TRAPPIST-1
Observation data Epoch J2000 Equinox J2000 | |
---|---|
Constellation | Aquarius |
Right ascension | 23h 06m 29.368s[1] |
Declination | −05° 02′ 29.04″[1] |
Apparent magnitude (V) | 18.798±0.082[2] |
Characteristics | |
Evolutionary stage | Main sequence |
Spectral type | M8V[3] |
Apparent magnitude (R) | 16.466±0.065[2] |
Apparent magnitude (I) | 14.024±0.115[2] |
Apparent magnitude (J) | 11.354±0.022[4] |
Apparent magnitude (H) | 10.718±0.021[4] |
Apparent magnitude (K) | 10.296±0.023[4] |
V−R color index | 2.332 |
R−I color index | 2.442 |
J−H color index | 0.636 |
J−K color index | 1.058 |
Astrometry | |
Proper motion (μ) | RA: 930.788[1] mas/yr Dec.: −479.038[1] mas/yr |
Parallax (π) | 80.2123 ± 0.0716 mas[1] |
Distance | 40.66 ± 0.04 ly (12.47 ± 0.01 pc) |
Details | |
Mass | 0.0898±0.0023[5] M☉ |
Radius | 0.1192±0.0013[5] R☉ |
Luminosity (bolometric) | 0.000553±0.000018[5] L☉ |
Surface gravity (log g) | 5.2396+0.0056 −0.0073[a][5] cgs |
Temperature | 2,566±26[5] K |
Metallicity [Fe/H] | 0.04±0.08[6] dex |
Rotation | 3.295±0.003 days[7] |
Rotational velocity (v sin i) | 6[8] km/s |
Age | 7.6±2.2[9] Gyr |
Other designations | |
Database references | |
SIMBAD | data |
Exoplanet Archive | data |
TRAPPIST-1 is a cool red dwarf star[c] with seven known exoplanets. It lies in the constellation Aquarius about 40.66 light-years away from Earth, and has a surface temperature of about 2,566 K (2,290 °C; 4,160 °F). Its radius is slightly larger than Jupiter and it has a mass of about 9% of the Sun. It is estimated to be 7.6 billion years old, making it older than the Solar System. The discovery of the star was first published in 2000.
Observations in 2016 from the Transiting Planets and Planetesimals Small Telescope (TRAPPIST) at La Silla Observatory in Chile and other telescopes led to the discovery of two terrestrial planets in orbit around TRAPPIST-1. In 2017, further analysis of the original observations identified five more terrestrial planets. It takes the seven planets between about 1.5 and 19 days to orbit around the star in circular orbits. They are likely tidally locked to TRAPPIST-1, such that one side of each planet always faces the star, leading to permanent day on one side and permanent night on the other. Their masses are comparable to that of Earth and they all lie in the same plane; from Earth they seem to move past the disk of the star.
Up to four of the planets – designated d, e, f and g – orbit at distances where temperatures are suitable for the existence of liquid water, and are thus potentially hospitable to life. There is no evidence of an atmosphere on any of the planets, and observations of TRAPPIST-1 b have ruled out the existence of an atmosphere. It is unclear whether radiation emissions from TRAPPIST-1 would allow for such atmospheres. The planets have low densities; they may consist of large amounts of volatile materials. Due to the possibility of several of the planets being habitable, the system has drawn interest from researchers and has appeared in popular culture.
Discovery
[edit]The star now known as TRAPPIST-1 was discovered in 1999 by astronomer John Gizis and colleagues[16] during a survey of close-by ultra-cool dwarf stars.[17][18] It appeared in sample C[16][17] of the surveyed stars, which was obtained in June 1999. Publication of the discovery took place in 2000.[19] The name is a reference to the TRansiting Planets and PlanetesImals Small Telescope (TRAPPIST)[11][d] project that discovered the first two exoplanets around the star.[23]
Its planetary system was discovered by a team led by Michaël Gillon, a Belgian astronomer[24] at the University of Liege,[25] in 2016[26] during observations made at the La Silla Observatory, Chile,[27][28] using the TRAPPIST telescope. The discovery was based on anomalies in the light curves[e] measured by the telescope in 2015. These were initially interpreted as indicating the existence of three planets. In 2016, separate discoveries revealed that the third planet was in fact multiple planets. The telescopes and observatories involved were[11] the Spitzer Space Telescope and the ground-based TRAPPIST, TRAPPIST-North in Oukaïmeden Observatory, Morocco, the South African Astronomical Observatory, and the Liverpool Telescopes and William Herschel Telescopes in Spain.[30]
The observations of TRAPPIST-1 are considered among the most important research findings of the Spitzer Space Telescope.[31] Complementing the findings were observations by the Himalayan Chandra Telescope, the United Kingdom Infrared Telescope, and the Very Large Telescope.[32] Since then, research has confirmed the existence of at least seven planets in the system,[33] the orbits of which have been calculated using measurements from the Spitzer and Kepler telescopes.[34] Some news reports incorrectly attributed the discovery of the TRAPPIST-1 planets to NASA; in fact the TRAPPIST project that led to their discovery received funding from both NASA and the European Research Council of the European Union (EU).[35]
Description
[edit]
TRAPPIST-1 is in the constellation Aquarius,[25] five degrees south of the celestial equator.[f][1][37] It is a relatively close star[38] located 40.66±0.04 light-years from Earth,[g][1] with a large proper motion[h][38] and no companion stars.[41]
It is a red dwarf of spectral class M8.0±0.5,[i][32][44] meaning it is relatively small and cold.[45] With a radius 12% of that of the Sun, TRAPPIST-1 is only slightly larger than the planet Jupiter (though much more massive).[32] Its mass is approximately 9% of that of the Sun,[45] being just sufficient to allow nuclear fusion to take place.[46][47] TRAPPIST-1's density is unusually low for a red dwarf.[48] It has a low effective temperature[j] of 2,566 K (2,293 °C) making it, as of 2022[update], the coldest-known star to host planets.[50] TRAPPIST-1 is cold enough for condensates to form in its photosphere;[k] these have been detected through the polarisation they induce in its radiation during transits of its planets.[52]
There is no evidence that it has a stellar cycle.[l][54] Its luminosity, emitted mostly as infrared radiation, is about 0.055% that of the Sun.[45][55] Low precision[56] measurements from the XMM-Newton satellite[57] and other facilities[58] show that the star emits faint radiation at short wavelengths such as x-rays and UV radiation.[m][57] There are no detectable radio wave emissions.[60]
Rotation period and age
[edit]Measurements of TRAPPIST-1's rotation have yielded a period of 3.3 days; earlier measurements of 1.4 days appear to have been caused by changes in the distribution of its starspots.[61] Its rotational axis may be slightly offset from that of its planets.[62]
Using a combination of techniques, the age of TRAPPIST-1 has been estimated at about 7.6±2.2 billion years,[63] making it older than the Solar System, which is about 4.5 billion years old.[64] It is expected to shine for ten trillion years – about 700 times[65] longer than the present age of the Universe[66] – whereas the Sun will run out of hydrogen and leave the main sequence[n] in a few billion years.[65]
Activity
[edit]Photospheric features have been detected on TRAPPIST-1.[68] The Kepler and Spitzer Space Telescopes have observed possible bright spots, which may be faculae,[o][70][71] although some of these may be too large to qualify as such.[72] Bright spots are correlated to the occurrence of some stellar flares.[p][74]
The star has a strong magnetic field[75] with a mean intensity of about 600 gauss.[q][77] The magnetic field drives high chromospheric[r][75] activity, and may be capable of trapping coronal mass ejections.[s][69][78]
According to Garraffo et al. (2017), TRAPPIST-1 loses about 3×10−14 solar masses per year[79] to the stellar wind, a rate which is about 1.5 times that of the Sun.[80] Dong et al. (2018) simulated the observed properties of TRAPPIST-1 with a mass loss of 4.1×10−15 solar masses per year.[79] Simulations to estimate mass loss are complicated because, as of 2019, most of the parameters that govern TRAPPIST-1's stellar wind are not known from direct observation.[81]
Planetary system
[edit]
TRAPPIST-1 is orbited by seven planets, designated TRAPPIST-1b, 1c, 1d, 1e, 1f, 1g, and 1h[82] in alphabetic order going out from the star.[t][85] These planets have orbital periods ranging from 1.5 to 19 days,[86][87][6] at distances of 0.011–0.059 astronomical units[u] (1,700,000–8,900,000 km).[89]
All the planets are much closer to their star than Mercury is to the Sun,[26] making the TRAPPIST-1 system very compact.[90] Kral et al. (2018) did not detect any comets around TRAPPIST-1,[91] and Marino et al. (2020) found no evidence of a Kuiper belt,[92] although it is uncertain whether a Solar System-like belt around TRAPPIST-1 would be observable from Earth.[93] Observations with the Atacama Large Millimeter Array found no evidence of a circumstellar dust disk.[94]
The inclinations of planetary orbits relative to the system's ecliptic are less than 0.1 degrees,[v][96] making TRAPPIST-1 the flattest planetary system in the NASA Exoplanet Archive.[97] The orbits are highly circular, with minimal eccentricities[w][90] and are well-aligned with the spin axis of TRAPPIST-1.[99] The planets orbit in the same plane and, from the perspective of the Solar System, transit TRAPPIST-1 during their orbit[100] and frequently pass in front of each other.[101]
Size and composition
[edit]The radii of the planets are estimated to range between 77.5+1.4
−1.4 and 112.9+1.5
−1.3% of Earth's radius.[102] The planet/star mass ratio of the TRAPPIST-1 system resembles that of the moon/planet ratio of the Solar System's gas giants.[103]
The TRAPPIST-1 planets are expected to have compositions that resemble each other[104] as well as that of Earth.[105] The estimated densities of the planets are lower than Earth's[34] which may imply that they have large amounts of volatile chemicals.[x] Alternatively, their cores may be smaller than that of Earth and therefore they may be rocky planets with less iron than that of Earth,[107][108] include large amounts of elements other than iron,[109] or their iron may exist in an oxidised form rather than as a core.[108] Their densities are too low for a pure magnesium silicate composition,[y] requiring the presence of lower-density compounds such as water.[111][112] Planets b, d, f, g and h are expected to contain large quantities of volatile chemicals.[113] The planets may have deep atmospheres and oceans, and contain vast amounts of ice.[114] Subsurface oceans, buried under icy shells, would form in the colder planets.[115] Several compositions are possible considering the large uncertainties in their densities.[116] The photospheric features of the star may introduce inaccuracies in measurements of the properties of TRAPPIST-1's planets,[68] including their densities being underestimated by 8+20
-7 percent,[117] and incorrect estimates of their water content.[118]
Resonance and tides
[edit]The planets are in orbital resonances.[119] The durations of their orbits have ratios of 8:5, 5:3, 3:2, 3:2, 4:3 and 3:2 between neighbouring planet pairs,[120] and each set of three is in a Laplace resonance.[z][90] Simulations have shown such resonances can remain stable over billions of years but that their stability is strongly dependent on initial conditions. Many configurations become unstable after less than a million years. The resonances enhance the exchange of angular momentum between the planets, resulting in measurable variations – earlier or later – in their transit times in front of TRAPPIST-1. These variations yield information on the planetary system,[122] such as the masses of the planets, when other techniques are not available.[123] The resonances and the proximity to the host star have led to comparisons between the TRAPPIST-1 system and the Galilean moons of Jupiter.[100] Kepler-223 is another exoplanet system with a TRAPPIST-1-like long resonance.[124]
The mutual interactions of the planets could prevent them from reaching full synchronisation, which would have important implications for the planets' climates. These interactions could force periodic or episodic full rotations of the planets' surfaces with respect to the star on timescales of several Earth years.[125] Vinson, Tamayo and Hansen (2019) found the planets TRAPPIST-1d, e and f likely have chaotic rotations due to mutual interactions, preventing them from becoming synchronised to their star. Lack of synchronisation potentially makes the planets more habitable.[126] Other processes that can prevent synchronous rotation are torques induced by stable triaxial deformation of the planets,[aa] which would allow them to enter 3:2 resonances.[128]
The closeness of the planets to TRAPPIST-1 results in tidal interactions[129] stronger than those on Earth.[130] All the planets have reached an equilibrium with slow planetary rotations and tidal locking,[129] which can lead to the synchronisation of a planet's rotation to its revolution around its star.[ab][132]
The planets are likely to undergo substantial tidal heating[133] due to deformations arising from their orbital eccentricities and gravitational interactions with one another.[134] Such heating would facilitate volcanism and degassing[ac] especially on the innermost planets, with degassing facilitating the establishment of atmospheres.[136] According to Luger et al. (2017), tidal heating of the four innermost planets is expected to be greater than Earth's inner heat flux.[137] For the outer planets Quick et al. (2020) noted that their tidal heating could be comparable to that in the Solar System bodies Europa, Enceladus, and Triton,[138] and may be sufficient to drive detectable cryovolcanic activity.[139]
Tidal heating could influence temperatures of the night sides and cold areas where volatiles may be trapped, and gases are expected to accumulate; it would also influence the properties of any subsurface oceans[140] where cryovolcanism,[ad][142] volcanism and hydrothermal venting[ae] could occur.[144] It may further be sufficient to melt the mantles of the four innermost planets, in whole or in part,[145] potentially forming subsurface magma oceans.[146] This heat source is likely dominant over radioactive decay, both of which have substantial uncertainties and are considerably less than the stellar radiation received.[147] Intense tides could fracture the planets' crusts even if they are not sufficiently strong to trigger the onset of plate tectonics.[148] Tides can also occur in the planetary atmospheres.[149]
Skies and impact of stellar light
[edit]
Because most of TRAPPIST-1's radiation is in the infrared region, there may be very little visible light on the planets' surfaces; Amaury Triaud, one of the system's co-discoverers, said the skies would never be brighter than Earth's sky at sunset[151] and only a little brighter than a night with a full moon. Ignoring atmospheric effects, illumination would be orange-red.[152] All of the planets would be visible from each other and would, in many cases, appear larger than Earth's Moon in the sky of Earth;[26] observers on TRAPPIST-1e, f and g, however, could never experience a total stellar eclipse.[ag][85] Assuming the existence of atmospheres, the star's long-wavelength radiation would be absorbed to a greater degree by water and carbon dioxide than sunlight on Earth; it would also be scattered less by the atmosphere[153] and less reflected by ice,[154] although the development of highly reflective hydrohalite ice may negate this effect.[155] The same amount of radiation results in a warmer planet compared to Sun-like irradiation;[153] more radiation would be absorbed by the planets' upper atmosphere than by the lower layers, making the atmosphere more stable and less prone to convection.[156]
Habitable zone
[edit]
For a dim star like TRAPPIST-1, the habitable zone[ah] is located closer to the star than for the Sun.[157] Three or four[57] planets might be located in the habitable zone; these include e, f, and g;[157] or d, e, and f.[75] As of 2017[update], this is the largest-known number of planets within the habitable zone of any known star or star system.[158] The presence of liquid water on any of the planets depends on several other factors, such as albedo (reflectivity),[159] the presence of an atmosphere[160] and any greenhouse effect.[161] Surface conditions are difficult to constrain without better knowledge of the planets' atmospheres.[160] A synchronously rotating planet might not entirely freeze over if it receives too little radiation from its star because the day-side could be sufficiently heated to halt the progress of glaciation.[162] Other factors for the occurrence of liquid water include the presence of oceans and vegetation;[163] the reflective properties of the land surface; the configuration of continents and oceans;[164] the presence of clouds;[165] and sea ice dynamics.[166] The effects of volcanic activity may extend the system's habitable zone to TRAPPIST-1h.[167] Even if the outer planets are too cold to be habitable, they may have ice-covered subsurface oceans[168] that may harbour life.[169]
Intense extreme ultraviolet (XUV) and X-ray radiation[170] can split water into its component parts of hydrogen and oxygen, and heat the upper atmosphere until they escape from the planet. This was thought to have been particularly important early in the star's history, when radiation was more intense and could have heated every planet's water to its boiling point.[154] This process is believed to have removed water from Venus.[171] In the case of TRAPPIST-1, different studies with different assumptions on the kinetics, energetics, and XUV emissions have come to different conclusions on whether any TRAPPIST-1 planet may retain substantial amounts of water. Because the planets are most likely synchronised to their host star, any water present could become trapped on the planets' night sides and would be unavailable to support life unless heat transport by the atmosphere[172] or tidal heating are intense enough to melt ice.[173]
Moons
[edit]No moons with a size comparable to Earth's have been detected in the TRAPPIST-1 system,[174] and they are unlikely in such a densely packed planetary system. This is because moons would likely be either destroyed by their planet's gravity after entering its Roche limit[ai] or stripped from the planet by leaving its Hill radius[aj][177] Although the TRAPPIST-1 planets appear in an analysis of potential exomoon hosts, they do not appear in the list of habitable-zone exoplanets that could host a moon for at least one Hubble time,[178] a timeframe slightly longer than the current age of the Universe.[179] Despite these factors, it is possible the planets could host moons.[180]
Magnetic effects
[edit]The TRAPPIST-1 planets are expected to be within the Alfvén surface of their host star,[181] the area around the star within which any planet would directly magnetically interact with the corona of the star, possibly destabilising any atmosphere the planet has.[182] Stellar energetic particles would not create a substantial radiation hazard for organisms on TRAPPIST-1 planets if atmospheres reached pressures of about 1 bar.[183] Estimates of radiation fluxes have considerable uncertainties due to the lack of knowledge about the structure of TRAPPIST-1's magnetic field.[184] Induction heating from the star's time-varying electrical and magnetic fields[145][185] may occur on its planets[186] but this would make no substantial contribution to their energy balance[147] and is vastly exceeded by tidal heating.[138]
Formation history
[edit]The TRAPPIST-1 planets most likely formed further from the star and migrated inwards,[187] although it is possible they formed in their current locations.[188] According to the most popular theory on the formation of the TRAPPIST-1 planets (Ormel et al. (2017)),[189] the planets formed when a streaming instability[ak] at the water-ice line gave rise to precursor bodies, which accumulated additional fragments and migrated inwards, eventually giving rise to planets.[191] The migration may initially have been fast and later slowed,[192] and tidal effects may have further influenced the formation processes.[193] The distribution of the fragments would have controlled the final mass of the planets, which would consist of approximately 10% water consistent with observational inference.[191] Resonant chains of planets like those of TRAPPIST-1 usually become unstable when the gas disk that gave rise to them dissipates, but in this case, the planets remained in resonance.[194] The resonance may have been either present from the system's formation and was preserved when the planets simultaneously moved inwards,[195] or it might have formed later when inward-migrating planets accumulated at the outer edge of the gas disk and interacted with each other.[188] Inward-migrating planets would contain substantial amounts of water – too much for it to entirely escape – whereas planets that formed in their current location would most likely lose all water.[196][197] According to Flock et al. (2019), the orbital distance of the innermost planet TRAPPIST-1b is consistent with the expected radius of an inward-moving planet around a star that was one order of magnitude brighter in the past,[198] and with the cavity in the protoplanetary disc created by TRAPPIST-1's magnetic field.[199] Alternatively, TRAPPIST-1h may have formed in or close to its current location.[200]
The presence of other bodies and planetesimals early in the system's history would have destabilised the TRAPPIST-1 planets' resonance if the bodies were massive enough.[201] Raymond et al. (2021) concluded the TRAPPIST-1 planets assembled in 1–2 million years, after which time little additional mass was accreted.[202] This would limit any late delivery of water to the planets[203] and also implies the planets cleared the neighbourhood[al] of any additional material.[204] The lack of giant impact events (the rapid formation of the planets would have quickly exhausted pre-planetary material) would help the planets preserve their volatile materials,[205] only once the planet formation process was complete.[206]
Due to a combination of high insolation, the greenhouse effect of water vapour atmospheres and remnant heat from the process of planet assembly, the TRAPPIST-1 planets would likely have initially had molten surfaces. Eventually the surfaces would cool until the magma oceans solidified, which in the case of TRAPPIST-1b may have taken between a few billions of years, or a few millions of years. The outer planets would then have become cold enough for water vapour to condense.[207]
List of planets
[edit]TRAPPIST-1b
[edit]TRAPPIST-1b has a semi-major axis of 0.0115 astronomical units (1,720,000 km)[208] and an orbital period of 1.51 Earth days. It is tidally locked to its star. The planet is outside the habitable zone;[209] its expected irradiation is more than four times that of Earth[209] and the James Webb Space Telescope (JWST) has measured a brightness temperature of 508+26
−27 K on the day side.[210] TRAPPIST-1b has a slightly larger measured radius and mass than Earth but estimates of its density imply it does not exclusively consist of rock.[211] Owing to its black-body temperature of 124 °C (397 K), TRAPPIST-1b may have had a runaway greenhouse effect similar to that of Venus;[75] JWST observations indicate that it has either no atmosphere at all or one nearly devoid of CO2.[212] Based on several climate models, the planet would have been desiccated by TRAPPIST-1's stellar wind and radiation;[213][214] it could be quickly losing hydrogen and therefore any hydrogen-dominated atmosphere.[am] Water, if any exists, could persist only in specific settings on the planet,[216] whose surface temperature could be as high as 1,200 °C (1,470 K), making TRAPPIST-1b a candidate magma ocean planet.[217] According to JWST observations, the planet has an albedo of about zero.[218]
TRAPPIST-1c
[edit]
TRAPPIST-1c has a semi-major axis of 0.0158 AU (2,360,000 km)[208] and orbits its star every 2.42 Earth days. It is close enough to TRAPPIST-1 to be tidally locked.[209] JWST observations have ruled out the existence of CO2-rich atmospheres,[219] Venus-like atmospheres, but water vapour- or oxygen-rich atmospheres or no-atmosphere scenarios are possible.[220] These data imply that relative to Earth or Venus, TRAPPIST-1 c has a lower carbon content.[221] TRAPPIST-1c is outside the habitable zone[209] as it receives about twice as much stellar irradiation as Earth[222] and thus either is or has been a runaway greenhouse.[75] Based on several climate models, the planet would have been desiccated by TRAPPIST-1's stellar wind and radiation.[213] TRAPPIST-1c could harbour water only in specific settings on its surface.[216] Observations in 2017 showed no escaping hydrogen,[58] but observations by the Hubble Space Telescope (HST) in 2020 indicated that hydrogen may be escaping at a rate of 1.4×107 g/s.[215]
TRAPPIST-1d
[edit]TRAPPIST-1d has a semi-major axis of 0.022 AU (3,300,000 km) and an orbital period of 4.05 Earth days. It is more massive but less dense than Mars.[223] Based on fluid dynamical arguments, TRAPPIST-1d is expected to have weak temperature gradients on its surface if it is tidally locked,[224] and may have significantly different stratospheric dynamics than that of Earth.[225] Several climate models suggest that the planet may[213] or may not have been desiccated by TRAPPIST-1's stellar wind and radiation;[213] density estimates, if confirmed, indicate it is not dense enough to consist solely of rock.[211] The current state of TRAPPIST-1d depends on its rotation and climatic factors like cloud feedback;[an][227] it is close to the inner edge of the habitable zone, but the existence of either liquid water or alternatively a runaway greenhouse effect (that would render it uninhabitable) are dependent on detailed atmospheric conditions.[228] Water could persist in specific settings on the planet.[216]
TRAPPIST-1e
[edit]TRAPPIST-1e has a semi-major axis of 0.029 AU (4,300,000 km)[208] and orbits its star every 6.10 Earth days.[229] It has density similar that of Earth.[230] Based on several climate models, the planet is the most likely of the system to have retained its water,[213] and the most likely to have liquid water for many climate states. A dedicated climate model project called TRAPPIST-1 Habitable Atmosphere Intercomparison (THAI) has been launched to study its potential climate states.[231] Based on observations of its Lyman-alpha radiation emissions, TRAPPIST-1e may be losing hydrogen at a rate of 0.6×107 g/s.[215]
TRAPPIST-1e is in a comparable position within the habitable zone to that of Proxima Centauri b,[ao][233][234] which also has an Earth-like density.[230] TRAPPIST-1e could have retained masses of water equivalent to several of Earth's oceans.[75] Moderate quantities of carbon dioxide could warm TRAPPIST-1e to temperatures suitable for the presence of liquid water.[214]
TRAPPIST-1f
[edit]TRAPPIST-1f has a semi-major axis of 0.038 AU (5,700,000 km)[208] and orbits its star every 9.21 Earth days.[229] It is likely too distant from its host star to sustain liquid water, being instead an entirely glaciated snowball planet[213] that might host a subsurface ocean.[235] Moderate quantities of CO2 could warm TRAPPIST-1f to temperatures suitable for the presence of liquid water.[216] Lakes or ponds with liquid water might form in places where tidal heating is concentrated.[236] TRAPPIST-1f may have retained masses of water equivalent to several of Earth's oceans[75] and which could comprise up to half of the planet's mass;[237] it could thus be an ocean planet.[ap][239]
TRAPPIST-1g
[edit]TRAPPIST-1g has a semi-major axis of 0.047 AU (7,000,000 km)[208] and orbits its star every 12.4 Earth days.[229] It is likely too distant from its host star to sustain liquid water, being instead a snowball planet[213] that might host a subsurface ocean.[235] Moderate quantities of CO2[216] or internal heat from radioactive decay and tidal heating may warm its surface to above the melting point of water.[240][115] TRAPPIST-1g may have retained masses of water equivalent to several of Earth's oceans;[75] density estimates of the planet, if confirmed, indicate it is not dense enough to consist solely of rock.[211] Up to half of its mass may be water.[237]
TRAPPIST-1h
[edit]TRAPPIST-1h has a semi-major axis of 0.062 astronomical units (9,300,000 km); it is the system's least massive known planet[208] and orbits its star every 18.9 Earth days.[229] It is likely too distant from its host star to sustain liquid water and may be a snowball planet,[213][115] or have a methane/nitrogen atmosphere resembling that of Titan.[241] It might host a subsurface ocean.[235] Large quantities of CO2, hydrogen or methane,[242] or internal heat from radioactive decay and tidal heating,[240] would be needed to warm TRAPPIST-1h to the point where liquid water could exist.[242] TRAPPIST-1h could have retained masses of water equivalent to several of Earth's oceans.[75]
Data table
[edit]Planet | Mass (ME) | Semi-major axis | Orbital period (days) | Orbital eccentricity[87] | Orbital inclination[86] | Radius (R🜨) | Radiant flux[86] | Temperature [87] | Surface gravity (g)[86] | ORb [aq] |
ORi [ar] |
---|---|---|---|---|---|---|---|---|---|---|---|
b | 1.374 ±0.069 |
0.01154 ±0.0001 |
1.510826 ±0.000006 |
0.00622 ±0.00304 |
89.728 ±0.165° |
1.116 +0.014 −0.012 |
4.153 ±0.160 |
397.6±3.8K (124.5 ± 3.8 °C; 256.0 ± 6.8 °F)[as] |
1.102 ±0.052 |
— | — |
c | 1.308 ±0.056 |
0.01580 ±0.00013 |
2.421937 ±0.000018 |
0.00654 ±0.00188 |
89.778 ±0.118° |
1.097 +0.014 −0.012 |
2.214 ±0.085 |
339.7±3.3K (66.6 ± 3.3 °C; 151.8 ± 5.9 °F) |
1.086 ±0.043 |
5:8 | 5:8 |
d | 0.388 ±0.012 |
0.02227 ±0.00019 |
4.049219 ±0.000026 |
0.00837 ±0.00093 |
89.896 ±0.077° |
0.770 +0.011 −0.010 |
1.115 ±0.04 |
286.2±2.8K (13.1 ± 2.8 °C; 55.5 ± 5.0 °F) |
0.624 ±0.019 |
3:8 | 3:5 |
e | 0.692 ±0.022 |
0.02925 ±0.00025 |
6.101013 ±0.000035 |
0.00510 ±0.00058 |
89.793 ±0.048° |
0.920 +0.013 −0.012 |
0.646 ±0.025 |
249.7±2.4K (−23.5 ± 2.4 °C; −10.2 ± 4.3 °F) |
0.817 ±0.024 |
1:4 | 2:3 |
f | 1.039 ±0.031 |
0.03849 ±0.00033 |
9.207540 ±0.000032 |
0.01007 ±0.00068 |
89.740 ±0.019° |
1.045 +0.013 −0.012 |
0.373 ±0.014 |
217.7±2.1K (−55.5 ± 2.1 °C; −67.8 ± 3.8 °F) |
0.951 ±0.024 |
1:6 | 2:3 |
g | 1.321 ±0.038 |
0.04683 ±0.0004 |
12.352446 ±0.000054 |
0.00208 ±0.00058 |
89.742 ±0.012° |
1.129 +0.015 −0.013 |
0.252 ±0.010 |
197.3±1.9K (−75.8 ± 1.9 °C; −104.5 ± 3.4 °F) |
1.035 ±0.026 |
1:8 | 3:4 |
h | 0.326 ±0.020 |
0.06189 ±0.00053 |
18.772866 ±0.000214 |
0.00567 ±0.00121 |
89.805 ±0.013° |
0.775 +0.014 −0.014 |
0.144 ±0.006 |
171.7±1.7K (−101.5 ± 1.7 °C; −150.6 ± 3.1 °F) |
0.570 ±0.038 |
1:12 | 2:3 |
Potential planetary atmospheres
[edit]
As of 2023[update], the existence of an atmosphere around TRAPPIST-1b has been ruled out by James Webb Space Telescope observations, and there is no evidence for the other planets in the system,[at][245] but atmospheres are not ruled out[219][au] and could be detected in the future.[247] The outer planets are more likely to have atmospheres than the inner planets.[187] Several studies have simulated how different atmospheric scenarios would look to observers, and the chemical processes underpinning these atmospheric compositions.[248] The visibility of an exoplanet and of its atmosphere scale with the inverse square of the radius of its host star.[247] Detection of individual components of the atmospheres – in particular CO2, ozone, and water[249] – would also be possible, although different components would require different conditions and different numbers of transits.[250] A contamination of the atmospheric signals through patterns in the stellar photosphere is a further impediment to detection.[251][252]
The existence of atmospheres around TRAPPIST-1's planets depends on the balance between the amount of atmosphere initially present, its rate of evaporation, and the rate at which it is built back up by meteorite impacts[av],[90] incoming material from a protoplanetary disk[aw],[255] and outgassing and volcanic activity.[256] Impact events may be particularly important in the outer planets because they can both add and remove volatiles; addition is likely dominant in the outermost planets where impact velocities are slower.[257][258] The formation conditions of the planets would give them large initial quantities of volatile materials,[187] including oceans over 100 times larger than those of Earth.[259]
If the planets are tidally locked to TRAPPIST-1, surfaces that permanently face away from the star can cool sufficiently for any atmosphere to freeze out on the night side.[260] This frozen-out atmosphere could be recycled through glacier-like flows to the day side with assistance from tidal or geothermal heating from below, or could be stirred by impact events. These processes could allow an atmosphere to persist.[261] In a carbon dioxide (CO2) atmosphere, carbon-dioxide ice is denser than water ice, under which it tends to be buried. CO2-water compounds named clathrates[ax] can form. Further complications are a potential runaway feedback loop between melting ice and evaporation, and the greenhouse effect.[263]
Numerical modelling and observations constrain the properties of hypothetical atmospheres around TRAPPIST-1 planets:[187]
- Theoretical calculations[264] and observations have ruled out the possibility the TRAPPIST-1 planets have hydrogen-rich[239][265] or helium-rich atmospheres.[266] Hydrogen-rich exospheres[ay] may be detectable[268] but have not been reliably detected,[269] except perhaps for TRAPPIST-1b and 1c by Bourrier et al. (2017).[200][14]
- Water-dominated atmospheres, though suggested by some density estimates, are improbable for the planets because they are expected to be unstable under the conditions around TRAPPIST-1, especially early in the star's life.[211] The spectral properties of the planets imply they do not have a cloud-free, water-rich atmosphere.[270]
- Oxygen-dominated atmospheres can form when radiation splits water into hydrogen and oxygen, and the hydrogen escapes due to its lighter mass. The existence of such an atmosphere and its mass depends on the initial water mass, on whether the oxygen is dragged out of the atmosphere by escaping hydrogen and of the state of the planet's surface; a partially molten surface could absorb sufficient quantities of oxygen to remove an atmosphere.[271][272]
- Atmospheres formed by ammonia and/or methane near TRAPPIST-1 would be destroyed by the star's radiation at a sufficient rate to quickly remove an atmosphere. The rate at which ammonia or methane are produced, possibly by organisms, would have to be considerably larger than that on Earth to sustain such an atmosphere. It is possible the development of organic hazes from ammonia or methane photolysis could shield the remaining molecules from degradation caused by radiation.[273] Ducrot et al. (2020) interpreted observational data as implying methane-dominated atmospheres are unlikely around TRAPPIST-1 planets.[274]
- Nitrogen-dominated atmospheres are particularly unstable with respect to atmospheric escape, especially on the innermost planets, although the presence of CO2 may slow evaporation.[275] Unless the TRAPPIST-1 planets initially contained far more nitrogen than Earth, they are unlikely to have retained such atmospheres.[276]
- CO2-dominated atmospheres escape slowly because CO2 effectively radiates away energy and thus does not readily reach escape velocity; on a synchronously rotating planet, however, CO2 can freeze out on the night side, especially if there are no other gases in the atmosphere. The decomposition of CO2 caused by radiation could yield substantial amounts of oxygen, carbon monoxide (CO),[214] and ozone.[277]
Theoretical modelling by Krissansen-Totton and Fortney (2022) suggests the inner planets most likely have oxygen-and-CO2-rich atmospheres, if any.[278] If the planets have an atmosphere, the amount of precipitation, its form and location would be determined by the presence and position of mountains and oceans, and the rotation period.[279] Planets in the habitable zone are expected to have an atmospheric circulation regime resembling Earth's tropical regions with largely uniform temperatures.[280] Whether greenhouse gases can accumulate on the outer TRAPPIST-1 planets in sufficient quantities to warm them to the melting point of water is controversial; on a synchronously rotating planet, CO2 could freeze and precipitate on the night side, and ammonia and methane would be destroyed by XUV radiation from TRAPPIST-1.[75] Carbon dioxide freezing-out can occur only on the outermost planets unless special conditions are met, and other volatiles do not freeze out.[281]
Stability
[edit]
The emission of extreme ultraviolet (XUV) radiation by a star has an important influence on the stability of its planets' atmospheres, their composition and the habitability of their surfaces.[281] It can cause the ongoing removal of atmospheres from planets.[90] XUV radiation-induced atmospheric escape has been observed on gas giants.[282] M dwarfs emit large amounts of XUV radiation;[281] TRAPPIST-1 and the Sun emit about the same amount of XUV radiation[az] and because TRAPPIST-1's planets are much closer to the star than the Sun's, they receive much more intense irradiation.[55] TRAPPIST-1 has been emitting radiation for much longer than the Sun.[284] The process of atmospheric escape has been modelled mainly in the context of hydrogen-rich atmospheres and little quantitative research has been done on those of other compositions such as water and CO2.[265]
TRAPPIST-1 has moderate to high stellar activity[ba],[32] and this may be another difficulty for the persistence of atmospheres and water on the planets:[27]
- Dwarfs of the spectral class M have intense flares;[281] TRAPPIST-1 averages about 0.38 flares per day[75] and four to six superflares[bb] per year.[287] Such flares would have only small impacts on atmospheric temperatures but would substantially affect the stability and chemistry of atmospheres.[90] According to Samara, Patsourakos and Georgoulis (2021), the TRAPPIST-1 planets are unlikely to be able to retain atmospheres against coronal mass ejections.[288]
- The stellar wind from TRAPPIST-1 may have a pressure 1,000 times larger than that of the Sun at Earth's orbit, which could destabilise atmospheres of the star's planets[289] up to planet f. The pressure would push the wind deep into the atmospheres,[213] facilitating loss of water and evaporation of the atmospheres.[90][241] Stellar wind-driven escape in the Solar System is largely independent from planetary properties such as mass,[290] scaling instead with the stellar wind mass flux impacting the planet.[291] Stellar wind from TRAPPIST-1 could remove the atmospheres of its planets on a timescale of 100 million to 10 billion years.[292]
- Ohmic heating[bc] of the atmosphere of TRAPPIST-1e, f, and g amounts to 5–15 times the heating from XUV radiation; if the heat is effectively absorbed, it could destabilise the atmospheres.[294]
The star's history also influences the atmospheres of its planets.[295] Immediately after its formation, TRAPPIST-1 would have been in a pre-main-sequence state, which may have lasted between hundreds of millions[281] and two billion years.[251] While in this state, it would have been considerably brighter than it is today and the star's intense irradiation would have impacted the atmospheres of surrounding planets, vaporising all common volatiles such as ammonia, CO2, sulfur dioxide, and water.[296] Thus, all of the system's planets would have been heated to a runaway greenhouse[bd] for at least part of their existence.[281] The XUV radiation would have been even higher during the pre-main-sequence stage.[90]
Possible life
[edit]Life may be possible in the TRAPPIST-1 system, and some of the star's planets are considered promising targets for its detection.[27] On the basis of atmospheric stability, TRAPPIST-1e is theoretically the planet most likely to harbour life; the probability that it does is considerably less than that of Earth. There are an array of factors at play:[297][298]
- Due to multiple interactions, TRAPPIST-1 planets are expected to have intense tides.[299] If oceans are present,[be] the tides could: lead to alternate flooding and drying of coastal landscapes triggering chemical reactions conducive to the development of life;[301] favour the evolution of biological rhythms such as the day-night cycle that otherwise would not develop in a synchronously rotating planet;[302] mix oceans, thus supplying and redistributing nutrients;[303] and stimulate periodic expansions of marine organisms similar to red tides on Earth.[304]
- TRAPPIST-1 may not produce sufficient quantities of radiation for photosynthesis to support an Earth-like biosphere.[305][306][307] Mullan and Bais (2018) speculated that radiation from flares may increase the photosynthetic potential of TRAPPIST-1,[308] but according to Lingam and Loeb (2019), the potential would still be small.[309]
- Due to the proximity of the TRAPPIST-1 planets, it is possible rock-encased microorganisms ripped[bf] from one planet may arrive at another planet while still viable inside the rock, allowing life to spread between the planets if it originates on one.[310]
- Too much UV radiation from a star can sterilise the surface of a planet[112][157] but too little may not allow the formation of chemical compounds that give rise to life.[14][311] Inadequate production of hydroxyl radicals by low stellar-UV emission may allow gases such as carbon monoxide that are toxic to higher life to accumulate in the planets' atmospheres.[312] The possibilities range from UV fluxes from TRAPPIST-1 being unlikely to be much larger than these of early Earth – even in the event that TRAPPIST-1's emissions of UV radiation are high[313] – to being sufficient to sterilise the planets if they do not have protective atmospheres.[314] As of 2020[update] it is unclear which effect would predominate around TRAPPIST-1,[251] although observations with the Kepler Space Telescope and the Evryscope telescopes indicate the UV flux may be insufficient for the formation of life or its sterilisation.[287]
- The outer planets in the TRAPPIST-1 system could host subsurface oceans similar to those of Enceladus and Europa in the Solar System.[315][115] Chemolithotrophy, the growth of organisms based on non-organic reduced compounds,[316] could sustain life in such oceans.[144] Very deep oceans may be inimical to the development of life.[317]
- Some planets of the TRAPPIST-1 system may have enough water to completely submerge their surfaces.[318] If so, this would have important effects on the possibility of life developing on the planets, and on their climates,[319] as weathering would decrease, starving the oceans of nutrients like phosphorus as well as potentially leading to the accumulation of carbon dioxide in their atmospheres.[320]
In 2017, a search for technosignatures that would indicate the existence of past or present technology in the TRAPPIST-1 system found only signals coming from Earth.[321] In less than two millennia, Earth will be transiting in front of the Sun from the viewpoint of TRAPPIST-1, making the detection of life on Earth from TRAPPIST-1 possible.[322]
Reception and scientific importance
[edit]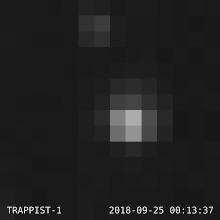
Public reaction and cultural impact
[edit]
The discovery of the TRAPPIST-1 planets drew widespread attention in major world newspapers, social media, streaming television and websites.[323][324] As of 2017[update], the discovery of TRAPPIST-1 led to the largest single-day web traffic to the NASA website.[325] NASA started a public campaign on Twitter to find names for the planets, which drew responses of varying seriousness, although the names of the planets will be decided by the International Astronomical Union.[326] The dynamics of the TRAPPIST-1 planetary system have been represented as music, such as Tim Pyle's Trappist Transits,[327] in Isolation's single Trappist-1 (A Space Anthem)[328] and Leah Asher's piano work TRAPPIST-1.[329] The alleged discovery of an SOS signal from TRAPPIST-1 was an April Fools prank by researchers at the High Energy Stereoscopic System in Namibia.[330] In 2018, Aldo Spadon created a giclée (digital artwork) named "TRAPPIST-1 Planetary System as seen from Space".[331] A website was dedicated to the TRAPPIST-1 system.[332]
Exoplanets are often featured in science-fiction works; books, comics and video games have featured the TRAPPIST-1 system, the earliest being The Terminator, a short story by Swiss author Laurence Suhner published in the academic journal that announced the system's discovery.[333] At least one conference was organised to recognise works of fiction featuring TRAPPIST-1.[334] The planets have been used as the basis of science education competitions[335] and school projects.[336][337] Websites offering TRAPPIST-1-like planets as settings of virtual reality simulations exist,[338] such as the "Exoplanet Travel Bureau"[339] and the "Exoplanets Excursion" – both by NASA.[340] Scientific accuracy has been a point of discussion for such cultural depictions of TRAPPIST-1 planets.[341]
Scientific importance
[edit]TRAPPIST-1 has drawn intense scientific interest.[342] Its planets are the most easily studied exoplanets within their star's habitable zone owing to their relative closeness, the small size of their host star, and because from Earth's perspective they frequently pass in front of their host star.[33] Future observations with space-based observatories and ground-based facilities may allow further insights into their properties such as density, atmospheres, and biosignatures.[bg] TRAPPIST-1 planets[344][345] are considered an important observation target for the James Webb Space Telescope[bh][342] and other telescopes under construction;[163] JWST began investigating the TRAPPIST-1 planets in 2023.[245] Together with the discovery of Proxima Centauri b, the discovery of the TRAPPIST-1 planets and the fact that three of the planets are within the habitable zone has led to an increase in studies on planetary habitability.[348] The planets are considered prototypical for the research on habitability of M dwarfs.[349] The star has been the subject of detailed studies[105] of its various aspects[350] including the possible effects of vegetation on its planets; the possibility of detecting oceans on its planets using starlight reflected off their surfaces;[351] possible efforts to terraform its planets;[352] and difficulties any inhabitants of the planets would encounter with discovering the law of gravitation[353] and with interstellar travel.[354]
The role EU funding played in the discovery of TRAPPIST-1 has been cited as an example of the importance of EU projects,[35] and the involvement of a Moroccan observatory as an indication of the Arab world's role in science. The original discoverers were affiliated with universities spanning Africa, Europe, and North America,[355] and the discovery of TRAPPIST-1 is considered to be an example of the importance of co-operation between observatories.[356] It is also one of the major astronomical discoveries from Chilean observatories.[357]
Exploration
[edit]TRAPPIST-1 is too distant from Earth to be reached by humans with current or expected technology.[358] Spacecraft mission designs using present-day rockets and gravity assists would need hundreds of millennia to reach TRAPPIST-1; even a theoretical interstellar probe travelling at the speed of light would need decades to reach the star. The speculative Breakthrough Starshot proposal for sending small, laser-accelerated, uncrewed probes would require around two centuries to reach TRAPPIST-1.[359]
See also
[edit]- HD 10180, a star with at least six known planets, and three more exoplanet candidates
- HD 110067, star with six known planets all orbiting in a rhythmic resonance
- LHS 1140, another star with a planetary system suitable for atmospheric studies
- List of potentially habitable exoplanets
- LP 890-9, another cool star with a planetary system
- Tabby's Star, another star with notable transit data
Notes
[edit]- ^ A log(g) of 2.992 for the Earth indicates that TRAPPIST-1 has a surface gravity approximately 177 times stronger than Earth's.
- ^ An internal name of the star used by the SPECULOOS project, as this planetary system was its first discovery.
- ^ A red dwarf is a very small and cold star. They are the most common type of star in the Milky Way.[15]
- ^ TRAPPIST is a 60-centimetre (24 in) telescope[11] intended to be a prototype for the "Search for habitable Planets EClipsing ULtra-cOOl Stars" project (SPECULOOS), which aims to identify planets around close, cold stars.[20][21] TRAPPIST is used to find exoplanets, and is preferentially employed on stars colder than 3,000 K (2,730 °C; 4,940 °F).[22]
- ^ When a planet moves in front of its star, it absorbs part of the star's radiation, which may be observed via telescopes.[29]
- ^ The celestial equator is the equator's projection into the sky.[36]
- ^ Based on parallax measurements;[1] the parallax is the position of a celestial object with respect to other celestial objects for a given position of Earth. It can be used to infer the distance of the object from Earth.[39]
- ^ The movement of the star in the sky, relative to background stars.[40]
- ^ Red dwarfs include the spectral type M and K.[42] Spectral types are used to categorise stars by their temperature.[43]
- ^ The effective temperature is the temperature a black body that emits the same amount of radiation would have.[49]
- ^ The photosphere is a thin layer at the surface of a star, where most of its light is produced.[51]
- ^ The solar cycle is the Sun's 11-year long period, during which solar output varies by about 0.1%.[53]
- ^ Including Lyman-alpha radiation[59]
- ^ The main sequence is the longest stage of a star's lifespan, when it is fusing hydrogen.[67]
- ^ Faculae are bright spots on the photosphere.[69]
- ^ Flares are presumably magnetic phenomena lasting for minutes or hours during which parts of the star emit more radiation than usual.[69] In the case of TRAPPIST-1, flares reach temperatures of no more than 9,000 K (8,730 °C; 15,740 °F).[73]
- ^ For comparison, a strong fridge magnet has a strength of about 100 gauss and Earth's magnetic field about 0.5 gauss.[76]
- ^ The chromosphere is an outer layer of a star.[69]
- ^ A coronal mass ejection is an eruption of coronal material to the outside of a star.[69][78]
- ^ Exoplanets are named in order of discovery as "b", "c" and so on; if multiple planets are discovered at once they are named in order of increasing orbital period.[83] The term "TRAPPIST-1a" is used to refer to the star itself.[84]
- ^ One astronomical unit (AU) is the mean distance between the Earth and the Sun.[88]
- ^ For comparison, Earth's orbit around the Sun is inclined by about 1.578 degrees.[95]
- ^ The inner two planets' orbits may be circular; the others could have a small eccentricity.[98]
- ^ A volatile is an element or compound with a low boiling point, such as ammonia, carbon dioxide, methane, nitrogen, sulfur dioxide or water.[106]
- ^ The composition of the mantle of rocky planets is typically approximated as a magnesium silicate.[110]
- ^ A Laplace resonance is an orbital resonance that consists of three bodies, similar to the Galilean moons Europa, Ganymede and Io around Jupiter.[121]
- ^ Where a planet, rather than being a symmetric sphere, has a different radius for each of the three main axes.[127]
- ^ This causes one half of the planet to perpetually face the star in a permanent day and the other half perpetually face away from the star in a permanent night.[131]
- ^ Degassing is the release of gases, which can end up forming an atmosphere, from the mantle or from magma.[135]
- ^ Cryovolcanism occurs when steam or liquid water, or aqueous fluids, erupt to a planet surface ordinarily too cold to host liquid water.[141]
- ^ Hydrothermal vents are hot springs that occur underwater, and are hypothesised to be places where life could originate.[143]
- ^ Not accounting for gravitational compression.[150]
- ^ That is, the inner planets could never cover the entire disk of TRAPPIST-1 from the vantage point of these planets.[85]
- ^ The habitable zone is the region around a star where temperatures are neither too hot nor too cold for the existence of liquid water; it is also called the "Goldilocks zone".[29][75]
- ^ The Roche limit is the distance at which a body is ripped apart by tides.[175]
- ^ The Hill radius is the maximum distance at which a planet's gravity can hold a moon without the star's gravity ripping the moon off.[176]
- ^ A streaming instability is a process where interactions between gas and solid particles cause the latter to clump together in filaments. These filaments can give rise to the precursor bodies of planets.[190]
- ^ According to the International Astronomical Union criteria, a body has to clear its neighbourhood to qualify as a planet in the Solar System.[204]
- ^ On the basis of the Lyman-alpha radiation emissions, TRAPPIST-1b may be losing hydrogen at a rate of 4.6×107 g/s.[215]
- ^ Clouds on the day side reflecting starlight could cool TRAPPIST-1d down to temperatures that allow the presence of liquid water.[226]
- ^ The exoplanet Proxima Centauri b resides in the habitable zone of the nearest star to the Solar System.[232]
- ^ Ocean bodies can still be referred to as such when they are covered by ice.[238]
- ^ Approximate orbital resonance with TRAPPIST-1b
- ^ Approximate orbital resonance with inward planet
- ^ Measured surface temperature of 503 K (230 °C; 446 °F).[244]
- ^ Bourrier et al. (2017) interpreted UV absorption data from the Hubble Space Telescope as implying the outer TRAPPIST-1 planets still have an atmosphere.[14]
- ^ Computer modelling indicates that the non-existence of an atmosphere around TRAPPIST-1 b and c does not imply the lack of same around the other planets.[246]
- ^ Impact events can also remove atmospheres, but a high rate of such "impact erosion" implies a mass of meteorites that is not compatible with the properties of the TRAPPIST-1 system.[253]
- ^ A protoplanetary disk is a disk of matter surrounding a star. Planets are thought to form in such disks.[254]
- ^ A clathrate is a chemical compound where one compound (or chemical element) e.g. carbon dioxide (or xenon), is trapped within a cage-like assembly of molecules from another compound.[262]
- ^ The exosphere is the region of an atmosphere where density is so low that atoms or molecules no longer collide. It is formed by atmospheric escape and the presence of a hydrogen-rich exosphere implies the presence of water.[267]
- ^ Different sources estimate that TRAPPIST-1 emits as much as the Sun at solar minimum,[14] the same amount[251] or more than the Sun.[283]
- ^ Stellar activity is the occurrence of luminosity changes, mostly in the X-ray bands, caused by a star's magnetic field.[285]
- ^ Flares with an energy of over 1×1034 ergs (1.0×1027 J).[286]
- ^ Ohmic heating takes place when electrical currents excited by the stellar wind flow through parts of the atmosphere, heating it.[293]
- ^ In a runaway greenhouse, all water on a planet is in the form of vapour.[296]
- ^ Non-ocean bearing planets can also be subject to tidal heating (or flexing), resulting in structural deformation.[300]
- ^ For example, meteorite impacts could break off rocks from planets at a sufficient speed that they escape its gravity.[310]
- ^ Biosignatures are properties of a planet that can be detected from far away and which suggest the existence of life, such as atmospheric gases that are produced by biological processes.[343]
- ^ As of 2017[update] they were among the smallest planets known where JWST would be able to detect atmospheres.[346] It is possible the JWST may not have time to reliably detect certain biosignatures such as methane and ozone.[347]
References
[edit]- ^ a b c d e f g h Gaia EDR3 2021.
- ^ a b c Costa et al. 2006, p. 1240.
- ^ Costa et al. 2006, p. 1234.
- ^ a b c Cutri et al. 2003, p. II/246.
- ^ a b c d e Agol et al. 2021, p. 1.
- ^ a b c Delrez et al. 2018, pp. 3577–3597.
- ^ Vida et al. 2017, p. 7.
- ^ Barnes et al. 2014, pp. 3094–3113.
- ^ Burgasser & Mamajek 2017, p. 7.
- ^ Martínez-Rodríguez et al. 2019, p. 3.
- ^ a b c d Turbet et al. 2020, p. 2.
- ^ Meadows & Schmidt 2020, p. 727.
- ^ Delrez et al. 2022, p. 2.
- ^ a b c d e Harbach et al. 2021, p. 3.
- ^ Gargaud et al. 2011, Red Dwarf.
- ^ a b Gizis et al. 2000, p. 1088.
- ^ a b Gillon et al. 2016, p. 225.
- ^ Gizis et al. 2000, p. 1085.
- ^ Gizis et al. 2000, p. 1086.
- ^ Barstow & Irwin 2016, p. 95.
- ^ Gillon et al. 2013, p. 1.
- ^ Shields, Ballard & Johnson 2016, p. 7.
- ^ Goldsmith 2018, p. 118.
- ^ Rinaldi & Núñez Ferrer 2017, p. 1.
- ^ a b Angosto, Zaragoza & Melón 2017, p. 85.
- ^ a b c Angosto, Zaragoza & Melón 2017, p. 86.
- ^ a b c Marov & Shevchenko 2020, p. 865.
- ^ Linsky 2019, p. 105.
- ^ a b Cisewski 2017, p. 23.
- ^ Gillon et al. 2017, p. 461.
- ^ Ducrot 2021, p. 4.
- ^ a b c d Gillon et al. 2016, p. 221.
- ^ a b Turbet et al. 2020, p. 3.
- ^ a b Agol et al. 2021, p. 2.
- ^ a b Rinaldi & Núñez Ferrer 2017, pp. 1–2.
- ^ Gargaud et al. 2011, Celestial Equator.
- ^ Barstow & Irwin 2016, p. 93.
- ^ a b Howell et al. 2016, p. 1.
- ^ Gargaud et al. 2011, Parallax.
- ^ Gargaud et al. 2011, Proper Motion.
- ^ Howell et al. 2016, pp. 1, 4.
- ^ The SAO Encyclopedia of Astronomy 2022, Red Dwarf.
- ^ Gargaud et al. 2011, Spectral Type.
- ^ Cloutier & Triaud 2016, p. 4019.
- ^ a b c Lienhard et al. 2020, pp. 3790–3808.
- ^ Goldsmith 2018, p. 82.
- ^ Fischer & Saur 2019, p. 2.
- ^ Gillon et al. 2020, p. 10.
- ^ Gargaud et al. 2011, Effective Temperature.
- ^ Delrez et al. 2022, p. 21.
- ^ Gargaud et al. 2011, Photosphere.
- ^ Miles-Páez et al. 2019, p. 38.
- ^ Gargaud et al. 2011, Variability (Stellar).
- ^ Glazier et al. 2020, p. 2.
- ^ a b Fabbian et al. 2017, p. 770.
- ^ Wilson et al. 2021, p. 10.
- ^ a b c Wilson et al. 2021, p. 1.
- ^ a b Wilson et al. 2021, p. 2.
- ^ Pineda & Hallinan 2018, p. 2.
- ^ Pineda & Hallinan 2018, p. 7.
- ^ Roettenbacher & Kane 2017, p. 2.
- ^ Günther et al. 2022, p. 13.
- ^ Burgasser & Mamajek 2017, p. 1.
- ^ Acton et al. 2017, p. 32.
- ^ a b Snellen 2017, p. 423.
- ^ Acton et al. 2017, p. 34.
- ^ Gargaud et al. 2011, Main Sequence.
- ^ a b Morris et al. 2018, p. 1.
- ^ a b c d e Gargaud et al. 2011, Sun (and Young Sun).
- ^ Morris et al. 2018, p. 5.
- ^ Linsky 2019, p. 250.
- ^ Morris et al. 2018, p. 6.
- ^ Howard et al. 2023, p. 17.
- ^ Gillon et al. 2020, p. 5.
- ^ a b c d e f g h i j k l Airapetian et al. 2020, p. 159.
- ^ MagLab 2022.
- ^ Kochukhov 2021, p. 28.
- ^ a b Mullan & Paudel 2019, p. 2.
- ^ a b Sakaue & Shibata 2021, p. 1.
- ^ Linsky 2019, pp. 147–150.
- ^ Fischer & Saur 2019, p. 6.
- ^ Gonzales et al. 2019, p. 2.
- ^ Schneider et al. 2011, p. 8.
- ^ Harbach et al. 2021, p. 2.
- ^ a b c Veras & Breedt 2017, p. 2677.
- ^ a b c d Agol et al. 2021, Tables.
- ^ a b c d Grimm et al. 2018.
- ^ Fraire et al. 2019, p. 1657.
- ^ Goldsmith 2018, p. 120.
- ^ a b c d e f g h Turbet et al. 2020, p. 8.
- ^ Kral et al. 2018, p. 2650.
- ^ Childs, Martin & Livio 2022, p. 4.
- ^ Martin & Livio 2022, p. 6.
- ^ Marino et al. 2020, p. 6071.
- ^ Handbook of Scientific Tables 2022, p. 2.
- ^ Agol et al. 2021, p. 14.
- ^ Heising et al. 2021, p. 1.
- ^ Brasser et al. 2022, p. 2373.
- ^ Demory et al. 2020, p. 19.
- ^ a b Maltagliati 2017, p. 1.
- ^ Kane et al. 2021, p. 1.
- ^ Srinivas 2017, p. 17.
- ^ Madhusudhan 2020, p. 6-5.
- ^ McDonough & Yoshizaki 2021, p. 9.
- ^ a b Linsky 2019, p. 198.
- ^ Gargaud et al. 2011, Volatile.
- ^ Agol et al. 2021, p. 30.
- ^ a b Gillon et al. 2020, p. 11.
- ^ Schlichting & Young 2022, p. 16.
- ^ Hakim et al. 2018, p. 3.
- ^ Hakim et al. 2018, p. 70.
- ^ a b Barth et al. 2021, p. 1326.
- ^ Grimm et al. 2018, p. 8.
- ^ Lingam & Loeb 2021, p. 594.
- ^ a b c d Quick et al. 2023.
- ^ Van Hoolst, Noack & Rivoldini 2019, p. 598.
- ^ Linsky 2019, p. 253.
- ^ Linsky 2019, p. 254.
- ^ Aschwanden et al. 2018, p. 6.
- ^ Grimm et al. 2018, p. 3.
- ^ Madhusudhan 2020, p. 11-2.
- ^ Grimm et al. 2018, p. 2.
- ^ Ducrot 2021, p. 5.
- ^ Meadows & Schmidt 2020, p. 4.
- ^ Turbet et al. 2020, p. 13.
- ^ Vinson, Tamayo & Hansen 2019, p. 5747.
- ^ Elshaboury et al. 2016, p. 5.
- ^ Zanazzi & Lai 2017, p. 2879.
- ^ a b Turbet et al. 2020, pp. 12–13.
- ^ Lingam & Loeb 2021, p. 144.
- ^ Goldsmith 2018, p. 123.
- ^ Wolf 2017, p. 1.
- ^ Turbet et al. 2018, p. 7.
- ^ Barr, Dobos & Kiss 2018, pp. 1–2.
- ^ Gargaud et al. 2011, Degassing.
- ^ Kislyakova et al. 2017, p. 880.
- ^ Luger et al. 2017, p. 2.
- ^ a b Quick et al. 2020, p. 19.
- ^ Quick et al. 2023, p. 13.
- ^ Turbet et al. 2018, p. 8.
- ^ Quick et al. 2023, p. 2.
- ^ Quick et al. 2023, p. 14.
- ^ Gargaud et al. 2011, Hot Vent Microbiology.
- ^ a b Kendall & Byrne 2020, p. 1.
- ^ a b Kislyakova et al. 2017, p. 878.
- ^ Barr, Dobos & Kiss 2018, p. 12.
- ^ a b Turbet et al. 2020, p. 14.
- ^ Zanazzi & Triaud 2019, p. 61.
- ^ JPL 2021.
- ^ Srinivas 2017, p. 16.
- ^ Radnóti 2021, p. 4.
- ^ a b O'Malley-James & Kaltenegger 2017, p. 27.
- ^ a b Bourrier et al. 2017, p. 7.
- ^ Shields & Carns 2018, p. 1.
- ^ Eager et al. 2020, p. 10.
- ^ a b c O'Malley-James & Kaltenegger 2017, p. 26.
- ^ Awiphan 2018, p. 13.
- ^ Gargaud et al. 2011, Albedo.
- ^ a b Alberti et al. 2017, p. 6.
- ^ Barstow & Irwin 2016, p. 92.
- ^ Checlair, Menou & Abbot 2017, p. 9.
- ^ a b Kral et al. 2018, p. 2649.
- ^ Rushby et al. 2020, p. 13.
- ^ Carone et al. 2018, p. 4677.
- ^ Yang & Ji 2018, p. 1.
- ^ O'Malley-James & Kaltenegger 2019, p. 4542.
- ^ Quick et al. 2023, p. 9.
- ^ Quick et al. 2023, p. 1.
- ^ Bourrier et al. 2017, p. 2.
- ^ Bolmont et al. 2017, p. 3729.
- ^ Bolmont et al. 2017, p. 3739.
- ^ Bolmont et al. 2017, p. 3740.
- ^ Kane 2017, p. 4.
- ^ Gargaud et al. 2011, Roche Limit.
- ^ Gargaud et al. 2011, Hill Radius/Sphere.
- ^ Kane 2017, p. 3.
- ^ Martínez-Rodríguez et al. 2019, p. 8.
- ^ Martínez-Rodríguez et al. 2019, p. 6.
- ^ Allen, Becker & Fuse 2018, p. 1.
- ^ Farrish et al. 2019, p. 7.
- ^ Farrish et al. 2019, p. 6.
- ^ Airapetian et al. 2020, p. 164.
- ^ Fraschetti et al. 2019, p. 11.
- ^ Grayver et al. 2022, p. 9.
- ^ Chao et al. 2021, p. 5.
- ^ a b c d Turbet et al. 2020, p. 36.
- ^ a b Turbet et al. 2020, p. 9.
- ^ Childs et al. 2023, p. 3750.
- ^ Ormel, Liu & Schoonenberg 2017, p. 3.
- ^ a b Liu & Ji 2020, p. 24.
- ^ Ogihara et al. 2022, p. 6.
- ^ Brasser et al. 2022, p. 2374.
- ^ Bean, Raymond & Owen 2021, p. 9.
- ^ Grimm et al. 2018, p. 13.
- ^ Marino et al. 2020, p. 6067.
- ^ Turbet et al. 2020, pp. 9–10.
- ^ Flock et al. 2019, p. 10.
- ^ Heising et al. 2021, p. 5.
- ^ a b Gressier et al. 2022, p. 2.
- ^ Raymond et al. 2021, p. 1.
- ^ Raymond et al. 2021, p. 2.
- ^ Raymond et al. 2021, p. 3.
- ^ a b Raymond et al. 2021, p. 4.
- ^ Gabriel & Horn 2021, p. 6.
- ^ Childs et al. 2023, p. 3762.
- ^ Krissansen-Totton & Fortney 2022, p. 8.
- ^ a b c d e f Grimm et al. 2018, p. 6.
- ^ a b c d Gillon et al. 2016, p. 222.
- ^ Lim et al. 2023, p. 2.
- ^ a b c d Turbet et al. 2020, p. 24.
- ^ Ih et al. 2023, p. 5.
- ^ a b c d e f g h i Linsky 2019, pp. 198–199.
- ^ a b c Turbet et al. 2020, p. 28.
- ^ a b c Grenfell et al. 2020, p. 11.
- ^ a b c d e Turbet et al. 2020, p. 29.
- ^ Grenfell et al. 2020, p. 18.
- ^ Lim et al. 2023, p. 7.
- ^ a b Lim et al. 2023, p. 9.
- ^ Lincowski et al. 2023, p. 8.
- ^ Teixeira et al. 2023, p. 12.
- ^ Agol et al. 2021, p. 21.
- ^ Stevenson 2019, p. 329.
- ^ Pierrehumbert & Hammond 2019, p. 285.
- ^ Carone et al. 2018, p. 4683.
- ^ Turbet et al. 2018, p. 17.
- ^ Turbet et al. 2018, p. 1.
- ^ Turbet et al. 2020, pp. 5–6.
- ^ a b c d Agol et al. 2021, p. 10.
- ^ a b Stevenson 2019, p. 327.
- ^ Turbet et al. 2020, pp. 29–30.
- ^ Meadows et al. 2018, p. 133.
- ^ Janjic 2017, p. 61.
- ^ Meadows et al. 2018, p. 141.
- ^ a b c Quick et al. 2023, p. 4.
- ^ Quick et al. 2023, p. 11.
- ^ a b Kane et al. 2021, p. 16.
- ^ Kane et al. 2021, p. 14.
- ^ a b Kane et al. 2021, p. 17.
- ^ a b Airapetian et al. 2020, p. 171.
- ^ a b Turbet et al. 2018, p. 2.
- ^ a b Turbet et al. 2020, p. 30.
- ^ Agol et al. 2021.
- ^ Greene et al. 2023.
- ^ a b Ih et al. 2023, p. 1.
- ^ Teixeira et al. 2023, pp. 8, 9.
- ^ a b Fortney 2018, p. 17.
- ^ Wunderlich et al. 2020, pp. 26–27.
- ^ Zhang et al. 2018, p. 1.
- ^ Turbet et al. 2020, p. 33.
- ^ a b c d Ducrot et al. 2020, p. 2.
- ^ Howard et al. 2023, p. 2.
- ^ Teixeira et al. 2023, p. 10.
- ^ Gargaud et al. 2011, Protoplanetary Disk.
- ^ Kral, Davoult & Charnay 2020, p. 770.
- ^ Hori & Ogihara 2020, p. 1.
- ^ Turbet et al. 2020, p. 10.
- ^ Kral et al. 2018, p. 2670.
- ^ Lingam & Loeb 2019a, p. 8.
- ^ Turbet et al. 2018, p. 9.
- ^ Turbet et al. 2018, p. 10.
- ^ Turbet et al. 2018, p. 14.
- ^ Turbet et al. 2018, pp. 14–15.
- ^ Turbet et al. 2020, p. 23.
- ^ a b Gillon et al. 2020, p. 14.
- ^ Gressier et al. 2022, p. 6.
- ^ dos Santos et al. 2019, p. 1.
- ^ dos Santos et al. 2019, p. 11.
- ^ Gillon et al. 2020, p. 15.
- ^ Edwards et al. 2020, p. 11.
- ^ Turbet et al. 2020, pp. 24–26.
- ^ Teixeira et al. 2023, p. 2.
- ^ Turbet et al. 2020, pp. 26–27.
- ^ Ducrot et al. 2020, p. 19.
- ^ Turbet et al. 2020, pp. 27–28.
- ^ Turbet et al. 2020, p. 37.
- ^ Wunderlich et al. 2020, p. 2.
- ^ Krissansen-Totton & Fortney 2022, p. 14.
- ^ Stevenson 2019, pp. 330–332.
- ^ Zhang 2020, p. 57.
- ^ a b c d e f Turbet et al. 2020, p. 6.
- ^ Wheatley et al. 2017, p. 74.
- ^ Turbet et al. 2020, pp. 7–8.
- ^ Acton et al. 2017, p. 33.
- ^ Gargaud et al. 2011, Activity (Magnetic).
- ^ Glazier et al. 2020, p. 1.
- ^ a b Glazier et al. 2020, p. 9.
- ^ Samara, Patsourakos & Georgoulis 2021, p. 1.
- ^ Linsky 2019, p. 191.
- ^ Dong et al. 2018, p. 262.
- ^ Teixeira et al. 2023, p. 5.
- ^ Dong et al. 2018, p. 264.
- ^ Cohen et al. 2018, p. 1.
- ^ Linsky 2019, p. 189.
- ^ Turbet et al. 2020, pp. 3, 5.
- ^ a b Turbet et al. 2020, p. 5.
- ^ Lingam & Loeb 2018a, p. 122.
- ^ Pidhorodetska et al. 2020, p. 2.
- ^ Lingam & Loeb 2018b, p. 973.
- ^ Barr, Dobos & Kiss 2018, p. 6.
- ^ Lingam & Loeb 2018b, pp. 969–970.
- ^ Lingam & Loeb 2018b, p. 971.
- ^ Lingam & Loeb 2018b, p. 972.
- ^ Lingam & Loeb 2018b, p. 975.
- ^ Lingam & Loeb 2019a, p. 11.
- ^ Covone et al. 2021, p. 3332.
- ^ Lingam & Loeb 2021, p. 347.
- ^ Mullan & Bais 2018, p. 11.
- ^ Lingam & Loeb 2019b, p. 5926.
- ^ a b Goldsmith 2018, p. 124.
- ^ Ranjan, Wordsworth & Sasselov 2017, pp. 2, 9.
- ^ Schwieterman et al. 2019, p. 5.
- ^ O'Malley-James & Kaltenegger 2017, p. 30.
- ^ Valio et al. 2018, p. 179.
- ^ Lingam & Loeb 2019c, p. 112.
- ^ Gargaud et al. 2011, Chemolithotroph.
- ^ Barth et al. 2021, p. 1344.
- ^ Guimond, Rudge & Shorttle 2022, pp. 16–17.
- ^ Guimond, Rudge & Shorttle 2022, p. 1.
- ^ Glaser et al. 2020, p. 7.
- ^ Pinchuk et al. 2019, p. 1.
- ^ Kaltenegger & Faherty 2021, p. 505.
- ^ Short & Stapelfeldt 2017, pp. 1, 28.
- ^ Díaz 2017, pp. 185–186.
- ^ Short & Stapelfeldt 2017, p. 28.
- ^ Physics World 2017, p. 1.
- ^ Riber 2018, p. 1.
- ^ Howell 2020, p. 3–34.
- ^ McKay 2021, p. 14.
- ^ Janjic 2017, p. 57.
- ^ Kanas 2019, p. 488.
- ^ Gibb 2022, p. 2.
- ^ Gillon 2020a, p. 35.
- ^ Gillon 2020b, p. 50.
- ^ Sein et al. 2021, p. 3.
- ^ Hughes 2022, p. 148.
- ^ Lane et al. 2022, p. 5.
- ^ Paladini 2019, pp. 239, 254.
- ^ Exoplanet Travel Bureau 2021.
- ^ AAS 2020, p. 309.
- ^ Fidrick et al. 2020, pp. 1–2.
- ^ a b Deming & Knutson 2020, p. 459.
- ^ Grenfell 2017, p. 2.
- ^ Madhusudhan 2019, p. 652.
- ^ Turbet et al. 2020, p. 31.
- ^ Morley et al. 2017, p. 1.
- ^ Chiao 2019, p. 880.
- ^ Lingam & Loeb 2018a, p. 116.
- ^ Madhusudhan 2020, p. I-7.
- ^ Delrez et al. 2022, p. 32.
- ^ Kopparla et al. 2018, p. 1.
- ^ Sleator & Smith 2017, pp. 1–2.
- ^ Wang 2022, p. 10.
- ^ Lingam & Loeb 2018c.
- ^ Determann 2019, pp. 168–169.
- ^ Gutiérrez et al. 2019, p. 41.
- ^ Guridi, Pertuze & Pfotenhauer 2020, p. 5.
- ^ Euroschool 2018, p. 10.
- ^ Srinivas 2017, p. 19.
Sources
[edit]- "Special Session 001" (PDF). Abstracts of the 235th AAS Meeting. Honolulu, Hawaii: American Astronomical Society. January 2020.
- Acton, C.; Slavney, S.; Arvidson, R. E.; Gaddis, L. R.; Gordon, M.; Lavoie, S. (2017). "The planetary data system" (PDF). Lunar Planet. Inf. Bull. 150: 2–11.
- Agol, Eric; Dorn, Caroline; Grimm, Simon L.; Turbet, Martin; Ducrot, Elsa; Delrez, Laetitia; Gillon, Michaël; Demory, Brice-Olivier; Burdanov, Artem; Barkaoui, Khalid; Benkhaldoun, Zouhair; Bolmont, Emeline; Burgasser, Adam; Carey, Sean; de Wit, Julien; Fabrycky, Daniel; Foreman-Mackey, Daniel; Haldemann, Jonas; Hernandez, David M.; Ingalls, James; Jehin, Emmanuel; Langford, Zachary; Leconte, Jérémy; Lederer, Susan M.; Luger, Rodrigo; Malhotra, Renu; Meadows, Victoria S.; Morris, Brett M.; Pozuelos, Francisco J.; Queloz, Didier; Raymond, Sean N.; Selsis, Franck; Sestovic, Marko; Triaud, Amaury H. M. J.; Van Grootel, Valérie (1 February 2021). "Refining the Transit-timing and Photometric Analysis of TRAPPIST-1: Masses, Radii, Densities, Dynamics, and Ephemerides". The Planetary Science Journal. 2 (1): 1. arXiv:2010.01074. Bibcode:2021PSJ.....2....1A. doi:10.3847/psj/abd022. ISSN 2632-3338. S2CID 222125312.
- Airapetian, V. S.; Barnes, R.; Cohen, O.; Collinson, G. A.; Danchi, W. C.; Dong, C. F.; Del Genio, A. D.; France, K.; Garcia-Sage, K.; Glocer, A.; Gopalswamy, N.; Grenfell, J. L.; Gronoff, G.; Güdel, M.; Herbst, K.; Henning, W. G.; Jackman, C. H.; Jin, M.; Johnstone, C. P.; Kaltenegger, L.; Kay, C. D.; Kobayashi, K.; Kuang, W.; Li, G.; Lynch, B. J.; Lüftinger, T.; Luhmann, J. G.; Maehara, H.; Mlynczak, M. G.; Notsu, Y.; Osten, R. A.; Ramirez, R. M.; Rugheimer, S.; Scheucher, M.; Schlieder, J. E.; Shibata, K.; Sousa-Silva, C.; Stamenković, V.; Strangeway, R. J.; Usmanov, A. V.; Vergados, P.; Verkhoglyadova, O. P.; Vidotto, A. A.; Voytek, M.; Way, M. J.; Zank, G. P.; Yamashiki, Y. (April 2020). "Impact of space weather on climate and habitability of terrestrial-type exoplanets". International Journal of Astrobiology. 19 (2): 136–194. arXiv:1905.05093. Bibcode:2020IJAsB..19..136A. doi:10.1017/S1473550419000132. ISSN 1475-3006. S2CID 152282234.
- Alberti, Tommaso; Carbone, Vincenzo; Lepreti, Fabio; Vecchio, Antonio (18 July 2017). "Comparative Climates of the Trappist-1 Planetary System: Results from a Simple Climate-vegetation Model". The Astrophysical Journal. 844 (1): 19. arXiv:1706.06005. Bibcode:2017ApJ...844...19A. doi:10.3847/1538-4357/aa78a2. S2CID 118972556.
- Allen, John; Becker, Christopher; Fuse, Christopher (1 January 2018). Stability of Moons in the Trappist-1 System. American Astronomical Society, AAS Meeting #231. American Astronomical Society Meeting Abstracts #231. Vol. 231. National Harbor, Maryland. p. 148.21. Bibcode:2018AAS...23114821A.
- Angosto, María Cascales; Zaragoza, Federico Mayor; Melón, José Miguel Ortiz (2017). Premios Nobel 2016 [Nobel Prizes 2016] (PDF) (Report) (in Spanish). Centro de Estudios Ramón Areces.
- Aschwanden, Markus J.; Scholkmann, Felix; Béthune, William; Schmutz, Werner; Abramenko, Valentina; Cheung, Mark C. M.; Müller, Daniel; Benz, Arnold; Chernov, Guennadi; Kritsuk, Alexei G.; Scargle, Jeffrey D.; Melatos, Andrew; Wagoner, Robert V.; Trimble, Virginia; Green, William H. (March 2018). "Order out of Randomness: Self-Organization Processes in Astrophysics". Space Science Reviews. 214 (2): 55. arXiv:1708.03394. Bibcode:2018SSRv..214...55A. doi:10.1007/s11214-018-0489-2. ISSN 1572-9672. S2CID 119064521.
- Awiphan, Supachai (2018). Exomoons to Galactic Structure. Springer Theses. doi:10.1007/978-3-319-90957-8. ISBN 978-3-319-90956-1.
- Barnes, J. R.; Jenkins, J. S.; Jones, H. R. A.; Jeffers, S. V.; Rojo, P.; Arriagada, P.; Jordán, A.; Minniti, D.; Tuomi, M.; Pinfield, D.; Anglada-Escudé, G. (11 April 2014). "Precision radial velocities of 15 M5–M9 dwarfs". Monthly Notices of the Royal Astronomical Society. 439 (3): 3094–3113. arXiv:1401.5350. Bibcode:2014MNRAS.439.3094B. doi:10.1093/mnras/stu172. S2CID 16005221.
- Barr, Amy C.; Dobos, Vera; Kiss, László L. (1 May 2018). "Interior structures and tidal heating in the TRAPPIST-1 planets". Astronomy & Astrophysics. 613: A37. arXiv:1712.05641. Bibcode:2018A&A...613A..37B. doi:10.1051/0004-6361/201731992. ISSN 0004-6361. S2CID 119516532.
- Barstow, J. K.; Irwin, P. G. J. (1 September 2016). "Habitable worlds with JWST: transit spectroscopy of the TRAPPIST-1 system?". Monthly Notices of the Royal Astronomical Society: Letters. 461 (1): L92–L96. arXiv:1605.07352. Bibcode:2016MNRAS.461L..92B. doi:10.1093/mnrasl/slw109. ISSN 1745-3933. S2CID 17058804.
- Barth, Patrick; Carone, Ludmila; Barnes, Rory; Noack, Lena; Mollière, Paul; Henning, Thomas (1 November 2021). "Magma Ocean Evolution of the TRAPPIST-1 Planets". Astrobiology. 21 (11): 1325–1349. arXiv:2008.09599. Bibcode:2021AsBio..21.1325B. doi:10.1089/ast.2020.2277. ISSN 1531-1074. PMID 34314604. S2CID 221246323.
- Bean, Jacob L.; Raymond, Sean N.; Owen, James E. (2021). "The Nature and Origins of Sub-Neptune Size Planets". Journal of Geophysical Research: Planets. 126 (1): e2020JE006639. arXiv:2010.11867. Bibcode:2021JGRE..12606639B. doi:10.1029/2020JE006639. ISSN 2169-9100. PMC 7900964. PMID 33680689.
- Bolmont, E.; Selsis, F.; Owen, J. E.; Ribas, I.; Raymond, S. N.; Leconte, J.; Gillon, M. (21 January 2017). "Water loss from terrestrial planets orbiting ultracool dwarfs: implications for the planets of TRAPPIST-1". Monthly Notices of the Royal Astronomical Society. 464 (3): 3728–3741. arXiv:1605.00616. Bibcode:2017MNRAS.464.3728B. doi:10.1093/mnras/stw2578. ISSN 1365-2966. S2CID 53687987.
- Bourrier, V.; de Wit, J.; Bolmont, E.; Stamenković, V.; Wheatley, P. J.; Burgasser, A. J.; Delrez, L.; Demory, B.-O.; Ehrenreich, D.; Gillon, M.; Jehin, E.; Leconte, J.; Lederer, S. M.; Lewis, N.; Triaud, A. H. M. J.; Van Grootel, V. (31 August 2017). "Temporal Evolution of the High-energy Irradiation and Water Content of TRAPPIST-1 Exoplanets". The Astronomical Journal. 154 (3): 121. arXiv:1708.09484. Bibcode:2017AJ....154..121B. doi:10.3847/1538-3881/aa859c. hdl:1721.1/112267. S2CID 44398519.
- Brasser, R.; Pichierri, G.; Dobos, V.; Barr, A. C. (29 July 2022). "Long-term tidal evolution of the TRAPPIST-1 system". Monthly Notices of the Royal Astronomical Society. 515 (2): 2373–2385. arXiv:2207.05336. doi:10.1093/mnras/stac1907. ISSN 1365-2966.
- Burgasser, Adam J.; Mamajek, Eric E. (17 August 2017). "On the Age of the TRAPPIST-1 System". The Astrophysical Journal. 845 (2): 110. arXiv:1706.02018. Bibcode:2017ApJ...845..110B. doi:10.3847/1538-4357/aa7fea. S2CID 119464994.
- Carone, L.; Keppens, R.; Decin, L.; Henning, Th. (1 February 2018). "Stratosphere circulation on tidally locked ExoEarths". Monthly Notices of the Royal Astronomical Society. 473 (4): 4672–4685. arXiv:1711.11446. Bibcode:2018MNRAS.473.4672C. doi:10.1093/mnras/stx2732. ISSN 1365-2966. S2CID 119049536.
- Chao, Keng-Hsien; deGraffenried, Rebecca; Lach, Mackenzie; Nelson, William; Truax, Kelly; Gaidos, Eric (1 May 2021). "Lava worlds: From early earth to exoplanets". Geochemistry. 81 (2): 125735. arXiv:2012.07337. Bibcode:2021ChEG...81l5735C. doi:10.1016/j.chemer.2020.125735. ISSN 0009-2819. S2CID 229153893.
- Checlair, Jade; Menou, Kristen; Abbot, Dorian S. (18 August 2017). "No Snowball on Habitable Tidally Locked Planets". The Astrophysical Journal. 845 (2): 132. arXiv:1705.08904. Bibcode:2017ApJ...845..132C. doi:10.3847/1538-4357/aa80e1. S2CID 13719958.
- Chiao, May (October 2019). "An infrared selfie". Nature Astronomy. 3 (10): 880. Bibcode:2019NatAs...3..880C. doi:10.1038/s41550-019-0919-4. ISSN 2397-3366. S2CID 204718432.
- Childs, Anna C.; Martin, Rebecca G.; Livio, Mario (1 October 2022). "Life on Exoplanets in the Habitable Zone of M Dwarfs?". The Astrophysical Journal Letters. 937 (2): L41. arXiv:2209.02860. Bibcode:2022ApJ...937L..41C. doi:10.3847/2041-8213/ac9052. S2CID 252110686.
- Childs, Anna C.; Shakespeare, Cody; Rice, David R.; Yang, Chao-Chin; Steffen, Jason H. (24 July 2023). "Composition constraints of the TRAPPIST-1 planets from their formation". Monthly Notices of the Royal Astronomical Society. 524 (3): 3749–3768. arXiv:2307.04989. doi:10.1093/mnras/stad2110.
- Cisewski, Jessi (2017). "In search of Earth analogues". Significance. 14 (2): 22–25. doi:10.1111/j.1740-9713.2017.01017.x. ISSN 1740-9713. S2CID 157345539.
- Cloutier, Ryan; Triaud, Amaury H. M. J. (11 November 2016). "Prospects for detecting the Rossiter–McLaughlin effect of Earth-like planets: the test case of TRAPPIST-1b and c". Monthly Notices of the Royal Astronomical Society. 462 (4): 4018–4027. arXiv:1608.01334. Bibcode:2016MNRAS.462.4018C. doi:10.1093/mnras/stw1953. ISSN 0035-8711. S2CID 16147394.
- Cohen, Ofer; Glocer, Alex; Garraffo, Cecilia; Drake, Jeremy J.; Bell, Jared M. (23 March 2018). "Energy Dissipation in the Upper Atmospheres of TRAPPIST-1 Planets". The Astrophysical Journal. 856 (1): L11. arXiv:1803.05089. Bibcode:2018ApJ...856L..11C. doi:10.3847/2041-8213/aab5b5. PMC 7493050. PMID 32944211.
- Costa, E.; Méndez, R. A.; Jao, W.-C.; Henry, T. J.; Subasavage, J. P.; Ianna, P. A. (4 August 2006). "The Solar Neighborhood. XVI. Parallaxes from CTIOPI: Final Results from the 1.5 m Telescope Program". The Astronomical Journal. 132 (3): 1234. Bibcode:2006AJ....132.1234C. CiteSeerX 10.1.1.622.2310. doi:10.1086/505706. S2CID 18952940.
- Covone, Giovanni; Ienco, Riccardo M.; Cacciapuoti, Luca; Inno, Laura (11 August 2021). "Efficiency of the oxygenic photosynthesis on Earth-like planets in the habitable zone". Monthly Notices of the Royal Astronomical Society. 505 (3): 3329–3335. arXiv:2104.01425. Bibcode:2021MNRAS.505.3329C. doi:10.1093/mnras/stab1357. ISSN 1365-2966. S2CID 233025250.
- Cutri, R. M.; Skrutskie, M. F.; Van Dyk, S.; Beichman, C. A.; Carpenter, J. M.; Chester, T.; Cambresy, L.; Evans, T.; Fowler, J.; Gizis, J.; Howard, E.; Huchra, J.; Jarrett, T.; Kopan, E. L.; Kirkpatrick, J. D.; Light, R. M.; Marsh, K. A.; McCallon, H.; Schneider, S.; Stiening, R.; Sykes, M.; Weinberg, M.; Wheaton, W. A.; Wheelock, S.; Zacarias, N. (June 2003). "VizieR Online Data Catalog: 2MASS All-Sky Catalog of Point Sources (Cutri+ 2003)". CDS/ADC Collection of Electronic Catalogues (2246): II/246. Bibcode:2003yCat.2246....0C.
- Delrez, L.; Gillon, M.; Triaud, A. H. M. J.; Demory, B-O.; de Wit, J.; Ingalls, J. G.; Agol, E.; Bolmont, E.; Burdanov, A.; Burgasser, A. J.; Carey, S. J.; Jehin, E.; Leconte, J.; Lederer, S.; Queloz, D.; Selsis, F.; Van Grootel, V. (11 April 2018). "Early 2017 observations of TRAPPIST-1 with Spitzer". Monthly Notices of the Royal Astronomical Society. 475 (3): 3577–3597. arXiv:1801.02554. Bibcode:2018MNRAS.475.3577D. doi:10.1093/mnras/sty051. ISSN 1365-2966. S2CID 54649681.
- Delrez, L.; Murray, C. A.; Pozuelos, F. J.; Narita, N.; Ducrot, E.; Timmermans, M.; Watanabe, N.; Burgasser, A. J.; Hirano, T.; Rackham, B. V.; Stassun, K. G.; Van Grootel, V.; Aganze, C.; Cointepas, M.; Howell, S.; Kaltenegger, L.; Niraula, P.; Sebastian, D.; Almenara, J. M.; Barkaoui, K.; Baycroft, T. A.; Bonfils, X.; Bouchy, F.; Burdanov, A.; Caldwell, D. A.; Charbonneau, D.; Ciardi, D. R.; Collins, K. A.; Daylan, T.; Demory, B.-O.; de Wit, J.; Dransfield, G.; Fajardo-Acosta, S. B.; Fausnaugh, M.; Fukui, A.; Furlan, E.; Garcia, L. J.; Gnilka, C. L.; Gómez Maqueo Chew, Y.; Gómez-Muñoz, M. A.; Günther, M. N.; Harakawa, H.; Heng, K.; Hooton, M. J.; Hori, Y.; Ikoma, M.; Jehin, E.; Jenkins, J. M.; Kagetani, T.; Kawauchi, K.; Kimura, T.; Kodama, T.; Kotani, T.; Krishnamurthy, V.; Kudo, T.; Kunovac, V.; Kusakabe, N.; Latham, D. W.; Littlefield, C.; McCormac, J.; Melis, C.; Mori, M.; Murgas, F.; Palle, E.; Pedersen, P. P.; Queloz, D.; Ricker, G.; Sabin, L.; Schanche, N.; Schroffenegger, U.; Seager, S.; Shiao, B.; Sohy, S.; Standing, M. R.; Tamura, M.; Theissen, C. A.; Thompson, S. J.; Triaud, A. H. M. J.; Vanderspek, R.; Vievard, S.; Wells, R. D.; Winn, J. N.; Zou, Y.; Zúñiga-Fernández, S.; Gillon, M. (8 September 2022). "Two temperate super-Earths transiting a nearby late-type M dwarf". Astronomy & Astrophysics. 667: A59. arXiv:2209.02831. Bibcode:2022A&A...667A..59D. doi:10.1051/0004-6361/202244041. ISSN 0004-6361. S2CID 252110654 – via arXiv.
- Deming, Drake; Knutson, Heather A. (May 2020). "Highlights of exoplanetary science from Spitzer". Nature Astronomy. 4 (5): 453–466. arXiv:2005.11331. Bibcode:2020NatAs...4..453D. doi:10.1038/s41550-020-1100-9. ISSN 2397-3366. S2CID 218870017.
- Demory, B.-O.; Pozuelos, F. J.; Chew, Y. Gómez Maqueo; Sabin, L.; Petrucci, R.; Schroffenegger, U.; Grimm, S. L.; Sestovic, M.; Gillon, M.; McCormac, J.; Barkaoui, K.; Benz, W.; Bieryla, A.; Bouchy, F.; Burdanov, A.; Collins, K. A.; Wit, J. de; Dressing, C. D.; Garcia, L. J.; Giacalone, S.; Guerra, P.; Haldemann, J.; Heng, K.; Jehin, E.; Jofré, E.; Kane, S. R.; Lillo-Box, J.; Maigné, V.; Mordasini, C.; Morris, B. M.; Niraula, P.; Queloz, D.; Rackham, B. V.; Savel, A. B.; Soubkiou, A.; Srdoc, G.; Stassun, K. G.; Triaud, A. H. M. J.; Zambelli, R.; Ricker, G.; Latham, D. W.; Seager, S.; Winn, J. N.; Jenkins, J. M.; Calvario-Velásquez, T.; Herrera, J. A. Franco; Colorado, E.; Zepeda, E. O. Cadena; Figueroa, L.; Watson, A. M.; Lugo-Ibarra, E. E.; Carigi, L.; Guisa, G.; Herrera, J.; Díaz, G. Sierra; Suárez, J. C.; Barrado, D.; Batalha, N. M.; Benkhaldoun, Z.; Chontos, A.; Dai, F.; Essack, Z.; Ghachoui, M.; Huang, C. X.; Huber, D.; Isaacson, H.; Lissauer, J. J.; Morales-Calderón, M.; Robertson, P.; Roy, A.; Twicken, J. D.; Vanderburg, A.; Weiss, L. M. (1 October 2020). "A super-Earth and a sub-Neptune orbiting the bright, quiet M3 dwarf TOI-1266". Astronomy & Astrophysics. 642: A49. arXiv:2009.04317. Bibcode:2020A&A...642A..49D. doi:10.1051/0004-6361/202038616. ISSN 0004-6361. S2CID 221554586.
- Determann, Jörg Matthias (2019). Space science and the Arab world: astronauts, observatories and nationalism in the Middle East. Bloomsbury Publishing. ISBN 978-1-83860-015-0. OCLC 1122719747.
- Díaz, R. F. (2017). "Exploring new worlds. A review on extrasolar planet observations". Boletin de la Asociacion Argentina de Astronomia la Plata Argentina. 59: 183–189. Bibcode:2017BAAA...59..183D.
- Dong, Chuanfei; Jin, Meng; Lingam, Manasvi; Airapetian, Vladimir S.; Ma, Yingjuan; van der Holst, Bart (9 January 2018). "Atmospheric escape from the TRAPPIST-1 planets and implications for habitability". Proceedings of the National Academy of Sciences. 115 (2): 260–265. arXiv:1705.05535. Bibcode:2018PNAS..115..260D. doi:10.1073/pnas.1708010115. ISSN 0027-8424. PMC 5777028. PMID 29284746. S2CID 31195274.
- dos Santos, Leonardo A.; Bourrier, Vincent; Ehrenreich, David; Kameda, Shingo (1 February 2019). "Observability of hydrogen-rich exospheres in Earth-like exoplanets". Astronomy & Astrophysics. 622: A46. arXiv:1812.02145. Bibcode:2019A&A...622A..46D. doi:10.1051/0004-6361/201833392. ISSN 0004-6361. S2CID 119013251.
- Ducrot, E.; Gillon, M.; Delrez, L.; Agol, E.; Rimmer, P.; Turbet, M.; Günther, M. N.; Demory, B.-O.; Triaud, A. H. M. J.; Bolmont, E.; Burgasser, A.; Carey, S. J.; Ingalls, J. G.; Jehin, E.; Leconte, J.; Lederer, S. M.; Queloz, D.; Raymond, S. N.; Selsis, F.; Van Grootel, V.; Wit, J. de (1 August 2020). "TRAPPIST-1: Global results of the Spitzer Exploration Science Program Red Worlds". Astronomy & Astrophysics. 640: A112. arXiv:2006.13826. Bibcode:2020A&A...640A.112D. doi:10.1051/0004-6361/201937392. ISSN 0004-6361. S2CID 220041987.
- Ducrot, Elsa (2 April 2021). "A brief history of the TRAPPIST-1 system Article sur invitation – Invited paper". Bulletin de la Société Royale des Sciences de Liège. 90. doi:10.25518/0037-9565.10277. ISSN 0037-9565. S2CID 246354436.
- Eager, Jake K.; Reichelt, David J.; Mayne, Nathan J.; Lambert, F. Hugo; Sergeev, Denis E.; Ridgway, Robert J.; Manners, James; Boutle, Ian A.; Lenton, Timothy M.; Kohary, Krisztian (1 July 2020). "Implications of different stellar spectra for the climate of tidally locked Earth-like exoplanets". Astronomy & Astrophysics. 639: A99. arXiv:2005.13002. Bibcode:2020A&A...639A..99E. doi:10.1051/0004-6361/202038089. ISSN 0004-6361. S2CID 218900900.
- Edwards, Billy; Changeat, Quentin; Mori, Mayuko; Anisman, Lara O.; Morvan, Mario; Yip, Kai Hou; Tsiaras, Angelos; Al-Refaie, Ahmed; Waldmann, Ingo; Tinetti, Giovanna (24 December 2020). "Hubble WFC3 Spectroscopy of the Habitable-zone Super-Earth LHS 1140 b". The Astronomical Journal. 161 (1): 44. arXiv:2011.08815. Bibcode:2021AJ....161...44E. doi:10.3847/1538-3881/abc6a5. S2CID 226975730.
- Elshaboury, S. M.; Abouelmagd, Elbaz I.; Kalantonis, V. S.; Perdios, E. A. (25 August 2016). "The planar restricted three-body problem when both primaries are triaxial rigid bodies: Equilibrium points and periodic orbits". Astrophysics and Space Science. 361 (9): 315. Bibcode:2016Ap&SS.361..315E. doi:10.1007/s10509-016-2894-x. ISSN 1572-946X. S2CID 254252200.
- "Explore the Surface – TRAPPIST 1d". Exoplanet Travel Bureau. NASA. Retrieved 16 November 2021.
- Fabbian, D.; Simoniello, R.; Collet, R.; Criscuoli, S.; Korhonen, H.; Krivova, N. A.; Oláh, K.; Jouve, L.; Solanki, S. K.; Alvarado-Gómez, J. D.; Booth, R.; García, R. A.; Lehtinen, J.; See, V. (2017). "The variability of magnetic activity in solar-type stars". Astronomische Nachrichten. 338 (7): 753–772. Bibcode:2017AN....338..753F. doi:10.1002/asna.201713403. ISSN 1521-3994. S2CID 53572712.
- Farrish, Alison O.; Alexander, David; Maruo, Mei; DeRosa, Marc; Toffoletto, Frank; Sciola, Anthony M. (30 October 2019). "Characterizing the Magnetic Environment of Exoplanet Stellar Systems". The Astrophysical Journal. 885 (1): 51. Bibcode:2019ApJ...885...51F. doi:10.3847/1538-4357/ab4652. S2CID 209907654.
- Fidrick, Dawn; Yeung, Gee; Niemack, Bob; Dixon, Don (17 August 2020). The Art and Science of Imaging Worlds: Griffith Observatory's planetarium show Signs of Life. ACM SIGGRAPH 2020 Talks. pp. 1–2. doi:10.1145/3388767.3411060. ISBN 9781450379717. S2CID 221178064.
- Fischer, Christian; Saur, Joachim (14 February 2019). "Time-variable Electromagnetic Star–Planet Interaction: The TRAPPIST-1 System as an Exemplary Case". The Astrophysical Journal. 872 (1): 113. arXiv:1901.02747. Bibcode:2019ApJ...872..113F. doi:10.3847/1538-4357/aafaf2. S2CID 119326120.
- Flock, Mario; Turner, Neal J.; Mulders, Gijs D.; Hasegawa, Yasuhiro; Nelson, Richard P.; Bitsch, Bertram (1 October 2019). "Planet formation and migration near the silicate sublimation front in protoplanetary disks". Astronomy & Astrophysics. 630: A147. arXiv:1910.03901. Bibcode:2019A&A...630A.147F. doi:10.1051/0004-6361/201935806. ISSN 0004-6361. S2CID 203027318.
- Fortney, Jonathan J. (2018). "Modeling Exoplanetary Atmospheres: An Overview". In Bozza, Valerio; Mancini, Luigi; Sozzetti, Alessandro (eds.). Astrophysics of Exoplanetary Atmospheres. Astrophysics and Space Science Library. Vol. 450. Cham: Springer International Publishing. pp. 51–88. arXiv:1804.08149. doi:10.1007/978-3-319-89701-1_2. ISBN 978-3-319-89700-4. S2CID 59406383.
- Fraire, Juan Andres; Feldmann, Marius; Walter, Felix; Fantino, Elena; Burleigh, Scott C. (August 2019). "Networking in Interstellar Dimensions: Communicating With TRAPPIST-1". IEEE Transactions on Aerospace and Electronic Systems. 55 (4): 1656–1665. Bibcode:2019ITAES..55.1656F. doi:10.1109/TAES.2018.2874149. hdl:11336/105240. ISSN 1557-9603. S2CID 117702090.
- Fraschetti, F.; Drake, J. J.; Alvarado-Gómez, J. D.; Moschou, S. P.; Garraffo, C.; Cohen, O. (18 March 2019). "Stellar Energetic Particles in the Magnetically Turbulent Habitable Zones of TRAPPIST-1-like Planetary Systems". The Astrophysical Journal. 874 (1): 21. arXiv:1902.03732. Bibcode:2019ApJ...874...21F. doi:10.3847/1538-4357/ab05e4. hdl:10150/633277. S2CID 119081355.
- Gabriel, Travis S. J.; Horn, Harrison W. (1 July 2021). "Dependencies of Mantle Shock Heating in Pairwise Accretion". The Astrophysical Journal Letters. 915 (2): L32. Bibcode:2021ApJ...915L..32G. doi:10.3847/2041-8213/abffd1. S2CID 235817461.
- "Gaia Early Data Release 3 (EDR3)". ESA Gaia Archive. European Space Agency. 2021. Retrieved 1 September 2023.
- Gargaud, Muriel; Amils, Ricardo; Quintanilla, José Cernicharo; Cleaves, Henderson James; Irvine, William M.; Pinti, Daniele L.; Viso, Michel, eds. (2011). Encyclopedia of Astrobiology. Berlin, Heidelberg: Springer Berlin Heidelberg. Bibcode:2011eab..book.....G. doi:10.1007/978-3-642-11274-4. ISBN 978-3-642-11271-3. S2CID 90186988.
- Gibb, Bruce C. (27 October 2022). "Beyond Hubble". Nature Chemistry. 14 (11): 1207–1209. Bibcode:2022NatCh..14.1207G. doi:10.1038/s41557-022-01080-2. ISSN 1755-4349. PMID 36302866. S2CID 253184326.
- Gillon, Michaël (February 2020). "Life under another Sun: From Science Fiction to Science". European Review. 28 (1): 18–39. doi:10.1017/S1062798719000267. hdl:2268/254712. ISSN 1062-7987. S2CID 210575064.
- Gillon, Michaël (2020). TRAPPIST-1: Toward the Comparative Study of Temperate Terrestrial Worlds (Report). pp. 49–52.
- Gillon, M.; Jehin, E.; Delrez, L.; Magain, P.; Opitom, C.; Sohy, S. (July 2013). SPECULOOS: search for habitable planets eclipsing ULtra-cOOl stars (PDF). Protostars and Planets VI. Heidelberg. hdl:2268/159868.
- Gillon, Michaël; Jehin, Emmanuël; Lederer, Susan M.; Delrez, Laetitia; de Wit, Julien; Burdanov, Artem; Van Grootel, Valérie; Burgasser, Adam J.; Triaud, Amaury H. M. J.; Opitom, Cyrielle; Demory, Brice-Olivier; Sahu, Devendra K.; Bardalez Gagliuffi, Daniella; Magain, Pierre; Queloz, Didier (May 2016). "Temperate Earth-sized planets transiting a nearby ultracool dwarf star". Nature. 533 (7602): 221–224. arXiv:1605.07211. Bibcode:2016Natur.533..221G. doi:10.1038/nature17448. ISSN 1476-4687. PMC 5321506. PMID 27135924.
- Gillon, Michaël; Triaud, Amaury H. M. J.; Demory, Brice-Olivier; Jehin, Emmanuël; Agol, Eric; Deck, Katherine M.; Lederer, Susan M.; de Wit, Julien; Burdanov, Artem; Ingalls, James G.; Bolmont, Emeline; Leconte, Jeremy; Raymond, Sean N.; Selsis, Franck; Turbet, Martin; Barkaoui, Khalid; Burgasser, Adam; Burleigh, Matthew R.; Carey, Sean J.; Chaushev, Aleksander; Copperwheat, Chris M.; Delrez, Laetitia; Fernandes, Catarina S.; Holdsworth, Daniel L.; Kotze, Enrico J.; Van Grootel, Valérie; Almleaky, Yaseen; Benkhaldoun, Zouhair; Magain, Pierre; Queloz, Didier (February 2017). "Seven temperate terrestrial planets around the nearby ultracool dwarf star TRAPPIST-1". Nature. 542 (7642): 456–460. arXiv:1703.01424. Bibcode:2017Natur.542..456G. doi:10.1038/nature21360. ISSN 1476-4687. PMC 5330437. PMID 28230125. S2CID 4391722.
- Gillon, Michaël; Meadows, Victoria; Agol, Eric; Burgasser, Adam J.; Deming, Drake; Doyon, René; Fortney, Jonathan; Kreidberg, Laura; Owen, James; Selsis, Franck; de Wit, Julien; Lustig-Yaeger, Jacob; Rackham, Benjamin V. (2 December 2020). "The TRAPPIST-1 JWST Community Initiative". Bulletin of the AAS. 52 (2): 0208. arXiv:2002.04798. Bibcode:2020BAAS...52.0208G. doi:10.3847/25c2cfeb.afbf0205. S2CID 211082517.
- Gizis, John E.; Monet, David G.; Reid, I. Neill; Kirkpatrick, J. Davy; Liebert, James; Williams, Rik J. (August 2000). "New Neighbors from 2MASS: Activity and Kinematics at the Bottom of the Main Sequence". The Astronomical Journal. 120 (2): 1085–1099. arXiv:astro-ph/0004361. Bibcode:2000AJ....120.1085G. doi:10.1086/301456. S2CID 18819321.
- Glaser, Donald M.; Hartnett, Hilairy Ellen; Desch, Steven J.; Unterborn, Cayman T.; Anbar, Ariel; Buessecker, Steffen; Fisher, Theresa; Glaser, Steven; Kane, Stephen R.; Lisse, Carey M.; Millsaps, Camerian; Neuer, Susanne; O’Rourke, Joseph G.; Santos, Nuno; Walker, Sara Imari; Zolotov, Mikhail (April 2020). "Detectability of Life Using Oxygen on Pelagic Planets and Water Worlds". The Astrophysical Journal. 893 (2): 163. arXiv:2004.03631. Bibcode:2020ApJ...893..163G. doi:10.3847/1538-4357/ab822d. ISSN 0004-637X.
- Glazier, Amy L.; Howard, Ward S.; Corbett, Hank; Law, Nicholas M.; Ratzloff, Jeffrey K.; Fors, Octavi; del Ser, Daniel (27 August 2020). "Evryscope and K2 Constraints on TRAPPIST-1 Superflare Occurrence and Planetary Habitability". The Astrophysical Journal. 900 (1): 27. arXiv:2006.14712. Bibcode:2020ApJ...900...27G. doi:10.3847/1538-4357/aba4a6. S2CID 220128346.
- Goldsmith, Donald (10 September 2018). Exoplanets: Hidden Worlds and the Quest for Extraterrestrial Life. Harvard University Press. Bibcode:2018ehwq.book.....G. doi:10.4159/9780674988897. ISBN 978-0-674-98889-7. S2CID 240182683.
- Gonzales, Eileen C.; Faherty, Jacqueline K.; Gagné, Jonathan; Teske, Johanna; McWilliam, Andrew; Cruz, Kelle (29 November 2019). "A Reanalysis of the Fundamental Parameters and Age of TRAPPIST-1". The Astrophysical Journal. 886 (2): 131. arXiv:1909.13859. Bibcode:2019ApJ...886..131G. doi:10.3847/1538-4357/ab48fc. S2CID 203594024.
- Grayver, Alexander; Bower, Dan J.; Saur, Joachim; Dorn, Caroline; Morris, Brett M. (7 December 2022). "Interior Heating of Rocky Exoplanets from Stellar Flares with Application to TRAPPIST-1". The Astrophysical Journal Letters. 941 (1): L7. arXiv:2211.06140. Bibcode:2022ApJ...941L...7G. doi:10.3847/2041-8213/aca287. S2CID 253499175.
- Grenfell, John Lee (13 November 2017). "A review of exoplanetary biosignatures". Physics Reports. 713: 1–17. arXiv:1710.03976. Bibcode:2017PhR...713....1G. doi:10.1016/j.physrep.2017.08.003. ISSN 0370-1573. S2CID 119400674.
- Grenfell, John Lee; Leconte, Jeremy; Forget, François; Godolt, Mareike; Carrión-González, Óscar; Noack, Lena; Tian, Feng; Rauer, Heike; Gaillard, Fabrice; Bolmont, Émeline; Charnay, Benjamin; Turbet, Martin (August 2020). "Possible Atmospheric Diversity of Low Mass Exoplanets – Some Central Aspects". Space Science Reviews. 216 (5): 98. arXiv:2101.01277. Bibcode:2020SSRv..216...98G. doi:10.1007/s11214-020-00716-4. ISSN 1572-9672. S2CID 225473867.
- Gressier, A.; Mori, M.; Changeat, Q.; Edwards, B.; Beaulieu, J.-P.; Marcq, E.; Charnay, B. (2022). "Near-infrared transmission spectrum of TRAPPIST-1 h using Hubble WFC3 G141 observations". Astronomy & Astrophysics. 658: A133. arXiv:2112.05510. Bibcode:2022A&A...658A.133G. doi:10.1051/0004-6361/202142140. ISSN 0004-6361. S2CID 245091619 – via arXiv.
- Grimm, Simon L.; Demory, Brice-Olivier; Gillon, Michaël; Dorn, Caroline; Agol, Eric; Burdanov, Artem; Delrez, Laetitia; Sestovic, Marko; Triaud, Amaury H. M. J.; Turbet, Martin; Bolmont, Émeline; Caldas, Anthony; Wit, Julien de; Jehin, Emmanuël; Leconte, Jérémy; Raymond, Sean N.; Van Grootel, Valérie; Burgasser, Adam J.; Carey, Sean; Fabrycky, Daniel; Heng, Kevin; Hernandez, David M.; Ingalls, James G.; Lederer, Susan; Selsis, Franck; Queloz, Didier (1 May 2018). "The nature of the TRAPPIST-1 exoplanets". Astronomy & Astrophysics. 613: A68. arXiv:1802.01377. Bibcode:2018A&A...613A..68G. doi:10.1051/0004-6361/201732233. ISSN 0004-6361. S2CID 3441829.
- Guimond, Claire Marie; Rudge, John F.; Shorttle, Oliver (1 March 2022). "Blue Marble, Stagnant Lid: Could Dynamic Topography Avert a Waterworld?". The Planetary Science Journal. 3 (3): 66. arXiv:2201.05636. Bibcode:2022PSJ.....3...66G. doi:10.3847/psj/ac562e. ISSN 2632-3338. S2CID 246015582.
- Guridi, Jose A.; Pertuze, Julio A.; Pfotenhauer, Sebastian M. (1 March 2020). "Natural laboratories as policy instruments for technological learning and institutional capacity building: The case of Chile's astronomy cluster". Research Policy. 49 (2): 103899. doi:10.1016/j.respol.2019.103899. ISSN 0048-7333. S2CID 197453914.
- Günther, Maximilian N.; Berardo, David A.; Ducrot, Elsa; Murray, Catriona A.; Stassun, Keivan G.; Olah, Katalin; Bouma, L. G.; Rappaport, Saul; Winn, Joshua N.; Feinstein, Adina D.; Matthews, Elisabeth C.; Sebastian, Daniel; Rackham, Benjamin V.; Seli, Bálint; J. Triaud, Amaury H. M.; Gillen, Edward; Levine, Alan M.; Demory, Brice-Olivier; Gillon, Michaël; Queloz, Didier; Ricker, George R.; Vanderspek, Roland K.; Seager, Sara; Latham, David W.; Jenkins, Jon M.; Brasseur, C. E.; Colón, Knicole D.; Daylan, Tansu; Delrez, Laetitia; Fausnaugh, Michael; Garcia, Lionel J.; Jayaraman, Rahul; Jehin, Emmanuel; Kristiansen, Martti H.; Kruijssen, J. M. Diederik; Pedersen, Peter Pihlmann; Pozuelos, Francisco J.; Rodriguez, Joseph E.; Wohler, Bill; Zhan, Zhuchang (1 April 2022). "Complex Modulation of Rapidly Rotating Young M Dwarfs: Adding Pieces to the Puzzle". The Astronomical Journal. 163 (4): 144. arXiv:2008.11681. Bibcode:2022AJ....163..144G. doi:10.3847/1538-3881/ac503c. S2CID 221319588.
- Gutiérrez, C. M.; Arnold, D.; Copley, D.; Copperwheat, C. M.; Harvey, E.; Jermak, H.; Knapen, J.; McGrath, A.; Oria, A.; Rebolo, R.; Steele, I. A.; Torres, M. (2019). "The new 4-m robotic telescope". Astronomische Nachrichten. 340 (1–3): 40–45. Bibcode:2019AN....340...40G. doi:10.1002/asna.201913556. ISSN 1521-3994. S2CID 133136386.
- Hakim, Kaustubh; Rivoldini, Attilio; Van Hoolst, Tim; Cottenier, Stefaan; Jaeken, Jan; Chust, Thomas; Steinle-Neumann, Gerd (1 October 2018). "A new ab initio equation of state of hcp-Fe and its implication on the interior structure and mass-radius relations of rocky super-Earths". Icarus. 313: 61–78. arXiv:1805.10530. Bibcode:2018Icar..313...61H. doi:10.1016/j.icarus.2018.05.005. ISSN 0019-1035. S2CID 119442637.
- National Astronomical Observatory of Japan (May 2022). Handbook of Scientific Tables. doi:10.1142/9789813278523_0001. ISBN 978-981-3278-53-0.
- Harbach, Laura M.; Moschou, Sofia P.; Garraffo, Cecilia; Drake, Jeremy J.; Alvarado-Gómez, Julián D.; Cohen, Ofer; Fraschetti, Federico (1 June 2021). "Stellar Winds Drive Strong Variations in Exoplanet Evaporative Outflow Patterns and Transit Absorption Signatures". The Astrophysical Journal. 913 (2): 130. arXiv:2012.05922. Bibcode:2021ApJ...913..130H. doi:10.3847/1538-4357/abf63a. S2CID 228375956.
- Heising, Matthew Z.; Sasselov, Dimitar D.; Hernquist, Lars; Luisa Tió Humphrey, Ana (1 June 2021). "How Flat Can a Planetary System Get? I. The Case of TRAPPIST-1". The Astrophysical Journal. 913 (2): 126. Bibcode:2021ApJ...913..126H. doi:10.3847/1538-4357/abf8a8. S2CID 219262616.
- Hori, Yasunori; Ogihara, Masahiro (28 January 2020). "Do the TRAPPIST-1 Planets Have Hydrogen-rich Atmospheres?". The Astrophysical Journal. 889 (2): 77. arXiv:1912.05749. Bibcode:2020ApJ...889...77H. doi:10.3847/1538-4357/ab6168. S2CID 209324289.
- Howard, Ward S.; Kowalski, Adam F.; Flagg, Laura; MacGregor, Meredith A.; Lim, Olivia; Radica, Michael; Piaulet, Caroline; Roy, Pierre-Alexis; Lafrenière, David; Benneke, Björn; Brown, Alexander; Espinoza, Néstor; Doyon, René; Coulombe, Louis-Philippe; Johnstone, Doug; Cowan, Nicolas B.; Jayawardhana, Ray; Turner, Jake D.; Dang, Lisa (1 December 2023). "Characterizing the Near-infrared Spectra of Flares from TRAPPIST-1 during JWST Transit Spectroscopy Observations". The Astrophysical Journal. 959 (1): 64. arXiv:2310.03792. Bibcode:2023ApJ...959...64H. doi:10.3847/1538-4357/acfe75.
- Howell, Steve B.; Everett, Mark E.; Horch, Elliott P.; Winters, Jennifer G.; Hirsch, Lea; Nusdeo, Dan; Scott, Nicholas J. (13 September 2016). "Speckle Imaging Excludes Low-Mass Companions Orbiting the Exoplanet Host Star Trappist-1". The Astrophysical Journal. 829 (1): L2. arXiv:1610.05269. Bibcode:2016ApJ...829L...2H. doi:10.3847/2041-8205/829/1/l2. S2CID 119183657.
- Howell, Steve B., ed. (September 2020). The NASA Kepler Mission. IOP Publishing. doi:10.1088/2514-3433/ab9823ch3. ISBN 978-0-7503-2296-6. S2CID 224941774.
- Hughes, Janette (2022). Hughes, Janette (ed.). Making, makers, makerspaces : the shift to making in 20 schools. Cham: Springer. doi:10.1007/978-3-031-09819-2. ISBN 978-3-031-09819-2. S2CID 251731356.
- Ih, Jegug; Kempton, Eliza M.-R.; Whittaker, Emily A.; Lessard, Madeline (July 2023). "Constraining the Thickness of TRAPPIST-1 b's Atmosphere from Its JWST Secondary Eclipse Observation at 15 μm". The Astrophysical Journal Letters. 952 (1): L4. arXiv:2305.10414. Bibcode:2023ApJ...952L...4I. doi:10.3847/2041-8213/ace03b. ISSN 2041-8205.
- Janjic, Aleksandar (2017). Lebensraum Universum. Bibcode:2017leun.book.....J. doi:10.1007/978-3-662-54787-8. ISBN 978-3-662-54786-1.
- "Comparison of TRAPPIST-1 to the Solar System". Jet Propulsion Laboratory. 22 January 2021. Retrieved 31 August 2023.
- Kaltenegger, L.; Faherty, J. K. (June 2021). "Past, present and future stars that can see Earth as a transiting exoplanet". Nature. 594 (7864): 505–507. arXiv:2107.07936. Bibcode:2021Natur.594..505K. doi:10.1038/s41586-021-03596-y. ISSN 1476-4687. PMID 34163055. S2CID 235626242.
- Kanas, Nick (2019). Star Maps: History, Artistry, and Cartography. Springer International Publishing. doi:10.1007/978-3-030-13613-0. ISBN 978-3-030-13612-3. S2CID 239353025.
- Kane, Stephen R.; Arney, Giada N.; Byrne, Paul K.; Dalba, Paul A.; Desch, Steven J.; Horner, Jonti; Izenberg, Noam R.; Mandt, Kathleen E.; Meadows, Victoria S.; Quick, Lynnae C. (February 2021). "The Fundamental Connections between the Solar System and Exoplanetary Science". Journal of Geophysical Research: Planets. 126 (2). arXiv:2012.11628. Bibcode:2021JGRE..12606643K. doi:10.1029/2020JE006643. ISSN 2169-9100. S2CID 233442891.
- Kane, Stephen R. (13 April 2017). "Worlds without Moons: Exomoon Constraints for Compact Planetary Systems". The Astrophysical Journal. 839 (2): L19. arXiv:1704.01688. Bibcode:2017ApJ...839L..19K. doi:10.3847/2041-8213/aa6bf2. S2CID 119380874.
- Kane, Stephen R.; Jansen, Tiffany; Fauchez, Thomas; Selsis, Franck; Ceja, Alma Y. (6 January 2021). "Phase Modeling of the TRAPPIST-1 Planetary Atmospheres". The Astronomical Journal. 161 (2): 53. arXiv:2012.00080. Bibcode:2021AJ....161...53K. doi:10.3847/1538-3881/abcfbe. S2CID 227238721.
- Kendall, M.; Byrne, P. K. (1 March 2020). Assessing Geological Conditions at the Ocean Floors of the TRAPPIST-1 Rocky Planets (PDF). 51st Lunar and Planetary Science Conference. The Woodlands, Texas. p. 3030. Bibcode:2020LPI....51.3030K.
- Kislyakova, K. G.; Noack, L.; Johnstone, C. P.; Zaitsev, V. V.; Fossati, L.; Lammer, H.; Khodachenko, M. L.; Odert, P.; Güdel, M. (December 2017). "Magma oceans and enhanced volcanism on TRAPPIST-1 planets due to induction heating". Nature Astronomy. 1 (12): 878–885. arXiv:1710.08761. Bibcode:2017NatAs...1..878K. doi:10.1038/s41550-017-0284-0. ISSN 2397-3366. S2CID 119429870.
- Kochukhov, Oleg (December 2021). "Magnetic fields of M dwarfs". The Astronomy and Astrophysics Review. 29 (1): 1. arXiv:2011.01781. Bibcode:2021A&ARv..29....1K. doi:10.1007/s00159-020-00130-3. ISSN 1432-0754. S2CID 226237078.
- Kopparla, Pushkar; Natraj, Vijay; Crisp, David; Bott, Kimberly; Swain, Mark R.; Yung, Yuk L. (10 September 2018). "Observing Oceans in Tightly Packed Planetary Systems: Perspectives from Polarization Modeling of the TRAPPIST-1 System". The Astronomical Journal. 156 (4): 143. Bibcode:2018AJ....156..143K. doi:10.3847/1538-3881/aad9a1. S2CID 125467757.
- Kral, Quentin; Wyatt, Mark C.; Triaud, Amaury H. M. J.; Marino, Sebastian; Thébault, Philippe; Shorttle, Oliver (11 September 2018). "Cometary impactors on the TRAPPIST-1 planets can destroy all planetary atmospheres and rebuild secondary atmospheres on planets f, g, and h". Monthly Notices of the Royal Astronomical Society. 479 (2): 2649–2672. arXiv:1802.05034. Bibcode:2018MNRAS.479.2649K. doi:10.1093/mnras/sty1677. ISSN 1365-2966. S2CID 118880067.
- Kral, Quentin; Davoult, Jeanne; Charnay, Benjamin (August 2020). "Formation of secondary atmospheres on terrestrial planets by late disk accretion". Nature Astronomy. 4 (8): 769–775. arXiv:2004.02496. Bibcode:2020NatAs...4..769K. doi:10.1038/s41550-020-1050-2. ISSN 2397-3366. S2CID 214802025.
- Krissansen-Totton, J.; Fortney, J. J. (1 July 2022). "Predictions for Observable Atmospheres of Trappist-1 Planets from a Fully Coupled Atmosphere–Interior Evolution Model". The Astrophysical Journal. 933 (1): 115. arXiv:2207.04164. Bibcode:2022ApJ...933..115K. doi:10.3847/1538-4357/ac69cb. S2CID 250374670.
- Lane, H. Chad; Gadbury, Matthew; Ginger, Jeff; Yi, Sherry; Comins, Neil; Henhapl, Jack; Rivera-Rogers, Aidan (28 November 2022). "Triggering STEM Interest With Minecraft in a Hybrid Summer Camp". Innovations in Remote Instruction. 3 (4). doi:10.1037/tmb0000077. S2CID 254344269.
- Lienhard, F.; Queloz, D.; Gillon, M.; Burdanov, A.; Delrez, L.; Ducrot, E.; Handley, W.; Jehin, E.; Murray, C. A.; Triaud, A. H. M. J.; Gillen, E.; Mortier, A.; Rackham, B. V. (2020). "Global Analysis of the TRAPPIST Ultra-Cool Dwarf Transit Survey". Monthly Notices of the Royal Astronomical Society. 497 (3): 3790–3808. arXiv:2007.07278. Bibcode:2020MNRAS.497.3790L. doi:10.1093/mnras/staa2054. ISSN 1365-2966. S2CID 220525769.
- Lim, Olivia; Benneke, Björn; Doyon, René; MacDonald, Ryan J.; Piaulet, Caroline; Artigau, Étienne; Coulombe, Louis-Philippe; Radica, Michael; L’Heureux, Alexandrine; Albert, Loïc; Rackham, Benjamin V.; Wit, Julien de; Salhi, Salma; Roy, Pierre-Alexis; Flagg, Laura; Fournier-Tondreau, Marylou; Taylor, Jake; Cook, Neil J.; Lafrenière, David; Cowan, Nicolas B.; Kaltenegger, Lisa; Rowe, Jason F.; Espinoza, Néstor; Dang, Lisa; Darveau-Bernier, Antoine (September 2023). "Atmospheric Reconnaissance of TRAPPIST-1 b with JWST/NIRISS: Evidence for Strong Stellar Contamination in the Transmission Spectra". The Astrophysical Journal Letters. 955 (1): L22. arXiv:2309.07047. Bibcode:2023ApJ...955L..22L. doi:10.3847/2041-8213/acf7c4. ISSN 2041-8205.
- Lincowski, Andrew P.; Meadows, Victoria S.; Zieba, Sebastian; Kreidberg, Laura; Morley, Caroline; Gillon, Michaël; Selsis, Franck; Agol, Eric; Bolmont, Emeline; Ducrot, Elsa; Hu, Renyu; Koll, Daniel D. B.; Lyu, Xintong; Mandell, Avi; Suissa, Gabrielle; Tamburo, Patrick (1 September 2023). "Potential Atmospheric Compositions of TRAPPIST-1 c Constrained by JWST/MIRI Observations at 15 μm". The Astrophysical Journal Letters. 955 (1): L7. arXiv:2308.05899. Bibcode:2023ApJ...955L...7L. doi:10.3847/2041-8213/acee02.
- Lingam, Manasvi; Loeb, Abraham (April 2018). "Physical constraints on the likelihood of life on exoplanets". International Journal of Astrobiology. 17 (2): 116–126. arXiv:1707.02996. Bibcode:2018IJAsB..17..116L. doi:10.1017/S1473550417000179. ISSN 1475-3006. S2CID 35978131.
- Lingam, Manasvi; Loeb, Abraham (July 2018). "Implications of Tides for Life on Exoplanets". Astrobiology. 18 (7): 967–982. arXiv:1707.04594. Bibcode:2018AsBio..18..967L. doi:10.1089/ast.2017.1718. ISSN 1531-1074. PMID 30010383. S2CID 51628150.
- Lingam, Manasvi; Loeb, Abraham (August 2018). "Limitations of Chemical Propulsion for Interstellar Escape from Habitable Zones Around Low-mass Stars". Research Notes of the AAS. 2 (3): 154. arXiv:1808.08141. Bibcode:2018RNAAS...2..154L. doi:10.3847/2515-5172/aadcf4. ISSN 2515-5172. S2CID 119470444.
- Lingam, Manasvi; Loeb, Abraham (11 June 2019). "Colloquium: Physical constraints for the evolution of life on exoplanets". Reviews of Modern Physics. 91 (2): 021002. arXiv:1810.02007. Bibcode:2019RvMP...91b1002L. doi:10.1103/RevModPhys.91.021002. S2CID 85501199.
- Lingam, Manasvi; Loeb, Abraham (1 June 2019). "Photosynthesis on habitable planets around low-mass stars". Monthly Notices of the Royal Astronomical Society. 485 (4): 5924–5928. arXiv:1901.01270. Bibcode:2019MNRAS.485.5924L. doi:10.1093/mnras/stz847. ISSN 1365-2966. S2CID 84843940.
- Lingam, Manasvi; Loeb, Abraham (April 2019). "Subsurface exolife". International Journal of Astrobiology. 18 (2): 112–141. arXiv:1711.09908. Bibcode:2019IJAsB..18..112L. doi:10.1017/S1473550418000083. ISSN 1475-3006. S2CID 102480854.
- Lingam, Manasvi; Loeb, Avi (21 June 2021). Life in the Cosmos. Harvard University Press. doi:10.4159/9780674259959. ISBN 978-0-674-25995-9. S2CID 242834912.
- Linsky, Jeffrey (2019). Host Stars and their Effects on Exoplanet Atmospheres: An Introductory Overview. Lecture Notes in Physics. Vol. 955. Springer International Publishing. doi:10.1007/978-3-030-11452-7. ISBN 978-3-030-11451-0. S2CID 181923774.
- Liu, Beibei; Ji, Jianghui (October 2020). "A tale of planet formation: from dust to planets". Research in Astronomy and Astrophysics. 20 (10): 164. arXiv:2009.02321. Bibcode:2020RAA....20..164L. doi:10.1088/1674-4527/20/10/164. S2CID 221507902.
- Luger, Rodrigo; Sestovic, Marko; Kruse, Ethan; Grimm, Simon L.; Demory, Brice-Olivier; Agol, Eric; Bolmont, Emeline; Fabrycky, Daniel; Fernandes, Catarina S.; Van Grootel, Valérie; Burgasser, Adam; Gillon, Michaël; Ingalls, James G.; Jehin, Emmanuël; Raymond, Sean N.; Selsis, Franck; Triaud, Amaury H. M. J.; Barclay, Thomas; Barentsen, Geert; Howell, Steve B.; Delrez, Laetitia; de Wit, Julien; Foreman-Mackey, Daniel; Holdsworth, Daniel L.; Leconte, Jérémy; Lederer, Susan; Turbet, Martin; Almleaky, Yaseen; Benkhaldoun, Zouhair; Magain, Pierre; Morris, Brett M.; Heng, Kevin; Queloz, Didier (22 May 2017). "A seven-planet resonant chain in TRAPPIST-1". Nature Astronomy. 1 (6): 0129. arXiv:1703.04166. Bibcode:2017NatAs...1E.129L. doi:10.1038/s41550-017-0129. ISSN 2397-3366. S2CID 54770728.
- Madhusudhan, Nikku (18 August 2019). "Exoplanetary Atmospheres: Key Insights, Challenges, and Prospects". Annual Review of Astronomy and Astrophysics. 57 (1): 617–663. arXiv:1904.03190. Bibcode:2019ARA&A..57..617M. doi:10.1146/annurev-astro-081817-051846. ISSN 0066-4146. S2CID 102350577.
- Madhusudhan, Nikku (2020). Exofrontiers: big questions in exoplanetary science. IOP Publishing. ISBN 978-0-7503-1472-5. OCLC 1285004266.
- Maltagliati, Luca (27 March 2017). "Exoplanets: Why should we care about TRAPPIST-1?". Nature Astronomy. 1 (4): 0104. Bibcode:2017NatAs...1E.104M. doi:10.1038/s41550-017-0104. ISSN 2397-3366. S2CID 125667140.
- Marino, S.; Wyatt, M. C.; Kennedy, G. M.; Kama, M.; Matrà, L.; Triaud, A. H. M. J.; Henning, Th. (11 March 2020). "Searching for a dusty cometary belt around TRAPPIST-1 with ALMA". Monthly Notices of the Royal Astronomical Society. 492 (4): 6067–6073. arXiv:1909.09158. Bibcode:2020MNRAS.492.6067M. doi:10.1093/mnras/staa266. ISSN 1365-2966. S2CID 202712440.
- Marov, M. Ya.; Shevchenko, I. I. (September 2020). "Exoplanets: nature and models". Physics-Uspekhi. 63 (9): 837–871. Bibcode:2020PhyU...63..837M. doi:10.3367/ufne.2019.10.038673. ISSN 1063-7869. S2CID 209965726.
- Martin, Rebecca G.; Livio, Mario (1 February 2022). "Asteroids and Life: How Special Is the Solar System?". The Astrophysical Journal Letters. 926 (2): L20. arXiv:2202.01352. Bibcode:2022ApJ...926L..20M. doi:10.3847/2041-8213/ac511c. S2CID 246485608.
- Martínez-Rodríguez, Héctor; Caballero, José Antonio; Cifuentes, Carlos; Piro, Anthony L.; Barnes, Rory (26 December 2019). "Exomoons in the Habitable Zones of M Dwarfs". The Astrophysical Journal. 887 (2): 261. arXiv:1910.12054. Bibcode:2019ApJ...887..261M. doi:10.3847/1538-4357/ab5640. S2CID 204904780.
- McDonough, William F.; Yoshizaki, Takashi (2 July 2021). "Terrestrial planet compositions controlled by accretion disk magnetic field". Progress in Earth and Planetary Science. 8 (1): 39. Bibcode:2021PEPS....8...39M. doi:10.1186/s40645-021-00429-4. ISSN 2197-4284. S2CID 235701559.
- McKay, Tristan (2021). A Semiotic Approach to Open Notations: Ambiguity as Opportunity. Elements in Music since 1945. Cambridge University Press. ISBN 978-1-108-81332-7.
- Meadows, Victoria S.; Schmidt, Britney E. (2020). Planetary astrobiology. University of Arizona Press. ISBN 978-0-8165-4006-8. OCLC 1096534611.
- Meadows, Victoria S.; Arney, Giada N.; Schwieterman, Edward W.; Lustig-Yaeger, Jacob; Lincowski, Andrew P.; Robinson, Tyler; Domagal-Goldman, Shawn D.; Deitrick, Russell; Barnes, Rory K.; Fleming, David P.; Luger, Rodrigo; Driscoll, Peter E.; Quinn, Thomas R.; Crisp, David (1 February 2018). "The Habitability of Proxima Centauri b: Environmental States and Observational Discriminants". Astrobiology. 18 (2): 133–189. arXiv:1608.08620. Bibcode:2018AsBio..18..133M. doi:10.1089/ast.2016.1589. ISSN 1531-1074. PMC 5820795. PMID 29431479.
- Miles-Páez, P. A.; Zapatero Osorio, M. R.; Pallé, E.; Metchev, S. A. (21 March 2019). "Time-resolved image polarimetry of TRAPPIST-1 during planetary transits". Monthly Notices of the Royal Astronomical Society: Letters. 484 (1): L38–L42. arXiv:1901.02041. Bibcode:2019MNRAS.484L..38M. doi:10.1093/mnrasl/slz001. ISSN 1745-3925. S2CID 119095657.
- Morley, Caroline V.; Kreidberg, Laura; Rustamkulov, Zafar; Robinson, Tyler; Fortney, Jonathan J. (22 November 2017). "Observing the Atmospheres of Known Temperate Earth-sized Planets with JWST". The Astrophysical Journal. 850 (2): 121. arXiv:1708.04239. Bibcode:2017ApJ...850..121M. doi:10.3847/1538-4357/aa927b.
- Morris, Brett M.; Agol, Eric; Davenport, James R. A.; Hawley, Suzanne L. (11 April 2018). "Possible Bright Starspots on TRAPPIST-1". The Astrophysical Journal. 857 (1): 39. arXiv:1803.04543. Bibcode:2018ApJ...857...39M. doi:10.3847/1538-4357/aab6a5. S2CID 55891098.
- Morris, Brett M.; Agol, Eric; Hebb, Leslie; Hawley, Suzanne L.; Gillon, Michaël; Ducrot, Elsa; Delrez, Laetitia; Ingalls, James; Demory, Brice-Olivier (17 August 2018). "Non-detection of Contamination by Stellar Activity in the Spitzer Transit Light Curves of TRAPPIST-1". The Astrophysical Journal Letters. 863 (2): L32. arXiv:1808.02808. Bibcode:2018ApJ...863L..32M. doi:10.3847/2041-8213/aad8aa. S2CID 53332500.
- Mullan, D. J.; Bais, H. P. (27 September 2018). "Photosynthesis on a Planet Orbiting an M Dwarf: Enhanced Effectiveness during Flares". The Astrophysical Journal. 865 (2): 101. arXiv:1807.05267. Bibcode:2018ApJ...865..101M. doi:10.3847/1538-4357/aadfd1. S2CID 119073856.
- Mullan, D. J.; Paudel, R. R. (27 February 2019). "Origin of Radio-quiet Coronal Mass Ejections in Flare Stars". The Astrophysical Journal. 873 (1): 1. arXiv:1902.00810. Bibcode:2019ApJ...873....1M. doi:10.3847/1538-4357/ab041b. S2CID 119420075.
- "Tesla Definition". National High Magnetic Field Laboratory. 18 November 2022. Retrieved 16 May 2023.
- Navarro, Thomas; Merlis, Timothy M.; Cowan, Nicolas B.; Gomez, Natalya (15 July 2022). "Atmospheric Gravitational Tides of Earth-like Planets Orbiting Low-mass Stars". The Planetary Science Journal. 3 (7): 162. arXiv:2207.06974. Bibcode:2022PSJ.....3..162N. doi:10.3847/PSJ/ac76cd. ISSN 2632-3338. S2CID 250526799.
- Ogihara, Masahiro; Kokubo, Eiichiro; Nakano, Ryuunosuke; Suzuki, Takeru K. (1 February 2022). "Rapid-then-slow migration reproduces mass distribution of TRAPPIST-1 system". Astronomy & Astrophysics. 658: A184. arXiv:2201.08840. Bibcode:2022A&A...658A.184O. doi:10.1051/0004-6361/202142354. ISSN 0004-6361. S2CID 246210342.
- O'Malley-James, Jack T.; Kaltenegger, L. (July 2017). "UV surface habitability of the TRAPPIST-1 system". Monthly Notices of the Royal Astronomical Society: Letters. 469 (1): L26–L30. arXiv:1702.06936. doi:10.1093/mnrasl/slx047. ISSN 1745-3933.
- O'Malley-James, Jack T.; Kaltenegger, Lisa (1 October 2019). "Biofluorescent Worlds – II. Biological fluorescence induced by stellar UV flares, a new temporal biosignature". Monthly Notices of the Royal Astronomical Society. 488 (4): 4530–4545. arXiv:1608.06930. doi:10.1093/mnras/stz1842. ISSN 1365-2966.
- Ormel, Chris W.; Liu, Beibei; Schoonenberg, Djoeke (1 August 2017). "Formation of TRAPPIST-1 and other compact systems". Astronomy & Astrophysics. 604: A1. arXiv:1703.06924. Bibcode:2017A&A...604A...1O. doi:10.1051/0004-6361/201730826. ISSN 0004-6361. S2CID 4606360.
- Paladini, Stefania (2019). The New Frontiers of Space: Economic Implications, Security Issues and Evolving Scenarios. Springer. ISBN 978-3-030-19941-8.
- "Churchill's big idea". Physics World. 30 (4): 3. April 2017. doi:10.1088/2058-7058/30/4/1. ISSN 2058-7058.
- Pidhorodetska, Daria; Fauchez, Thomas J.; Villanueva, Geronimo L.; Domagal-Goldman, Shawn D.; Kopparapu, Ravi K. (July 2020). "Detectability of Molecular Signatures on TRAPPIST-1e through Transmission Spectroscopy Simulated for Future Space-based Observatories". The Astrophysical Journal Letters. 898 (2): L33. arXiv:2001.01338. Bibcode:2020ApJ...898L..33P. doi:10.3847/2041-8213/aba4a1. hdl:11603/20595. S2CID 209862793.
- Pierrehumbert, Raymond T.; Hammond, Mark (5 January 2019). "Atmospheric Circulation of Tide-Locked Exoplanets". Annual Review of Fluid Mechanics. 51 (1): 275–303. Bibcode:2019AnRFM..51..275P. doi:10.1146/annurev-fluid-010518-040516. ISSN 0066-4189. S2CID 125645319.
- Pinchuk, Pavlo; Margot, Jean-Luc; Greenberg, Adam H.; Ayalde, Thomas; Bloxham, Chad; Boddu, Arjun; Chinchilla-Garcia, Luis Gerardo; Cliffe, Micah; Gallagher, Sara; Hart, Kira; Hesford, Brayden; Mizrahi, Inbal; Pike, Ruth; Rodger, Dominic; Sayki, Bade; Schneck, Una; Tan, Aysen; Xiao, Yinxue "Yolanda"; Lynch, Ryan S. (19 February 2019). "A Search for Technosignatures from TRAPPIST-1, LHS 1140, and 10 Planetary Systems in the Kepler Field with the Green Bank Telescope at 1.15–1.73 GHz". The Astronomical Journal. 157 (3): 122. arXiv:1901.04057. Bibcode:2019AJ....157..122P. doi:10.3847/1538-3881/ab0105. S2CID 113397518.
- Pineda, J. Sebastian; Hallinan, Gregg (24 October 2018). "A Deep Radio Limit for the TRAPPIST-1 System". The Astrophysical Journal. 866 (2): 155. arXiv:1806.00480. Bibcode:2018ApJ...866..155P. doi:10.3847/1538-4357/aae078. S2CID 119209821.
- Quick, Lynnae C.; Roberge, Aki; Mlinar, Amy Barr; Hedman, Matthew M. (August 2020). "Forecasting Rates of Volcanic Activity on Terrestrial Exoplanets and Implications for Cryovolcanic Activity on Extrasolar Ocean Worlds". Publications of the Astronomical Society of the Pacific. 132 (1014): 084402. Bibcode:2020PASP..132h4402Q. doi:10.1088/1538-3873/ab9504. ISSN 0004-6280. S2CID 219964895.
- Quick, Lynnae C.; Roberge, Aki; Mendoza, Guadalupe Tovar; Quintana, Elisa V.; Youngblood, Allison A. (1 October 2023). "Prospects for Cryovolcanic Activity on Cold Ocean Planets". The Astrophysical Journal. 956 (1): 29. Bibcode:2023ApJ...956...29Q. doi:10.3847/1538-4357/ace9b6.
- Radnóti, Katalin (1 May 2021). "Exoplanets in physics classes". Journal of Physics: Conference Series. 1929 (1): 012015. Bibcode:2021JPhCS1929a2015R. doi:10.1088/1742-6596/1929/1/012015. ISSN 1742-6596. S2CID 235591431.
- Ranjan, Sukrit; Wordsworth, Robin; Sasselov, Dimitar D. (11 July 2017). "The Surface UV Environment on Planets Orbiting M Dwarfs: Implications for Prebiotic Chemistry and the Need for Experimental Follow-up". The Astrophysical Journal. 843 (2): 110. arXiv:1705.02350. Bibcode:2017ApJ...843..110R. doi:10.3847/1538-4357/aa773e. S2CID 119502156.
- Raymond, Sean N.; Izidoro, Andre; Bolmont, Emeline; Dorn, Caroline; Selsis, Franck; Turbet, Martin; Agol, Eric; Barth, Patrick; Carone, Ludmila; Dasgupta, Rajdeep; Gillon, Michael; Grimm, Simon L. (25 November 2021). "An upper limit on late accretion and water delivery in the TRAPPIST-1 exoplanet system". Nature Astronomy. 6: 80–88. arXiv:2111.13351. doi:10.1038/s41550-021-01518-6. ISSN 2397-3366. S2CID 244668317.
- "Red Dwarf". COSMOS - The SAO Encyclopedia of Astronomy. Swinburne University of Technology. Retrieved 31 July 2022.
- Riber, Adrián García (June 2018). PLANETHESIZER: SONIFICATION CONCERT (PDF). The 24th International Conference on Auditory Display (ICAD 2018). Michigan Technological University.
- Rinaldi, David; Núñez Ferrer, Jorge (March 2017). "Cheers to a new solar system – and EU investment strategy. CEPS Commentary, 7 March 2017". CEPS.
- Roettenbacher, Rachael M.; Kane, Stephen R. (14 December 2017). "The Stellar Activity of TRAPPIST-1 and Consequences for the Planetary Atmospheres". The Astrophysical Journal. 851 (2): 77. arXiv:1711.02676. Bibcode:2017ApJ...851...77R. doi:10.3847/1538-4357/aa991e. S2CID 73535657.
- Rushby, Andrew J.; Shields, Aomawa L.; Wolf, Eric T.; Laguë, Marysa; Burgasser, Adam (26 November 2020). "The Effect of Land Albedo on the Climate of Land-dominated Planets in the TRAPPIST-1 System". The Astrophysical Journal. 904 (2): 124. arXiv:2011.03621. Bibcode:2020ApJ...904..124R. doi:10.3847/1538-4357/abbe04. S2CID 226281770.
- Sakaue, Takahito; Shibata, Kazunari (1 September 2021). "An M Dwarf's Chromosphere, Corona, and Wind Connection via Nonlinear Alfvén Waves". The Astrophysical Journal. 919 (1): 29. arXiv:2106.12752. Bibcode:2021ApJ...919...29S. doi:10.3847/1538-4357/ac0e34. S2CID 235624132.
- Samara, Evangelia; Patsourakos, Spiros; Georgoulis, Manolis K. (1 March 2021). "A Readily Implemented Atmosphere Sustainability Constraint for Terrestrial Exoplanets Orbiting Magnetically Active Stars". The Astrophysical Journal Letters. 909 (1): L12. arXiv:2102.07837. Bibcode:2021ApJ...909L..12S. doi:10.3847/2041-8213/abe416. S2CID 231933691.
- Schlichting, Hilke E.; Young, Edward D. (1 May 2022). "Chemical Equilibrium between Cores, Mantles, and Atmospheres of Super-Earths and Sub-Neptunes and Implications for Their Compositions, Interiors, and Evolution". The Planetary Science Journal. 3 (5): 127. arXiv:2107.10405. Bibcode:2022PSJ.....3..127S. doi:10.3847/psj/ac68e6. ISSN 2632-3338. S2CID 236171388.
- Schneider, J.; Dedieu, C.; Sidaner, P. Le; Savalle, R.; Zolotukhin, I. (1 August 2011). "Defining and cataloging exoplanets: the exoplanet.eu database". Astronomy & Astrophysics. 532: A79. arXiv:1106.0586. Bibcode:2011A&A...532A..79S. doi:10.1051/0004-6361/201116713. ISSN 0004-6361. S2CID 55994657.
- Schwieterman, Edward W.; Reinhard, Christopher T.; Olson, Stephanie L.; Harman, Chester E.; Lyons, Timothy W. (10 June 2019). "A Limited Habitable Zone for Complex Life". The Astrophysical Journal. 878 (1): 19. arXiv:1902.04720. Bibcode:2019ApJ...878...19S. doi:10.3847/1538-4357/ab1d52. S2CID 118948604.
- Scheidenberger, Christoph; Pfützner, Marek, eds. (2018). The Euroschool on Exotic Beams - Vol. 5. Lecture Notes in Physics. Vol. 948. doi:10.1007/978-3-319-74878-8. ISBN 978-3-319-74878-8. S2CID 220615062.
- Sein, Alexandr; Duncan, Colton; Zhong, Patrick; Koock, Elise; Lee, Waylon; Jakubik, Connor; McHenry, Neil; Bruno, Ashley; Chamitoff, Gregory (2021). "STEM Education Through Virtual Space System Design Competitions". AIAA Scitech 2021 Forum. AIAA Scitech 2021 Forum. American Institute of Aeronautics and Astronautics. doi:10.2514/6.2021-0481. ISBN 978-1-62410-609-5. S2CID 234272238.
- Shields, Aomawa L.; Ballard, Sarah; Johnson, John Asher (5 December 2016). "The habitability of planets orbiting M-dwarf stars". Physics Reports. 663: 1–38. arXiv:1610.05765. Bibcode:2016PhR...663....1S. doi:10.1016/j.physrep.2016.10.003. ISSN 0370-1573. S2CID 119248081.
- Shields, Aomawa L.; Carns, Regina C. (25 October 2018). "Hydrohalite Salt-albedo Feedback Could Cool M-dwarf Planets". The Astrophysical Journal. 867 (1): 11. arXiv:1808.09977. Bibcode:2018ApJ...867...11S. doi:10.3847/1538-4357/aadcaa. S2CID 76652437.
- Short, Kendra; Stapelfeldt, Karl (2017). Exoplanet exploration program update (PDF) (Report). NASA Exoplanet Exploration Program. Archived from the original (PDF) on 9 December 2021.
- Sleator, Roy D.; Smith, Niall (4 May 2017). "TRAPPIST-1: The dawning of the age of Aquarius". Bioengineered. 8 (3): 194–195. doi:10.1080/21655979.2017.1306998. ISSN 2165-5979. PMC 5470511. PMID 28324663.
- Snellen, Ignas A. G. (February 2017). "Earth's seven sisters". Nature. 542 (7642): 421–422. doi:10.1038/542421a. hdl:1887/75076. ISSN 1476-4687. PMID 28230129. S2CID 205092857.
- Srinivas, Susheela (August 2017). "Are There Habitable Worlds Out There? – The Quest for Exoplanets" (PDF). Science Reporter. 54 (8): 14–20. ISSN 0036-8512.
- Stevenson, David S. (2019). Red Dwarfs: Their Geological, Chemical, and Biological Potential for Life. Springer International Publishing. doi:10.1007/978-3-030-25550-3. ISBN 978-3-030-25549-7. S2CID 203546646.
- Teixeira, Katie E.; Morley, Caroline V.; Foley, Bradford J.; Unterborn, Cayman T. (December 2023). "The Carbon-deficient Evolution of TRAPPIST-1c". The Astrophysical Journal. 960 (1): 44. arXiv:2311.17699. doi:10.3847/1538-4357/ad0cec. ISSN 0004-637X.
- Turbet, Martin; Bolmont, Emeline; Leconte, Jeremy; Forget, François; Selsis, Franck; Tobie, Gabriel; Caldas, Anthony; Naar, Joseph; Gillon, Michaël (1 April 2018). "Modeling climate diversity, tidal dynamics and the fate of volatiles on TRAPPIST-1 planets". Astronomy & Astrophysics. 612: A86. arXiv:1707.06927. Bibcode:2018A&A...612A..86T. doi:10.1051/0004-6361/201731620. ISSN 0004-6361. S2CID 53990543.
- Turbet, Martin; Bolmont, Emeline; Bourrier, Vincent; Demory, Brice-Olivier; Leconte, Jérémy; Owen, James; Wolf, Eric T. (August 2020). "A Review of Possible Planetary Atmospheres in the TRAPPIST-1 System". Space Science Reviews. 216 (5): 100. arXiv:2007.03334. Bibcode:2020SSRv..216..100T. doi:10.1007/s11214-020-00719-1. ISSN 1572-9672. PMC 7378127. PMID 32764836.
- Wang, Jessie (1 June 2022). "Law of Gravity Blurred by Perturbed Planetary Orbits for Alien Observers". Journal of Physics: Conference Series. 2287 (1): 012039. Bibcode:2022JPhCS2287a2039W. doi:10.1088/1742-6596/2287/1/012039. ISSN 1742-6596. S2CID 250290787.
- Wheatley, Peter J.; Louden, Tom; Bourrier, Vincent; Ehrenreich, David; Gillon, Michaël (11 February 2017). "Strong XUV irradiation of the Earth-sized exoplanets orbiting the ultracool dwarf TRAPPIST-1". Monthly Notices of the Royal Astronomical Society: Letters. 465 (1): L74–L78. arXiv:1605.01564. Bibcode:2017MNRAS.465L..74W. doi:10.1093/mnrasl/slw192. ISSN 1745-3933. S2CID 30087787.
- Wilson, David J.; Froning, Cynthia S.; Duvvuri, Girish M.; France, Kevin; Youngblood, Allison; Schneider, P. Christian; Berta-Thompson, Zachory; Brown, Alexander; Buccino, Andrea P.; Hawley, Suzanne; Irwin, Jonathan; Kaltenegger, Lisa; Kowalski, Adam; Linsky, Jeffrey; Parke Loyd, R. O.; Miguel, Yamila; Pineda, J. Sebastian; Redfield, Seth; Roberge, Aki; Rugheimer, Sarah; Tian, Feng; Vieytes, Mariela (1 April 2021). "The Mega-MUSCLES Spectral Energy Distribution of TRAPPIST-1". The Astrophysical Journal. 911 (1): 18. arXiv:2102.11415. Bibcode:2021ApJ...911...18W. doi:10.3847/1538-4357/abe771. S2CID 232014177.
- Wolf, Eric T. (6 April 2017). "Assessing the Habitability of the TRAPPIST-1 System Using a 3D Climate Model". The Astrophysical Journal Letters. 839 (1): L1. arXiv:1703.05815. Bibcode:2017ApJ...839L...1W. doi:10.3847/2041-8213/aa693a. S2CID 119082049.
- Wunderlich, Fabian; Scheucher, Markus; Godolt, M.; Grenfell, J. L.; Schreier, F.; Schneider, P. C.; Wilson, D. J.; Sánchez-López, A.; López-Puertas, M.; Rauer, H. (29 September 2020). "Distinguishing between Wet and Dry Atmospheres of TRAPPIST-1 e and f". The Astrophysical Journal. 901 (2): 126. arXiv:2006.11349. Bibcode:2020ApJ...901..126W. doi:10.3847/1538-4357/aba59c. S2CID 219966834.
- Valio, Adriana; Estrela, Raissa; Cabral, Luisa; Grangeiro, Abel (August 2018). "The biological impact of superflares on planets in the Habitable Zone". Proceedings of the International Astronomical Union. 14 (S345): 176–180. doi:10.1017/S1743921319002035. ISSN 1743-9213. S2CID 216905441.
- Van Hoolst, Tim; Noack, Lena; Rivoldini, Attilio (1 January 2019). "Exoplanet interiors and habitability". Advances in Physics: X. 4 (1): 1630316. Bibcode:2019AdPhX...430316V. doi:10.1080/23746149.2019.1630316. S2CID 198417434.
- Veras, Dimitri; Breedt, Elmé (1 July 2017). "Eclipse, transit and occultation geometry of planetary systems at exo-syzygy". Monthly Notices of the Royal Astronomical Society. 468 (3): 2672–2683. arXiv:1703.03414. doi:10.1093/mnras/stx614. ISSN 1365-2966.
- Vida, Krisztián; Kővári, Zsolt; Pál, András; Oláh, Katalin; Kriskovics, Levente (2 June 2017). "Frequent flaring in the TRAPPIST-1 system – unsuited for life?". The Astrophysical Journal. 841 (2): 124. arXiv:1703.10130. Bibcode:2017ApJ...841..124V. doi:10.3847/1538-4357/aa6f05. S2CID 118827117.
- Vinson, Alec M.; Tamayo, Daniel; Hansen, Brad M. S. (1 August 2019). "The Chaotic Nature of TRAPPIST-1 Planetary Spin States". Monthly Notices of the Royal Astronomical Society. 488 (4): 5739–5747. arXiv:1905.11419. Bibcode:2019MNRAS.488.5739V. doi:10.1093/mnras/stz2113. ISSN 1365-2966. S2CID 167217467.
- Yang, J.; Ji, W. (1 December 2018). Proxima b, TRAPPIST 1e, and LHS 1140b: Increased Ice Coverages by Sea Ice Dynamics. American Geophysical Union, Fall Meeting 2018. AGU Fall Meeting Abstracts. Vol. 2018. Washington DC. pp. P43G–3826. Bibcode:2018AGUFM.P43G3826Y.
- Zanazzi, J. J.; Lai, Dong (11 August 2017). "Triaxial deformation and asynchronous rotation of rocky planets in the habitable zone of low-mass stars". Monthly Notices of the Royal Astronomical Society. 469 (3): 2879–2885. arXiv:1702.07327. Bibcode:2017MNRAS.469.2879Z. doi:10.1093/mnras/stx1076. ISSN 1365-2966. S2CID 119430179.
- Zanazzi, J. J.; Triaud, Amaury H. M. J. (1 June 2019). "The ability of significant tidal stress to initiate plate tectonics". Icarus. 325: 55–66. arXiv:1711.09898. Bibcode:2019Icar..325...55Z. doi:10.1016/j.icarus.2019.01.029. ISSN 0019-1035. S2CID 96450290.
- Zhang, Xi (July 2020). "Atmospheric regimes and trends on exoplanets and brown dwarfs". Research in Astronomy and Astrophysics. 20 (7): 099. arXiv:2006.13384. Bibcode:2020RAA....20...99Z. doi:10.1088/1674-4527/20/7/99. ISSN 1674-4527. S2CID 220042096.
- Zhang, Zhanbo; Zhou, Yifan; Rackham, Benjamin V.; Apai, Dániel (4 October 2018). "The Near-infrared Transmission Spectra of TRAPPIST-1 Planets b, c, d, e, f, and g and Stellar Contamination in Multi-epoch Transit Spectra". The Astronomical Journal. 156 (4): 178. arXiv:1802.02086. Bibcode:2018AJ....156..178Z. doi:10.3847/1538-3881/aade4f. hdl:10150/631598. S2CID 118938032.
- Greene, Thomas P.; Bell, Taylor J.; Ducrot, Elsa; Dyrek, Achrène; Lagage, Pierre-Olivier; Fortney, Jonathan J. (March 2023). "Thermal Emission from the Earth-sized Exoplanet TRAPPIST-1 b using JWST". Nature. 618 (7963): 39–42. arXiv:2303.14849. Bibcode:2023Natur.618...39G. doi:10.1038/s41586-023-05951-7. PMID 36972683. S2CID 257767242.
Further reading
[edit]- Arcand, Kimberly K.; Price, Sara R.; Watzke, Megan (2020). "Holding the Cosmos in Your Hand: Developing 3D Modeling and Printing Pipelines for Communications and Research". Frontiers in Earth Science. 8: 541. arXiv:2012.02789. Bibcode:2020FrEaS...8..541A. doi:10.3389/feart.2020.590295. ISSN 2296-6463.
- Dzombeta, Krstinja; Percy, John (31 October 2019). Flare Stars: A Short Review (Report).
- Fauchez, Thomas J.; Turbet, Martin; Wolf, Eric T.; Boutle, Ian; Way, Michael J.; Del Genio, Anthony D.; Mayne, Nathan J.; Tsigaridis, Konstantinos; Kopparapu, Ravi K.; Yang, Jun; Forget, Francois; Mandell, Avi; Domagal Goldman, Shawn D. (21 February 2020). "TRAPPIST-1 Habitable Atmosphere Intercomparison (THAI): motivations and protocol version 1.0". Geoscientific Model Development. 13 (2): 707–716. arXiv:2002.10950. Bibcode:2020GMD....13..707F. doi:10.5194/gmd-13-707-2020. ISSN 1991-959X. S2CID 211296491.
External links
[edit]
- "The discovery team's official website". TRAPPIST.one.
- "Ultracool dwarf with planets". ESOcast 83. European Southern Observatory.