Nonmetal: Difference between revisions
trim redundant cite |
Update citation |
||
Line 36: | Line 36: | ||
==Definition and applicable elements== |
==Definition and applicable elements== |
||
[[File:Arsen 1a.jpg|thumb|Like [[carbon]], [[arsenic]] (here sealed in a container to prevent [[tarnishing]]) vaporises rather than melts when heated. The vapor is lemon-yellow and smells like [[garlic]].<ref>[[#Parkes1943|Parkes & Mellor 1943, p. 740]]</ref> The chemistry of arsenic is predominately nonmetallic in nature.<ref |
[[File:Arsen 1a.jpg|thumb|Like [[carbon]], [[arsenic]] (here sealed in a container to prevent [[tarnishing]]) vaporises rather than melts when heated. The vapor is lemon-yellow and smells like [[garlic]].<ref>[[#Parkes1943|Parkes & Mellor 1943, p. 740]]</ref> The chemistry of arsenic is predominately nonmetallic in nature.<ref>[[#Pascoe|Pascoe 1982, p. 3]]</ref>|alt=Two dull silver clusters of crystalline shards.]] |
||
A nonmetal is a [[chemical element]] characterized, in general, by its relatively low [[density]] and high [[electronegativity]]. More broadly speaking, nonmetals are distinguished by their deficiency in metallic properties, including attributes such as luster or shininess, the ability to be [[malleability|flattened into thin sheets]] or [[ductility|drawn into wires]], efficient [[Thermal conductivity|thermal]] and [[electrical conductivity]], and the tendency to produce [[Base (chemistry)|basic]] rather than [[acid]]ic [[oxide]]s when combined with oxygen.<ref>[[#Glinka1958|Glinka 1958, p. 77]]; [[#Oxtoby2015|Oxtoby, Gillis & Butler 2015, p. I.23]]</ref> The classification of elements as nonmetals lacks a universally stringent definition,<ref>[[#Godovikov|Godovikov & Nenasheva 2020, p. 4]]; [[#Sanderson57|Sanderson 1957, p. 229]]; [[#Morley|Morely & Muir 1892, p. 241]]</ref> resulting in variations among sources regarding which elements are included within this category. The criteria applied for such categorization hinge upon the specific properties that are deemed most indicative of a nonmetallic or metallic character.<ref name=Kneen218>[[#Kneen1972|Kneen, Rogers & Simpson 1972, pp. 218–219]]</ref>{{#tag:ref|Metallic or nonmetallic character is usually taken to be indicated by [[#Suggested_distinguishing_criteria|one property rather than two or more properties]].|group=n}} |
A nonmetal is a [[chemical element]] characterized, in general, by its relatively low [[density]] and high [[electronegativity]]. More broadly speaking, nonmetals are distinguished by their deficiency in metallic properties, including attributes such as luster or shininess, the ability to be [[malleability|flattened into thin sheets]] or [[ductility|drawn into wires]], efficient [[Thermal conductivity|thermal]] and [[electrical conductivity]], and the tendency to produce [[Base (chemistry)|basic]] rather than [[acid]]ic [[oxide]]s when combined with oxygen.<ref>[[#Glinka1958|Glinka 1958, p. 77]]; [[#Oxtoby2015|Oxtoby, Gillis & Butler 2015, p. I.23]]</ref> The classification of elements as nonmetals lacks a universally stringent definition,<ref>[[#Godovikov|Godovikov & Nenasheva 2020, p. 4]]; [[#Sanderson57|Sanderson 1957, p. 229]]; [[#Morley|Morely & Muir 1892, p. 241]]</ref> resulting in variations among sources regarding which elements are included within this category. The criteria applied for such categorization hinge upon the specific properties that are deemed most indicative of a nonmetallic or metallic character.<ref name=Kneen218>[[#Kneen1972|Kneen, Rogers & Simpson 1972, pp. 218–219]]</ref>{{#tag:ref|Metallic or nonmetallic character is usually taken to be indicated by [[#Suggested_distinguishing_criteria|one property rather than two or more properties]].|group=n}} |
||
Line 213: | Line 213: | ||
=== Metalloid === |
=== Metalloid === |
||
{{main|Metalloid}} |
{{main|Metalloid}} |
||
[[File:Realgar-229713.jpg|thumb|A crystal of [[realgar]], also known as "ruby sulphur" or "ruby of arsenic", an arsenic sulfide mineral As<sub>4</sub>S<sub>4</sub>. The two elements involved each have a predominately nonmetallic chemistry.<ref |
[[File:Realgar-229713.jpg|thumb|A crystal of [[realgar]], also known as "ruby sulphur" or "ruby of arsenic", an arsenic sulfide mineral As<sub>4</sub>S<sub>4</sub>. The two elements involved each have a predominately nonmetallic chemistry.<ref>[[#Bailar1965|Bailar, Moeller & Kleinberg 1965, p. 477]]</ref><ref>[[#Mee|Mee 1964, p. 153]]</ref>|alt=a cluster of bright cherry-red crystals]] |
||
The category of metalloids encompasses six elements that are commonly recognized as such: boron, silicon, germanium, arsenic, antimony, and tellurium, all of which exhibit a metallic appearance. On the standard periodic table, these elements occupy a diagonal region within the p-block, extending from boron in the upper left to tellurium in the lower right. This region straddles the [[dividing line between metals and nonmetals|boundary between metals and nonmetals]], as depicted on some periodic tables.<ref name="Vernon2013"/> |
The category of metalloids encompasses six elements that are commonly recognized as such: boron, silicon, germanium, arsenic, antimony, and tellurium, all of which exhibit a metallic appearance. On the standard periodic table, these elements occupy a diagonal region within the p-block, extending from boron in the upper left to tellurium in the lower right. This region straddles the [[dividing line between metals and nonmetals|boundary between metals and nonmetals]], as depicted on some periodic tables.<ref name="Vernon2013"/> |
||
Line 868: | Line 868: | ||
* <span id="Partington"></span>Partington JR 1944, ''A Text-book of Inorganic Chemistry'', 5th ed., Macmillan & Co., London |
* <span id="Partington"></span>Partington JR 1944, ''A Text-book of Inorganic Chemistry'', 5th ed., Macmillan & Co., London |
||
* <span id="Partington1964"></span>Partington JR 1964, ''A history of chemistry,'' vol. 4, Macmillan, London |
* <span id="Partington1964"></span>Partington JR 1964, ''A history of chemistry,'' vol. 4, Macmillan, London |
||
* <span id="Pascoe></span>Pascoe KJ 1982, ''An Introduction to the Properties of Engineering Materials,'' 3rd ed., Von Nostrand Reinhold (UK), Wokingham, Berkshire, {{ISBN|978-0-442-30233-7}} |
|||
* <span id="Petruševski"></span>Petruševski VM & Cvetković J 2018, "On the 'true position' of hydrogen in the Periodic Table", ''Foundations of Chemistry'', vol. 20, pp. 251–260, {{doi|10.1007/s10698-018-9306-y}} |
* <span id="Petruševski"></span>Petruševski VM & Cvetković J 2018, "On the 'true position' of hydrogen in the Periodic Table", ''Foundations of Chemistry'', vol. 20, pp. 251–260, {{doi|10.1007/s10698-018-9306-y}} |
||
* <span id="Phillips1965"></span>Phillips CSG & Williams RJP 1965, ''Inorganic Chemistry'', vol. 1, Principles and non-metals, Clarendon Press, Oxford |
* <span id="Phillips1965"></span>Phillips CSG & Williams RJP 1965, ''Inorganic Chemistry'', vol. 1, Principles and non-metals, Clarendon Press, Oxford |
Revision as of 12:02, 19 September 2023
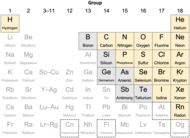
Periodic table excerpt (full table below) showing how often elements are effectively classified as nonmetals:
Nearby metals have a white background.
Lack of consensus in the definition of nonmetal causes different authors and sources to categorize different elements in this category.[n 1]
_____
† While hydrogen (H) is typically positioned within group 1 of the periodic table, as depicted in the full table below, some representations may place it above fluorine (F) in group 17.[n 2]
‡ Theory and experimental evidence suggest astatine (At) is a metal;[4] it is treated as such in this article.

A nonmetal is a type of chemical element that, in general, has a low density and high electronegativity (the ability of an atom in a molecule to attract electrons to itself). They encompass a diverse selection of elements, ranging from colorless gases like hydrogen to solid substances with a shiny appearance, such as carbon in its graphite form. Nonmetals are usually poor conductors of heat and electricity. When they exist in solid form, they tend to be brittle or crumbly owing to the limited mobility of their electrons. This stands in contrast to metals, which are known for their efficient electrical conductivity and malleability, often being readily transformed into thin sheets and drawn into wires due to the free movement of their electrons. Additionally, whereas compounds of metals tend to be basic in nature those of nonmetals tend to be acidic.
Within the realm of elemental composition, two nonmetals, namely hydrogen and helium, constitute an overwhelming 99% of the observable ordinary matter in the universe by mass. Moreover, five nonmetallic elements, namely hydrogen, carbon, nitrogen, oxygen, and silicon, constitute the majority of the Earth's crust, atmosphere, oceans and biosphere, underscoring their pivotal role in the composition of the planet.
The unique properties exhibited by nonmetallic elements render them essential for various specialized applications, often complementing the capabilities of metallic elements. Vital to the composition of living organisms are the nonmetals hydrogen, oxygen, carbon, and nitrogen, which constitute a significant portion of their structural makeup. Beyond biology, nonmetallic elements play crucial roles in several industries, including electronics, energy storage, agriculture, and chemical production.
While the term "non-metallic" has roots dating back to as far as 1566, there is no widely agreed precise definition of a nonmetal. This ambiguity arises from the existence of elements that exhibit a marked combination of metallic and nonmetallic attributes. The classification of such borderline cases as nonmetals varies depending on the specific criteria employed for classification. Generally, the number of elements recognized as nonmetals falls within the range of 14 to 23 (or 24), reflecting the fluid nature of this classification.
Definition and applicable elements

A nonmetal is a chemical element characterized, in general, by its relatively low density and high electronegativity. More broadly speaking, nonmetals are distinguished by their deficiency in metallic properties, including attributes such as luster or shininess, the ability to be flattened into thin sheets or drawn into wires, efficient thermal and electrical conductivity, and the tendency to produce basic rather than acidic oxides when combined with oxygen.[7] The classification of elements as nonmetals lacks a universally stringent definition,[8] resulting in variations among sources regarding which elements are included within this category. The criteria applied for such categorization hinge upon the specific properties that are deemed most indicative of a nonmetallic or metallic character.[9][n 3]
While Steudel, in his 2020 textbook Chemistry of the Non-metals[10][n 4] compiled a list of twenty-three chemical elements identified as nonmetals, any such list is open to debate and revision.[11] Among these elements, fourteen are commonly and consistently recognized as nonmetals, encompassing hydrogen, oxygen, nitrogen, and sulfur, as well as the highly reactive halogens: fluorine, chlorine, bromine, and iodine.[11] The noble gases, including helium, neon, argon, krypton, xenon, and radon, are also unequivocally categorized as nonmetals, a classification endorsed by Hawley's Condensed Chemical Dictionary.[11]
A degree of ambiguity surrounds the classification of carbon, phosphorus, and selenium, which have usually been designated as nonmetals, while some other sources have considered them as metalloids.[12] Elements traditionally categorized as metalloids, such as boron; silicon and germanium; arsenic and antimony; and tellurium are sometimes positioned as an intermediary class between metals and nonmetals when the criteria employed for differentiation prove inconclusive.[13] Conversely, these elements may also be classified as nonmetals due to their predominantly nonmetallic nature, characterized by weakly acidic chemistry.[14]
Out of the 118 known chemical elements,[15] approximately 20% fall under the classification of nonmetals.[16] However, the status of a select few elements remains subject to ongoing scrutiny and debate. Astatine, which is the fifth element among the halogens, often garners limited attention due to its extreme rarity and intense radioactivity.[17] That said, both theoretical considerations and experimental evidence have suggested that astatine is a metal.[4][n 5] Further contributing to the evolving landscape of elemental classification are the superheavy elements, including copernicium (element 112), flerovium (element 114), and oganesson (element 118). While initial indications point to the possibility of these elements being categorized as nonmetals, their definitive classification has yet to be confirmed.[20]
General properties
- Properties noted in this section refer to the elements in their most stable forms in ambient conditions unless otherwise mentioned
Physical
of some nonmetallic elements
Approximately half of nonmetallic elements exist in gaseous states, with the majority of the remainder being lustrous solids. Notably, bromine stands as the singular nonmetal that manifests as a liquid, exhibiting such volatility that it often maintains a layer of vapor above its surface. Among solid nonmetals, sulfur is the sole example characterized by a distinct coloration.[n 6] The fluid nonmetals are distinguished by their relatively low densities, as well as low melting and boiling points, coupled with a deficiency in their ability to conduct heat and electricity effectively.[23] Solid nonmetals, on the other hand, exhibit low densities, brittleness, limited mechanical and structural strength,[24] and a variable range of electrical conductivity, ranging from poor to moderate.[n 7]
The varied internal structures and bonding arrangements of the nonmetals explain their differences in form. Those nonmetals that exist as isolated atoms, such as xenon, or as discrete molecules, as seen in oxygen, sulfur, and bromine, typically display low melting and boiling points. Many of them remain in the gaseous state at room temperature, due to the weak London dispersion forces that govern the interactions between their atoms or molecules.[28] Conversely, nonmetals that assemble into large-scale structures, such as chains consisting of up to 1,000 atoms, exemplified by selenium,[29] two-dimensional sheets like carbon in its graphite form,[30] or three-dimensional lattices, as observed in silicon,[31] tend to possess higher melting and boiling points. These nonmetals invariably manifest as solids because the energy required to overcome their stronger covalent bonds is considerably higher.[32] In certain instances, nonmetals positioned closer to the left side of the periodic table or further down a given column may exhibit some weak metallic interactions between their molecules, chains, or layers. This phenomenon aligns with their proximity to metallic elements on the periodic table. Such interactions are discernible in boron,[33] carbon,[34] phosphorus,[35] arsenic,[36] selenium,[37] antimony,[38] tellurium[39] and iodine.[40]
Nonmetallic elements exhibit a wide range of visual characteristics, encompassing shiny appearances, distinct colors, or a lack of color altogether. The lustrous sheen observed in elements such as boron, graphitic carbon, silicon, black phosphorus, germanium, arsenic, selenium, antimony, tellurium, and iodine can be attributed to their structural arrangements, which entail varying degrees of delocalized (free-moving) electrons. These electrons disperse incoming visible light,[41] resulting in the observed metallic shine. On the other hand, colored nonmetals, which include sulfur, fluorine, chlorine, and bromine, possess the ability to selectively absorb certain colors (wavelengths) of light while transmitting others. This selective absorption and transmission lead to the exhibition of distinct colors. For example, chlorine, as noted by Elliot,[42] displays a notable yellow-green hue, which arises from its capacity to absorb a broad range of wavelengths in the violet and blue regions of the electromagnetic spectrum.[n 8] In the case of colorless nonmetals, namely hydrogen, nitrogen, oxygen, and the noble gases, the binding forces that hold their electrons are sufficiently robust. Consequently, these nonmetals do not undergo any absorption within the visible portion of the electromagnetic spectrum, thereby allowing for the complete transmission of all visible light.[44]
The electrical and thermal conductivities, as well as the brittleness observed in nonmetals, can be attributed to their internal atomic arrangements. In contrast to metals, where the presence of freely moving and evenly distributed electrons[45] facilitates high conductivity and malleability, nonmetals generally exhibit limited electron mobility.[46] Noteworthy electrical and thermal conductivity is observed in a select few nonmetals, namely carbon, arsenic, and antimony.[n 9] Exceptional thermal conductivity is also evident in boron, silicon, phosphorus, and germanium,[25] with this property resulting from the transmission of thermal energy through vibrational movements within their crystalline lattices.[47] Moderate electrical conductivity is discernible in a broader set of nonmetals, including boron, silicon, phosphorus, germanium, selenium, tellurium, and iodine.[n 10] Moreover, plasticity—a quality associated with malleability and ductility—is observed in specific nonmetals under unique conditions. Notable instances include exfoliated(expanded) graphite[49][50] and carbon nanotube wires in carbon,[51] white phosphorus (which remains pliable at room temperature and can be cut with a knife),[52] plastic sulfur,[53] and selenium wires, drawn from the molten state.[54]
The physical differences between metals and nonmetals are a result of both internal and external atomic interactions. Internally, the positive charge stemming from the protons within an atom's nucleus exerts a stabilizing influence, preventing the outer electrons from moving freely. Externally, these same electrons experience attractive forces emanating from the protons in neighboring atoms. When the external forces surpass, or are equivalent to, the internal forces, the outer electrons acquire the ability to move freely between atoms, thus leading to the manifestation of metallic properties. Conversely, when the internal forces predominate, nonmetallic properties are the anticipated outcome.[55]
Allotropes
Aspect | Metals | Nonmetals |
---|---|---|
Electronegativity | Lower than nonmetals, with some exceptions[57] |
Relatively high |
Chemical bonding | ||
Seldom form covalent bonds |
Frequently form covalent bonds | |
Metallic bonds (alloys) between metals |
Covalent bonds between nonmetals | |
Ionic bonds between nonmetals and metals | ||
Oxidation states |
Positive | Negative or positive |
Oxides | Basic in lower oxides; increasingly acidic in higher oxides |
Acidic; never basic[58] |
In aqueous solution[59] |
Exist as cations | Exist as anions or oxyanions |
Many nonmetallic elements exhibit various allotropic forms, each with its distinct physical properties that may vary between metallic and nonmetallic.[60] For instance, carbon, a versatile nonmetal, can manifest as graphite, diamond, and other forms, with graphite displaying relatively good electrical conductivity, while diamond is transparent and an extremely poor conductor.[61] Carbon further exists in various allotropic structures, including buckminsterfullerene,[62] and amorphous[63] and paracrystalline (mixed amorphous and crystalline)[64] variations. Iodine among the halogen nonmetals, the other unclassified nonmetals, and metalloids all show allotropic variations. Allotropic forms of the noble gases are not known.
Chemical
Nonmetals exhibit distinctive characteristics in their chemical behavior, marked by their comparatively high electronegativity values[65] and a propensity to form acidic compounds. For instance, when nonmetals, including metalloids, react with nitric acid, the resultant products often manifest as either acids themselves or oxides with prevailing acidic properties.[n 11]
Unlike metals, which typically donate electrons during chemical reactions, nonmetals tend to gain or share electrons. This behavior arises from their pursuit of achieving electron configurations akin to the noble gases, known for their stable outer electron shells. For nonmetals, this electron configuration alignment is succinctly encapsulated by the duet and octet rules, offering heuristics for understanding their electron interactions. Metals, on the other hand, follow a less rigidly predictive 18-electron rule.[68]
Nonmetals also generally exhibit higher ionization energies, electron affinities, and standard reduction potentials compared to metals. In broad terms, an element's nonmetallic character tends to increase in tandem with the elevation of these values, and with electronegativity.[69]
The chemical distinctions between metals and nonmetals primarily arise from the interplay of attractive forces between an atom's positively charged nucleus and its negatively charged outer electrons. In progressing from left to right across each period of the periodic table, the nuclear charge escalates,[70] concurrent with an associated decrease in atomic radius.[71] This phenomenon results from the intensified attraction of the outer electrons toward the nucleus.[72] In the case of metals, the nuclear charge effect is generally less pronounced compared to nonmetallic elements. Consequently, metals tend to lose electrons during bonding, leading to the formation of positively charged or polarized atoms or ions. Conversely, nonmetals, owing to their stronger nuclear charge, have a proclivity to gain those same electrons, thus giving rise to negatively charged ions or polarized atoms.[73]
The number of compounds formed by nonmetals is vast.[74] In a "top 20" table detailing the elements most frequently encountered in a register of 895,501,834 compounds recorded by the Chemical Abstracts Service as of November 2, 2021, nonmetals occupied the first 10 positions. This dominance was underscored by hydrogen, carbon, oxygen, and nitrogen collectively appearing in a majority (80%) of the compounds. Silicon, which falls under the category of metalloids, held the 11th position. The highest-rated metal, iron, accounted for a mere 0.14% of occurrences, placing it in the 12th position.[75] Examples of compounds involving nonmetals include boric acid (H
3BO
3), used in ceramic glazes;[76] selenocysteine (C
3H
7NO
2Se), the 21st amino acid of life;[77] phosphorus sesquisulfide (P4S3), used in the production of strike anywhere matches;[78] and teflon ((C
2F
4)n),[79] renowned for its application in non-stick coatings for various cookware.
Complications


The chemistry of nonmetals is further complicated by the presence of anomalies observed in the first row of each periodic table block. These anomalies are particularly notable in elements such as hydrogen, boron (which exhibits characteristics of both nonmetals and metalloids), carbon, nitrogen, oxygen, and fluorine. In progressing to later rows, these anomalies evolve into secondary periodic patterns or non-uniform trends within most of the p-block groups.[80] Additionally, in the heavier nonmetals, unusual oxidation states become apparent, further adding to the intricacies of their chemical behavior.
First row anomaly
The first row anomaly, starting with hydrogen, primarily arises from the unique electron configurations exhibited by these elements. Hydrogen, in particular, is renowned for its versatility in forming various types of chemical bonds. Most commonly, it engages in covalent bonding. However, it can also relinquish its single electron when in aqueous solution, resulting in the formation of a naked proton with tremendous polarizing capabilities.[81] This proton readily attaches itself to the lone electron pair of an oxygen atom within a water molecule, thereby serving as a foundation for the principles of acid-base chemistry.[82] Hydrogen atoms within molecules can also establish secondary, albeit weaker, bonds with atoms or groups of atoms in neighboring molecules. As observed by Cressey, these bonds contribute to numerous phenomena, including the hexagonal symmetry of snowflakes, the structural integrity of DNA's double helix, the three-dimensional configuration of proteins, and even the elevation of water's boiling point, facilitating the preparation of a decent cup of tea.[83]
Elements ranging from hydrogen and helium to boron through neon exhibit unusually compact atomic radii. This phenomenon stems from the absence of inner analogues for the 1s and 2p subshells in these elements (that is, there is no zero shell and no 1p subshell). Consequently, they experience minimal electron repulsion effects, in contrast to the 3p, 4p, and 5p subshells found in heavier elements.[84] Ionization energies and electronegativities within this subset of elements are correspondingly higher than anticipated based on periodic trends. The diminutive atomic radii of carbon, nitrogen, and oxygen further facilitate the formation of double or triple bonds in these elements' compounds.[85]
While it would normally be expected for hydrogen and helium to be situated atop the s-block elements, the pronounced first row anomaly associated with these two elements justifies alternative placements. In certain periodic table representations, hydrogen is positioned above fluorine in group 17 rather than over lithium in group 1. Similarly, helium is often situated above neon in group 18 instead of over beryllium in group 2.[86]
Secondary periodicity
A distinct alternation occurs in certain periodic trends upon descending groups 13 to 15 and to a lesser extent in groups 16 to 17.[87] Following the initial row of d-block metals, spanning scandium to zinc, the 3d electrons found in the subsequent p-block elements—namely, gallium (classified as a metal), germanium, arsenic, selenium, and bromine—exhibit a reduced capacity to effectively shield the growing positive nuclear charge. A similar phenomenon becomes evident with the introduction of the fourteen f-block metals located between barium and lutetium. This collective effect gives rise to atomic radii that are smaller than what might be anticipated for elements starting from hafnium (Hf) onward.[88][n 13]
Unusual oxidation states
The larger atomic radii observed in the heavier nonmetals within groups 15 to 18 facilitate the attainment of higher bulk coordination numbers and contribute to lower electronegativity values. This characteristic enhances their ability to accommodate higher positive charges, allowing these elements to exhibit oxidation states other than the lowest associated with their respective groups, that is 3, 2, 1, or 0. Notable instances of this phenomenon are evident in compounds like phosphorus pentachloride (PCl5), sulfur hexafluoride (SF6), iodine heptafluoride (IF7), and xenon difluoride (XeF2).[90]
Types
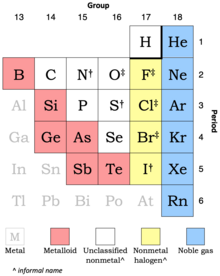
† moderately strong oxidizing agents
‡ strong oxidizing agents.[n 14]
Classification methodologies for nonmetals can encompass a range of approaches, varying from as few as two types to as many as six or seven. For instance, the periodic table employed by Encyclopædia Britannica distinguishes noble gases, halogens, and other nonmetals, while allocating elements commonly identified as metalloids into two types, "other metals" and "other nonmetals". Conversely, the periodic table endorsed by the Royal Society of Chemistry adopts a distinct color scheme for each of its eight primary groups, with nonmetals being distributed across seven of these types.[102]
When examining the periodic table from right to left, three or four distinct types of nonmetals can typically be identified; these include:
- The noble gases, known for their relatively inert chemical behavior.[103]
- A group of highly reactive halogen elements encompassing fluorine, chlorine, bromine, and iodine, commonly referred to as nonmetal halogens.[104] or halogen nonmetals[105] (the term used here) or stable halogens.[106]
- A category of unclassified nonmetals, which comprises elements such as hydrogen, carbon, nitrogen, and oxygen. This group lacks a universally recognized collective name.[n 16]
- The chemically weak metalloids,[115] which are sometimes classified as nonmetals and at other times considered distinct from nonmetals.[n 17]
Given the metalloids occupy "frontier territory",[117] where metals meet nonmetals, their treatment can vary among authors and classification schemes. Some consider metalloids as distinct from both metals and nonmetals, while others categorize them as nonmetals[118] or as a subset within the nonmetal classification.[119] In certain cases, specific metalloids, such as arsenic and antimony, are counted as metals due to their resemblances to heavy metals.[120][n 18] In this context, metalloids are here categorized as nonmetals in light of their characteristic chemical behavior,[115] and for the sake of comparison.
Apart from the metalloids, there is some degree of ambiguity and overlap among the other types of nonmetals, which is not uncommon in classification systems.[121] Elements like carbon, phosphorus, selenium, and iodine exhibit traits that border on the characteristics of metalloids and display certain metallic properties. Hydrogen also shows some metallic character. Among the noble gases, radon stands out as the most metallic, demonstrating unusual cationic behavior for a nonmetal.[122]
Noble gas

Six nonmetals fall under the category of noble gases namely helium, neon, argon, krypton, xenon, and the radioactive radon. In traditional periodic tables, these elements occupy the far-right column. They are referred to as noble gases due to their distinctive characteristic of exceptionally low chemical reactivity.[103]
Noble gases share several common properties, being colorless, odorless, and nonflammable. Their closed outer electron shells result in feeble interatomic forces of attraction, leading to remarkably low melting and boiling points.[123] Consequently, all noble gases exist in the gaseous state under standard conditions, including those with atomic masses surpassing that of many typically solid elements.[124]
From a chemical perspective, noble gases exhibit relatively high ionization energies, either nil or negative electron affinities, and relatively high to very high electronegativity. The number of compounds formed by noble gases now counts in the hundreds and continues to expand,[125] with the majority involving combinations of oxygen or fluorine with either krypton, xenon, or radon.[126]
In terms of the periodic table, a noteworthy analogy can be drawn between the noble gases and noble metals, such as platinum and gold, due to their shared reluctance to form compounds with other elements.[127] For instance, xenon, when in the +8 oxidation state, gives rise to a pale yellow explosive oxide known as XeO4. Conversely, osmium, another noble metal, yields a yellow and highly oxidizing oxide,[128] OsO4. Parallels can also be observed in the chemical formulas of oxyfluorides, such as XeO2F4 and OsO2F4, and XeO3F2 and OsO3F2.[129]
The Earth's atmosphere contains approximately 1015 tonnes of noble gases,[130] with an additional presence of up to 7% helium in natural gas.[131] Radon, a noble gas, emanates from rocks as a byproduct of the natural decay processes of uranium and thorium.[132] Furthermore, it is estimated that the Earth's core may contain around 1013 tons of xenon, predominantly in the form of stable XeFe3 and XeNi3 intermetallic compounds. This phenomenon may account for the observed depletion of more than 90% of the expected amount of xenon in the Earth's atmosphere through studies of its composition.[133]
Halogen

2, the most common fluorine mineral, between two quartzes. It is the main source of fluorine for commercial uses.[134]
While the halogen nonmetals exhibit pronounced reactivity and corrosiveness, they can also be found in various everyday compounds, such as toothpaste (containing NaF), ordinary table salt (NaCl), swimming pool disinfectants (NaBr), and food supplements (KI). The term 'halogen' derives from the Greek words meaning "salt former."[135]
Physically, fluorine and chlorine present as pale yellow and yellowish-green gases, respectively; bromine is a reddish-brown liquid, typically overlaid by a layer of vaporous fumes; and iodine, under white light, appears as a solid with a metallic sheen.[91] Electrically, the first three elements are insulators, while iodine behaves as a semiconductor along its crystallographic planes.[136]
From a chemical standpoint, halogen nonmetals are characterized by high ionization energies, electron affinities, and electronegativity values, often displaying strong oxidizing properties.[137] This is reflected in their corrosive nature.[138] All four halogens exhibit a propensity to form predominantly ionic compounds with metals,[139] in contrast to the remaining nonmetals, except for oxygen, which tend to form primarily covalent compounds with metals.[n 19] The halogen nonmetals represent the pinnacle of nonmetallic character, exemplified by their highly reactive and strongly electronegative nature.[143]
In the context of the periodic table, the highly nonmetallic halogens, which belong to Group 17, find their counterparts in the form of the highly reactive alkali metals, including sodium and potassium, situated in Group 1.[144] It is noteworthy that most of the alkali metals, seemingly emulating the halogen nonmetals, are known to form −1 anions, a behavior rarely occurring among metals.[145]
The halogen nonmetals are commonly found in minerals associated with salts. Fluorine, for example, is present in fluorite (CaF2), a mineral that enjoys widespread occurrence. Chlorine, bromine, and iodine can be found in various brines. Interestingly, a 2012 study reported the discovery of native fluorine (F
2) by weight in antozonite, constituting approximately 0.04% of its weight. These inclusions were attributed to the effects of radiation emanating from tiny amounts of uranium impurities.[146]
Metalloid
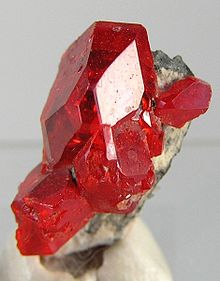
The category of metalloids encompasses six elements that are commonly recognized as such: boron, silicon, germanium, arsenic, antimony, and tellurium, all of which exhibit a metallic appearance. On the standard periodic table, these elements occupy a diagonal region within the p-block, extending from boron in the upper left to tellurium in the lower right. This region straddles the boundary between metals and nonmetals, as depicted on some periodic tables.[12]
Metalloids are characterized by their brittleness and varying electrical conductivity, ranging from poor to good. Boron, silicon, germanium, and tellurium are known as semiconductors. On the other hand, arsenic and antimony possess electronic structures akin to semimetals, although they also exhibit less stable semiconducting forms.[12]
As to their chemical behavior, metalloids generally display characteristics more in line with chemically weak nonmetals. Within the group of nonmetallic elements, they tend to exhibit the lowest ionization energies, electron affinities, and electronegativity values, and they are relatively modest as oxidizing agents. Additionally, metalloids are inclined to form alloys when combined with metals.[12]
In terms of the periodic table, just to the left of the weakly nonmetallic metalloids, there exists a less clearly defined set of weakly metallic metals. This category includes elements like tin, lead, and bismuth,[149] most often referred to as post-transition metals.[150] Dingle[151] explains the situation as follows:
... with 'no-doubt' metals on the far left of the table, and no-doubt non-metals on the far right ... the gap between the two extremes is bridged first by the poor (post-transition) metals, and then by the metalloids—which, perhaps by the same token, might collectively be renamed the 'poor non-metals'.
Metalloids are typically encountered in compounds alongside oxygen, sulfur, or, in the case of tellurium, gold or silver.[152] For example, boron is commonly found in boron-oxygen borate minerals, often present in volcanic spring waters. Silicon is a constituent of silicon-oxygen minerals, such as silica (commonly found in sand). Germanium, arsenic, and antimony are primarily encountered as components of sulfide ores. Tellurium is typically found in telluride minerals that often accompany gold or silver deposits. In some instances, native forms of arsenic, antimony, and tellurium have been reported.[153]
Unclassified

After categorizing nonmetallic elements into noble gases, halogens, and metalloids, the remaining seven nonmetals encompass hydrogen, carbon, nitrogen, oxygen, phosphorus, sulfur, and selenium.
Physically, the unclassified nonmetals appear to lack rhyme or reason. In their most stable forms, three of them exist as colorless gases (H, N, O), three exhibit a metallic appearance (C, P, Se), and one presents as yellow (S). Electrically, graphitic carbon behaves as a semimetal within its planes[155] and a semiconductor perpendicular to those planes.[156] Phosphorus and selenium are both semiconductors,[157] while hydrogen, nitrogen, oxygen, and sulfur are insulators.[n 20]
Continuing the theme, these elements are recognized for their diversity, making it challenging to assign a collective classification.[159] They are often referred to as "other nonmetals"[160] or simply "nonmetals," residing between the metalloids and the halogens.[161]
Consequently, their chemistry is typically taught separately, based on their respective positions within four different groups on the periodic table. For instance: hydrogen in group 1; the group 14 nonmetals, which may include silicon and germanium; the group 15 nonmetals, including nitrogen, phosphorus, and potentially arsenic and antimony; and the group 16 nonmetals, comprising oxygen, sulfur, selenium, and possibly tellurium. There are alternative subdivisions based on individual author preferences.[n 21]
Hydrogen exhibits a dual nature, displaying characteristics both akin to a metal and those typical of a nonmetal.[163] Resembling a metal, it can readily lose its single electron,[164] substituting for alkali metals in standard alkali metal structures[165] and forming alloy-like hydrides with certain transition metals, characterized by metallic bonding.[166] Conversely, it exists as an insulating diatomic gas, a hallmark of nonmetals, and during chemical reactions, it tends to acquire the electron configuration of helium.[167] This transformation is achieved through the formation of ionic or covalent bonds,[168] or by attaching itself to a lone pair of electrons.[169]
Despite their diversity, the unclassified nonmetals share several common properties. Unlike the highly reactive halogens,[170] most of them occur naturally in the environment.[171] They play significant roles in biological[172] and geochemical processes.[159] While their physical and chemical characteristics lean toward the moderately non-metallic,[159] they all exhibit corrosive tendencies. Hydrogen can corrode metals, carbon can corrosion can occur in fuel cells,[173] dissolved nitrogen or sulfur contributes to acid rain, oxygen causes iron to rust, and white phosphorus, the least stable form, ignites in air, leaving behind phosphoric acid residue. Untreated selenium in soils can produce corrosive hydrogen selenide gas. When combined with metals, the unclassified nonmetals can create exceptionally hard (interstitial or refractory) compounds[174] thanks to their relatively small atomic radii and sufficiently low ionization energies.[159] Additionally, they tend to form bonds with themselves, particularly in solid compounds.[175] Diagonal periodic table relationships on the periodic table among these nonmetals mirror similar relationships found among the metalloids.[176]
In the context of the periodic table, an analogy can be drawn between the unclassified nonmetals and transition metals and a geographic comparison can be made. The unclassified nonmetals are situated between the strongly nonmetallic halogens on the right and the weakly nonmetallic metalloids on the left. In contrast, the transition metals occupy a region between the more reactive metals found on the left side of the periodic table and the less reactive metals on the right, essentially forming a transitional bridge between the two.[177]
Unclassified nonmetals are commonly found in their elemental forms (oxygen, sulfur) or in association with either of these two elements. Examples of their occurrence include:[152]
- Hydrogen is abundant in the Earth's oceans as a component of water and can be found in natural gas, including methane and hydrogen sulfide.[178]
- Carbon is present in various forms, such as carbonates found in limestone, dolomite, and marble.[179] It also occurs as graphite, mainly within metamorphic silicate rocks,[180] resulting from the compression and heating of sedimentary carbon compounds.[181]
- Oxygen is a major constituent of the Earth's atmosphere and is present in oceans as part of water. It can also be found in the Earth's crust in the form of oxide minerals.[182]
- Phosphorus is widely distributed in nature, primarily as phosphorus-oxygen phosphates.[183][184]
- Elemental sulfur can be discovered near hot springs and volcanic regions across different regions worldwide. Sulfur minerals are also prevalent, commonly existing as sulfides or oxygen-sulfur sulfates.[183][185]
- Selenium is often encountered in metal sulfide ores, where it can partially replace sulfur. In certain instances, elemental selenium can be found.[186]
Prevalence and access
Abundance
Domain | Main components | Next most abundant |
---|---|---|
Crust | O 61%, Si 20% | H 2.9% |
Atmosphere | N 78%, O 21% | Ar 0.5% |
Hydrosphere | O 66.2%, H 33.2% | Cl 0.3% |
Biomass | O 63%, C 20%, H 10% | N 3.0% |
Hydrogen and helium are recognized as the predominant elements in the universe, collectively constituting an estimated 99% of all ordinary matter. These two elements also comprise over 99.9% of the total number of atoms present.[188] Oxygen follows as the next most abundant element, representing approximately 0.1% of the universe's elemental composition.[189] Less than five per cent of the universe is believed to be made of ordinary matter, represented by stars, planets, and living beings. The balance is hypothesized to be made of dark energy and dark matter, both of which are currently poorly understood.[190]
Five nonmetals—hydrogen, carbon, nitrogen, oxygen, and silicon—play pivotal roles in shaping the Earth's composition. These elements collectively constitute the foundational building blocks of the Earth's crust, atmosphere, hydrosphere, and biomass. Their relative quantities, as outlined in the accompanying table, emphasize their fundamental significance in the terrestrial environment.
Extraction

Nonmetals, and metalloids, are extracted in their raw forms from:[171]
- brine—chlorine, bromine, iodine;
- liquid air—nitrogen, oxygen, neon, argon, krypton, xenon;
- minerals—boron (borate minerals); carbon (coal; diamond; graphite); fluorine (fluorite); silicon (silica); phosphorus (phosphates); antimony (stibnite, tetrahedrite); iodine (in sodium iodate and sodium iodide);
- natural gas—hydrogen, helium, sulfur; and
- ores, as processing byproducts—germanium (zinc ores); arsenic (copper and lead ores); selenium, tellurium (copper ores); and radon (uranium-bearing ores).
Cost
Day to day costs will vary depending on purity, quantity,[n 22] market conditions, and supplier surcharges.[195]
Based on the available literature as of April 2023, the cited costs of most nonmetals are less than the $US0.74 per gram cost of silver.[196] The exceptions are boron, phosphorus, germanium, xenon, and radon (notionally):
- Boron costs around $25 per gram for 99.7% pure polycrystalline chunks with a particle size of about 1 cm.[197] Earlier, in 1997, boron was quoted at $280 per gram for polycrystalline 4-to-6-mm-diameter rods of 99.999% purity,[198] about 10 times the then $28.35 per gram cost of gold.[199]
- In 2020, phosphorus in its most-stable black form could "cost up to $1,000 per gram",[200] more than 15 times the cost of gold, whereas ordinary red phosphorus, in 2017, was priced at about $3.40 per kilogram.[201] Researchers hoped to be able to reduce the cost of black phosphorus to as low as $1 per gram.[200]
- Germanium and xenon cost about $1.30 and $7.60 per gram.[202]
- Up to 2013, radon was available from the National Institute of Standards and Technology for $1,636 per 0.2 ml unit of issue, equivalent to about $86,000,000 per gram, with no indication of a discount for bulk quantities.[203]
Uses

The distinctive properties exhibited by nonmetallic elements offer a wide array of applications, often unattainable through the use of metallic elements alone. An impressive facet of nonmetals is their pervasive presence in living organisms, with hydrogen, oxygen, carbon, and nitrogen serving as the elemental building blocks of life itself. Furthermore, these elements have important roles across various industries, ranging from cutting-edge electronics and advanced energy storage systems to the agricultural sector and large-scale chemical production. A few noteworthy examples include:
- Carbon's versatile utility—Carbon fibers, renowned for their exceptional strength-to-weight ratio, find wide-ranging applications in aerospace, automotive manufacturing, and sports equipment development. The remarkable properties of graphene, composed of a single layer of carbon atoms, encompass outstanding electrical and thermal conductivity, rendering it invaluable in electronic devices, energy storage solutions, and the creation of composite materials.[205]
- Nitrogen boosts agriculture—Nitrogen, a fundamental component in fertilizer production, substantially bolsters crop growth and enhances agricultural yields. Its low-temperature attributes render it indispensable for cryogenic applications in preserving biological samples and facilitating food freezing processes.[206]
- Oxygen's vital role—Oxygen, essential for human respiration, plays an indispensable role in life support systems and medical settings where it aids individuals with respiratory difficulties. Moreover, it serves as a critical component in various industrial processes, including metal smelting and waste incineration, due to its combustion-supporting capabilities.[207]
- Silicon empowers electronics—Silicon, the cornerstone of the electronics industry, underpins the manufacture of microchips, solar cells, and an assortment of electronic components. Silicon dioxide (silica) serves as a key material in the production of glass, ceramics, and optical fibers, offering versatile applications in the creation of windows, lenses, and advanced communication networks.[208]
- Sulfur's industrial significance—Sulfur finds extensive utility in the manufacturing of sulfuric acid, a widely used industrial chemical. It also plays a crucial role in the synthesis of various organic compounds. In materials engineering, sulfur compounds are instrumental in the vulcanization process, which enhances the strength and flexibility of rubber-based products.[209]
Shared uses of different subsets of the nonmetals encompass their presence in, or specific uses in the fields of (inert) air replacements; dyestuffs; flame retardants or extinguishers; household accoutrements; lasers and lighting; mineral acids; plug-in hybrid vehicles; and welding gases.[171][210] To the extent that metalloids show metallic character, they have speciality uses extending to (for example) oxide glasses and alloying components.[211]
History, background, and taxonomy
Discovery

The majority of nonmetallic elements were discovered in the 18th and 19th centuries. A handful of nonmetals had already been recognized in antiquity and later periods. Carbon, sulfur, and antimony were among the early nonmetals known to humanity. Arsenic's discovery traces back to the Middle Ages, accredited to the work of Albertus Magnus. A remarkable moment in the history of nonmetal discovery occurred in 1669 when Hennig Brand successfully isolated phosphorus from urine. Curiously, helium, identified in 1868, holds a unique distinction as the only element not initially discovered on Earth itself.[n 23] Radon emerged as the most recently uncovered nonmetal, its detection marking the close of the 19th century.[171]
The isolation of nonmetallic elements relied on a spectrum of chemical and physical techniques. These methods included spectroscopy, fractional distillation, radiation detection, electrolysis, ore acidification, displacement reactions, combustion and controlled heating processes. Some nonmetals were available in nature as free elements, while others necessitated elaborate processes for extraction:
- Noble gases, those elusive elements characterized by their low reactivity, made their debut through interesting methods. Helium was initially detected via its unique yellow line in the coronal spectrum of the sun. Later, it was observed escaping as bubbles from uranite UO2 when dissolved in acid. Neon, argon, krypton, and xenon, on the other hand, were procured through the fractional distillation of air. The discovery of radon followed three years after Henri Becquerel's pioneering work on radiation in 1896.[213]
- For the halogen nonmetals, isolation from their halides involved techniques such as electrolysis, acid addition, or displacement. These endeavors were not without risks, as some chemists tragically lost their lives in their pursuit to isolate fluorine.[214]
- The unclassified nonmetals encompassed a variegated history. Carbon occurred naturally in various forms like charcoal, soot, graphite, and diamond. Nitrogen was detected by examining air from which oxygen had been carefully removed. Oxygen itself was obtained through the heating of mercurous oxide. Phosphorus emerged from the heating of ammonium sodium hydrogen phosphate (Na(NH4)HPO4), a compound found in urine.[215] Sulfur occurred naturally as a free element, simplifying its isolation process. Selenium,[n 24] was first identified as a residue in sulfuric acid.[217]
- Metalloids were typically isolated through the heating of their oxides ((boron, silicon, arsenic, tellurium) or a sulfide (germanium).[171] Antimony, in an unusual twist, was found in its native form and could also be obtained through heating its sulfide compound.[218]
Origin and use of the term

While a distinction had been made between metals and other mineral substances since ancient times it was only towards the end of the 18th century that a rudimentary classification of chemical elements as either metallic or nonmetallic substances appeared. A further nine decades would pass before the term "nonmetal" was widely adopted.
Around the year 340 BCE, in Book III of the treatise Meteorology, the ancient Greek philosopher Aristotle categorized substances found within the Earth into two distinct groups: metals and "fossiles".[n 25] The latter category encompassed various minerals, including realgar, ochre, ruddle, sulfur, cinnabar, and other substances that he referred to as "stones which cannot be melted".[220]
Up to the Middle Ages the situation had remained unchanged aside from the terminology. In the fourteenth century, an English alchemist named Richardus Anglicus elaborated upon the classification of minerals in the text Correctorium Alchemiae. Within this work, he posited the existence of two primary types of minerals. The first, which he termed "major minerals", included well-known metals such as gold, silver, copper, tin, lead, and iron. In contrast, the second category, denoted as "minor minerals", encompassed substances such as salts, atramenta (iron sulfate), alums, vitriol, arsenic, orpiment, sulfur and the like, which are not metallic bodies.[221]
The term "nonmetallic" finds its historical roots tracing back to at least the 16th century. In a 1566 medical treatise, the French physician Loys de L'Aunay wrote of the distinct properties exhibited by substances derived from plant sources. He made a noteworthy comparison between the characteristics of such materials originating from what he termed metallic soils and non-metallic soils.[222]
Later, and more relevantly, the French chemist Nicolas Lémery, in his Universal Treatise on Simple Drugs, Arranged Alphabetically (1699) mentions metallic minerals and nonmetallic minerals. According to him, the substance "cadmia" potentially belonged to either the first category, as with cobaltum (cobaltite), or the second, as exemplified by what used to be referred to as calamine, a mixed ore of zinc carbonate and silicate.[223]
The showcase moment in the systematic classification of chemical elements, including as metallic and nonmetallic substances, occurred in 1789 with the appearance of Antoine Lavoisier's revolutionary[224] work Traité élémentaire de chimie. Lavoisier, a French chemist, published the first modern (for its time) list of chemical elements, categorizing them into distinct groups as gases, metallic substances, nonmetallic substances, and earths (heat-resistant oxides).[225] His work gained widespread recognition and was republished in twenty-three editions spanning six languages within its first seventeen years, significantly advancing the understanding of chemistry across Europe and America.[226]
The eventual and uniform adoption of the term "nonmetal" underwent a tortuous developmental period of nigh on nine decades. In 1811, Swedish chemist Berzelius introduced the term "metalloids"[227][228] to describe nonmetallic elements, noting their ability to form negatively charged ions with oxygen in aqueous solution.[229][230] Although Berzelius' terminology gained widespread acceptance,[227] it later faced criticism. Some commentators viewed it as counterintuitive,[230] misapplied,[231] or even invalid.[232][233] By 1855, some authors preferred using "nonmetal" over "metalloid" to describe nonmetallic elements. In 1864, it was reported that the term "metalloids" for nonmetals was still endorsed by leading authorities. However, there were reservations about its appropriateness. The idea of instead designating elements like arsenic as metalloids had been contemplated.[234] In an 1875 observation, Kemshead noted that elements were categorized into two groups: non-metals (or metalloids) and metals. He commented that the term "non-metal", despite its compounded nature, was more precise and had since become the universally accepted nomenclature.[235]
Suggested distinguishing criteria
Physical | Chemical Electron related |
In 1809, the British chemist and inventor Humphry Davy made a groundbreaking discovery that revolutionized the understanding of metals and nonmetals.[258] His isolation of sodium and potassium marked a significant departure from the traditional method of distinguishing metals based on their ponderousness or relatively high densities.[259] Sodium and potassium, in contrast, exhibited a remarkable behavior—they floated on water. However, their classification as metals was firmly established by their chemical properties.[260]
As early as 1811, efforts were made to further refine the distinction between metals and nonmetals using various properties. These properties encompassed physical, chemical, and electron-related characteristics. The table provided here enumerates 22 such properties, organized by type and the date of their discovery.
One of the most widely recognized (but unreliable) properties used in this context is the change in electrical conductivity with temperature. Typically, metals exhibit an increase in electrical conductivity as temperature decreases, while nonmetals display the opposite trend.[248] However, certain exceptions challenge this generalization. Plutonium, carbon, arsenic, and antimony, for instance, defy the norm. Plutonium's electrical conductivity increases when heated within a specific temperature range of −175 to +125 °C.[261] Similarly, despite its common classification as a nonmetal, carbon also experiences an increase in electrical conductivity when subjected to heating.[262] Arsenic and antimony, occasionally categorized as nonmetals, exhibit behavior akin to carbon, further underscoring the complexity of the distinction between metals and nonmetals.[263]
Kneen and colleagues proposed that the classification of nonmetals becomes feasible once a singular criterion for metallicity is established. They acknowledged the existence of differing plausible classifications, emphasizing that while these classifications might differ somewhat, they would generally align in their delineation of nonmetals.[9]
However, Emsley pointed out the complexity of this task, asserting that no single property alone can unequivocally assign elements to either the metal or nonmetal category.[264] Furthermore, Jones emphasized that classification systems typically rely on more than two attributes to define distinct types.[265]
EN | ||
Density | < 1.9 | ≥ 1.9 |
< 7 gm/cm3 | Groups 1 and 2 Sc, Y, La Ce, Pr, Eu, Yb Ti, Zr, V; Al, Ga |
Noble gases F, Cl, Br, I H, C, N, P, O, S, Se B, Si, Ge, As, Sb, Te^ |
> 7 gm/cm3 | Nd, Pm, Sm, Gd, Tb, Dy Ho, Er, Tm, Lu; Ac–Es; Hf, Nb, Ta; Cr, Mn, Fe, Co, Zn, Cd, In, Tl, Pb |
Ni, Mo, W, Tc, Re, Platinum group metals, Coinage metals, Hg; Sn, Bi, Po, At |
^ italicized elements are commonly recognized by some authors as metalloids |
An approach to distinguishing between metallic and nonmetallic properties was suggested by Johnson, emphasizing the significance of physical properties, while acknowledging the potential need for other properties in certain ambiguous cases. His observations highlighted several key distinctions:[270]
- Physical state—Elements that exist as gases or are nonconductors are typically categorized as nonmetals.
- Solid nonmetals—Solid nonmetals exhibit characteristics such as hardness and brittleness or softness and crumbliness, setting them apart from metals that are generally malleable and ductile.
- Chemical behavior—Nonmetal oxides tend to be acidic, providing another useful criterion for identifying nonmetals.
Hein and Arena noted that nonmetals typically possess relatively low densities and high electronegativity,[65] as corroborated by the data in the accompanying table. The nonmetallic elements predominantly occupy the top left quadrant of this table, where both densities and electronegativity values are relatively high. The remaining three quadrants are primarily populated by metals.
While some authors opt for a further subdivision of elements into metals, metalloids, and nonmetals, Oderberg contests this approach and argues that, by the principles of categorization, anything not classified as a metal should be considered a nonmetal.[271]
Development of types

A basic taxonomy of nonmetals was set out in 1844, by Alphonse Dupasquier a French doctor, pharmacist and chemist.[272] To facilitate the study of nonmetals, he wrote:[273]
- They will be divided into four groups or sections, as in the following:
- Organogens O, N, H, C
- Sulphuroids S, Se, P
- Chloroides F, Cl, Br, I
- Boroids B, Si.
An echo of Dupasquier's fourfold classification is seen in the modern types of nonmetal. The organogens and sulphuroids represent the set of unclassified nonmetals. The chloroide nonmetals came to be independently referred to as halogens.[274] The boroid nonmetals were expanded into the metalloids, starting from as early as 1864.[234] The noble gases, as a discrete grouping, were counted among the nonmetals from as early as 1900.[275]
Comparison
Some properties of various elemental types, including metals, metalloids, unclassified nonmetals, halogen nonmetals, and noble gases, are summarized in the following table.[n 28] These properties are based on the elements' most stable forms at standard ambient conditions and are presented in a loose order of ease of determination. The chemical properties are listed in a progressive manner, moving from general characteristics to more specific details. The dashed line around the metalloids signifies that, depending on the author or classification scheme, these elements may or may not be recognized as a distinct class or type of elements. Metals are included in this overview as a reference point.
The properties exhibited by these elemental groups generally exhibit a left-to-right progression in terms of metallic-to-nonmetallic character or average values. This arrangement effectively divides the periodic table into metals and nonmetals, revealing varying degrees of gradation within the nonmetallic elements.[276]
The periodic table provides a valuable framework for understanding these distinctions, offering insights into the multifarious nature of elements and their placement within the metallic to nonmetallic spectrum.
Physical property | Metals alkali, alkaline earth, lanthanide, actinide, transition, post-transition |
Metalloids boron, silicon, germanium, arsenic, antimony, tellurium |
Unclassified nonmetals hydrogen, carbon, nitrogen, phosphorus, oxygen, sulfur, selenium |
Halogen nonmetals fluorine, chlorine, bromine, iodine |
Noble gases helium, neon, argon, krypton, xenon, radon |
---|---|---|---|---|---|
Form and heft[277] |
|
|
|
|
|
Appearance | lustrous[23] | lustrous[280] | colorless[285] | ||
Elasticity | mostly malleable and ductile[23] (Hg is liquid) | brittle[280] | C, black P, S, Se brittle; all four have less stable non-brittle forms[286][n 29] | iodine is brittle[288] | not applicable |
Electrical conductivity | good[n 30] |
|
|
|
poor[n 34] |
Electronic structure[292] | metallic (Bi is a semimetal) | semimetal (As, Sb) or semiconductor |
|
semiconductor (I) or insulator | insulator |
Chemical property | Metals alkali, alkaline earth, lanthanide, actinide, transition, post-transition |
Metalloids boron, silicon, germanium, arsenic, antimony, tellurium |
Unclassified nonmetals hydrogen, carbon, nitrogen, phosphorus, oxygen, sulfur, selenium |
Halogen nonmetals fluorine, chlorine, bromine, iodine |
Noble gases helium, neon, argon, krypton, xenon, radon |
General chemical behavior |
|
weakly nonmetallic[n 35] | moderately nonmetallic[295] | strongly nonmetallic[296] | |
Oxides |
|
||||
Compounds with metals | alloys[23] or intermetallic compounds[314] | tend to form alloys or intermetallic compounds[315] | mainly ionic[139] | simple compounds in ambient conditions not known[n 38] | |
Ionization energy (kJ mol−1)‡ [319] |
|
|
|
|
|
Electronegativity (Pauling)[n 39]‡ [321] |
|
|
|
|
|
† Hydrogen can also form alloy-like hydrides[166] ‡ The labels low, moderate, high, and very high are arbitrarily based on the value spans listed in the table |
See also
- CHON (carbon, hydrogen, oxygen, nitrogen)
- List of nonmetal monographs
- Metallization pressure
- Nonmetal (astrophysics)
- Nonmetal (physics)
- Period 1 elements (hydrogen, helium)
- Properties of nonmetals (and metalloids) by group
Notes
- ^ For example, of those authors who choose to recognise metalloids as a distinct type of chemical element, about a quarter of them include selenium.[1]
- ^ Hydrogen has historically been placed over one or more of lithium, boron,[2] carbon, or fluorine; or over no group at all; or over all main groups simultaneously, and therefore may or may not be adjacent to other nonmetals.[3]
- ^ Metallic or nonmetallic character is usually taken to be indicated by one property rather than two or more properties.
- ^ Steudel's monograph is an updated translation of the fifth German edition of 2013, incorporating the literature up to Spring 2019.
- ^ When synthesized in 1940 the discoverers of astatine considered it to be a metal.[18] Subsequently it was reported to show both metallic and nonmetallic properties.[19] In 2013, on the basis of relativistic studies, astatine was predicted to be a metal.[4] For a summary of the properties of astatine see the Metalloid article.
- ^ Solid iodine has a silvery metallic appearance under white light at room temperature.[21] It volatizes at ordinary and higher temperatures, passing from solid to gas; its vapours are violet-colored.[22]
- ^ The solid nonmetals have electrical conductivity values ranging from 10−18 S•cm−1 for sulfur[25] to 3 × 104 in graphite[26] or 3.9 × 104 for arsenic;[27] cf. 0.69 × 104 for manganese to 63 × 104 for silver, metals both.[25]
- ^ The absorbed light may be converted to heat or re-emitted in all directions so that the emission spectrum is thousands of times weaker than the incident light radiation.[43]
- ^ Thermal conductivity values for metals range from 6.3 W m−1 K−1 for neptunium to 429 for silver; cf. antimony 24.3, arsenic 50, and carbon 2000.[25] Electrical conductivity values of metals range from 0.69 S•cm−1 × 104 for manganese to 63 × 104 for silver; cf. carbon 3 × 104,[26] arsenic 3.9 × 104 and antimony 2.3 × 104.[25]
- ^ These elements being semiconductors.[48]
- ^ Acids are formed by boron, phosphorus, selenium, arsenic, iodine;[66] oxides by carbon, silicon, germanium, sulfur, antimony, and tellurium.[67]
- ^ These elements are hydrogen and helium in the s-block; boron to neon in the p-block; scandium to zinc in the d-block; and lanthanum to ytterbium in the f-block.
- ^ The net result is an even-odd difference between periods (except in the s-block) that is sometimes known as secondary periodicity: elements in even periods have smaller atomic radii and prefer to lose fewer electrons, while elements in odd periods (except the first) differ in the opposite direction. Many properties in the p-block then show a zigzag rather than a smooth trend along the group. For example, phosphorus and antimony in odd periods of group 15 readily reach the +5 oxidation state, whereas nitrogen, arsenic, and bismuth in even periods prefer to stay at +3.[89]
- ^ The seven nonmetals marked with single or double daggers each have a lackluster appearance and discrete molecular structures, but for I which has a metallic appearance under white light.[91] The remaining reactive nonmetallic elements have giant covalent structures, but for H which is a diatomic gas.[92]
The single dagger nonmetals N, S and iodine are somewhat hobbled as "strong" nonmetals.
While N has a high electronegativity, it is a reluctant anion former,[93] and a pedestrian oxidizing agent unless combined with a more active nonmetal like O or F.[94]
S reacts in the cold with alkalic and post-transition metals, and Cu, Ag and Hg,[95] but otherwise has low values of ionization energy, electron affinity, and electronegativity compared to the averages of the others; it is regarded as being not a particularly good oxidizing agent.[96]
Iodine is sufficiently corrosive to cause lesions resembling thermal burns, if handled without suitable protection,[97] and tincture of iodine will smoothly dissolve Au.[98] That said, while "F, Cl and Br will all oxidize Fe2+ (aq) to Fe3+(aq) ... iodine ... is such a [relatively] weak oxidizing agent that it cannot remove electrons from Fe(II) ions to form Fe(III) ions."[99] Thus, for the reaction X2 + 2e− → 2X−(aq) the reduction potentials are F +2.87 V; Cl +1.36; Br +1.09; I +0.54. Here Fe3+ + e− → Fe3+ +0.77.[100] Thus F2, Cl2 and Br2 will oxidize Fe2+ to Fe3+ but Fe3+ will oxidize I− to I2. Iodine has previously been referred to as a moderately strong oxidizing agent.[101] - ^ The quote marks are not found in the source; they are used here to make it clear that the source employs the word non-metals as a formal term for the subset of chemical elements in question, rather than applying to nonmetals generally.
- ^ Varying configurations of these nonmetals have been referred to as, for example, basic nonmetals,[107] bioelements,[108] central nonmetals,[109] CHNOPS,[110] essential elements,[111] "non-metals",[112][n 15] orphan nonmetals,[113] or redox nonmetals.[114]
- ^ Tshitoyan et al. (2019) conducted a machine-based analysis of the proximity of names of the elements based on 3.3 million abstracts published between 1922 and 2018 in more than 1,000 journals. The resulting map shows that "chemically similar elements are seen to cluster together and the overall distribution exhibits a topology reminiscent of the periodic table itself".[116]
- ^ Jones takes a philosophical or pragmatic view to these questions. He writes: "Though classification is an essential feature of all branches of science, there are always hard cases at the boundaries. The boundary of a class is rarely sharp ... Scientists should not lose sleep over the hard cases. As long as a classification system is beneficial to economy of description, to structuring knowledge and to our understanding, and hard cases constitute a small minority, then keep it. If the system becomes less than useful, then scrap it and replace it with a system based on different shared characteristics".[121]
- ^ Metal oxides are usually ionic.[140] On the other hand, oxides of metals with high oxidation states are usually either polymeric or covalent.[141] A polymeric oxide has a linked structure composed of multiple repeating units.[142]
- ^ Sulfur, an insulator, and selenium, a semiconductor are each photoconductors—their electrical conductivities increase by up to six orders of magnitude when exposed to light.[158]
- ^ For example, Wulfsberg divides the nonmetals, including B, Si, Ge, As, Sb, Te, Xe, into very electronegative nonmetals (Pauling electronegativity over 2.8) and electronegative nonmetals (1.9 to 2.8). This results in N and O being very electronegative nonmetals, along with the halogens; and H, C, P, S and Se being electronegative nonmetals. Se is further recognized as a semiconducting metalloid.[162]
- ^ For example, as at April 2023, the commercial price of silicon was $4 per pound or $0.0088 per gram.[193] On the other hand, the price quoted for a 335 gram sample of silicon for hobbyists and science enthusiasts was about $57, or 0.170 per gram, or about 20 times the commercial price.[194]
- ^ How helium acquired the -ium suffix is explained in the following passage by its discoverer, William Lockyer: "I took upon myself the responsibility of coining the word helium ... I did not know whether the substance ... was a metal like calcium or a gas like hydrogen, but I did know that it behaved like hydrogen [being found in the sun] and that hydrogen, as Dumas had stated, behaved as a metal".[212]
- ^ Berzelius, who discovered selenium, thought it had the properties of a metal, combined with the properties of sulfur.[216]
- ^ Not to be confused with the modern usage of fossil to refer to the preserved remains, impression, or trace of any once-living thing
- ^ The Goldhammer-Herzfeld ratio is roughly equal to the cube of the atomic radius divided by the molar volume.[239] More specifically, it is the ratio of the force holding an individual atom's outer electrons in place with the forces on the same electrons from interactions between the atoms in the solid or liquid element. When the interatomic forces are greater than, or equal to, the atomic force, outer electron itinerancy is indicated and metallic behaviour is predicted. Otherwise nonmetallic behaviour is anticipated.[240]
- ^ (a) Up to element 99 (Es), with the values taken from Aylward and Findlay.[266]
(b) Weighable amounts of the extremely radioactive elements At (element 85), Fr (87), and elements with an atomic number higher than Es (99), have not been prepared.[267]
(c) The density values used for At and Fr are theoretical estimates.[268]
(d) Bjerrum classified "heavy metals" as those metals with densities above 7 g/cm3.[269]
(e) Vernon specified a minimum electronegativity of 1.9 for the metalloids, on the revised Pauling scale.[12] - ^ See also Properties of metals, metalloids and nonmetals, which treats metalloids as a class of their own.
- ^ Carbon as exfoliated (expanded) graphite,[49][287] and as carbon nanotube wire;[51] phosphorus as white phosphorus (soft as wax, pliable and can be cut with a knife, at room temperature);[52] sulfur as plastic sulfur;[53] and selenium as selenium wires.[54]
- ^ Metals have electrical conductivity values of from 6.9×103 S•cm−1 for manganese to 6.3×105 for silver.[289]
- ^ Metalloids have electrical conductivity values of from 1.5×10−6 S•cm−1 for boron to 3.9×104 for arsenic.[290]
- ^ Unclassified nonmetals have electrical conductivity values of from ca. 1×10−18 S•cm−1 for the elemental gases to 3×104 in graphite.[291]
- ^ The halogen nonmetals have electrical conductivity values of from ca. 1×10−18 S•cm−1 for F and Cl to 1.7×10−8 S•cm−1 for iodine.[291][136]
- ^ The elemental gases have electrical conductivity values of ca. 1×10−18 S•cm−1.[291]
- ^ They always give "compounds less acidic in character than the corresponding compounds of the [typical] nonmetals".[280]
- ^ Arsenic trioxide reacts with sulfur trioxide, forming arsenic "sulfate" As2(SO4)3.[303]
- ^ CO and N2O are "formally the anhydrides of formic and hyponitrous acid, respectively: CO + H2O → H2CO2 (HCOOH, formic acid); N2O + H2O → H2N2O2 (hyponitrous acid)".[307]
- ^ Disodium helide (Na2He) is a compound of helium and sodium that is stable at high pressures above 113 GPa. Argon forms an alloy with nickel, at 140 GPa and close to 1,500 K however at this pressure argon is no longer a noble gas.[318]
- ^ Values for the noble gases are from Rahm, Zeng and Hoffmann.[320]
References
Citations
- ^ Vernon 2013
- ^ Luchinskii & Trifonov 1981, pp. 200–220
- ^ Rayner-Canham 2020, p. 212
- ^ a b c Restrepo et al. 2006, p. 411; Thornton & Burdette 2010, p. 86; Hermann, Hoffmann & Ashcroft 2013, pp. 11604‒1‒11604‒5
- ^ Parkes & Mellor 1943, p. 740
- ^ Pascoe 1982, p. 3
- ^ Glinka 1958, p. 77; Oxtoby, Gillis & Butler 2015, p. I.23
- ^ Godovikov & Nenasheva 2020, p. 4; Sanderson 1957, p. 229; Morely & Muir 1892, p. 241
- ^ a b Kneen, Rogers & Simpson 1972, pp. 218–219
- ^ Steudel 2020, p. 43
- ^ a b c Larrañaga, Lewis & Lewis 2016, p. 988
- ^ a b c d e f Vernon 2013
- ^ Hill & Holman 2017, p. 162
- ^ Vernon 2020, p. 220; Rochow 1966, p. 4
- ^ IUPAC Periodic Table of the Elements
- ^ Johnson 2007, p. 13
- ^ Bodner & Pardue 1993, p. 354; Cherim 1971, p. 98
- ^ Vasáros & Berei 1985, p. 109
- ^ Nefedov et al. 1968, p. 87
- ^ Mewes et al. 2019; Smits et al. 2020; Florez et al. 2022
- ^ Koenig 1962, p. 108
- ^ Tidy 1887, pp. 107–108
- ^ a b c d Kneen, Rogers & Simpson 1972, pp. 261–264
- ^ Phillips 1973, p. 7
- ^ a b c d e Aylward & Findlay 2008, pp. 6–12
- ^ a b Jenkins & Kawamura 1976, p. 88
- ^ Carapella 1968, p. 30
- ^ Zumdahl & DeCoste 2010, pp. 455, 456, 469, A40; Earl & Wilford 2021, p. 3-24
- ^ Still 2016, p. 120
- ^ Wiberg 2001, pp. 780
- ^ Wiberg 2001, pp. 824, 785
- ^ Earl & Wilford 2021, p. 3-24
- ^ Siekierski & Burgess 2002, p. 86
- ^ Charlier, Gonze & Michenaud 1994
- ^ Taniguchi et al. 1984, p. 867: "... black phosphorus ... [is] characterized by the wide valence bands with rather delocalized nature."; Morita 1986, p. 230; Carmalt & Norman 1998, p. 7: "Phosphorus ... should therefore be expected to have some metalloid properties."; Du et al. 2010. Interlayer interactions in black phosphorus, which are attributed to van der Waals-Keesom forces, are thought to contribute to the smaller band gap of the bulk material (calculated 0.19 eV; observed 0.3 eV) as opposed to the larger band gap of a single layer (calculated ~0.75 eV).
- ^ Wiberg 2001, pp. 742
- ^ Evans 1966, pp. 124–25
- ^ Wiberg 2001, pp. 758
- ^ Stuke 1974, p. 178; Donohue 1982, pp. 386–87; Cotton et al. 1999, p. 501
- ^ Steudel 2000, p. 601: "... Considerable orbital overlap can be expected. Apparently, intermolecular multicenter bonds exist in crystalline iodine that extend throughout the layer and lead to the delocalization of electrons akin to that in metals. This explains certain physical properties of iodine: the dark color, the luster and a weak electric conductivity, which is 3400 times stronger within the layers then perpendicular to them. Crystalline iodine is thus a two-dimensional semiconductor."; Segal 1989, p. 481: "Iodine exhibits some metallic properties ..."
- ^ Wiberg 2001, p. 416; Wiberg is here referring to iodine.
- ^ Elliot 1929, p. 629
- ^ Fox 2010, p. 31
- ^ Wibaut 1951, p. 33: "Many substances ...are colourless and therefore show no selective absorption in the visible part of the spectrum."
- ^ Kneen, Rogers & Simpson 1972, pp. 85–86, 237
- ^ Salinas 2019, p. 379
- ^ Yang 2004, p. 9
- ^ Wiberg 2001, pp. 416, 574, 681, 824, 895, 930; Siekierski & Burgess 2002, p. 129
- ^ a b Chung 1987
- ^ Godfrin & Lauter 1995
- ^ a b Janas, Cabrero-Vilatela & Bulmer 2013
- ^ a b Faraday 1853, p. 42; Holderness & Berry 1979, p. 255
- ^ a b Partington 1944, p. 405
- ^ a b Regnault 1853, p. 208
- ^ Edwards 2000, pp. 100, 102–103; Herzfeld 1927, pp. 701–705
- ^ Kneen, Rogers & Simpson 1972, pp. 263‒264
- ^ Langley & Hattori 2014, p. 214
- ^ a b Abbott 1966, p. 18
- ^ Brown et al. 2014, p. 237
- ^ Barton 2021, p. 200
- ^ Borg & Dienes 1992, p. 26
- ^ Wiberg 2001, p. 796
- ^ Shang et al. 2021
- ^ Tang et al. 2021
- ^ a b Hein & Arena 2013, pp. 226, G-6
- ^ Lidin 1996, pp. 22, 29; 322, 165; 381, 173–174; 12, 147; 157 [B; P; Se; As; I]; Housecroft & Sharpe 2008, p. 472 [I]
- ^ Lidin 1996, pp. 52, 58; 386; 140; 361, 365; 372, 376; 403 [C; Si; Ge; S; Sb; Te]; Rochow 1973, p. 1338 [Si]; Sanderson 1967, p. 172 [Ge]; Shkol'nikov 2010, p. 2127 [Sb]; Wiberg 2001, pp. 592 [Te]
- ^ Matson & Orbaek 2013, p. 85
- ^ Yoder, Suydam & Snavely 1975, p. 58
- ^ Young et al. 2018, p. 753
- ^ Brown et al. 2014, p. 227
- ^ Siekierski & Burgess 2002, pp. 21, 133, 177
- ^ Moore 2016; Burford, Passmore & Sanders 1989, p. 54
- ^ King & Caldwell 1954, p. 17; Brady & Senese 2009, p. 69
- ^ Chemical Abstracts Service 2021
- ^ Emsley 2011, pp. 81
- ^ Cockell 2019, p. 210
- ^ Scott 2014, p. 3
- ^ Emsley 2011, p. 184
- ^ Kneen, Rogers & Simpson 1972, pp. 226, 360
- ^ Lee 1996, p. 240
- ^ Greenwood & Earnshaw 2002, p. 43
- ^ Cressey 2010
- ^ Siekierski & Burgess 2002, pp. 24–25
- ^ Siekierski & Burgess 2002, p. 23
- ^ Petruševski & Cvetković 2018; Grochala 2018
- ^ Siekierski & Burgess 2002, pp. 52, 101, 111, 124, 194
- ^ Greenwood & Earnshaw 2002, pp. 27, 1232, 1234
- ^ Scerri 2020, pp. 407–420
- ^ Cox 2004, p. 146
- ^ a b Vernon 2013, p. 1706
- ^ Wiberg 2001, passim
- ^ Vernon 2020, p. 222
- ^ Atkins & Overton 2010, pp. 377, 389
- ^ Moody 1991, p. 391
- ^ Rodgers 2012, p. 504; Wulfsberg 2000, p. 726
- ^ Stellman 1998, chapter 104–211
- ^ Nakao 1992, p. 426–427
- ^ Hill & Holman 2000, p. 196
- ^ Wiberg 2001, pp. 1761–1762
- ^ Young 2006, p. 1285
- ^ Encyclopædia Britannica 2021
- ^ a b Matson & Orbaek 2013, p. 203
- ^ Chambers & Holliday 1982, pp. 273–274; Bohlmann 1992, p. 213; Jentzsch 2015, p. 247
- ^ Kernion 2019, p. 191; Cao et al. 2021, pp. 20–21; Hussain et al. 2023
- ^ Vassilakis, Kalemos & Mavridis 2014, p. 1; Hanley & Koga 2018, p. 24; Kaiho 2017, ch. 2, p. 1
- ^ Williams 2007, pp. 1550–1561: H, C, N, P, O, S
- ^ Wächtershäuser 2014, p. 5: H, C, N, P, O, S, Se
- ^ Hengeveld & Fedonkin, pp. 181–226: C, N, P, O, S
- ^ Wakeman 1899, p. 562
- ^ Fraps 1913, p. 11: H, C, Si, N, P, O, S, Cl
- ^ Parameswaran at al. 2020, p. 210: H, C, N, P, O, S, Se
- ^ Knight 2002, p. 148: H, C, N, P, O, S, Se
- ^ Fraústo da Silva & Williams 2001, p. 500: H, C, N, O, S, Se
- ^ a b Bailar et al. 1989, p. 742
- ^ Tshitoyan et al. 2019, pp. 95–98
- ^ Russell & Lee 2005, p. 419
- ^ Hampel & Hawley 1976, p. 174;
- ^ Goodrich 1844, p. 264; The Chemical News 1897, p. 189; Hampel & Hawley 1976, p. 191; Lewis 1993, p. 835; Hérold 2006, pp. 149–50
- ^ Tyler 1948, p. 105; Reilly 2002, pp. 5–6
- ^ a b Jones 2010, pp. 169–71
- ^ Stein 1983, p. 165
- ^ Jolly 1966, p. 20
- ^ Clugston & Flemming 2000, pp. 100–101, 104–105, 302
- ^ Maosheng 2020, p. 962
- ^ Mazej 2020
- ^ Wiberg 2001, p. 1131
- ^ Thompson 2004
- ^ Vernon 2020, p. 229
- ^ Cox 2000, pp. 258–259; Möller 2003, p. 173; Trenberth & Smith 2005, p. 864
- ^ Emsley 2011, p. 220
- ^ Emsley 2011, p. 440
- ^ Zhu et al. 2014, pp. 644–648
- ^ Emsley 2011, p. 181
- ^ Wiberg 2001, pp. 4022
- ^ a b Greenwood & Earnshaw 2002, p. 804
- ^ Rudolph 1973, p. 133: "Oxygen and the halogens in particular ... are therefore strong oxidizing agents."
- ^ Daniel & Rapp 1976, p. 55
- ^ a b Cotton et al. 1999, p. 554
- ^ Woodward et al. 1999, pp. 133–194
- ^ Phillips & Williams 1965, pp. 478–479
- ^ Moeller et al. 2012, p. 314
- ^ Lanford 1959, p. 176
- ^ Rayner-Canham 2020, p. 92, 139
- ^ Massey 2000, p. 113
- ^ Schmedt, Mangstl & Kraus 2012, p. 7847‒7849
- ^ Bailar, Moeller & Kleinberg 1965, p. 477
- ^ Mee 1964, p. 153
- ^ Masterton, Hurley & Neth 2011, p. 38
- ^ McCue 1963, p. 264
- ^ Dingle 2017, p. 101
- ^ a b Cox 1997, pp. 130–132; Emsley 2011, passim
- ^ Hurlbut 1961, p. 132
- ^ Emsley 2011, p. 478
- ^ Greenwood & Earnshaw 2002, p. 277
- ^ Atkins et al. 2006, p. 320
- ^ Greenwood & Earnshaw 2002, p. 482; Berger 1997, p. 86
- ^ Moss 1952, pp. 180, 202
- ^ a b c d Cao et al. 2021, pp. 20–21
- ^ Challoner 2014, p. 5; Government of Canada 2015; Gargaud et al. 2006, p. 447
- ^ Crichton 2012, p. 6; Scerri 2013; Los Alamos National Laboratory 2021
- ^ Wulfsberg 2000, pp. 273–274, 620
- ^ Seese & Daub 1985, p. 65
- ^ MacKay, MacKay & Henderson 2002, p. 209
- ^ Cousins, Davidson & García-Vivó 2013, pp. 11809–11811
- ^ a b Cao et al. 2021, p. 4
- ^ Liptrot 1983, p. 161
- ^ Wiberg 2001, pp. 257–258, 261–262
- ^ Scott & Kanda 1962, p. 153
- ^ Taylor 1960, p. 316
- ^ a b c d e Emsley 2011, passim
- ^ Crawford 1968, p. 540; Benner, Ricardo & Carrigan 2018, pp. 167—168: "The stability of the carbon—carbon bond ... has made it the first choice element to scaffold biomolecules. Hydrogen is needed for many reasons; at the very least, it terminates C–C chains. Heteroatoms (atoms that are neither carbon nor hydrogen) determine the reactivity of carbon-scaffolded biomolecules. In ... life, these are oxygen, nitrogen and, to a lesser extent, sulfur, phosphorus, selenium, and an occasional halogen."
- ^ Zhao, Tu & Chan 2021
- ^ Messler 2011, p. 10
- ^ King et al. 1994, p. 1344; Powell & Tims 1974, pp. 189–191; Cao et al. 2021, pp. 20–21
- ^ Vernon 2020, pp. 221–223; Rayner-Canham 2020, p. 216
- ^ Atkins 2001, pp. 24–25
- ^ National Center for Biotechnology Information 2021
- ^ Emsley 2011, p. 113
- ^ Greenwood & Earnshaw 2002, p. 270–271
- ^ Khan 2001, p. 59
- ^ Emsley 2011, pp. 376, 380, 640
- ^ a b Cox 1997, pp. 130
- ^ Emsley 2011, p. 393
- ^ Emsley 2011, pp. 515–516, 518
- ^ Boyd 2011, p. 570
- ^ Nelson 1987, p. 732: crust, atmosphere, hydrosphere; Fortescue 2012, pp. 56, 65: biomass
- ^ MacKay, MacKay & Henderson 2002, p. 200
- ^ Cox 1997, pp. 17, 19
- ^ Ostriker & Steinhardt 2001, pp. 46‒53; Zhu 2020, p. 27
- ^ Höll et al. 2007
- ^ U.S. Geological Survey 2023, p. 78
- ^ U.S. Geological Survey 2023, p. 153
- ^ Billing Metals & Manufacturing
- ^ U.S. Geological Survey 2023, p. 72, 86, 147
- ^ U.S. Geological Survey 2023, pp. 32, 34, 78, 82, 84, 91, 156, 158, 160, 170, 176; Kopteva, Kalimullin & Tcvetkov 2021, p. 1; Oztemel, Salt & Salt 2022, p. 5; Neice & Zornow 2016, p. 1268; Howe-Grant 1995, p. 17; Dalakov, Neuber & Herzog R 2020; Boysen, Cristóbal & Hilbig 2020, pp. 475–489; Gardner & Menon 2018, p. 455; Rajarathnam & Assallo 2016, p. 3; Xia, Ning & Zhu 2020
- ^ Chand, Kumar & Bhumla 2022, p. 22058
- ^ Berger 1997, p. 42
- ^ U.S. Geological Survey 1998
- ^ a b Boise State University 2020
- ^ Hu, Shen & Yu 2017, p. 595
- ^ Gardner & Menon 2018, p. 454; U.S. Geological Survey 2023, p. 78
- ^ National Institute of Standards and Technology 2013
- ^ Bolin 2017, p. 2-1
- ^ Emsley 2011, pp. 114–115
- ^ Emsley 2011, pp. 363–364
- ^ Emsley 2011, pp. 376–377; 379
- ^ Emsley 2011, pp. 484–489
- ^ Emsley 2011, pp. 511, 516–517
- ^ Bhuwalka et al. 2021, pp. 10097–10107
- ^ Gaffney & Marley 2017, p. 27
- ^ Labinger 2019, p. 305
- ^ Emsley 2011, pp. 42–43, 219–220, 263–264, 341, 441–442, 596, 609
- ^ Emsley 2011, pp. 84, 128, 180–181, 247
- ^ Cook 1923, p. 124
- ^ Weeks 1945, p. 161
- ^ Emsley 2011, pp. 113, 363, 378, 477, 514–515
- ^ Weeks 1945, p. 22; Emsley 2011, p. 40
- ^ Lavoisier 1790, p. 175
- ^ Jordan 2016
- ^ Stillman 1924, p. 213
- ^ de L'Aunay 1566, p. 7
- ^ Lémery 1699, p. 118; Dejonghe 1998, p. 329
- ^ Strathern 2000, p. 239
- ^ Criswell p. 1140
- ^ Salzberg 1991, p. 204
- ^ a b Goldsmith 1982, p. 526
- ^ Berzelius 1811, p. 258
- ^ Partington 1964, p. 168
- ^ a b Bache 1832, p. 250
- ^ Roscoe & Schormlemmer 1894, p. 4
- ^ Glinka 1959, p. 76
- ^ Hérold 2006, pp. 149–150
- ^ a b The Chemical News and Journal of Physical Science 1864
- ^ Kemshead 1875, p. 13
- ^ Kendall 1811, pp. 298–303
- ^ Brande 1821, p. 5
- ^ Edwards & Sienko 1983, pp. 691–96
- ^ Edwards & Sienko 1983, p. 693
- ^ Herzfeld 1927; Edwards 2000, pp. 100–03
- ^ Kubaschewski 1949, pp. 931–940
- ^ Remy 1956, p. 9
- ^ White 1962, p. 106: It makes a ringing sound when struck.
- ^ Johnson 1966, pp. 3–4
- ^ Horvath 1973, pp. 335–336
- ^ Rao & Ganguly 1986
- ^ Smith & Dwyer 1991, p. 65: The difference between melting point and boiling point.
- ^ a b Herman 1999, p. 702
- ^ Hill, Holman & Hulme 2017, p. 182: Atomic conductance is the electrical conductivity of one mole of a substance. It is equal to electrical conductivity divided by molar volume.
- ^ Suresh & Koga 2001, pp. 5940–5944
- ^ Johnson 2007, pp. 15–16
- ^ a b Edwards 2010, pp. 941–965
- ^ Povh & Rosin 2017, p. 131
- ^ Beach 1911
- ^ Stott 1956, pp. 100–102
- ^ Parish 1977, p. 178
- ^ Sanderson 1957, p. 229
- ^ Hare & Bache 1836, p. 310
- ^ Chambers 1743: "That which distinguishes metals from all other bodies ... is their heaviness ..."
- ^ Edwards 2000, p. 85
- ^ Russell & Lee 2005, p. 466
- ^ Atkins et al. 2006, pp. 320–21
- ^ Zhigal'skii & Jones 2003, p. 66
- ^ Emsley 1971, p. 1
- ^ Jones 2010, p. 169
- ^ Aylward & Findlay 2008, pp. 6–13; 126
- ^ Edelstein & Morrs 2009, p. 123
- ^ Arblaster JW (ed.) 2018, p. 269; Lavrukhina & Pozdnyakov 1970, p. 269
- ^ Bjerrum N 1936
- ^ Su et al. 2020, pp. 1621–1649
- ^ Oderberg 2007, p. 97
- ^ Bertomeu-Sánchez, Garcia-Belmar & Bensaude-Vincent 2002, pp. 248–249
- ^ Dupasquier 1844, pp. 66–67
- ^ Berzelius 1832, pp. 248–276
- ^ Renouf 1901, pp. 268
- ^ Vernon 2020, pp. 217–225
- ^ Tregarthen 2003, p. 10
- ^ Lewis 1993, pp. 28, 827
- ^ Lewis 1993, pp. 28, 813
- ^ a b c Rochow 1966, p. 4
- ^ Wiberg 2001, p. 780; Emsley 2011, p. 397; Rochow 1966, pp. 23, 84
- ^ Kneen, Rogers & Simpson 1972, pp. 321, 404, 436
- ^ Kneen, Rogers & Simpson 1972, p. 439
- ^ Kneen, Rogers & Simpson 1972, p. 465
- ^ Kneen, Rogers & Simpson 1972, p. 308
- ^ Wiberg 2001, pp. 505, 681, 781; Glinka 1958, p. 355
- ^ Godfrin & Lauter 1995, pp. 216‒218
- ^ Wiberg 2001, p. 416
- ^ Desai, James & Ho 1984, p. 1160; Matula 1979, p. 1260
- ^ Schaefer 1968, p. 76; Carapella 1968, pp. 29‒32
- ^ a b c Bogoroditskii & Pasynkov 1967, p. 77; Jenkins & Kawamura 1976, p. 88
- ^ Keeler & Wothers 2013, p. 293
- ^ Kneen, Rogers & Simpson 1972, p. 264
- ^ Rayner-Canham 2018, p. 203
- ^ Welcher 2001, p. 3–32: "The elements change from ... metalloids, to moderately active nonmetals, to very active nonmetals, and to a noble gas."
- ^ Mackin 2014, p. 80
- ^ Johnson 1966, pp. 105–108
- ^ Stein 1969, pp. 5396‒5397; Pitzer 1975, pp. 760‒761
- ^ Porterfield 1993, p. 336
- ^ a b Rao 2002, p. 22
- ^ Wells 1984, p. 534
- ^ Rochow 1966, p. 4; Atkins et al. 2006, pp. 8, 122–123
- ^ Wiberg 2001, p. 750
- ^ Sidorov 1960, pp. 599‒603
- ^ a b c d Puddephatt & Monaghan 1989, p. 59
- ^ Sanderson 1967, p. 172; Mingos 2019, p. 27
- ^ House 2008, p. 441
- ^ McMillan 2006, p. 823
- ^ Mingos 2019, p. 27; Sanderson 1967, p. 172
- ^ King 1995, p. 182
- ^ Wiberg 2001, p. 399
- ^ Kläning & Appelman 1988, p. 3760
- ^ Ritter 2011, p. 10
- ^ Yamaguchi & Shirai 1996, p. 3
- ^ Vernon 2020, p. 223
- ^ Vernon 2020, p. 220
- ^ Woodward et al. 1999, p. 134
- ^ Dalton 2019
- ^ Aylward & Findlay 2008, p. 132
- ^ Rahm, Zeng & Hoffmann 2019, p. 345
- ^ Aylward & Findlay 2008, p. 126
Bibliography
- Abbott D 1966, An Introduction to the Periodic Table, J. M. Dent & Sons, London
- Arblaster JW (ed.) 2018, Selected Values of the Crystallographic Properties of Elements, ASM International, Materials Park, Ohio, ISBN 978-1-62708-154-2
- Atkins PA 2001, The Periodic Kingdom: A Journey Into the Land of the Chemical Elements, Phoenix, London, ISBN 978-1-85799-449-0
- Atkins PA et al. 2006, Shriver & Atkins' Inorganic Chemistry, 4th ed., Oxford University Press, Oxford, ISBN 978-0-7167-4878-6
- Atkins PA & Overton T 2010, Shriver & Atkins' Inorganic Chemistry, 5th ed., Oxford University Press, Oxford, ISBN 978-0-19-923617-6
- Aylward G and Findlay T 2008, SI Chemical Data, 6th ed., John Wiley & Sons Australia, Milton, ISBN 978-0-470-81638-7
- Bache AD 1832, An essay on chemical nomenclature, prefixed to the treatise on chemistry; by J. J. Berzelius, American Journal of Science, vol. 22, pp. 248–277
- Bailar JC, Moeller T & Kleinberg J 1965, University Chemistry, DC Heath, Boston
- Bailar JC et al. 1989, Chemistry, 3rd ed., Harcourt Brace Jovanovich, San Diego, ISBN 978-0-15-506456-0
- Barton AFM 2021, States of Matter, States of Mind, CRC Press, Boca Raton, ISBN 978-0-7503-0418-4
- Beach FC (ed.) 1911, The Americana: A universal reference library, vol. XIII, Mel–New, Metalloid, Scientific American Compiling Department, New York
- Benner SA, Ricardo A & Carrigan MA 2018, "Is there a common chemical model for life in the universe?", in Cleland CE & Bedau MA (eds.), The Nature of Life: Classical and Contemporary Perspectives from Philosophy and Science, Cambridge University Press, Cambridge, ISBN 978-1-108-72206-3
- Berger LI 1997, Semiconductor Materials, CRC Press, Boca Raton, ISBN 978-0-8493-8912-2
- Bertomeu-Sánchez JR, Garcia-Belmar A & Bensaude-Vincent B 2002, "Looking for an order of things: Textbooks and chemical classifications in nineteenth century France", Ambix, vol. 49, no. 3, doi:10.1179/amb.2002.49.3.227
- Berzelius JJ & Bache AD 1832, "An essay on chemical nomenclature, prefixed to the treatise on chemistry", The American Journal of Science and Arts, vol. 22
- Billing Metals & Manufacturing, Silicon, Large Collectors sample. Element 14., accessed May 2, 2023
- Bjerrum N 1936, Bjerrum's Inorganic Chemistry, Heinemann, London
- Bodner GM & Pardue HL 1993, Chemistry, An Experimental Science, John Wiley & Sons, New York, ISBN 0-471-59386-9
- Bogoroditskii NP & Pasynkov VV 1967, Radio and Electronic Materials, Iliffe Books, London
- Bohlmann R 1992, "Synthesis of halides", in Winterfeldt E (ed.), Heteroatom manipulation, Pergamon Press, Oxford, ISBN 978-0-08-091249-3
- Boise State University 2020, "Cost-effective manufacturing methods breathe new life into black phosphorus research", Micron School of Materials Science and Engineering, accessed July 9, 2021
- Bolin P 2017, "Gas-insulated substations", in McDonald JD (ed.), Electric Power Substations Engineering, 3rd, ed., CRC Press, Boca Raton, FL, ISBN 978-1-4398-5638-3
- Borg RG & Dienes GJ 1992, The Physical Chemistry of Solids, Academic Press, Boston, ISBN 978-0-12-118420-9
- Boyd R 2011, "Selenium stories", Nature Chemistry, vol. 3, doi:10.1038/nchem.1076
- Boysen B, Cristóbal J & Hilbig J 2020, "Economic and environmental assessment of water reuse in industrial parks: case study based on a Model Industrial Park", Journal of Water Reuse and Desalination, vol. 10, no. 4, pp. 475–489, doi:10.2166/wrd.2020.034
- Brady JE & Senese F 2009, Chemistry: The study of Matter and its Changes, 5th ed., John Wiley & Sons, New York, ISBN 978-0-470-57642-7
- Brande WT 1821, A Manual of Chemistry, vol. II, John Murray, London
- Brown TL et al. 2014, Chemistry: The Central Science, 3rd ed., Pearson Australia: Sydney, ISBN 978-1-4425-5460-3
- Burford N, Passmore J & Sanders JCP 1989, "The preparation, structure, and energetics of homopolyatomic cations of groups 16 (the chalcogens) and 17 (the halogens)", in Liebman JF & Greenberg A (eds.), From atoms to polymers: isoelectronic analogies, VCH, New York, ISBN 978-0-89573-711-3
- Cao C et al. 2021, "Understanding periodic and non-periodic chemistry in periodic tables", Frontiers in Chemistry, vol. 8, no. 813, doi:10.3389/fchem.2020.00813
- Carapella SC 1968, "Arsenic" in Hampel CA (ed.), The Encyclopedia of the Chemical Elements, Reinhold, New York
- Carmalt CJ & Norman NC 1998, 'Arsenic, antimony and bismuth: Some general properties and aspects of periodicity', in Norman NC (ed.), Chemistry of Arsenic, Antimony and Bismuth, Blackie Academic & Professional, London, pp. 1–38, ISBN 0-7514-0389-X
- Challoner J 2014, The Elements: The New Guide to the Building Blocks of our Universe, Carlton Publishing Group, ISBN 978-0-233-00436-5
- Chambers E 1743, in "Metal", Cyclopedia: Or an Universal Dictionary of Arts and Sciences (etc.), vol. 2, D Midwinter, London
- Chambers C & Holliday AK 1982, Inorganic Chemistry, Butterworth & Co., London, ISBN 978-0-408-10822-5
- Chand H, Kumar A & Bhumla P 2022, "Scalable production of ultrathin boron nanosheets from a low-cost precursor", Advanced Materials Interfaces, vol. 9, no. 2, doi:10.1002/admi.202200508
- Charlier J-C, Gonze X, Michenaud J-P 1994, First-principles study of the stacking effect on the electronic properties of graphite(s), Carbon, vol. 32, no. 2, pp. 289–99, doi:10.1016/0008-6223(94)90192-9
- Chemical Abstracts Service 2021, CAS REGISTRY database as of November 2, Case #01271182
- Cherim SM 1971, Chemistry for Laboratory Technicians, Saunders, Philadelphia, ISBN 978-0-7216-2515-7
- Chung DD 1987, "Review of exfoliated graphite", Journal of Materials Science, vol. 22, doi:10.1007/BF01132008
- Clugston MJ & Flemming R 2000, Advanced Chemistry, Oxford University Press, Oxford, ISBN 978-0-19-914633-8
- Cockell C 2019, The Equations of Life: How Physics Shapes Evolution, Atlantic Books, London, ISBN 978-1-78649-304-0
- Cook CG 1923, Chemistry in Everyday Life: With Laboratory Manual, D Appleton, New York
- Cotton A et al. 1999, Advanced Inorganic Chemistry, 6th ed., Wiley, New York, ISBN 978-0-471-19957-1
- Cousins DM, Davidson MG & García-Vivó D 2013, "Unprecedented participation of a four-coordinate hydrogen atom in the cubane core of lithium and sodium phenolates", Chemical Communications, vol. 49, doi:10.1039/C3CC47393G
- Cox AN (ed.) 2000, Allen's Astrophysical Quantities, 4th ed., AIP Press, New York, ISBN 978-0-387-98746-0
- Cox PA 1997, The Elements: Their Origins, Abundance, and Distribution, Oxford University Press, Oxford, ISBN 978-0-19-855298-7
- Cox T 2004, Inorganic Chemistry, 2nd ed., BIOS Scientific Publishers, London, ISBN 978-1-85996-289-3
- Crawford FH 1968, Introduction to the Science of Physics, Harcourt, Brace & World, New York
- Crichton R 2012, Biological Inorganic Chemistry: A New Introduction to Molecular Structure and Function, 2nd ed., Elsevier, Amsterdam, ISBN 978-0-444-53783-6
- Cressey D 2010, "Chemists re-define hydrogen bond", Nature newsblog, accessed August 23, 2017
- Criswell B 2007, "Mistake of having students be Mendeleev for just a day", Journal of Chemical Education, vol. 84, no. 7, pp. 1140–1144, doi:10.1021/ed084p1140
- Dalakov P, Neuber E & Herzog R 2020, "Innovative neon refrigeration unit operating down to 30 K", MATEC Web of Conferences, vol. 324, doi:10.1051/matecconf/202032401003
- Dalton L 2019, "Argon reacts with nickel under pressure-cooker conditions", Chemical & Engineering News, accessed November 6, 2019
- Daniel PL & Rapp RA 1976, "Halogen corrosion of metals", in Fontana MG & Staehle RW (eds.), Advances in Corrosion Science and Technology, Springer, Boston, doi:10.1007/978-1-4615-9062-0_2
- de L'Aunay L 1566, Responce au discours de maistre Iacques Grevin, docteur de Paris, qu'il a escript contre le livre de maistre Loys de l'Aunay, medecin en la Rochelle, touchant la faculté de l'antimoine (Response to the Speech of Master Jacques Grévin,... Which He Wrote Against the Book of Master Loys de L'Aunay,... Touching the Faculty of Antimony), De l'Imprimerie de Barthelemi Berton, La Rochelle
- Dejonghe L 1998, "Zinc–lead deposits of Belgium", Ore Geology Reviews, vol. 12, no. 5, 329–354, doi:10.1016/s0169-1368(98)00007-9
- Desai PD, James HM & Ho CY 1984, "Electrical resistivity of aluminum and manganese", Journal of Physical and Chemical Reference Data, vol. 13, no. 4, doi:10.1063/1.555725
- Dingle A 2017, The Elements: An Encyclopedic Tour of the Periodic Table, Quad Books, Brighton, ISBN 978-0-85762-505-2
- Donohue J 1982, The Structures of the Elements, Robert E. Krieger, Malabar, Florida, ISBN 978-0-89874-230-5
- Du Y, Ouyang C, Shi S & Lei M 2010, "Ab initio studies on atomic and electronic structures of black phosphorus", Journal of Applied Physics, vol. 107, no. 9, pp. 093718–1–4, doi:10.1063/1.3386509
- Dupasquier A 1844, Traité élémentaire de chimie industrielle, Charles Savy Juene, Lyon
- Earl B & Wilford D 2021, Cambridge O Level Chemistry, Hodder Education, London, ISBN 978-1-3983-1059-9
- Edelstein NM & Morrs LR 2009, "Chemistry of the actinide elements", in Nagy S (ed.), Radiochemistry and Nuclear Chemistry: Volume II, Encyclopedia of Life Support Systems, EOLSS Publishers, Oxford, pp. 118–176, ISBN 978-1-84826-577-6
- Edwards PP 2000, "What, why and when is a metal?", in Hall N (ed.), The New Chemistry, Cambridge University, Cambridge, pp. 85–114, ISBN 978-0-521-45224-3
- Edwards PP et al. 2010, "... a metal conducts and a non-metal doesn’t", Philosophical Transactions of the Royal Society A, 2010, vol, 368, no. 1914, doi:10.1098/rsta.2009.0282
- Edwards PP & Sienko MJ 1983, "On the occurrence of metallic character in the periodic table of the elements", Journal of Chemical Education, vol. 60, no. 9, doi:10.1021/ed060p691, PMID 25666074
- Elliot A 1929, "The absorption band spectrum of chlorine", Proceedings of the Royal Society A, vol. 123, no. 792, pp. 629–644, doi:10.1098/rspa.1929.0088
- Emsley J 1971, The Inorganic Chemistry of the Non-metals, Methuen Educational, London, ISBN 978-0-423-86120-4
- Emsley J 2011, Nature's Building Blocks: An A–Z Guide to the Elements, Oxford University Press, Oxford, ISBN 978-0-19-850341-5
- Encyclopædia Britannica 2021, Periodic table, accessed September 21, 2021
- Evans RC 1966, An Introduction to Crystal Chemistry, 2nd ed., Cambridge University, Cambridge
- Faraday M 1853, The Subject Matter of a Course of Six Lectures on the Non-metallic Elements, (arranged by John Scoffern), Longman, Brown, Green, and Longmans, London
- Florez et al. 2022, From the gas phase to the solid state: The chemical bonding in the superheavy element flerovium, The Journal of Chemical Physics, vol. 157, 064304, doi:10.1063/5.0097642
- Fortescue JAC 2012, Environmental Geochemistry: A Holistic Approach, Springer-Verlag, New York, ISBN 978-1-4612-6047-9
- Fox M 2010, Optical Properties of Solids, 2nd ed., Oxford University Press, New York, ISBN 978-0-19-957336-3
- Fraps GS 1913, Principles of Agricultural Chemistry, The Chemical Publishing Company, Easton, PA
- Fraústo da Silva JJR & Williams RJP 2001, The Biological Chemistry of the Elements: The Inorganic Chemistry of Life, 2nd ed., Oxford University Press, Oxford, ISBN 978-0-19-850848-9
- Gaffney J & Marley N 2017, General Chemistry for Engineers, Elsevier, Amsterdam, ISBN 978-0-12-810444-6
- Gardner AJ & Menon DK 2018, "Moving to human trials for argon neuroprotection in neurological injury: A narrative review", British Journal of Anaesthesia, vol. 120, no. 4, pp. 453–468, doi:10.1016/j.bja.2017.10.017
- Gargaud M et al. (eds.) 2006, Lectures in Astrobiology, vol. 1, part 1: The Early Earth and Other Cosmic Habitats for Life, Springer, Berlin, ISBN 978-3-540-29005-6
- Glinka N 1958, General chemistry, Sobolev D (trans.), Foreign Languages Publishing House, Moscow
- Glinka N 1959, General chemistry, Foreign Languages Publishing House, Moscow
- Godfrin H & Lauter HJ 1995, "Experimental properties of 3He adsorbed on graphite", in Halperin WP (ed.), Progress in Low Temperature Physics, volume 14, Elsevier Science B.V., Amsterdam, ISBN 978-0-08-053993-5
- Godovikov AA & Nenasheva N 2020, Structural-chemical Systematics of Minerals, 3rd ed., Springer, Cham, Switzerland, ISBN 978-3-319-72877-3
- Goldsmith RH 1982, 'Metalloids', Journal of Chemical Education, vol. 59, no. 6, pp. 526–527, doi:10.1021/ed059p526
- Goodrich BG 1844, A Glance at the Physical Sciences, Bradbury, Soden & Co., Boston
- Government of Canada 2015, Periodic table of the elements, accessed August 30, 2015
- Greenwood NN & Earnshaw A 2002, Chemistry of the Elements, 2nd ed., Butterworth-Heinemann, ISBN 978-0-7506-3365-9
- Grochala W 2018, "On the position of helium and neon in the Periodic Table of Elements", Foundations of Chemistry, vol. 20, pp. 191–207, doi:10.1007/s10698-017-9302-7
- Hampel CA & Hawley GG 1976, Glossary of Chemical Terms, Van Nostrand Reinhold, New York, ISBN 978-0-442-23238-2
- Hanley JJ & Koga KT 2018, "Halogens in terrestrial and cosmic geochemical systems: Abundances, geochemical behaviours, and analytical methods" in The Role of Halogens in Terrestrial and Extraterrestrial Geochemical Processes: Surface, Crust, and Mantle, Harlov DE & Aranovich L (eds.), Springer, Cham, ISBN 978-3-319-61667-4
- Hare RA & Bache F 1836, Compendium of the Course of Chemical Instruction in the Medical Department of the University of Pennsylvania, 3rd ed., JG Auner, Philadelphia
- Hein M & Arena S 2013, Foundations of College Chemistry, John Wiley & Sons, ISBN 978-1-118-29823-7
- Hengeveld R & Fedonkin MA 2007, "Bootstrapping the energy flow in the beginning of life", Acta Biotheoretica, vol. 55, doi:10.1007/s10441-007-9019-4
- Herman ZS 1999, "The nature of the chemical bond in metals, alloys, and intermetallic compounds, according to Linus Pauling", in Maksić, ZB, Orville-Thomas WJ (eds.), 1999, Pauling's Legacy: Modern Modelling of the Chemical Bond, Elsevier, Amsterdam, doi:10.1016/S1380-7323(99)80030-2
- Hermann A, Hoffmann R & Ashcroft NW 2013, "Condensed astatine: Monatomic and metallic", Physical Review Letters, vol. 111, doi:10.1103/PhysRevLett.111.116404
- Hérold A 2006, "An arrangement of the chemical elements in several classes inside the periodic table according to their common properties", Comptes Rendus Chimie, vol. 9, no. 1, doi:10.1016/j.crci.2005.10.002
- Herzfeld K 1927, "On atomic properties which make an element a metal", Physical Review, vol. 29, no. 5, doi:10.1103/PhysRev.29.701
- Hill G & Holman J 2000, Chemistry in Context, 5th ed., Nelson Thornes, Cheltenham, ISBN 0-17-448307-4
- Hill G, Holman J & Hulme PG 2017, Chemistry in Context, 7th ed., Oxford University Press, Oxford, ISBN 978-0-19-839618-5
- Holderness A & Berry M 1979, Advanced Level Inorganic Chemistry, 3rd ed., Heinemann Educational Books, London, ISBN 978-0-435-65435-1
- Höll, Kling & Schroll E 2007, "Metallogenesis of germanium—A review", Ore Geology Reviews, vol. 30, nos. 3–4, pp. 145–180, doi:10.1016/j.oregeorev.2005.07.034
- Horvath AL 1973, "Critical temperature of elements and the periodic system", Journal of Chemical Education, vol. 50, no. 5, doi:10.1021/ed050p335
- House JE 2008, Inorganic Chemistry, Elsevier, Amsterdam, ISBN 978-0-12-356786-4
- Housecroft CE & Sharpe AG 2008, Inorganic Chemistry, 3rd ed., Prentice-Hall, Harlow, ISBN 978-0-13-175553-6
- Howe-Grant MI (ed.) 1995, Fluorine Chemistry: A Comprehensive Treatment, John Wiley and Sons, New York, p. 17, ISBN 978-0-471-12031-5
- Hu Z, Shen Z & Yu JC 2017, "Phosphorus containing materials for photocatalytic hydrogen evolution", Green Chemistry, vol. 19, no. 3, pp. 588–613, doi:10.1039/C6GC02825J
- Hurlbut Jr CS 1961, Manual of Mineralogy, 15th ed., John Wiley & Sons, New York
- Hussain et al. 2023, "Tuning the electronic properties of molybdenum di-sulphide monolayers via doping using first-principles calculations", Physica Scripta, vol. 98, no. 2, doi:10.1088/1402-4896/acacd1
- IUPAC Periodic Table of the Elements, accessed October 11, 2021
- Janas D, Cabrero-Vilatela, A & Bulmer J 2013, "Carbon nanotube wires for high-temperature performance", Carbon, vol. 64, pp. 305–314, doi:10.1016/j.carbon.2013.07.067
- Jenkins GM & Kawamura K 1976, Polymeric Carbons—Carbon Fibre, Glass and Char, Cambridge University Press, Cambridge, ISBN 978-0-521-20693-8
- Jentzsch AV & Matile S 2015, "Anion transport with halogen bonds", in Metrangolo P & Resnati G (eds.), Halogen Bonding I: Impact on Materials Chemistry and Life Sciences, Springer, Cham, ISBN 978-3-319-14057-5
- Johnson D (ed.) 2007, Metals and Chemical Change, RSC Publishing, Cambridge, ISBN 978-0-85404-665-2
- Johnson RC 1966, Introductory Descriptive Chemistry, WA Benjamin, New York
- Jolly WL 1966, The Chemistry of the Non-metals, Prentice-Hall, Englewood Cliffs, New Jersey
- Jones BW 2010, Pluto: Sentinel of the Outer Solar System, Cambridge University, Cambridge, ISBN 978-0-521-19436-5
- Jordan JM 2016 " 'Ancient episteme' and the nature of fossils: a correction of a modern scholarly error", History and Philosophy of the Life Sciences, vol. 38, no, 1, pp. 90–116, doi:10.1007/s40656-015-0094-6
- Kaiho T 2017, Iodine Made Simple, CRC Press, e-book, doi:10.1201/9781315158310
- Keeler J & Wothers P 2013, Chemical Structure and Reactivity: An Integrated Approach, Oxford University Press, Oxford, ISBN 978-0-19-960413-5
- Kemshead WB 1875, Inorganic chemistry, William Collins, Sons, & Company, London
- Kendall EA 1811, Pocket Encyclopædia, 2nd ed., vol. III, Longman, Hurst, Rees, Orme, and Co., London
- Kernion MC & Mascetta JA 2019, Chemistry: The Easy Way, 6th ed., Kaplan, New York, ISBN 978-1-4380-1210-0
- Khan N 2001, An Introduction to Physical Geography, Concept Publishing, New Delhi, ISBN 978-81-7022-898-1
- King RB 1994, Encyclopedia of Inorganic Chemistry, vol. 3, John Wiley & Sons, New York, ISBN 978-0-471-93620-6
- King RB 1995, Inorganic Chemistry of Main Group Elements, VCH, New York, ISBN 978-1-56081-679-9
- King GB & Caldwell WE 1954, The Fundamentals of College Chemistry, American Book Company, New York
- Kläning UK & Appelman EH 1988, "Protolytic properties of perxenic acid", Inorganic Chemistry, vol. 27, no. 21, doi:10.1021/ic00294a018
- Kneen WR, Rogers MJW & Simpson P 1972, Chemistry: Facts, Patterns, and Principles, Addison-Wesley, London, ISBN 978-0-201-03779-1
- Knight J 2002, Science of Everyday Things: Real-life chemistry, Gale Group, Detroit, ISBN 9780787656324
- Koenig SH 1962, in Proceedings of the International Conference on the Physics of Semiconductors, held at Exeter, July 16−20, 1962, The Institute of Physics and the Physical Society, London
- Kopteva A, Kalimullin L & Tcvetkov P 2021, "Prospects and obstacles for green hydrogen production in Russia", Energies, vol. 14, no. 3, pp. 1–21, doi:10.3390/en14030718
- Kosanke et al. 2012, Encyclopedic Dictionary of Pyrotechnics (and Related Subjects), Part 3 – P to Z, Pyrotechnic Reference Series No. 5, Journal of Pyrotechnics, Whitewater, Colorado, ISBN 978-1-889526-21-8
- Kubaschewski O 1949, "The change of entropy, volume and binding state of the elements on melting", Transactions of the Faraday Society, vol. 45, doi:10.1039/TF9494500931
- Kugler HK & Keller C (eds) 1985, Gmelin Handbook of Inorganic and Organometallic chemistry, 8th ed., 'At, Astatine', system no. 8a, Springer-Verlag, Berlin, ISBN 3-540-93516-9
- Labinger JA 2019, "The history (and pre-history) of the discovery and chemistry of the noble gases", in Giunta CJ, Mainz VV & Girolami GS (eds.), 150 Years of the Periodic Table: A Commemorative Symposium, Springer Nature, Cham, Switzerland, ISBN 978-3-030-67910-1
- Lanford OE 1959, Using Chemistry, McGraw-Hill, New York
- Langley RH & Hattori H 2014, 1,001 Practice Problems: Chemistry For Dummies, John Wiley & Sons, Hoboken, NJ, ISBN 978-1-118-54932-2
- Larrañaga MD, Lewis RJ & Lewis RA 2016, Hawley's Condensed Chemical Dictionary, 16th ed., Wiley, Hoboken, New York, ISBN 978-1-118-13515-0
- Lavoisier A 1790, Elements of Chemistry, R Kerr (trans.), William Creech, Edinburgh
- Lavrukhina AK & Pozdnyakov AA 1970, Analytical Chemistry of Technetium, Promethium, Astatine, and Francium, R Kondor, trans., Ann Arbor–Humphrey Science Publishers, Ann Arbor, ISBN 978-0-250-39923-9
- Lee JD 1996, Concise Inorganic Chemistry, 5th ed., Blackwell Science, Oxford, ISBN 978-0-632-05293-6
- Lémery N 1714, Traité universel des drogues simples, mises en ordre alphabetique, L d'Houry, Paris, p. 118
- Lewis RJ 1993, Hawley's Condensed Chemical Dictionary, 12th ed., Van Nostrand Reinhold, New York, ISBN 978-0-442-01131-4
- Lidin RA 1996, Inorganic Substances Handbook, Begell House, New York, ISBN 978-0-8493-0485-9
- Liptrot GF 1983, Modern Inorganic Chemistry, 4th ed., Bell & Hyman, ISBN 978-0-7135-1357-8
- Los Alamos National Laboratory 2021, Periodic Table of Elements: A Resource for Elementary, Middle School, and High School Students, accessed September 19, 2021
- Luchinskii GP & Trifonov DN 1981, "Some problems of chemical elements classification and the structure of the periodic system", in Uchenie o Periodichnosti. Istoriya i Sovremennoct, (Russian) Nauka, Moscow
- MacKay KM, MacKay RA & Henderson W 2002, Introduction to Modern Inorganic Chemistry, 6th ed., Nelson Thornes, Cheltenham, ISBN 978-0-7487-6420-4
- Mackin M 2014, Study Guide to Accompany Basics for Chemistry, Elsevier Science, Saint Louis, ISBN 978-0-323-14652-4
- Maosheng M 2020, "Noble gases in solid compounds show a rich display of chemistry with enough pressure", Frontiers in Chemistry, vol. 8, doi:10.3389/fchem.2020.570492
- Massey AG 2000, Main Group Chemistry, 2nd ed., John Wiley & Sons, Chichester, ISBN 978-0-471-49039-5
- Masterton W, Hurley C & Neth E 2011, Chemistry: Principles and Reactions, 7th ed., Brooks/Cole, Belmont, California, ISBN 978-1-111-42710-8
- Matson M & Orbaek AW 2013, Inorganic Chemistry for Dummies, John Wiley & Sons: Hoboken, ISBN 978-1-118-21794-8
- Matula RA 1979, "Electrical resistivity of copper, gold, palladium, and silver", Journal of Physical and Chemical Reference Data, vol. 8, no. 4, doi:10.1063/1.555614
- Mazej Z 2020, "Noble-gas chemistry more than half a century after the first report of the noble-gas compound", Molecules, vol. 25, no. 13, doi:10.3390/molecules25133014, PMID 32630333, PMC 7412050
- McCue JJ 1963, World of Atoms: An Introduction to Physical Science, Ronald Press, New York
- McMillan P 2006, "A glass of carbon dioxide", Nature, vol. 441, doi:10.1038/441823a
- Mee AJ 1964, Physical Chemistry, Aldine Publishing, Chicago
- Messler Jr RW 2011, The Essence of Materials for Engineers, Jones and Bartlett Learning, Sudbury, Massachusetts, ISBN 978-0-7637-7833-0
- Mewes et al. 2019, Copernicium: A relativistic noble liquid, Angewandte Chemie International Edition, vol. 58, pp. 17964–17968, doi:10.1002/anie.201906966
- Mingos DMP 2019, "The discovery of the elements in the Periodic Table", in Mingos DMP (ed.), The Periodic Table I. Structure and Bonding, Springer Nature, Cham, doi:10.1007/978-3-030-40025-5
- Moeller T et al. 2012, Chemistry: With Inorganic Qualitative Analysis, Academic Press, New York, ISBN 978-0-12-503350-3
- Möller D 2003, Luft: Chemie, Physik, Biologie, Reinhaltung, Recht, Walter de Gruyter, Berlin, ISBN 978-3-11-016431-2
- Moody B 1991, Comparative Inorganic Chemistry, 3rd ed., Edward Arnold, London, ISBN 978-0-7131-3679-1
- Moore JT 2016, Chemistry for Dummies, 2nd ed., ch. 16, Tracking periodic trends, John Wiley & Sons: Hoboken, ISBN 978-1-119-29728-4
- Morita A 1986, 'Semiconducting black phosphorus', Journal of Applied Physics A, vol. 39, no. 4, pp. 227–42, doi:10.1007/BF00617267
- Morely HF & Muir MM 1892, Watt's Dictionary of Chemistry, vol. 3, Longman's Green, and Co., London
- Moss, TS 1952, Photoconductivity in the Elements, Butterworths Scientific, London
- Nakao Y 1992, "Dissolution of noble metals in halogen–halide–polar organic solvent systems", Journal of the Chemical Society, Chemical Communications, no. 5, doi:10.1039/C39920000426
- National Center for Biotechnology Information 2021, "PubChem compound summary for CID 402, Hydrogen sulfide", accessed August 31, 2021
- National Institute of Standards and Technology 2013, SRM 4972 – Radon-222 Emanation Standard, accessed August 1, 2021
- Nefedov VD et al. 1968, 'Astatine', Russian Chemical Reviews, vol. 37, no. 2, pp. 87–98, doi:10.1070/rc1968v037n02abeh0
- Neice AE & Zornow MH 2016, "Editorial: Xenon anaesthesia for all, or only a select few?", Anaesthesia, vol. 71, no. 11, pp. 1259–1272 (1268), doi:10.1111/anae.13569
- Nelson PG 1987, "Important elements", Journal of Chemical Education, vol. 68, no. 9, doi:10.1021/ed068p732
- Oderberg DS 2007, Real Essentialism, Routledge, New York, ISBN 978-1-134-34885-5
- Ostriker JP & Steinhardt PJ 2001, "The quintessential universe", Scientific American, vol. 284, no. 1, pp. 46–53 PMID 11132422, doi:10.1038/scientificamerican0101-46
- Oxtoby DW, Gillis HP & Butler LJ 2015, Principles of Modern Chemistry, 8th ed., Cengage Learning, Boston, ISBN 978-1-305-07911-3
- Oztemel BH, Salt I, Salt Y 2022, "Carbon dioxide utilization: Process simulation of synthetic fuel production from flue gases", Chemical Industry and Chemical Engineering Quarterly, vol. 28, no. 4, doi:10.2298/CICEQ211025005B
- Parameswaran P et al. 2020, "Phase evolution and characterization of mechanically alloyed hexanary Al16.6Mg16.6Ni16.6Cr16.6Ti16.6Mn16.6 high entropy alloy", Metal Powder Report, vol. 75, no. 4, doi:10.1016/j.mprp.2019.08.001
- Parish RV 1977, The Metallic Elements, Longman, London, ISBN 978-0-582-44278-8
- Parkes GD & Mellor JW 1943, Mellor's Nodern Inorganic Chemistry, Longmans, Green and Co., London
- Partington JR 1944, A Text-book of Inorganic Chemistry, 5th ed., Macmillan & Co., London
- Partington JR 1964, A history of chemistry, vol. 4, Macmillan, London
- Pascoe KJ 1982, An Introduction to the Properties of Engineering Materials, 3rd ed., Von Nostrand Reinhold (UK), Wokingham, Berkshire, ISBN 978-0-442-30233-7
- Petruševski VM & Cvetković J 2018, "On the 'true position' of hydrogen in the Periodic Table", Foundations of Chemistry, vol. 20, pp. 251–260, doi:10.1007/s10698-018-9306-y
- Phillips CSG & Williams RJP 1965, Inorganic Chemistry, vol. 1, Principles and non-metals, Clarendon Press, Oxford
- Phillips JC 1973, "The chemical structure of solids", in Hannay NB (ed.), Treatise on Solid State Chemistry, vol. 1, Plenum Press, New York, pp. 1–42, ISBN 978-1-4684-2663-2
- Pitzer K 1975, "Fluorides of radon and elements 118", Journal of the Chemical Society, Chemical Communications, no. 18, doi:10.1039/C3975000760B
- Porterfield WW 1993, Inorganic Chemistry, Academic Press, San Diego, ISBN 978-0-12-562980-5
- Povh B & Rosina M 2017, Scattering and Structures: Essentials and Analogies in Quantum Physics, 2nd ed., Springer, Berlin, doi:10.1007/978-3-662-54515-7
- Powell P & Timms P 1974, The Chemistry of the Non-Metals, Chapman and Hall, London, ISBN 978-0-412-12200-2
- Puddephatt RJ & Monaghan PK 1989, The Periodic Table of the Elements, 2nd ed., Clarendon Press, Oxford, ISBN 978-0-19-855516-2
- Rahm M, Zeng T & Hoffmann R 2019, "Electronegativity seen as the ground-state average valence electron binding energy", Journal of the American Chemical Society, vol. 141, no. 1, pp. 342−351, doi:10.1021/jacs.8b10246
- Rajarathnam GP & Assallo AM 2016, The Zinc/bromine Flow Battery: Materials Challenges and Practical Solutions for Technology Advancement, Springer, Singapore, p. 3, ISBN 978-981-287-645-4
- Rao KY 2002, Structural Chemistry of Glasses, Elsevier, Oxford, ISBN 978-0-08-043958-7
- Rao CNR & Ganguly PA 1986, "New criterion for the metallicity of elements", Solid State Communications, vol. 57, no. 1, pp. 5–6, doi:10.1016/0038-1098(86)90659-9
- Rayner-Canham G 2018, "Organizing the transition metals", in Scerri E & Restrepo G (Ed's.) Mendeleev to Oganesson: A multidisciplinary perspective on the periodic table, Oxford University, New York, ISBN 978-0-190-668532
- Rayner-Canham G 2020, The Periodic Table: Past, Present and Future, World Scientific, New Jersey, ISBN 978-981-121-850-7
- Regnault MV 1853, Elements of Chemistry, vol. 1, 2nd ed., Clark & Hesser, Philadelphia
- Reilly C 2002, Metal Contamination of Food, Blackwell Science, Oxford, ISBN 978-0-632-05927-0
- Remy H 1956, Treatise on Inorganic Chemistry, Anderson JS (trans.), Kleinberg J (ed.), vol. II, Elsevier, Amsterdam
- Renouf E 1901, "Lehrbuch der Anorganischen Chemie", Science, vol. 13, no. 320, doi:10.1126/science.13.320.268
- Restrepo G, Llanos EJ & Mesa H 2006, "Topological space of the chemical elements and its properties", Journal of Mathematical Chemistry, vol. 39, doi:10.1007/s10910-005-9041-1
- Ritter SK 2011, "The case of the missing xenon", Chemical & Engineering News, vol. 89, no. 9, ISSN 0009-2347
- Rochow EG 1966, The Metalloids, DC Heath and Company, Boston
- Rochow EG 1973, "Silicon", in Bailar JC et al. (eds.), Comprehensive Inorganic Chemistry, vol. 1, Pergamon Press, Oxford, ISBN 978-0-08-015655-2
- Rodgers GE 2012, Descriptive Inorganic, Coordination, and Solid State Chemistry, 3rd ed., Brooks/Cole, Belmont, California, ISBN 978-0-8400-6846-0
- Roscoe HE & Schorlemmer FRS 1894, A treatise on chemistry: Volume II: The metals, D Appleton, New York
- Royal Society of Chemistry 2021, Periodic Table: Non-metal, accessed September 3, 2021
- Rudolph J 1973, Chemistry for the Modern Mind, Macmillan, New York
- Russell AM & Lee KL 2005, Structure-Property Relations in Nonferrous Metals, Wiley-Interscience, New York, ISBN 0-471-64952-X
- Salinas JT 2019 Exploring Physical Science in the Laboratory, Moreton Publishing, Englewood, Colorado, ISBN 978-1-61731-753-8
- Salzberg HW 1991, From Caveman to Chemist: Circumstances and Achievements, American Chemical Society, Washington, DC, ISBN 0-8412-1786-6
- Sanderson RT 1957, "An electronic distinction between metals and nonmetals", Journal of Chemical Education, vol. 34, no. 5, doi:10.1021/ed034p229
- Sanderson RT 1967, Inorganic Chemistry, Reinhold, New York
- Scerri E (ed.) 2013, 30-Second Elements: The 50 Most Significant Elements, Each Explained In Half a Minute, Ivy Press, London, ISBN 978-1-84831-616-4
- Scerri E 2020, The Periodic Table: Its Story and Its Significance, Oxford University Press, New York, ISBN 978-0-19091-436-3
- Schaefer JC 1968, "Boron" in Hampel CA (ed.), The Encyclopedia of the Chemical Elements, Reinhold, New York
- Schlager N & Lauer J (eds.) 2000, Science and Its Times: 1700–1799, volume 4 of Science and Its Times: Understanding the Social Significance of Scientific Discovery, Gale Group, ISBN 978-0-7876-3932-7
- Schmedt auf der Günne J, Mangstl M & Kraus F 2012, "Occurrence of difluorine F2 in nature—In situ proof and quantification by NMR spectroscopy", Angewandte Chemie International Edition, vol. 51, no. 31, doi:10.1002/anie.201203515
- Scott D 2014, Around the World in 18 Elements, Royal Society of Chemistry, e-book, ISBN 978-1-78262-509-4
- Scott EC & Kanda FA 1962, The Nature of Atoms and Molecules: A General Chemistry, Harper & Row, New York
- Seese WS & Daub GH 1985, Basic Chemistry, 4th ed., Prentice-Hall, Englewood Cliffs, NJ, ISBN 978-0-13-057811-2
- Segal BG 1989, Chemistry: Experiment and Theory, 2nd ed., John Wiley & Sons, New York, ISBN 0-471-84929-4
- Shang et al. 2021, "Ultrahard bulk amorphous carbon from collapsed fullerene", Nature, vol. 599, pp. 599–604, doi:10.1038/s41586-021-03882-9
- Shkol’nikov EV 2010, "Thermodynamic characterization of the amphoterism of oxides M2O3 (M = As, Sb, Bi) and their hydrates in aqueous media, Russian Journal of Applied Chemistry, vol. 83, no. 12, pp. 2121–2127, doi:10.1134/S1070427210120104
- Sidorov TA 1960, "The connection between structural oxides and their tendency to glass formation", Glass and Ceramics, vol. 17, no. 11, doi:10.1007BF00670116
- Siekierski S & Burgess J 2002, Concise Chemistry of the Elements, Horwood Press, Chichester, ISBN 978-1-898563-71-6
- Smith A & Dwyer C 1991, Key Chemistry: Investigating Chemistry in the Contemporary World: Book 1: Materials and Everyday Life, Melbourne University Press, Carlton, Victoria, ISBN 978-0-522-84450-4
- Smits et al. 2020, "Oganesson: A noble gas element that is neither noble nor a gas", Angewandte Chemie International Edition, vol. 59, pp. 23636–23640, doi:10.1002/anie.202011976
- Stein L 1969, "Oxidized radon in halogen fluoride solutions", Journal of the American Chemical Society, vol. 19, no. 19, doi:10.1021/ja01047a042
- Stein L 1983, "The chemistry of radon", Radiochimica Acta, vol. 32, doi:10.1524/ract.1983.32.13.163
- Stellman JM (ed.) 1998, Encyclopaedia of Occupational Health and Safety, vol. 4, 4th ed., International Labour Office, Geneva, ISBN 978-92-2-109817-1
- Steudel R 1977, Chemistry of the Non-metals: With an Introduction to atomic Structure and Chemical Bonding, Walter de Gruyter, Berlin, ISBN 978-3-11-004882-7
- Steudel R & Eckert B 2003, "Solid sulfur allotropes", in Steudel R (ed.), Elemental Sulfur and Sulfur-rich Compounds I, Springer-Verlag, Berlin, ISBN 978-3-540-40191-9
- Steudel R 2020, Chemistry of the Non-metals: Syntheses - Structures - Bonding - Applications, in collaboration with D Scheschkewitz, Berlin, Walter de Gruyter, doi:10.1515/9783110578065
- Still B 2016 The Secret Life of the Periodic Table, Cassell, London, ISBN 978-1-84403-885-5
- Stillman JM 1924, The Story of Early Chemistry, D. Appleton, New York
- Stott RWA 1956, Companion to Physical and Inorganic Chemistry, Longmans, Green and Co, London
- Stuke J 1974, "Optical and electrical properties of selenium", in Zingaro RA & Cooper WC (eds.), Selenium, Van Nostrand Reinhold, New York, pp. 174
- Strathern P 2000, Mendeleyev's dream: The Quest for the Elements, Hamish Hamilton, London, ISBN 978-0-8412-1786-7
- Suresh CH & Koga NA 2001, "A consistent approach toward atomic radii”, Journal of Physical Chemistry A, vol. 105, no. 24. doi:10.1021/jp010432b
- Tang et al. 2021, "Synthesis of paracrystalline diamond", Nature, vol. 599, pp. 605–610, doi:10.1038/s41586-021-04122-w
- Taniguchi M, Suga S, Seki M, Sakamoto H, Kanzaki H, Akahama Y, Endo S, Terada S & Narita S 1984, 'Core-exciton induced resonant photoemission in the covalent semiconductor black phosphorus', Solid State Communications, vo1. 49, no. 9, pp. 867–7, doi:10.1016/0038-1098(84)90441-1
- Taylor MD 1960, First Principles of Chemistry, Van Nostrand, Princeton
- The Chemical News and Journal of Physical Science 1864, "Notices of books: Manual of the Metalloids", vol. 9, p. 22
- The Chemical News and Journal of Physical Science 1897, "Notices of books: A Manual of Chemistry, Theoretical and Practical", by WA Tilden", vol. 75, pp. 188–189
- Thompson M 2004, Osmium tetroxide (OsO4), Molecule of the Month, (May), doi:10.6084/m9.figshare.5437084
- Thornton BF & Burdette SC 2010, "Finding eka-iodine: Discovery priority in modern times", Bulletin for the history of chemistry, vol. 35, no. 2, accessed September 14, 2021
- Tidy CM 1887, Handbook of Modern Chemistry, 2nd ed., Smith, Elder & Co., London
- Tregarthen L 2003, Preliminary Chemistry, Macmillan Education: Melbourne, ISBN 978-0-7329-9011-4
- Trenberth KE & Smith L 2005, "The mass of the atmosphere: A constraint on global analyses", Journal of Climate, vol. 18, no. 6, doi:10.1175/JCLI-3299.1
- Tshitoyan et al. 2019, "Unsupervised word embeddings capture latent knowledge from materials science literature", Nature, vol. 571, doi:10.1038/s41586-019-1335-8
- Tyler PM 1948, From the Ground Up: Facts and Figures of the Mineral Industries of the United States, McGraw-Hill, New York
- U.S. Geological Survey 1998, Mineral Commodity Summaries, U.S. Geological Survey, accessed 27 August 2023
- U.S. Geological Survey 2023, Mineral Commodity Summaries, U.S. Geological Survey
- Vasáros L & Berei K 1985, 'General Properties of Astatine', pp. 107–28, in Kugler & Keller
- Vassilakis AA, Kalemos A & Mavridis A 2014, "Accurate first principles calculations on chlorine fluoride ClF and its ions ClF±", Theoretical Chemistry Accounts, vol. 133, no. 1436, doi:10.1007/s00214-013-1436-7
- Vernon R 2013, "Which elements are metalloids?", Journal of Chemical Education, vol. 90, no. 12, 1703‒1707, doi:10.1021/ed3008457
- Vernon R 2020, "Organising the metals and nonmetals", Foundations of Chemistry, vol. 22, doi:10.1007/s10698-020-09356-6 (open access)
- Wächtershäuser G 2014, "From chemical invariance to genetic variability", in Weigand W and Schollhammer P (eds.), Bioinspired Catalysis: Metal Sulfur Complexes, Wiley-VCH, Weinheim, doi:10.1002/9783527664160.ch1
- Wakeman TH 1899, "Free thought—Past, present and future", Free Thought Magazine, vol. 17
- Wasewar KL 2021, "Intensifying approaches for removal of selenium", in Devi et al. (eds.), Selenium contamination in water, John Wiley & Sons, Hoboken, pp. 319–355, ISBN 978-1-119-69354-3
- Weeks ME 1945, Discovery of the Elements, 5th ed., Journal of Chemical Education, Easton, Pennsylvania
- Welcher SH 2001, High marks: Regents Chemistry Made Easy, 2nd ed., High Marks Made Easy, New York, ISBN 978-0-9714662-4-1
- Wells AF 1984, Structural Inorganic Chemistry, 5th ed., Clarendon Press, Oxford, ISBN 978-0-19-855370-0
- White JH 1962, Inorganic Chemistry: Advanced and Scholarship Levels, University of London Press, London
- Wibaut P 1951, Organic Chemistry, Elsevier Publishing Company, New York
- Wiberg N 2001, Inorganic Chemistry, Academic Press, San Diego, ISBN 978-0-12-352651-9
- Williams RPJ 2007, "Life, the environment and our ecosystem", Journal of Inorganic Biochemistry, vol. 101, nos. 11–12, doi:10.1016/j.jinorgbio.2007.07.006
- Woodward et al. 1999, "The electronic structure of metal oxides", In Fierro JLG (ed.), Metal Oxides: Chemistry and Applications, CRC Press, Boca Raton, ISBN 1-4200-2812-X
- Wulfsberg G 1987, Principles of Descriptive Chemistry, Brooks/Cole, Belmont CA, ISBN 978-0-534-07494-4
- Wulfsberg G 2000, Inorganic Chemistry, University Science Books, Sausalito, California, ISBN 978-1-891389-01-6
- Xia G-J, Ning Z-X & Zhu X-M 2020, “Effect of low-frequency oscillation on plasma focusing in krypton hall thruster", Journal of Propulsion and Power, vol. 36, no. 1, pp. Journal of Propulsion and Power, doi:10.2514/1.B37599
- Yamaguchi M & Shirai Y 1996, "Defect structures", in Stoloff NS & Sikka VK (eds.), Physical Metallurgy and Processing of Intermetallic Compounds, Chapman & Hall, New York, ISBN 978-1-4613-1215-4
- Yang J 2004, "Theory of thermal conductivity", in Tritt TM (ed.), Thermal Conductivity: Theory, Properties, and Applications, Kluwer Academic/Plenum Publishers, New York, pp. 1–20, ISBN 978-0-306-48327-1,
- Yoder CH, Suydam FH & Snavely FA 1975, Chemistry, 2nd ed, Harcourt Brace Jovanovich, New York, ISBN 978-0-15-506470-6
- Young JA 2006, "Iodine", Journal of Chemical Education, vol. 83, no. 9, doi:10.1021/ed083p1285
- Young et al. 2018, General Chemistry: Atoms First, Cengage Learning: Boston, ISBN 978-1-337-61229-6
- Zhao J, Tu Z & Chan SH 2021, "Carbon corrosion mechanism and mitigation strategies in a proton exchange membrane fuel cell (PEMFC): A review", Journal of Power Sources, vol. 488, #229434, doi:10.1016/j.jpowsour.2020.229434
- Zhigal'skii GP & Jones BK 2003, The Physical Properties of Thin Metal Films, Taylor & Francis, London, ISBN 978-0-415-28390-8
- Zhu W 2020, Chemical Elements In Life, World Scientific, Singapore, ISBN 978-981-121-032-7
- Zhu et al. 2014, "Reactions of xenon with iron and nickel are predicted in the Earth's inner core", Nature Chemistry, vol. 6, doi:10.1038/nchem.1925, PMID 24950336
- Zumdahl SS & DeCoste DJ 2010, Introductory Chemistry: A Foundation, 7th ed., Cengage Learning, Mason, Ohio, ISBN 978-1-111-29601-8
External links
Media related to Nonmetals at Wikimedia Commons