This |
* [[Cast iron]] contains 2% – 4.0% [[carbon]], 1% – 6% [[silicon]], and small amounts of [[manganese]]. Contaminants present in pig iron that negatively affect material properties, such as sulfur and phosphorus, have been reduced to an acceptable level. It has a melting point in the range of 1420–1470 K, which is lower than either of its two main components, and makes it the first product to be melted when carbon and iron are heated together. Its mechanical properties vary greatly, dependent upon the form [[carbon]] takes in the alloy. 'White' cast irons contain their carbon in the form of [[cementite]], or iron carbide. This hard, brittle compound dominates the mechanical properties of white cast irons, rendering them hard, but unresistant to shock. The broken surface of a white cast iron is full of fine facets of the broken carbide, a very pale, silvery, shiny material, hence the appellation. In [[grey iron]] the carbon exists free as fine flakes of [[graphite]], and also renders the material brittle due to the stress-raising nature of the sharp edged flakes of graphite. A newer variant of grey iron, referred to as [[ductile iron]] is specially treated with trace amounts of [[magnesium]] to alter the shape of graphite to spheroids, or nodules, vastly increasing the toughness and strength of the material. |
||
* [[Carbon steel]] contains 2.0% [[carbon]] or less,<ref name="kts">{{Citation | title = Classification of Carbon and Low-Alloy Steels | url = http://www.key-to-steel.com/Articles/Art62.htm | accessdate = January 5, 2008}}</ref> with small amounts of [[manganese]], [[sulfur]], [[phosphorus]], and [[silicon]]. |
* [[Carbon steel]] contains 2.0% [[carbon]] or less,<ref name="kts">{{Citation | title = Classification of Carbon and Low-Alloy Steels | url = http://www.key-to-steel.com/Articles/Art62.htm | accessdate = January 5, 2008}}</ref> with small amounts of [[manganese]], [[sulfur]], [[phosphorus]], and [[silicon]]. |
||
* [[Wrought iron]] contains less than 0.25% carbon.<ref name="msts"/> It is a tough, malleable product, but not as fusible as pig iron. If honed to an edge, it loses it quickly.{{Fact|date=January 2008}} Wrought iron is characterised by the presence of fine fibers of [[slag]] entrapped in the metal. Wrought iron is more corrosion resistant than steel. It has been almost completely replaced by [[mild steel]] for traditional "wrought iron" products and [[blacksmith]]ing. Mild steel does not have the same corrosion resistance but is cheaper and more widely available. |
* [[Wrought iron]] contains less than 0.25% carbon.<ref name="msts"/> It is a tough, malleable product, but not as fusible as pig iron. If honed to an edge, it loses it quickly.{{Fact|date=January 2008}} Wrought iron is characterised by the presence of fine fibers of [[slag]] entrapped in the metal. Wrought iron is more corrosion resistant than steel. It has been almost completely replaced by [[mild steel]] for traditional "wrought iron" products and [[blacksmith]]ing. Mild steel does not have the same corrosion resistance but is cheaper and more widely available. |
||
* [[Alloy steel]]s contain varying amounts of carbon as well as other metals, such as [[chromium]], [[vanadium]], [[molybdenum]], [[nickel]], [[tungsten]], etc. They are used for structural purposes, as their alloy content raises their cost and necessitates justification of their use. Recent developments in ferrous metallurgy have produced a growing range of microalloyed steels, also termed 'HSLA' or high-strength, low alloy steels, containing tiny additions to produce high strengths and often spectacular toughness at minimal cost. |
|||
* |
|||
* [[Iron(III) oxide]]s are used in the production of [[magnetic storage]] media in computers. They are often mixed with other compounds, and retain their magnetic properties in solution. |
|||
The main disadvantage of iron and steel is that pure iron, and most of its alloys, suffer badly from [[rust]] if not protected in some way. [[Paint]]ing, [[galvanization]], [[passivation]], plastic coating and [[Bluing (steel)|bluing]] are some techniques used to protect iron from rust by excluding [[water]] and [[oxygen]] or by sacrificial protection. |
|||
Iron is believed to be the critical missing nutrient in the ocean that limits the growth of [[plankton]]. Experimental [[iron fertilization]] of areas of the ocean using [[iron(II) sulfate]] has proven successful in increasing plankton growth.<ref>{{cite journal | author=Vivian Marx | title=The Little Plankton That Could…Maybe | journal=Scientific American | year=2002|url=http://www.sciam.com/article.cfm?articleID=000A5750-8AC2-1D9C-815A809EC5880000 }}</ref><ref>{{cite web | last = Melinda Ferguson, David Labiak, Andrew Madden, Joseph Peltier | title = The Effect of Iron on Plankton Use of CO2 | work = CEM 181H | url = http://www.cem.msu.edu/~cem181h/projects/96/iron/cem.html | accessdate = 2007-05-05 }}</ref><ref>{{cite web | last = Dopyera | first = Caroline | title = The Iron Hypothesis | publisher = EARTH |date=October, 1996 | url = http://www.palomar.edu/oceanography/iron.htm | accessdate = 2007-05-05 }}</ref> Larger scaled efforts are being attempted with the hope that iron seeding and ocean plankton growth can remove [[carbon dioxide]] from the atmosphere, thereby counteracting the [[greenhouse effect]] that is generally agreed by climatologists to cause [[global warming]].<ref>{{cite news | last = O'Conner | first = Steve | title = Researchers 'seed' ocean with iron to soak up CO2 | publisher = THE INDEPENDENT | date = [[2007-05-03]] | url = http://gcaptain.com/maritime/discoverer/story.php?title=Researchers_seed_ocean_with_iron_to_soak_up_CO2_-_Independent_Online_Edition_-_Climate_Change | accessdate = 2007-05-05 }}</ref> |
|||
===Iron compounds=== |
|||
{{main|Iron compounds}} |
|||
[[Image:Iron(III) chloride hexahydrate.jpg|right|thumb|Iron chloride hexahydrate]] |
|||
* Iron(III) acetate (Fe(C<sub>2</sub>H<sub>3</sub>O<sub>2</sub>)<sub>3</sub> is used in the [[dye]]ing of [[cloth]]. |
|||
* Iron(III) ammonium oxalate (Fe(NH<sub>4</sub>)<sub>3</sub>(C<sub>2</sub>O<sub>4</sub>)<sub>4</sub>) is used in [[blueprint]]s. |
|||
* Iron(III) arsenate (FeAsO<sub>4</sub>) is used in [[insecticide]]. |
|||
* [[Iron(III) chloride]] (FeCl<sub>3</sub>) is used: in [[water]] purification and sewage treatment, in the dyeing of cloth, as a coloring agent in [[paint]]s, as an additive in animal feed, and as an etching material for engravement, [[photography]] and printed circuits. |
|||
* Iron(III) chromate (Fe<sub>2</sub>(CrO<sub>4</sub>)<sub>3</sub>) is used as a yellow pigment for paints and [[ceramic]]. |
|||
* [[Iron(III) hydroxide]] (Fe(OH)<sub>3</sub>) is used as a brown [[pigment]] for [[rubber]] and in water purification systems. |
|||
* [[Iron(III) phosphate]] (FePO<sub>4</sub>) is used in [[fertilizer]] and as an additive in human and animal food. |
|||
* [[Iron(II) acetate]] (Fe(C<sub>2</sub>H<sub>3</sub>O<sub>2</sub>)<sub>2</sub> is used in the dyeing of fabrics and [[leather]], and as a [[wood]] preservative. |
|||
* [[Iron(II) gluconate]] (Fe(C<sub>6</sub>H<sub>11</sub>O<sub>7</sub>)<sub>2</sub>) is used as a dietary supplement in [[iron pill]]s. |
|||
* Iron(II) oxalate (FeC<sub>2</sub>O<sub>4</sub>) is used as yellow pigment for paints, [[plastic]]s, [[glass]] and ceramic, and in [[photography]]. |
|||
* [[Iron(II) sulfate]] (FeSO<sub>4</sub>) is used in water purification and sewage treatment systems, as a [[catalyst]] in the production of [[ammonia]], as an ingredient in fertilizer and [[herbicide]], as an additive in animal feed, in wood preservative and as an additive to [[flour]] to increase iron levels. |
|||
* Iron-Fluorine complex (FeF<sub>6</sub>)<sup>3-</sup> is found in solutions containing both Fe(III) [[ions]] and [[fluoride]] [[ions]]. |
|||
== Historical aspects== |
|||
{{Main|History of ferrous metallurgy}} |
|||
[[Image:Chinese Puddle and Blast Furnace.jpg|thumb|right|245px|The [[Puddling (metallurgy)|puddling]] process of smelting iron [[ore]] to make [[wrought iron]] from [[pig iron]], with the right illustration displaying men working a [[blast furnace]], from the ''Tiangong Kaiwu'' encyclopedia, published 1637 by [[Song Yingxing]].]] |
|||
The first iron used by mankind during [[prehistory]] came from meteors. The [[smelting]] of iron in [[bloomery|bloomeries]] probably began in [[Anatolia]] or the [[Caucasus]] in the second millennium BC or the latter part of the preceding one. [[Cast iron]] was first produced in [[China]] about 550 BC, but not in Europe until the medieval period. During the [[medieval]] period, means were found in Europe of producing [[wrought iron]] from [[cast iron]] (in this context known as [[pig iron]]) using [[finery forge]]s. For all these processes, [[charcoal]] was required as fuel. |
|||
[[Steel]] (with a smaller carbon content than [[pig iron]] but more than [[wrought iron]]) was first [[Iron_Age#The_transition_from_bronze_to_iron|produced in antiquity]]. New methods of producing it by [[carburizing]] bars of iron in the [[cementation process]] were devised in the 17th century AD. In the [[Industrial Revolution]], new methods of producing bar iron without charcoal were devised and these were later applied to produce steel. In the late 1850s, [[Henry Bessemer]] invented a new steelmaking process, involving blowing air through molten pig iron, to produce mild steel. This and other 19th century and later processes have led to [[wrought iron]] no longer being produced. |
|||
== Production of iron from iron ore == |
|||
{{seealso|Iron ore}} |
|||
The production of iron or steel is a process unless the desired final product is [[cast iron]]. The first stage is to produce [[pig iron]] in a [[blast furnace]]. The second is to make [[wrought iron]] or [[steel]] from pig iron by a further process. |
|||
===Blast furnace=== |
|||
{{main|Blast furnace}} |
|||
[[Image:Iron-Making.jpg|thumb|right|How Iron was extracted in the 19th century]] |
|||
[[Image:2005iron ore.PNG|thumb|left|Iron output in 2005]] |
|||
[[Image:LightningVolt Iron Ore Pellets.jpg|thumb|right|This heap of [[iron ore]] pellets will be used in [[steel]] production.]] |
|||
Ninety percent of all [[mining]] of metallic [[ore]]s is for the extraction of iron. Industrially, iron is produced starting from [[iron ore]]s, principally [[haematite]] (nominally Fe<sub>2</sub>O<sub>3</sub>) and [[magnetite]] (Fe<sub>3</sub>O<sub>4</sub>) by a [[carbothermic]] reaction (reduction with [[carbon]]) in a [[blast furnace]] at temperatures of about 2000 °C. In a blast furnace, iron ore, carbon in the form of [[coke (fuel)|coke]], and a ''flux'' such as [[limestone]] (which is used to remove impurities in the ore which would otherwise clog the furnace with solid material) are fed into the top of the furnace, while a blast of heated [[Earth's atmosphere|air]] is forced into the furnace at the bottom. |
|||
In the furnace, the [[coke (fuel)|coke]] reacts with [[oxygen]] in the air blast to produce [[carbon monoxide]]: |
|||
:2 [[carbon|C]] + [[oxygen|O<sub>2</sub>]] → 2 [[carbon monoxide|CO]] |
|||
The carbon monoxide reduces the iron ore (in the [[chemical equation]] below, hematite) to molten iron, becoming [[carbon dioxide]] in the process: |
|||
:3 [[carbon monoxide|CO]] + [[haematite|Fe<sub>2</sub>O<sub>3</sub>]] → 2 Fe + 3 [[carbon dioxide|CO<sub>2</sub>]] |
|||
The flux is present to melt impurities in the ore, principally [[silicon dioxide]] [[sand]] and other [[silicate]]s. Common fluxes include limestone (principally [[calcium carbonate]]) and dolomite (calcium-magnesium carbonate). Other fluxes may be used depending on the impurities that need to be removed from the ore. In the heat of the furnace the limestone flux decomposes to [[calcium oxide]] (quicklime): |
|||
:[[calcium carbonate|CaCO<sub>3</sub>]] → [[calcium oxide|CaO]] + [[carbon dioxide|CO<sub>2</sub>]] |
|||
Then calcium oxide combines with silicon dioxide to form a ''slag''. |
|||
:[[calcium oxide|CaO]] + [[silicon dioxide|SiO<sub>2</sub>]] → [[wollastonite|CaSiO<sub>3</sub>]] |
|||
The slag melts in the heat of the furnace. In the bottom of the furnace, the molten slag floats on top of the more dense molten iron, and apertures in the side of the furnace are opened to run off the iron and the slag separately. The iron once cooled, is called [[pig iron]], while the slag can be used as a material in [[road]] construction or to improve mineral-poor soils for [[agriculture]].{{Fact|date=January 2008}} |
|||
In 2005, approximately 1,544 Mt (million [[metric ton]]s) of iron ore was produced worldwide. China was the top producer of iron ore with at least one-fourth world share followed by Brazil, Australia and India, reports the [[British Geological Survey]]. |
|||
===Further processes=== |
|||
Pig iron is not pure iron, but has 4-5% carbon dissolved in it. This is subsequently reduced to [[steel]] or commercially pure iron, known as [[wrought iron]], using other furnaces or converters. |
|||
<!-- This section ought to be expanded a little, explainign the differnece between steel and wrought iron and referring to "main" articles on these. --> |
|||
== Isotopes == |
|||
{{Main|Isotopes of iron}} |
|||
Naturally occurring iron consists of four [[isotope]]s: 5.845% of radioactive <sup>54</sup>Fe (half-life: >3.1×10<sup>22</sup> years), 91.754% of stable <sup>56</sup>Fe, 2.119% of stable <sup>57</sup>Fe and 0.282% of stable <sup>58</sup>Fe. |
|||
<sup>60</sup>Fe is an [[extinct radionuclide]] of long [[half-life]] (1.5 million years). |
|||
Much of the past work on measuring the isotopic composition of Fe has centered on determining <sup>60</sup>Fe variations due to processes accompanying [[nucleosynthesis]] (i.e., [[meteorite]] studies) and ore formation. In the last decade however, advances in [[mass spectrometry]] technology have allowed the detection and quantification of minute, naturally occurring variations in the ratios of the [[stable isotope]]s of iron. Much of this work has been driven by the [[Earth science|Earth]] and [[planetary science]] communities, although applications to biological and industrial systems are beginning to emerge.<ref>Dauphas, N. & Rouxel, O. 2006. Mass spectrometry and natural variations of iron isotopes. ''Mass Spectrometry Reviews'', '''25,''' 515-550</ref> |
|||
The isotope <sup>56</sup>Fe is of particular interest to nuclear scientists. A common misconception is that this isotope represents the most stable nucleus possible, and that it thus would be impossible to perform fission or fusion on <sup>56</sup>Fe and still liberate energy. This is not true, as both <sup>62</sup>Ni and <sup>58</sup>Fe are more stable, being the most stable nuclei. However, since <sup>56</sup>Fe is much more easily produced from lighter nuclei in nuclear reactions, it is the endpoint of fusion chains inside [[Metallicity#Population III stars|extremely massive stars]] and is therefore common in the universe, relative to other [[Metallicity|metals]]. |
|||
In phases of the meteorites ''Semarkona'' and ''Chervony Kut'' a correlation between the concentration of <sup>60</sup>[[Nickel|Ni]], the [[daughter product]] of <sup>60</sup>Fe, and the abundance of the stable iron isotopes could be found which is evidence for the existence of <sup>60</sup>Fe at the time of formation of the solar system. Possibly the energy released by the decay of <sup>60</sup>Fe contributed, together with the energy released by decay of the radionuclide <sup>26</sup>[[Aluminium|Al]], to the remelting and [[planetary differentiation|differentiation]] of [[asteroid]]s after their formation 4.6 billion years ago. The abundance of <sup>60</sup>[[nickel|Ni]] present in [[wikt:extraterrestrial|extraterrestrial]] material may also provide further insight into the origin of the [[solar system]] and its early history. |
|||
Of the stable isotopes, only <sup>57</sup>Fe has a nuclear [[spin (physics)|spin]] (−1/2). |
|||
==Iron in organic synthesis== |
|||
The use of iron metal filings in organic synthesis is mainly for the [[reduction of nitro compounds|reduction]] of [[nitro compound]]s.<ref>Fox, B. A.; Threlfall, T. L. ''[[Organic Syntheses]]'', Coll. Vol. 5, p.346 (1973); Vol. 44, p.34 (1964). ([http://www.orgsyn.org/orgsyn/prep.asp?prep=cv5p0346 Article])</ref> Additionally, iron has been used for [[desulfurization]]s,<ref>Blomquist, A. T.; Dinguid, L. I. ''[[J. Org. Chem.]]'' '''1947''', ''12'', 718 & 723.</ref> [[redox|reduction]] of [[aldehyde]]s,<ref>Clarke, H. T.; Dreger, E. E. ''[[Org. Syn.]]'', Coll. Vol. 1, p.304 (1941); Vol. 6, p.52 (1926). ([http://www.orgsyn.org/orgsyn/prep.asp?prep=cv1p0304 Article]).</ref> and the [[deoxygenation]] of amine oxides.<ref>den Hertog, J.; Overhoff, J. ''Recl. Trav. Chim. Pays-Bas'' '''1950''', ''69'', 468.</ref> |
|||
== Iron in biology == |
|||
[[Image:Heme.svg|thumb|right|Structure of [[Heme|Heme b]]]] |
|||
{{main|Human iron metabolism}} |
|||
Iron is essential to nearly all known [[organism]]s. In [[cell (biology)|cell]]s, iron is generally stored in the centre of [[metalloprotein]]s, because "free" iron -- which binds non-specifically to many cellular components -- can catalyse production of toxic [[free radical]]s. |
|||
In animals, plants, and fungi, iron is often incorporated into the [[heme]] complex. Heme is an essential component of [[cytochrome]] proteins, which mediate [[redox]] reactions, and of oxygen carrier proteins such as [[hemoglobin]], [[myoglobin]], and [[leghemoglobin]]. Inorganic iron also contributes to redox reactions in the [[iron-sulfur cluster]]s of many [[enzyme]]s, such as [[nitrogenase]] (involved in the synthesis of [[ammonia]] from [[nitrogen]] and [[hydrogen]]) and [[hydrogenase]]. [[Non-heme iron proteins]] include the [[enzymes]] [[methane monooxygenase]] (oxidizes [[methane]] to [[methanol]]), [[ribonucleotide reductase]] (reduces [[ribose]] to [[deoxyribose]]; [[DNA replication|DNA biosynthesis]]), [[hemerythrin]]s ([[oxygen]] transport and fixation in [[marine biology#other sea life|marine invertebrate]]s) and [[purple acid phosphatase]] ([[hydrolysis]] of [[phosphate]] [[ester]]s). |
|||
Iron distribution is heavily regulated in [[mammal]]s, partly because iron has a high potential for biological toxicity. Iron distribution is also regulated because many bacteria require iron, so restricting its availability to bacteria (generally by [[wiktionary:sequester|sequester]]ing it inside cells) can help to prevent or limit infections. This is probably the reason for the relatively low amounts of iron in mammalian milk. A major component of this regulation is the protein [[transferrin]], which binds iron absorbed from the [[duodenum]] and carries it in the [[bloodstream|blood]] to cells.<ref>{{cite web |
|||
|url=http://biology.plosjournals.org/perlserv/?request=get-document&doi=10.1371/journal.pbio.0000079 |
|||
|title=How Mammals Acquire and Distribute Iron Needed for Oxygen-Based Metabolism |
|||
|author=Tracey A. Rouault |
|||
|accessdate=2006-06-19 |
|||
}}</ref> |
|||
=== Nutrition and dietary sources === |
|||
Good sources of dietary iron include [[red meat]], [[fish]], [[poultry]], [[lentil]]s, [[bean]]s, [[leaf vegetable]]s, [[tofu]], [[chickpea]]s, [[black-eyed peas]], potatoes with skin, bread made from completely whole-grain flour, [[molasses]], [[teff]] and [[farina (food)|farina]]. Iron in meat is more easily absorbed than iron in vegetables.<ref>[http://www.eatwell.gov.uk/healthissues/irondeficiency/ Food Standards Agency - Eat well, be well - Iron deficiency<!-- Bot generated title -->]</ref> |
|||
Iron provided by [[dietary supplement]]s is often found as [[ferrous fumarate|iron (II) fumarate]], although iron sulfate is cheaper and is absorbed equally well. Elemental iron, despite being absorbed to a much smaller extent (stomach acid is sufficient to convert some of it to ferrous iron), is often added to foods such as breakfast cereals or "enriched" wheat flour (where it is listed as "reduced iron" in the list of ingredients). Iron is most available to the body when chelated to amino acids - iron in this form is ten to fifteen times more bioavailable<ref name="pmid11377130">{{cite journal |author=Pineda O, Ashmead HD |title=Effectiveness of treatment of iron-deficiency anemia in infants and young children with ferrous bis-glycinate chelate |journal=Nutrition |volume=17 |issue=5 |pages=381–4 |year=2001 |pmid=11377130 |doi=}}</ref> than any other, and is also available for use as a common [[iron supplements|iron supplement]]. Often the amino acid chosen for this purpose is the cheapest and most common amino acid, glycine, leading to "iron glycinate" supplements.<ref name=Ashmead>{{cite book |
|||
|last = Ashmead |
|||
|first = H. DeWayne |
|||
|authorlink = |
|||
|coauthors = |
|||
|year = 1989 |
|||
|title = ''Conversations on Chelation and Mineral Nutrition'' |
|||
|publisher = Keats Publishing |
|||
|location = |
|||
|id = ISBN 0-87983-501-X |
|||
}}</ref> The [[Recommended Dietary Allowance|RDA]] for iron varies considerably based on age, gender, and source of dietary iron ([[heme]]-based iron has higher [[bioavailability]]).<ref>{{cite web |
|||
|url=http://www.iom.edu/Object.File/Master/7/294/0.pdf |
|||
|title=Dietary Reference Intakes: Elements |
|||
|format=PDF |
|||
}}</ref> Infants will require iron supplements if they are not breast-fed. [[Blood donation|Blood donors]] are at special risk of low iron levels and are often advised to supplement their iron intake. |
|||
===Regulation of iron uptake=== |
|||
Excessive iron can be toxic, because free ferrous iron reacts with [[peroxide]]s to produce [[free radical]]s, which are highly reactive and can damage [[DNA]], [[proteins]], [[lipids]], and other cellular components. Thus, iron toxicity occurs when there is free iron in the cell, which generally occurs when iron levels exceed the capacity of [[transferrin]] to bind the iron. |
|||
[[human iron metabolism|Iron uptake]] is tightly regulated by the human body, which has no physiological means of excreting iron, so controls iron levels solely by regulating uptake. Although uptake is regulated, large amounts of ingested iron can cause excessive levels of iron in the blood, because high iron levels can cause damage to the cells of the [[gastrointestinal tract]] that prevents them from regulating iron absorption. High blood concentrations of iron damage cells in the [[heart]], [[liver]] and elsewhere, which can cause serious problems, including long-term organ damage and even death. |
|||
Humans experience iron toxicity above 20 milligrams of iron for every [[kilogram]] of mass, and 60 milligrams per kilogram is a [[lethal dose]].<ref name="emed-topic285"> |
|||
{{cite web |
|||
|url=http://www.emedicine.com/emerg/topic285.htm |
|||
|title=Toxicity, Iron |
|||
|publisher=Emedicine |
|||
|accessdate=2006-06-19 |
|||
}}</ref> Over-consumption of iron, often the result of children eating large quantities of [[ferrous sulfate]] tablets intended for adult consumption, is one of the most common toxicological causes of death in children under six.<ref name="emed-topic285"/> The [[Dietary Reference Intake|DRI]] lists the Tolerable Upper Intake Level (UL) for adults as 45 [[milligram|mg]]/day. For children under fourteen years old the UL is 40 mg/day. |
|||
Regulation of iron uptake is impaired in some people as a result of a genetic defect that maps to the HLA-H gene region on chromosome 6. In these people, excessive iron intake can result in [[iron overload disorder]]s, such as [[hemochromatosis]]. Many people have a genetic susceptibility to iron overload without realizing it or being aware of a family history of the problem. For this reason, it is advised that people should not take iron supplements unless they suffer from [[iron deficiency (medicine)|iron deficiency]] and have consulted a doctor. Hemochromatosis is estimated to cause disease in between 0.3 and 0.8% of Caucasians. <ref> Durupt S, Durieu I, Nove-Josserand R, et al: [Hereditary hemochromatosis]. Rev Med Interne 2000 Nov; 21(11): 961-71[Medline].</ref> |
|||
The medical management of iron toxicity is complex, and can include use of a specific [[chelation|chelating]] agent called [[deferoxamine]] to bind and expel excess iron from the body. |
|||
==Bibliography== |
|||
*[http://periodic.lanl.gov/elements/26.html Los Alamos National Laboratory — Iron] |
|||
*H. R. Schubert, ''History of the British Iron and Steel Industry ... to 1775 AD'' (Routledge, London, 1957) |
|||
*R. F. Tylecote, ''History of Metallurgy'' (Institute of Materials, London 1992). |
|||
*R. F. Tylecote, 'Iron in the Industrial Revolution' in J. Day and R. F. Tylecote, ''The Industrial Revolution in Metals'' (Institute of Materials 1991), 200-60. |
|||
*[http://www.webelements.com/webelements/elements/text/Fe/xtal.html Crystal structure of iron] |
|||
==References== |
|||
{{Reflist}} |
|||
{{Refbegin}} |
|||
* Doulias PT, Christoforidis S, Brunk UT, Galaris D. Endosomal and lysosomal effects of desferrioxamine: protection of HeLa cells from hydrogen peroxide-induced DNA damage and induction of cell-cycle arrest. Free Radic Biol Med. 2003;35:719-28. |
|||
{{Refend}} |
|||
==See also== |
|||
<!-- Please keep this list tidy and in alphabetical order. Avoid links prominently featured in article. --> |
|||
{{wiktionarypar|iron}} |
|||
{{Commons|Iron}} |
|||
* [[El Mutún]] in [[Bolivia]], where 20% of the world's accessible iron and [[magnesium]] is located |
|||
* [[Iron (metaphor)]] |
|||
* [[Iron Age]] |
|||
* [[Iron fertilization]] - Fertilization of oceans to stimulate [[phytoplankton]] growth |
|||
* [[Pelletizing]] - Process of creation of iron ore pellets |
|||
* [[Al-Hadid]] (Iron) in the [[Qur'an]] |
|||
* [[Specht Building]] - A historic landmark in [[Omaha, Nebraska]] utilizing an iron facade. |
|||
* [[Iron in mythology]] |
|||
==External links== |
|||
* [http://www.webelements.com/webelements/elements/text/Fe/index.html WebElements.com – Iron] |
|||
* [http://education.jlab.org/itselemental/ele026.html It's Elemental – Iron] |
|||
* [http://hyperphysics.phy-astr.gsu.edu/hbase/nucene/nucbin2.html The Most Tightly Bound Nuclei] |
|||
[[Category:Dietary minerals]] |
|||
[[Category:Iron| ]] |
|||
[[Category:Chemical elements|Iron]] |
|||
[[Category:Recyclable materials]] |
|||
[[Category:Transition metals]] |
|||
[[Category:Ferromagnetic materials]] |
|||
[[Category:Building materials]] |
|||
[[Category:Pyrotechnic fuels]] |
|||
{{Link FA|af}} |
|||
{{Link FA|eo}} |
|||
{{Link FA|pt}} |
|||
{{Link FA|ru}} |
|||
<!-- interwiki --> |
|||
[[af:Yster]] |
|||
[[als:Eisen]] |
|||
[[ar:حديد]] |
|||
[[ast:Fierro]] |
|||
[[az:Dəmir]] |
|||
[[bn:লোহা]] |
|||
[[zh-min-nan:Fe (goân-sò͘)]] |
|||
[[be:Жалеза]] |
|||
[[be-x-old:Жалеза]] |
|||
[[bs:Željezo]] |
|||
[[br:Houarn]] |
|||
[[bg:Желязо]] |
|||
[[ca:Ferro]] |
|||
[[cv:Тимĕр]] |
|||
[[cs:Železo]] |
|||
[[co:Ferru]] |
|||
[[cy:Haearn]] |
|||
[[da:Jern]] |
|||
[[de:Eisen]] |
|||
[[et:Raud]] |
|||
[[el:Σίδηρος]] |
|||
[[es:Hierro]] |
|||
[[eo:Fero]] |
|||
[[eu:Burdina]] |
|||
[[fa:آهن]] |
|||
[[fr:Fer]] |
|||
[[fur:Fier]] |
|||
[[ga:Iarann]] |
|||
[[gd:Iarann]] |
|||
[[gl:Fe (elemento)]] |
|||
[[gu:લોખંડ]] |
|||
[[ko:철]] |
|||
[[hy:Երկաթ]] |
|||
[[hi:लोहम्]] |
|||
[[hr:Željezo]] |
|||
[[io:Fero]] |
|||
[[id:Besi]] |
|||
[[ia:Ferro]] |
|||
[[is:Járn]] |
|||
[[it:Ferro]] |
|||
[[he:ברזל]] |
|||
[[ka:რკინა]] |
|||
[[sw:Chuma]] |
|||
[[kg:Kibende]] |
|||
[[ht:Fè]] |
|||
[[ku:Hesin]] |
|||
[[la:Ferrum]] |
|||
[[lv:Dzelzs]] |
|||
[[lb:Eisen]] |
|||
[[lt:Geležis]] |
|||
[[li:Iezer]] |
|||
[[ln:Ebendé]] |
|||
[[jbo:tirse]] |
|||
[[hu:Vas]] |
|||
[[mk:Железо]] |
|||
[[ml:ഇരുമ്പ്]] |
|||
[[mt:Ħadid]] |
|||
[[mi:Rino]] |
|||
[[mr:लोखंड]] |
|||
[[ms:Besi]] |
|||
[[mn:Төмөр]] |
|||
[[nah:Tlīltepoztli]] |
|||
[[nl:IJzer (element)]] |
|||
[[ja:鉄]] |
|||
[[no:Jern]] |
|||
[[nn:Jern]] |
|||
[[nrm:Fé]] |
|||
[[oc:Fèrre]] |
|||
[[uz:Temir]] |
|||
[[nds:Iesen]] |
|||
[[pl:Żelazo]] |
|||
[[pt:Ferro]] |
|||
[[ksh:Eisen]] |
|||
[[ro:Fier]] |
|||
[[qu:Chuki]] |
|||
[[ru:Железо]] |
|||
[[sa:लोहम्]] |
|||
[[sco:Airn]] |
|||
[[sq:Hekuri]] |
|||
[[scn:Ferru]] |
|||
[[simple:Iron]] |
|||
[[sk:Železo]] |
|||
[[sl:Železo]] |
|||
[[sr:Гвожђе (хемијски елемент)]] |
|||
[[sh:Željezo]] |
|||
[[fi:Rauta]] |
|||
[[sv:Järn]] |
|||
[[ta:இரும்பு]] |
|||
[[te:ఇనుము]] |
|||
[[th:เหล็ก]] |
|||
[[vi:Sắt]] |
|||
[[tg:Оҳан]] |
|||
[[tr:Demir]] |
|||
[[uk:Залізо]] |
|||
[[yi:אייזן]] |
|||
[[zh-yue:鐵]] |
|||
[[zh:铁]] |
Revision as of 20:33, 7 March 2008
- For other uses, see Iron (disambiguation).
- Fe redirects here. For other uses, see FE.
![]() | ||||||||||||||||||||||||||||||||||||||||||||||
Iron | ||||||||||||||||||||||||||||||||||||||||||||||
---|---|---|---|---|---|---|---|---|---|---|---|---|---|---|---|---|---|---|---|---|---|---|---|---|---|---|---|---|---|---|---|---|---|---|---|---|---|---|---|---|---|---|---|---|---|---|
Pronunciation | /ˈaɪərn/ | |||||||||||||||||||||||||||||||||||||||||||||
Allotropes | see Allotropes of iron | |||||||||||||||||||||||||||||||||||||||||||||
Appearance | lustrous metallic with a grayish tinge | |||||||||||||||||||||||||||||||||||||||||||||
Standard atomic weight Ar°(Fe) | ||||||||||||||||||||||||||||||||||||||||||||||
Iron in the periodic table | ||||||||||||||||||||||||||||||||||||||||||||||
| ||||||||||||||||||||||||||||||||||||||||||||||
Atomic number (Z) | 26 | |||||||||||||||||||||||||||||||||||||||||||||
Group | group 8 | |||||||||||||||||||||||||||||||||||||||||||||
Period | period 4 | |||||||||||||||||||||||||||||||||||||||||||||
Block | d-block | |||||||||||||||||||||||||||||||||||||||||||||
Electron configuration | [Ar] 3d6 4s2 | |||||||||||||||||||||||||||||||||||||||||||||
Electrons per shell | 2, 8, 14, 2 | |||||||||||||||||||||||||||||||||||||||||||||
Physical properties | ||||||||||||||||||||||||||||||||||||||||||||||
Phase at STP | solid | |||||||||||||||||||||||||||||||||||||||||||||
Melting point | 1811 K (1538 °C, 2800 °F) | |||||||||||||||||||||||||||||||||||||||||||||
Boiling point | 3134 K (2861 °C, 5182 °F) | |||||||||||||||||||||||||||||||||||||||||||||
Density (at 20° C) | 7.874 g/cm3 [3] | |||||||||||||||||||||||||||||||||||||||||||||
when liquid (at m.p.) | 6.98 g/cm3 | |||||||||||||||||||||||||||||||||||||||||||||
Heat of fusion | 13.81 kJ/mol | |||||||||||||||||||||||||||||||||||||||||||||
Heat of vaporization | 340 kJ/mol | |||||||||||||||||||||||||||||||||||||||||||||
Molar heat capacity | 25.10 J/(mol·K) | |||||||||||||||||||||||||||||||||||||||||||||
Vapor pressure
| ||||||||||||||||||||||||||||||||||||||||||||||
Atomic properties | ||||||||||||||||||||||||||||||||||||||||||||||
Oxidation states | −4, −2, −1, 0, +1,[4] +2, +3, +4, +5,[5] +6, +7[6] (an amphoteric oxide) | |||||||||||||||||||||||||||||||||||||||||||||
Electronegativity | Pauling scale: 1.83 | |||||||||||||||||||||||||||||||||||||||||||||
Ionization energies |
| |||||||||||||||||||||||||||||||||||||||||||||
Atomic radius | empirical: 126 pm | |||||||||||||||||||||||||||||||||||||||||||||
Covalent radius | Low spin: 132±3 pm High spin: 152±6 pm | |||||||||||||||||||||||||||||||||||||||||||||
Van der Waals radius | 194 [1] pm | |||||||||||||||||||||||||||||||||||||||||||||
![]() | ||||||||||||||||||||||||||||||||||||||||||||||
Other properties | ||||||||||||||||||||||||||||||||||||||||||||||
Natural occurrence | primordial | |||||||||||||||||||||||||||||||||||||||||||||
Crystal structure | α-Fe: body-centered cubic (bcc) (cI2) | |||||||||||||||||||||||||||||||||||||||||||||
Lattice constant | a = 286.65 pm (at 20 °C)[3] | |||||||||||||||||||||||||||||||||||||||||||||
Crystal structure | γ-Fe (912–1394 °C): face-centered cubic (fcc) (cF4) | |||||||||||||||||||||||||||||||||||||||||||||
Lattice constant | a = 364.68 pm (at 916°C)[7] | |||||||||||||||||||||||||||||||||||||||||||||
Thermal expansion | 12.07×10−6/K (at 20 °C)[3] | |||||||||||||||||||||||||||||||||||||||||||||
Thermal conductivity | 80.4 W/(m⋅K) | |||||||||||||||||||||||||||||||||||||||||||||
Electrical resistivity | 96.1 nΩ⋅m (at 20 °C) | |||||||||||||||||||||||||||||||||||||||||||||
Curie point | 1043 K | |||||||||||||||||||||||||||||||||||||||||||||
Magnetic ordering | ferromagnetic | |||||||||||||||||||||||||||||||||||||||||||||
Young's modulus | 211 GPa | |||||||||||||||||||||||||||||||||||||||||||||
Shear modulus | 82 GPa | |||||||||||||||||||||||||||||||||||||||||||||
Bulk modulus | 170 GPa | |||||||||||||||||||||||||||||||||||||||||||||
Speed of sound thin rod | 5120 m/s (at r.t.) (electrolytic) | |||||||||||||||||||||||||||||||||||||||||||||
Poisson ratio | 0.29 | |||||||||||||||||||||||||||||||||||||||||||||
Mohs hardness | 4 | |||||||||||||||||||||||||||||||||||||||||||||
Vickers hardness | 608 MPa | |||||||||||||||||||||||||||||||||||||||||||||
Brinell hardness | 200–1180 MPa | |||||||||||||||||||||||||||||||||||||||||||||
CAS Number | 7439-89-6 | |||||||||||||||||||||||||||||||||||||||||||||
History | ||||||||||||||||||||||||||||||||||||||||||||||
Discovery | before 5000 BC | |||||||||||||||||||||||||||||||||||||||||||||
Symbol | "Fe": from Latin ferrum | |||||||||||||||||||||||||||||||||||||||||||||
Isotopes of iron | ||||||||||||||||||||||||||||||||||||||||||||||
| ||||||||||||||||||||||||||||||||||||||||||||||
Iron (Template:PronEng) is a chemical element with the symbol Fe (Latin: ferrum) and atomic number 26. Iron is a group 8 and period 4 element. Iron is a lustrous, silvery soft metal. It is one of the few ferromagnetic elements.
Iron and nickel are notable for being the final elements produced by stellar nucleosynthesis, and are therefore the heaviest elements which do not require a red giant or supernova for formation. Iron and nickel are therefore the most abundant metals in metallic meteorites and in the dense-metal cores of planets such as Earth. Iron and iron alloys are also the most common source of ferromagnetic materials in everyday use.
Occurrence
Iron is believed to be the sixth most abundant element in the universe, formed as the final act of nucleosynthesis by carbon burning in massive stars. While it makes up only about 5% of the Earth's crust, the earth's core is believed to consist largely of an iron-nickel alloy comprising 35% of the mass of the Earth as a whole. Iron is consequently the most abundant element on Earth, but only the fourth most abundant element in the Earth's crust[2] where it is the second most abundant metal after aluminium. Most of the iron in the crust is found combined with oxygen as iron oxide minerals such as hematite and magnetite.[citation needed]. About 1 in 20 meteorites consist of the unique iron-nickel minerals taenite (35-80% iron) and kamacite (90-95% iron). Although rare, meteorites are the major form of natural metallic iron on the earth's surface.
The reason for Mars' red colour is thought to be an iron-oxide-rich soil.
See also Iron minerals.

Characteristics
Iron is a metal extracted mainly from the iron ore hematite. It oxidises readily in air and water to form Fe
2O
3 and is rarely found as a free element. In order to obtain elemental iron, oxygen and other impurities must be removed by chemical reduction. The properties of iron can be modified by alloying it with various other metals and some non-metals, notably carbon and silicon to form steels.
Nuclei of iron have some of the highest binding energies per nucleon, surpassed only by the nickel isotope 62Ni. The universally most abundant of the highly stable nuclides is, however, 56Fe. This is formed by nuclear fusion in stars. Although a further tiny energy gain could be extracted by synthesizing 62Ni, conditions in stars are unsuitable for this process to be favoured, and iron abundance on Earth greatly favors iron over nickel, and also presumably in supernova element production.[9]
Iron (as Fe2+, ferrous ion) is a necessary trace element used by almost all living organisms. The only exceptions are several organisms that live in iron-poor environments and have evolved to use different elements in their metabolic processes, such as manganese instead of iron for catalysis, or hemocyanin instead of hemoglobin. Iron-containing enzymes, usually containing heme prosthetic groups, participate in catalysis of oxidation reactions in biology, and in transport of a number of soluble gases. See hemoglobin, cytochrome, and catalase.
Allotropes
Iron represents perhaps the best-known example for allotropy in a metal. There are four allotropic forms of iron, known as alpha, beta, gamma, and delta.
As molten iron cools down it crystallises at 1535°C into its delta allotrope, which has a body-centred cubic (BCC) crystal structure. As it cools further its crystal structure changes to face-centred cubic (FCC) at 1401°C, when it is known as gamma-iron, or austenite. At 912°C the crystal structure again becomes BCC as beta-iron4 is formed, and at 770°C (the Curie point, Tc ) the iron becomes magnetic as alpha-iron, also known as ferrite, which is also BCC, is formed. Thus there is no change in crystalline structure, but there is a change in 'domain structure', where each domain contains iron atoms with a particular electronic spin. In unmagnetised iron, all the electronic spins of the atoms within one domain are in the same direction. However, in neighbouring domains they point in various directions and thus cancel out. In magnetised iron, the electronic spins of all the domains are all aligned, so that the magnetic effects of neighbouring domains reinforce each other. Although each domain contains billions of atoms, they are very small, about one thousandth of a centimetre across.
Iron, of course, is of most importance when mixed with certain other metals and with carbon to form steels. There are many types of steels, all with different properties; and an understanding of the properties of the allotropes of iron is key to the manufacture of good quality steels.
Alpha iron, also known as ferrite, is the most stable form of iron at normal temperatures. It is a fairly soft metal that can dissolve only a small concentration of carbon (no more than 0.021% by mass at 910 °C).
Above 912°C and up to 1401°C alpha iron undergoes a phase transition from body-centred cubic to the face-centred cubic configuration of gamma iron, also called austenite. This is similarly soft and metallic but can dissolve considerably more carbon (as much as 2.04% by mass at 1146°C). This form of iron is used in the type of stainless steel used for making cutlery, and hospital and food-service equipment.
Applications
Iron is the most widely used of all the metals, accounting for 95% of worldwide metal production. Its low cost and high strength make it indispensable in and engineering applications such as the construction of machinery and machine tools, automobiles, the hulls of large ships, and structural components for buildings. Since pure iron is quite soft, it is most commonly used in the form of Steels. Some of the forms in which iron is produced commercially include:
- Pig iron has 3.5 - 4.5% carbon[10] and contains varying amounts of contaminants such as sulfur, silicon and phosphorus. Its only significance is that of an intermediate step on the way from iron ore to cast iron and steel.
- Cast iron contains 2% – 4.0% carbon, 1% – 6% silicon, and small amounts of manganese. Contaminants present in pig iron that negatively affect material properties, such as sulfur and phosphorus, have been reduced to an acceptable level. It has a melting point in the range of 1420–1470 K, which is lower than either of its two main components, and makes it the first product to be melted when carbon and iron are heated together. Its mechanical properties vary greatly, dependent upon the form carbon takes in the alloy. 'White' cast irons contain their carbon in the form of cementite, or iron carbide. This hard, brittle compound dominates the mechanical properties of white cast irons, rendering them hard, but unresistant to shock. The broken surface of a white cast iron is full of fine facets of the broken carbide, a very pale, silvery, shiny material, hence the appellation. In grey iron the carbon exists free as fine flakes of graphite, and also renders the material brittle due to the stress-raising nature of the sharp edged flakes of graphite. A newer variant of grey iron, referred to as ductile iron is specially treated with trace amounts of magnesium to alter the shape of graphite to spheroids, or nodules, vastly increasing the toughness and strength of the material.
- Carbon steel contains 2.0% carbon or less,[11] with small amounts of manganese, sulfur, phosphorus, and silicon.
- Wrought iron contains less than 0.25% carbon.[10] It is a tough, malleable product, but not as fusible as pig iron. If honed to an edge, it loses it quickly.[citation needed] Wrought iron is characterised by the presence of fine fibers of slag entrapped in the metal. Wrought iron is more corrosion resistant than steel. It has been almost completely replaced by mild steel for traditional "wrought iron" products and blacksmithing. Mild steel does not have the same corrosion resistance but is cheaper and more widely available.
- Alloy steels contain varying amounts of carbon as well as other metals, such as chromium, vanadium, molybdenum, nickel, tungsten, etc. They are used for structural purposes, as their alloy content raises their cost and necessitates justification of their use. Recent developments in ferrous metallurgy have produced a growing range of microalloyed steels, also termed 'HSLA' or high-strength, low alloy steels, containing tiny additions to produce high strengths and often spectacular toughness at minimal cost.
- Iron(III) oxides are used in the production of magnetic storage media in computers. They are often mixed with other compounds, and retain their magnetic properties in solution.
The main disadvantage of iron and steel is that pure iron, and most of its alloys, suffer badly from rust if not protected in some way. Painting, galvanization, passivation, plastic coating and bluing are some techniques used to protect iron from rust by excluding water and oxygen or by sacrificial protection.
Iron is believed to be the critical missing nutrient in the ocean that limits the growth of plankton. Experimental iron fertilization of areas of the ocean using iron(II) sulfate has proven successful in increasing plankton growth.[12][13][14] Larger scaled efforts are being attempted with the hope that iron seeding and ocean plankton growth can remove carbon dioxide from the atmosphere, thereby counteracting the greenhouse effect that is generally agreed by climatologists to cause global warming.[15]
Iron compounds
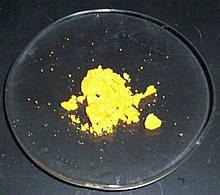
- Iron(III) ammonium oxalate (Fe(NH4)3(C2O4)4) is used in blueprints.
- Iron(III) arsenate (FeAsO4) is used in insecticide.
- Iron(III) chloride (FeCl3) is used: in water purification and sewage treatment, in the dyeing of cloth, as a coloring agent in paints, as an additive in animal feed, and as an etching material for engravement, photography and printed circuits.
- Iron(III) chromate (Fe2(CrO4)3) is used as a yellow pigment for paints and ceramic.
- Iron(III) hydroxide (Fe(OH)3) is used as a brown pigment for rubber and in water purification systems.
- Iron(III) phosphate (FePO4) is used in fertilizer and as an additive in human and animal food.
- Iron(II) acetate (Fe(C2H3O2)2 is used in the dyeing of fabrics and leather, and as a wood preservative.
- Iron(II) gluconate (Fe(C6H11O7)2) is used as a dietary supplement in iron pills.
- Iron(II) oxalate (FeC2O4) is used as yellow pigment for paints, plastics, glass and ceramic, and in photography.
- Iron(II) sulfate (FeSO4) is used in water purification and sewage treatment systems, as a catalyst in the production of ammonia, as an ingredient in fertilizer and herbicide, as an additive in animal feed, in wood preservative and as an additive to flour to increase iron levels.
- Iron-Fluorine complex (FeF6)3- is found in solutions containing both Fe(III) ions and fluoride ions.
Historical aspects

The first iron used by mankind during prehistory came from meteors. The smelting of iron in bloomeries probably began in Anatolia or the Caucasus in the second millennium BC or the latter part of the preceding one. Cast iron was first produced in China about 550 BC, but not in Europe until the medieval period. During the medieval period, means were found in Europe of producing wrought iron from cast iron (in this context known as pig iron) using finery forges. For all these processes, charcoal was required as fuel.
Steel (with a smaller carbon content than pig iron but more than wrought iron) was first produced in antiquity. New methods of producing it by carburizing bars of iron in the cementation process were devised in the 17th century AD. In the Industrial Revolution, new methods of producing bar iron without charcoal were devised and these were later applied to produce steel. In the late 1850s, Henry Bessemer invented a new steelmaking process, involving blowing air through molten pig iron, to produce mild steel. This and other 19th century and later processes have led to wrought iron no longer being produced.
Production of iron from iron ore
The production of iron or steel is a process unless the desired final product is cast iron. The first stage is to produce pig iron in a blast furnace. The second is to make wrought iron or steel from pig iron by a further process.
Blast furnace


Ninety percent of all mining of metallic ores is for the extraction of iron. Industrially, iron is produced starting from iron ores, principally haematite (nominally Fe2O3) and magnetite (Fe3O4) by a carbothermic reaction (reduction with carbon) in a blast furnace at temperatures of about 2000 °C. In a blast furnace, iron ore, carbon in the form of coke, and a flux such as limestone (which is used to remove impurities in the ore which would otherwise clog the furnace with solid material) are fed into the top of the furnace, while a blast of heated air is forced into the furnace at the bottom.
In the furnace, the coke reacts with oxygen in the air blast to produce carbon monoxide:
The carbon monoxide reduces the iron ore (in the chemical equation below, hematite) to molten iron, becoming carbon dioxide in the process:
The flux is present to melt impurities in the ore, principally silicon dioxide sand and other silicates. Common fluxes include limestone (principally calcium carbonate) and dolomite (calcium-magnesium carbonate). Other fluxes may be used depending on the impurities that need to be removed from the ore. In the heat of the furnace the limestone flux decomposes to calcium oxide (quicklime):
Then calcium oxide combines with silicon dioxide to form a slag.
The slag melts in the heat of the furnace. In the bottom of the furnace, the molten slag floats on top of the more dense molten iron, and apertures in the side of the furnace are opened to run off the iron and the slag separately. The iron once cooled, is called pig iron, while the slag can be used as a material in road construction or to improve mineral-poor soils for agriculture.[citation needed]
In 2005, approximately 1,544 Mt (million metric tons) of iron ore was produced worldwide. China was the top producer of iron ore with at least one-fourth world share followed by Brazil, Australia and India, reports the British Geological Survey.
Further processes
Pig iron is not pure iron, but has 4-5% carbon dissolved in it. This is subsequently reduced to steel or commercially pure iron, known as wrought iron, using other furnaces or converters.
Isotopes
Naturally occurring iron consists of four isotopes: 5.845% of radioactive 54Fe (half-life: >3.1×1022 years), 91.754% of stable 56Fe, 2.119% of stable 57Fe and 0.282% of stable 58Fe. 60Fe is an extinct radionuclide of long half-life (1.5 million years).
Much of the past work on measuring the isotopic composition of Fe has centered on determining 60Fe variations due to processes accompanying nucleosynthesis (i.e., meteorite studies) and ore formation. In the last decade however, advances in mass spectrometry technology have allowed the detection and quantification of minute, naturally occurring variations in the ratios of the stable isotopes of iron. Much of this work has been driven by the Earth and planetary science communities, although applications to biological and industrial systems are beginning to emerge.[16]
The isotope 56Fe is of particular interest to nuclear scientists. A common misconception is that this isotope represents the most stable nucleus possible, and that it thus would be impossible to perform fission or fusion on 56Fe and still liberate energy. This is not true, as both 62Ni and 58Fe are more stable, being the most stable nuclei. However, since 56Fe is much more easily produced from lighter nuclei in nuclear reactions, it is the endpoint of fusion chains inside extremely massive stars and is therefore common in the universe, relative to other metals.
In phases of the meteorites Semarkona and Chervony Kut a correlation between the concentration of 60Ni, the daughter product of 60Fe, and the abundance of the stable iron isotopes could be found which is evidence for the existence of 60Fe at the time of formation of the solar system. Possibly the energy released by the decay of 60Fe contributed, together with the energy released by decay of the radionuclide 26Al, to the remelting and differentiation of asteroids after their formation 4.6 billion years ago. The abundance of 60Ni present in extraterrestrial material may also provide further insight into the origin of the solar system and its early history. Of the stable isotopes, only 57Fe has a nuclear spin (−1/2).
Iron in organic synthesis
The use of iron metal filings in organic synthesis is mainly for the reduction of nitro compounds.[17] Additionally, iron has been used for desulfurizations,[18] reduction of aldehydes,[19] and the deoxygenation of amine oxides.[20]
Iron in biology
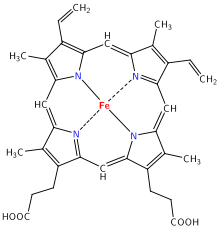
Iron is essential to nearly all known organisms. In cells, iron is generally stored in the centre of metalloproteins, because "free" iron -- which binds non-specifically to many cellular components -- can catalyse production of toxic free radicals.
In animals, plants, and fungi, iron is often incorporated into the heme complex. Heme is an essential component of cytochrome proteins, which mediate redox reactions, and of oxygen carrier proteins such as hemoglobin, myoglobin, and leghemoglobin. Inorganic iron also contributes to redox reactions in the iron-sulfur clusters of many enzymes, such as nitrogenase (involved in the synthesis of ammonia from nitrogen and hydrogen) and hydrogenase. Non-heme iron proteins include the enzymes methane monooxygenase (oxidizes methane to methanol), ribonucleotide reductase (reduces ribose to deoxyribose; DNA biosynthesis), hemerythrins (oxygen transport and fixation in marine invertebrates) and purple acid phosphatase (hydrolysis of phosphate esters).
Iron distribution is heavily regulated in mammals, partly because iron has a high potential for biological toxicity. Iron distribution is also regulated because many bacteria require iron, so restricting its availability to bacteria (generally by sequestering it inside cells) can help to prevent or limit infections. This is probably the reason for the relatively low amounts of iron in mammalian milk. A major component of this regulation is the protein transferrin, which binds iron absorbed from the duodenum and carries it in the blood to cells.[21]
Nutrition and dietary sources
Good sources of dietary iron include red meat, fish, poultry, lentils, beans, leaf vegetables, tofu, chickpeas, black-eyed peas, potatoes with skin, bread made from completely whole-grain flour, molasses, teff and farina. Iron in meat is more easily absorbed than iron in vegetables.[22]
Iron provided by dietary supplements is often found as iron (II) fumarate, although iron sulfate is cheaper and is absorbed equally well. Elemental iron, despite being absorbed to a much smaller extent (stomach acid is sufficient to convert some of it to ferrous iron), is often added to foods such as breakfast cereals or "enriched" wheat flour (where it is listed as "reduced iron" in the list of ingredients). Iron is most available to the body when chelated to amino acids - iron in this form is ten to fifteen times more bioavailable[23] than any other, and is also available for use as a common iron supplement. Often the amino acid chosen for this purpose is the cheapest and most common amino acid, glycine, leading to "iron glycinate" supplements.[24] The RDA for iron varies considerably based on age, gender, and source of dietary iron (heme-based iron has higher bioavailability).[25] Infants will require iron supplements if they are not breast-fed. Blood donors are at special risk of low iron levels and are often advised to supplement their iron intake.
Regulation of iron uptake
Excessive iron can be toxic, because free ferrous iron reacts with peroxides to produce free radicals, which are highly reactive and can damage DNA, proteins, lipids, and other cellular components. Thus, iron toxicity occurs when there is free iron in the cell, which generally occurs when iron levels exceed the capacity of transferrin to bind the iron.
Iron uptake is tightly regulated by the human body, which has no physiological means of excreting iron, so controls iron levels solely by regulating uptake. Although uptake is regulated, large amounts of ingested iron can cause excessive levels of iron in the blood, because high iron levels can cause damage to the cells of the gastrointestinal tract that prevents them from regulating iron absorption. High blood concentrations of iron damage cells in the heart, liver and elsewhere, which can cause serious problems, including long-term organ damage and even death.
Humans experience iron toxicity above 20 milligrams of iron for every kilogram of mass, and 60 milligrams per kilogram is a lethal dose.[26] Over-consumption of iron, often the result of children eating large quantities of ferrous sulfate tablets intended for adult consumption, is one of the most common toxicological causes of death in children under six.[26] The DRI lists the Tolerable Upper Intake Level (UL) for adults as 45 mg/day. For children under fourteen years old the UL is 40 mg/day.
Regulation of iron uptake is impaired in some people as a result of a genetic defect that maps to the HLA-H gene region on chromosome 6. In these people, excessive iron intake can result in iron overload disorders, such as hemochromatosis. Many people have a genetic susceptibility to iron overload without realizing it or being aware of a family history of the problem. For this reason, it is advised that people should not take iron supplements unless they suffer from iron deficiency and have consulted a doctor. Hemochromatosis is estimated to cause disease in between 0.3 and 0.8% of Caucasians. [27]
The medical management of iron toxicity is complex, and can include use of a specific chelating agent called deferoxamine to bind and expel excess iron from the body.
Bibliography
- Los Alamos National Laboratory — Iron
- H. R. Schubert, History of the British Iron and Steel Industry ... to 1775 AD (Routledge, London, 1957)
- R. F. Tylecote, History of Metallurgy (Institute of Materials, London 1992).
- R. F. Tylecote, 'Iron in the Industrial Revolution' in J. Day and R. F. Tylecote, The Industrial Revolution in Metals (Institute of Materials 1991), 200-60.
- Crystal structure of iron
References
- ^ "Standard Atomic Weights: Iron". CIAAW. 1993.
- ^ Prohaska, Thomas; Irrgeher, Johanna; Benefield, Jacqueline; Böhlke, John K.; Chesson, Lesley A.; Coplen, Tyler B.; Ding, Tiping; Dunn, Philip J. H.; Gröning, Manfred; Holden, Norman E.; Meijer, Harro A. J. (2022-05-04). "Standard atomic weights of the elements 2021 (IUPAC Technical Report)". Pure and Applied Chemistry. doi:10.1515/pac-2019-0603. ISSN 1365-3075.
- ^ a b c Arblaster, John W. (2018). Selected Values of the Crystallographic Properties of Elements. Materials Park, Ohio: ASM International. ISBN 978-1-62708-155-9.
- ^ Ram, R. S.; Bernath, P. F. (2003). "Fourier transform emission spectroscopy of the g4Δ–a4Δ system of FeCl". Journal of Molecular Spectroscopy. 221 (2): 261. Bibcode:2003JMoSp.221..261R. doi:10.1016/S0022-2852(03)00225-X.
- ^ Demazeau, G.; Buffat, B.; Pouchard, M.; Hagenmuller, P. (1982). "Recent developments in the field of high oxidation states of transition elements in oxides stabilization of six-coordinated Iron(V)". Zeitschrift für anorganische und allgemeine Chemie. 491: 60–66. doi:10.1002/zaac.19824910109.
- ^ Lu, J.; Jian, J.; Huang, W.; Lin, H.; Li, J; Zhou, M. (2016). "Experimental and theoretical identification of the Fe(VII) oxidation state in FeO4−". Physical Chemistry Chemical Physics. 18 (45): 31125–31131. Bibcode:2016PCCP...1831125L. doi:10.1039/C6CP06753K. PMID 27812577.
- ^ Cardarelli, François (2008). Materials Handbook: A Concise Desktop Reference. London: Springer. p. 65. ISBN 1-84628-668-9.
- ^ Kondev, F. G.; Wang, M.; Huang, W. J.; Naimi, S.; Audi, G. (2021). "The NUBASE2020 evaluation of nuclear properties" (PDF). Chinese Physics C. 45 (3): 030001. doi:10.1088/1674-1137/abddae.
- ^ Iron and Nickel Abundances in H~II Regions and Supernova Remnants
- ^ a b Camp, James McIntyre (1920). The Making, Shaping and Treating of Steel. Pittsburgh: Carnegie Steel Company. pp. 173–174.
{{cite book}}
: Unknown parameter|coauthor=
ignored (|author=
suggested) (help) - ^ Classification of Carbon and Low-Alloy Steels, retrieved January 5, 2008
- ^ Vivian Marx (2002). "The Little Plankton That Could…Maybe". Scientific American.
- ^ Melinda Ferguson, David Labiak, Andrew Madden, Joseph Peltier. "The Effect of Iron on Plankton Use of CO2". CEM 181H. Retrieved 2007-05-05.
{{cite web}}
: CS1 maint: multiple names: authors list (link) - ^ Dopyera, Caroline (October, 1996). "The Iron Hypothesis". EARTH. Retrieved 2007-05-05.
{{cite web}}
: Check date values in:|date=
(help) - ^ O'Conner, Steve (2007-05-03). "Researchers 'seed' ocean with iron to soak up CO2". THE INDEPENDENT. Retrieved 2007-05-05.
{{cite news}}
: Check date values in:|date=
(help) - ^ Dauphas, N. & Rouxel, O. 2006. Mass spectrometry and natural variations of iron isotopes. Mass Spectrometry Reviews, 25, 515-550
- ^ Fox, B. A.; Threlfall, T. L. Organic Syntheses, Coll. Vol. 5, p.346 (1973); Vol. 44, p.34 (1964). (Article)
- ^ Blomquist, A. T.; Dinguid, L. I. J. Org. Chem. 1947, 12, 718 & 723.
- ^ Clarke, H. T.; Dreger, E. E. Org. Syn., Coll. Vol. 1, p.304 (1941); Vol. 6, p.52 (1926). (Article).
- ^ den Hertog, J.; Overhoff, J. Recl. Trav. Chim. Pays-Bas 1950, 69, 468.
- ^ Tracey A. Rouault. "How Mammals Acquire and Distribute Iron Needed for Oxygen-Based Metabolism". Retrieved 2006-06-19.
- ^ Food Standards Agency - Eat well, be well - Iron deficiency
- ^ Pineda O, Ashmead HD (2001). "Effectiveness of treatment of iron-deficiency anemia in infants and young children with ferrous bis-glycinate chelate". Nutrition. 17 (5): 381–4. PMID 11377130.
- ^ Ashmead, H. DeWayne (1989). Conversations on Chelation and Mineral Nutrition. Keats Publishing. ISBN 0-87983-501-X.
{{cite book}}
: Cite has empty unknown parameter:|coauthors=
(help) - ^ "Dietary Reference Intakes: Elements" (PDF).
- ^ a b "Toxicity, Iron". Emedicine. Retrieved 2006-06-19.
- ^ Durupt S, Durieu I, Nove-Josserand R, et al: [Hereditary hemochromatosis]. Rev Med Interne 2000 Nov; 21(11): 961-71[Medline].
- Doulias PT, Christoforidis S, Brunk UT, Galaris D. Endosomal and lysosomal effects of desferrioxamine: protection of HeLa cells from hydrogen peroxide-induced DNA damage and induction of cell-cycle arrest. Free Radic Biol Med. 2003;35:719-28.
See also


- El Mutún in Bolivia, where 20% of the world's accessible iron and magnesium is located
- Iron (metaphor)
- Iron Age
- Iron fertilization - Fertilization of oceans to stimulate phytoplankton growth
- Pelletizing - Process of creation of iron ore pellets
- Al-Hadid (Iron) in the Qur'an
- Specht Building - A historic landmark in Omaha, Nebraska utilizing an iron facade.
- Iron in mythology
External links
Template:Link FA Template:Link FA Template:Link FA Template:Link FA