User:Grimhelm/History of science
Science is a body of empirical, theoretical, and practical knowledge about the natural world, produced by a global community of researchers making use of scientific methods, which emphasize the observation, explanation, and adequate prediction of real world phenomena by experiment. Given the dual status of science as objective knowledge and as a human construct, good historiography of science draws on the historical methods of both intellectual history and social history.
Tracing the exact origins of modern science is possible through the many important texts which have survived from the classical world. However, the word scientist is relatively recent—first coined by William Whewell in the 19th century. Previously, people investigating nature called themselves natural philosophers.
While empirical investigations of the natural world have been described since Ancient Greece (for example, by Thales, Aristotle, and others), and scientific methods have been employed since the Middle Ages (for example, by Ibn al-Haytham, Abū Rayhān al-Bīrūnī and Roger Bacon), the dawn of modern science is generally traced back to the early modern period, during what is known as the Scientific Revolution that took place in 16th and 17th century Europe.
Scientific methods are considered to be so fundamental to modern science that some — especially philosophers of science and practicing scientists — consider earlier inquiries into nature to be pre-scientific. Traditionally, historians of science have defined science sufficiently broadly to include those inquiries.[1]
Historiography and academic study[edit]
As an academic field, history of science began with the publication of William Whewell's History of the Inductive Sciences (first published in 1837). A more formal study of the history of science as an independent discipline was launched by George Sarton's publications, Introduction to the History of Science (published in 1927) and the Isis journal (founded in 1912). The history of mathematics, history of technology, and history of philosophy are distinct areas of research and are covered in other articles. Mathematics is closely related to but distinct from natural science (at least in the modern conception). Technology is likewise closely related to but clearly differs from the search for empirical truth. Philosophy differs from science in its engagement in analysis and normative discourse, among other differences. In practice science, mathematics, technology, and philosophy are obviously deeply entwined, and clear lines demarcating them are not evident until the 19th century (when science first became professionalized). History of science has therefore been deeply informed by the histories of mathematics, technology, and philosophy—even as those fields have become increasingly autonomous.
Theories and sociology of the history of science[edit]
Much of the study of the history of science has been devoted to answering questions about what science is, how it functions, and whether it exhibits large-scale patterns and trends.[2] The sociology of science in particular has focused on the ways in which scientists work, looking closely at the ways in which they "produce" and "construct" scientific knowledge. Since the 1960s, a common trend in science studies (the study of the sociology and history of science) has been to emphasize the "human component" of scientific knowledge, and to de-emphasize the view that scientific data are self-evident, value-free, and context-free.[3]
A major subject of concern and controversy in the philosophy of science has been the nature of theory change in science. Karl Popper argued that scientific knowledge is progressive and cumulative; Thomas Kuhn, that scientific knowledge moves through "paradigm shifts" and is not necessarily progressive; and Paul Feyerabend, that scientific knowledge is not cumulative or progressive and that there can be no demarcation in terms of method between science and any other form of investigation.[4]
Since the publication of Kuhn's The Structure of Scientific Revolutions in 1970,[5] historians, sociologists, and philosophers of science have debated the meaning and objectivity of science.
Historical factors affecting science[edit]
Culture and religion[edit]
Technology, mathematics and proto-science[edit]
Development of science[edit]
Early cultures[edit]
In prehistoric times, advice and knowledge was passed from generation to generation in an oral tradition. The development of writing enabled knowledge to be stored and communicated across generations with much greater fidelity. Combined with the development of agriculture, which allowed for a surplus of food, it became possible for early civilizations to develop, because more time could be devoted to tasks other than survival.
Many ancient civilizations collected astronomical information in a systematic manner through simple observation. Though they had no knowledge of the real physical structure of the planets and stars, many theoretical explanations were proposed. Basic facts about human physiology were known in some places, and alchemy was practiced in several civilizations. Considerable observation of macrobiotic flora and fauna was also performed.
Science in the Fertile Crescent[edit]
From their beginnings in Sumer (now Iraq) around 3500 BC the Mesopotamian peoples began to attempt to record some observations of the world with extremely thorough numerical data. But their observations and measurements were seemingly taken for purposes other than for scientific laws. A concrete instance of Pythagoras' law was recorded, as early as the 18th century BC: the Mesopotamian cuneiform tablet Plimpton 322 records a number of Pythagorean triplets (3,4,5) (5,12,13). ..., dated 1900 BC, possibly millennia before Pythagoras, [2] but an abstract formulation of the Pythagorean theorem was not.[6]
Significant advances in Ancient Egypt include astronomy, mathematics and medicine.[7] Their geometry was a necessary outgrowth of surveying to preserve the layout and ownership of farmland, which was flooded annually by the Nile river. The 3,4,5 right triangle and other rules of thumb served to represent rectilinear structures, and the post and lintel architecture of Egypt. Egypt was also a center of alchemy research for much of the Mediterranean.
Science in the Greco-Roman world[edit]
In Classical Antiquity, the inquiry into the workings of the universe took place both in investigations aimed at such practical goals as establishing a reliable calendar or determining how to cure a variety of illnesses and in those abstract investigations known as natural philosophy. The ancient people who are considered the first scientists may have thought of themselves as natural philosophers, as practitioners of a skilled profession (for example, physicians), or as followers of a religious tradition (for example, temple healers).
The earliest Greek philosophers, known as the pre-Socratics, provided competing answers to the question found in the myths of their neighbors: "How did the ordered cosmos in which we live come to be?"[8] The pre-Socratic philosopher Thales, dubbed the "father of science", was the first to postulate non-supernatural explanations for natural phenomena such as lightning and earthquakes. Pythagoras of Samos founded the Pythagorean school, which investigated mathematics for its own sake, and was the first to postulate that the Earth is spherical in shape. Subsequently, Plato and Aristotle produced the first systematic discussions of natural philosophy, which did much to shape later investigations of nature. Their development of deductive reasoning was of particular importance and usefulness to later scientific inquiry.
The important legacy of this period included substantial advances in factual knowledge, especially in anatomy, zoology, botany, mineralogy, geography, mathematics and astronomy; an awareness of the importance of certain scientific problems, especially those related to the problem of change and its causes; and a recognition of the methodological importance of applying mathematics to natural phenomena and of undertaking empirical research.[9] In the Hellenistic age scholars frequently employed the principles developed in earlier Greek thought: the application of mathematics and deliberate empirical research, in their scientific investigations.[10] Thus, clear unbroken lines of influence lead from ancient Greek and Hellenistic philosophers, to medieval Muslim philosophers and scientists, to the European Renaissance and Enlightenment, to the secular sciences of the modern day. Neither reason nor inquiry began with the Ancient Greeks, but the Socratic method did, along with the idea of Forms, great advances in geometry, logic, and the natural sciences. Benjamin Farrington, former Professor of Classics at Swansea University wrote in 1944:
- "Men were weighing for thousands of years before Archimedes worked out the laws of equilibrium; they must have had practical and intuitional knowledge of the principles involved. What Archimedes did was to sort out the theoretical implications of this practical knowledge and present the resulting body of knowledge as a logically coherent system."
and again:
- "With astonishment we find ourselves on the threshold of modern science. Nor should it be supposed that by some trick of translation the extracts have been given an air of modernity. Far from it. The vocabulary of these writings and their style are the source from which our own vocabulary and style have been derived."[11]
The level of achievement in Hellenistic astronomy and engineering is impressively shown by the Antikythera mechanism (150-100 BC). The astronomer Aristarchus of Samos was the first known person to propose a heliocentric model of the solar system, while the geographer Eratosthenes accurately calculated the circumference of the Earth. Hipparchus (ca. 190 – ca. 120 BC) produced the first systematic star catalog. In medicine, Herophilos (335 - 280 BC) was the first to base his conclusions on dissection of the human body and to describe the nervous system. Hippocrates (ca. 460 BC – ca. 370 BC) and his followers were first to describe many diseases and medical conditions. Galen (129 – ca. 200 AD) performed many audacious operations—including brain and eye surgeries— that were not tried again for almost two millennia. The mathematician Euclid laid down the foundations of mathematical rigor and introduced the concepts of definition, axiom, theorem and proof still in use today in his Elements, considered the most influential textbook ever written.[12] Archimedes, considered one of the greatest mathematicians of all time,[13] is credited with using the method of exhaustion to calculate the area under the arc of a parabola with the summation of an infinite series, and gave a remarkably accurate approximation of Pi.[14] He is also known in physics for laying the foundations of hydrostatics and the explanation of the principle of the lever.
Theophrastus wrote some of the earliest descriptions of plants and animals, establishing the first taxonomy and looking at minerals in terms of their properties such as hardness. Pliny the Elder produced what is one of the largest encyclopedias of the natural world in 77 AD, and must be regarded as the rightful successor to Theophrastus.
For example, he accurately describes the octahedral shape of the diamond, and proceeds to mention that diamond dust is used by engravers to cut and polish other gems owing to its great hardness. His recognition of the importance of crystal shape is a precursor to modern crystallography, while mention of numerous other minerals presages mineralogy. He also recognises that other minerals have characteristic crystal shapes, but in one example, confuses the crystal habit with the work of lapidaries. He was also the first to recognise that amber was a fossilized resin from pine trees because he had seen samples with trapped insects within them.
Science in India[edit]
This article needs additional citations for verification. (August 2008) |
The wootz, crucible and stainless steels were invented in India, and were widely exported, resulting in "Damascus steel" by the year 1000.[15]
The Hindus excel in the manufacture of iron, and in the preparations of those ingredients along with which it is fused to obtain that kind of soft iron which is usually styled Indian steel (Hindiah). They also have workshops wherein are forged the most famous sabres in the world.[16]
Indian astronomer and mathematician Aryabhata (476-550), in his Aryabhatiya (499) and Aryabhata Siddhanta, worked out an accurate heliocentric model of gravitation, including elliptical orbits, the circumference of the earth, and the longitudes of planets around the Sun. He also introduced a number of trigonometric functions (including sine, versine, cosine and inverse sine), trigonometric tables, and techniques and algorithms of algebra. In the 7th century, Brahmagupta recognized gravity as a force of attraction.[17] He also lucidly explained the use of zero as both a placeholder and a decimal digit, along with the Hindu-Arabic numeral system now used universally throughout the world. Arabic translations of the two astronomers' texts were soon available in the Islamic world, introducing what would become Arabic numerals to the Islamic World by the 9th century.[18][19]
The first 12 chapters of the Siddhanta Shiromani, written by Bhāskara in the 12th century, cover topics such as: mean longitudes of the planets; true longitudes of the planets; the three problems of diurnal rotation; syzygies; lunar eclipses; solar eclipses; latitudes of the planets; risings and settings; the moon's crescent; conjunctions of the planets with each other; conjunctions of the planets with the fixed stars; and the patas of the sun and moon. The 13 chapters of the second part cover the nature of the sphere, as well as significant astronomical and trigometric calculations based on it.
During the 14th-16th centuries, the Kerala school of astronomy and mathematics made significant advances in astronomy and especially mathematics, including fields such as trigonometry and calculus. In particular, Madhava of Sangamagrama is considered the "founder of mathematical analysis".[20]
Science in China[edit]
Ancient China had a long and rich history of technological contribution. The so-called "Four Great Inventions of ancient China" were the compass, gunpowder, papermaking and printing, which had an enormous impact on the development of Chinese civilization and far-ranging global effects. According to 16th century English philosopher Francis Bacon, writing in Novum Organum, they brought about so many changes "that no empire, no sect, no star seems to have exerted greater power and influence in human affairs than these mechanical discoveries."[21] According the British scholar Joseph Needham, the technological accomplishments of China included early seismological detectors, the water-powered celestial globe and the double-action piston pump; cast iron, the blast furnace and sliding calipers; natural gas as fuel, a solid fuel rocket and the multistage rocket.[citation needed] In Chinese mathematics, the 13th century Qin Jiushao was the first to introduce the practical concept of the zero symbol.[22] Before this innovation, blank spaces had been used instead in the system of counting rods.[23] Pascal's triangle was first illustrated in China by Yang Hui in his book Xiangjie Jiuzhang Suanfa (详解九章算法), although it was described earlier around 1100 by Jia Xian.[24]

As Toby E. Huff notes, pre-modern Chinese science developed precariously without solid scientific theory, while there was a lacking of consistent systemic treatment in comparison to contemporaneous medieval works such as the 12th century Gratian of Bologna's Concordance and Discordant Canons.[25] This inherent flaw in Chinese scientific discourse was lamented even by the mathematician Yang Hui (1238–1298), who criticised earlier mathematicians such as Li Chunfeng for being content to use methods without working out their theoretical origins or principles.[26]
There were noted advances in traditional Chinese medicine, and Emperor Gaozong of the Tang Dynasty commissioned the scholarly compilation of a materia medica in 657, documenting 833 medicinal substances taken from stones, minerals, metals, plants, herbs, animals, vegetables, fruits, and cereal crops.[27] In his Bencao Tujing ('Illustrated Pharmacopoeia'), the 11th century scholar and statesman Su Song not only systematically categorized herbs and minerals according to their pharmaceutical uses, but also took an interest in zoology.[28][29][30][31] In their pursuit for an elixir of life and desire to create gold from various mixtures of materials, the Chinese Taoists became heavily associated with alchemy.[32] Joseph Needham labeled their pursuits as proto-scientific rather than merely pseudoscience.[32] However, cultural factors prevented these Chinese achievements from developing into what could be called "science".[33] According to Needham, it was the religious and philosophical framework of the Chinese intellectuals which made them unable to believe in the ideas of laws of nature:
It was not that there was no order in nature for the Chinese, but rather that it was not an order ordained by a rational personal being, and hence there was no conviction that rational personal beings would be able to spell out in their lesser earthly languages the divine code of laws which he had decreed aforetime. The Taoists, indeed, would have scorned such an idea as being too naïve for the subtlety and complexity of the universe as they intuited it.[34]
Science in the Middle Ages[edit]
With the division of the Empire, the Western Roman Empire lost contact with much of its past. The Library of Alexandria, which had suffered since it fell under Roman rule,[35] had been destroyed by 642, shortly after the Arab conquest of Egypt.[36][37] While the Byzantine Empire still held learning centers such as Constantinople, Western Europe's knowledge was concentrated in monasteries until the development of medieval universities in the 12th and 13th centuries. The curriculum of monastic schools included the study of the few available ancient texts and of new works on practical subjects like medicine[38] and timekeeping.[39]
Meanwhile, in the Middle East, Greek philosophy was able to find some support under the newly created Arab Empire. With the spread of Islam in the 7th and 8th centuries, a period of Muslim scholarship, known as the Islamic Golden Age, lasted until the 16th century. This scholarship was aided by several factors. The use of a single language, Arabic, allowed communication without need of a translator. Access to Greek and Latin texts from the Byzantine Empire along with Indian sources of learning provided Muslim scholars a knowledge base to build upon.
Science in the Islamic world[edit]

Islamic civilisation made a number of significant mathematical and technological contributions during its "Golden Age". The work of the 9th century Persian mathematician Muhammad ibn Musa al-Khwarizmi gave the name to the concept of both the algorithm and algebra, as well as establishing the basis for trigonometry. Al-Khwarizmi and his contemporaries made several refinements to Indian numerals, such as the introduction of decimal point notation, before "Arabic numerals" were transmitted to Europe in the 12th century. Another contemporary of al-Khwarizmi, the Sabian mathematician Al-Battani, made contributions to both astronomy and trigonometry. In Islamic Iberia, the 11th-century Arab engineer Ibn Khalaf al-Muradi created the first geared clock; a water clock that employed both segmental and epicyclic gearing.[40]
In general, Muslim scientists placed far greater emphasis on experiment than had the Greeks.[41] This led to an early scientific method being developed in the Muslim world, where significant progress in methodology was made, beginning with Ibn al-Haytham's experiments in his Book of Optics (c. 1000).[42] Al-Haytham was "pioneer of the modern scientific method", whose "investigations are based not on abstract theories, but on experimental evidences… [which] were systematic and repeatable."[43] The most important development was the use of experiments to distinguish between competing scientific theories set within a generally empirical orientation.
In astronomy, al-Battani also improved upon the measurements of Hipparchus (preserved in the translation of Ptolemy's Almagest) and of the precession of the Earth's axis. The corrections made to the geocentric model by al-Battani, Ibn al-Haytham,[44] Averroes and the Maragha astronomers such as Nasir al-Din al-Tusi, Mo'ayyeduddin Urdi and Ibn al-Shatir were later incorporated into the Copernican heliocentric model.[45][46] Heliocentric theories may have also been discussed by several other Muslim astronomers such as Ja'far ibn Muhammad Abu Ma'shar al-Balkhi,[47] Abu-Rayhan Biruni, Abu Said al-Sijzi,[48] Qutb al-Din al-Shirazi, and 'Umar al-Katibi al-Qazwini.[49] This

Historians of science differ in their views of Islamic science: specifically as to the relative significance of the Islamic contribution, whether the Islamic world developed what could truly be called "science", and the extent to which the religious framework was a factor. The traditionalist view, as exemplified by Bertrand Russell,[50] holds that Islamic science, while admirable in many technical ways, lacked the intellectual energy required for innovation and was chiefly important as a preserver of ancient knowledge and transmitter to medieval Europe. The revisionist view, as exemplified by Abdus Salam,[51] George Saliba[52] John M. Hobson[53] holds that a Muslim scientific revolution occurred during the Middle Ages;[54][55] scholars such as Donald Routledge Hill and Ahmad Y Hassan express the view that Islam was the driving force behind the Muslim achievements,[56] while Robert Briffault even sees Islamic science as the foundation of modern science.[41] Others, such as Stanley Jaki and Thomas Woods, maintain that medieval Orthodox Islamic scholars "absolutely rejected any conception of the universe that involved consistent physical laws", which would have restricted the omnipotence and "absolute autonomy" of Allah, and that "Muslim scientists typically occurred in spite of Islam rather than because of it".[57] The most prominent view in recent scholarship, however, as exemplified by Toby E. Huff,[58][59] Will Durant,[60] Fielding H. Garrison,[61] Muhammad Iqbal[62] Hossein Nasr and Bernard Lewis,[63] holds that Muslim scientists did help in laying the foundations for an experimental science with their contributions to the scientific method and their empirical, experimental and quantitative approach to scientific inquiry, but that their work cannot be considered a scientific revolution, with the exception of Ibn al-Haytham's Book of Optics which is widely considered a revolution in the fields of optics and visual perception.[64][65][66][67][68][69]
Science in Medieval Europe[edit]

In line with earlier Greek thought, the 4th century bishop and theologian, St. Augustine of Hippo, wrote that the creation story in Genesis should not be read too literally. In his book De Genesi ad literam, he stated that he believed that in some cases new creatures had come about through the "decomposition" of earlier forms of life.[70] For Augustine, "plant, fowl and animal life are not perfect… but created in a state of potentiality", unlike what he considered the theologically perfect forms of angels, the firmament and the human soul, and had evolved "slowly over time" — an idea which suggests that he had put forward an early form of evolution.[71][72][73] According to Stephen Hawking, Augustine was also the first to point out that time was a property of the universe, and that it had no meaning prior to the beginning of the universe.[74] Significant technological advances were also made in medieval Europe, particularly in the monasteries, as with the first clock built by Pope Sylvester II in 996, the early flight of the 11th century Eilmer of Malmesbury and the metallurgical expertise of the Cistercian Order.
From at least the 11th century, European intellectual discourse evolved within the context of Scholasticism: the medieval philosophical framework commited to the indespensible use of Reason and the Dialectic.[75] As the tradition matured, Scholastic treatises commonly followed a set pattern of posing a question, weighing both sides of the argument, giving the author's opinion and refuting objections.[75] The intellectually rigorous Cathedral School of Chartres became involved in "defining the basic concept of the cosmos from which all later specialized sciences were to grow; [and] systematically reconstructing the scientific knowledge of the past".[76] The creation of the first universities from the great Cathedral schools in the 12th century allowed for the flowering of formal and sustained scientific inquiry[77] — so that in the following century Pope Alexander IV could describe them as "rivers of science which water and make fertile the soil of the universal Church".[78]
Meanwhile, contact with the Islamic world in Spain and Sicily afforded access to the scientific texts and commentaries of Greek and Muslim authors.[79] European translation programmes such as the 12th century Toledo School of Translators provided the translation of Arabic texts into Latin, while in Italy Latin translations could be made directly from the original Greek.[79] The Latin scholars discovered that there were notable contradictions between Catholic doctrine and Aristotellian philosophy.[79] St. Thomas Aquinas, a prominent theologian and admirer of Aristotle, resolved this supposed conflict between faith and science by stating that any apparent contradictions that arose indicated flaws in the understanding either of religion or philosophy.[80]
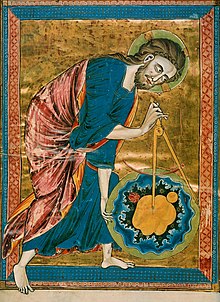
The de-animation of nature, a contribution of Christian thought from as early as the Patristic period, was another essential precondition for the emergence of science.[81] In particular, Thierry of Chartres rejected the notion that the universe was an organism or somehow divine and not subject to natural laws,[82] while the 14th century Jean Buridan developed the theory of impetus to overcome the deficiencies of Aristotelian mechanics, showing that the same laws governed celestial and terrestrial motion.[83] In the 13th century, the Chancellor of Oxford and Bishop of Lincoln, Robert Grosseteste, became the first man to write down a complete set of steps for conducting a scientific experiment;[84] while a contemporaneous teacher at Oxford, the Franciscan Roger Bacon, is considered a forerunner of the modern scientific method.[85] Influenced by his reading of Arabic writers[86] and widely admired for his work in mathematics and optics,[85] Bacon emphasised experience and experiment in his Opus Maius: "Without experiment, nothing can be adequately known. An argument proves theoretically, but does not give the certitude necessary to remove all doubt."[85] Another Franciscan, William of Ockham, introduced the principle of parsimony (also known as Ockham's razor), which developed a new form of logic based on an empiricist theory of knowledge — "seen as a forerunner of a modern age of analysis".[87]
The work of Pierre Duhem opened up the history of medieval science as a serious academic discipline.[88] Duhem believed that Bishop Stephen Tempier's Condemnation of 1277, which condemned certain Aristotelian teachings at Paris as "errors", liberated Christian thought from the dogmatic acceptance of Aristotelianism and in doing so marked the birth of science.[88] Although scholars have disagreed over the relative influence of the Condemnations, historians of science recognise their effects as positive.[89] The accelerating trend has been to underline the crucial role of the Church; particularly the Catholic view of God as an orderly, rational being, who "ordered all things by measure, number, weight" (Wisdom 11:21), as indespensible for the emergence of scientific thought.[90] In the Christian milieu, Scholastic thinkers saw the universe as "rational, predictable and intelligible", and that experience and mathematics, particularly geometry, were the vehicles for rendering that universe comprehensible.[91]
Western science in the modern era[edit]
Renaissance and Early modern science[edit]

The intellectual revitalisation of Europe that began with the Renaissance of the 12th century went into decline after the trauma of the Black Death in the 1340s. In the following centuries, the scientific life of Europe came close to a standstill; it has been said that with the exception of Nicholas Copernicus' theory of heliocentrism, the history of Western science from 1350 to 1600 is one of relative stagnation.[92] The Northern Renaissance showed a decisive shift in focus from Aristoteleian natural philosophy to chemistry and the biological sciences (botany, anatomy, and medicine).[93] However, while bibliophilia lay at the heart of the Renaissance,[94] the period was also a time of irrationalism, in which Europe experienced an "occult revival" and alchemy and astrology reached their height.[92][95] However, although alchemists interpreted their results using astrology, cabbalistic number theory and herbalism, it is still possible that they were the "true predecessor of the later scientific community"; "cosmopolitan" alchemists were particularly active at the court of Rudolph II, where many of them held "responsible positions".[96]
The "Scientific Revolution" is generally held to have taken place between the mid-16th and mid-17th centuries; although its precepts did not necessarily accord with the prevailing ideas and practices, and it was still the age of the alchemists, astrologers and magicians.[97] The revolution began with the astronomical observations of Copernicus, a Dominican cleric and famed astronomer in his day.[97][98] Copernicus tested his hypothesis by detailed experiment and measurement, fully supporting his De Revolutionibus with statistical data.[99] In the early 17th century, the Italian physicist Galileo Galilei made further observations that undermined the Ptolemaic system and supported Copernicanism, including the discovery of the moons of Jupiter and the phases of Venus.[100] In 1610, Galileo's work was welcomed by prominent churchmen, scholars and scientist-priests alike, including Pope Paul V, Christopher Clavius and Christoph Grienberger.[101] Although initially permitted to teach Copernicanism as an elegant theoretical model, Galileo's later insistence on Copernicanism as literal truth without conclusive evidence resulted in his subjection to Church censure.[102]
The Scientific Revolution culminated in the work of Isaac Newton, the President of the Royal Society in London, who published his Philosophiæ Naturalis Principia Mathematica in 1687.[103] Although Newton himself dabbled in alchemy,[104][105] his Laws of Motion and Gravity provided the basis of physics for the next two centuries.[103] Other significant scientific advances were made by Edmond Halley, Robert Hooke, Christiaan Huygens, Tycho Brahe, Johannes Kepler, Gottfried Leibniz, and Blaise Pascal. The scientific method was also better developed as the modern way of thinking and emphasised experimentation and reason over traditional considerations.
The 17th century "Age of Reason" opened the avenues to the decisive steps towards modern science, which took place during the 18th century "Age of Enlightenment". Directly based on the works [citation needed] of Newton, Descartes, Pascal and Leibniz, the way was now clear to the development of modern mathematics, physics and technology by the generation of Benjamin Franklin (1706–1790), Leonhard Euler (1707–1783), Georges-Louis Leclerc (1707–1788) and Jean le Rond d'Alembert (1717–1783), epitomized in the appearance of Denis Diderot's Encyclopédie between 1751 and 1772. The impact of this process was not limited to science and technology, but affected philosophy (Immanuel Kant, David Hume), religion (notably with the appearance of positive atheism, and the increasingly significant impact of science upon religion), and society and politics in general (Adam Smith, Voltaire), the French Revolution of 1789 setting a bloody cesura indicating the beginning of political modernity[citation needed].
Late modern science[edit]
The Scientific Revolution established science as the preeminent source for the growth of knowledge.[citation needed] During the 19th century, the practice of science became professionalized and institutionalized in ways which continued through the 20th century. As the role of scientific knowledge grew in society, it became incorporated with many aspects of the functioning of nation-states.
The history of science is marked by a chain of advances in technology and knowledge that have always complemented each other. Technological innovations bring about new discoveries and are bred by other discoveries which inspire new possibilities and approaches to longstanding science issues.
Emergence of independent fields in the natural sciences[edit]
Astronomy[edit]

Astronomy is one of the oldest independent branches of the sciences. Aristarchus of Samos published work on how to determine the sizes and distances of the Sun and the Moon, and Eratosthenes used this work to figure the size of the Earth. Hipparchus later discovered the precession of the Earth.
In 1543, Nicolaus Copernicus published his De Revolutionibus as a general outline of astronomy. Copernican astronomy shared the ideas of perfectly spherical heavenly bodies, circular orbits and constant planetary speed with its Greek precursors, but it differed from the geocentric universe of Ptolemy with its modern heliocentric model.[106] Galileo's own observations improved upon Copernicanism; his observation of mountains on the moon undermined the ancient conception of heavenly bodies as perfect spheres.[107] In the 17th century, Johannes Kepler introduced his laws of planetary motion and his theory of elliptical orbits. This latter theory had the advantage of simplicity over the competing models of Ptolemy, Copernicus and Tycho Brahe, and was confirmed by the observations of the Italian astronomer Giovanni Cassini.[108]
The astronomical community during the Scientific Revolution was also closely linked to the scientist-priests of the Jesuit Order, who provided correspondence, verification of results and financial assistance to their fellow astronomers.[109] The Jesuit polymath Roger Boscovich published widely on sunspots, the Aurora Borealis and the motion of the heavenly bodies — in particular, he developed the first geometric method for calculating a planet's orbit based on three observations of its position.[110] Until improvements in observational instruments in the 18th century, cathedrals across Europe served as the main observatories; between 1655 and 1736, for example, astronomers such as Cassini were able to make some 4500 observations at the Basilica of San Petronio in Bologna.[111]

Advances in astronomy and in optical systems in the 19th century resulted in the first observation of an asteroid (1 Ceres) in 1801, and the discovery of Neptune in 1846.
George Gamow, Ralph Alpher, and Robert Hermann had calculated that there should be evidence for a Big Bang in the background temperature of the universe.[112] In 1964, Arno Penzias and Robert Wilson[113] discovered a 3 kelvin background hiss in their Bell Labs radiotelescope, which was evidence for this hypothesis, and formed the basis for a number of results that helped determine the age of the universe.
Supernova SN1987A was observed by astronomers on Earth both visually, and in a triumph for neutrino astronomy, by the solar neutrino detectors at Kamiokande. But the solar neutrino flux was a fraction of its theoretically-expected value. This discrepancy forced a change in some values in the standard model for particle physics.
Physics[edit]
Galileo Galilei's Two New Sciences formed the basis of modern physics. Galileo made use of experiments to validate physical theories, a key element of the scientific method.
In 1687, Isaac Newton published the Principia Mathematica, detailing two comprehensive and successful physical theories: Newton's laws of motion, which led to classical mechanics; and Newton's Law of Gravitation, which describes the fundamental force of gravity. Newton's Laws of Motion and Gravity provided the basis of physics for the next two centuries.[103]
In 1758, Roger Boscovich's Theory of Natural Philosophy put forward "the first coherent description of an atomic theory", anticipated the aims and many of the features of 20th century atomic physics, and qualitatively predicted the prenetrability of matter by high-speed particles and the possibility of states of matter of exceptionally high density.[114] As the creator of fundamental atomic physics, Boscovich's work was greatly admired by Michael Faraday, Dmitri Mendeleev, Clerk Maxwell and Lord Kelvin.[115]
The behaviour of electricity and magnetism was studied by Faraday, Ohm, and others during the early 19th century. These studies led to the unification of the two phenomena into a single theory of electromagnetism, by Maxwell (known as Maxwell's equations).
A revolution in physics began at the start of the 20th century, in which the paradigms of Newton's classical mechanics came into question. From 1900 on, Max Planck, Albert Einstein, Niels Bohr and others developed quantum theories to explain various anomalous experimental results, by introducing discrete energy levels. Not only did quantum mechanics show that the laws of motion did not hold on small scales, but Einstein's theory of general relativity in 1915 showed that the fixed background of spacetime, on which both Newtonian mechanics and special relativity depended, could not exist. In 1925, Werner Heisenberg and Erwin Schrödinger formulated quantum mechanics, which explained the preceding quantum theories. Edwin Hubble's observation in 1929, that the speed at which galaxies recede positively correlates with their distance, led to the understanding that the universe is expanding, and the formulation of the Big Bang theory by Georges Lemaître.
Further developments took place during World War II, which led to the practical application of radar and the development and use of the atomic bomb. Though the process had begun with the invention of the cyclotron by Ernest O. Lawrence in the 1930s, physics in the postwar period entered into a phase of what historians have called "Big Science", requiring massive machines, budgets, and laboratories in order to test their theories and move into new frontiers. State governments became the primary patron of physics, based on the recognition that supporing "basic" research could often lead to technologies with military and industrial applications. Currently, efforts are underway to unify the mutually inconsistent theories of general relativity and quantum mechanics.
Chemistry[edit]
In the Islamic world, the 9th century "father of chemistry" Geber[116][117][118][119] introduced a systematic and experimental approach to scientific research based in the laboratory, in contrast to the ancient Greek and Egyptian alchemists whose works were often allegorical and unintelligble.[120] He also invented and named the alembic (al-anbiq), chemically analysed many chemical substances, composed lapidaries, distinguished between alkalis and acids, and manufactured hundreds of drugs.[121] Ja'far al-Sadiq,[122] Alkindus,[123] Abū al-Rayhān al-Bīrūnī,[124] Avicenna[125] and Ibn Khaldun refuted the practice of alchemy and the theory of the transmutation of metals; and Tusi described an early version of the conservation of mass, noting the ability of matter to change but not to disappear.[126] Rhazes was the first to refute Aristotle's theory of four classical elements, as well as designing and describing more than twenty scientific instruments.[127]
In the 17th century, the work of the Jesuit polymath Athanasius Kircher helped to debunk alchemy.[128]
Robert Boyle,[129] Antoine Lavoisier, [129] Jöns Berzelius[130][131] and John Dalton[129] have traditionally been considered the "founders" chemistry. Boyle, an Anglo-Irish scientist, first introduced the distinction between chemistry and alchemy in The Sceptical Chymist (1661). This distinction was reinforced by the gravimetric experimental practices of medical chemists such as William Cullen, Joseph Black, Torbern Bergman and Pierre Macquer. In the 18th century, other fundamental steps were taken by Joseph Priestly and Henry Cavendish, who explored the compound natures of air and water respectively.[132] Priestly's experiments disproved the reigning theory of the "Phlogiston", an invisible form of matter accepted by most scientists of the period,[133] at around the same time that Roger Boscovich introduced his atomic theory.[114] Above all, Lavoisier discovered the workings of chemical reactions, when he produced the compound mercuric oxide in his experiments to measure how much oxygen was absorbed when quicksilver was burned in a closed flask.[134] He also coined the names of the elements "hydrogen" and "oxygen", helped produce a list of elements with their new nomenclature and published the first chemical textbook in 1789.[135] It was the Swede Berzelius who provided the modern technique of chemical formula notation.

John Dalton worked out his own atomic theory in 1803, although the question took a century to settle as proven, and formulated the law of mass relationships. By the 1860s, 60 of the 90 elements that occur in nature were known.[136] In 1869, Dmitri Mendeleev composed his Periodic Table of elements based on his provisional Periodic Law: that when arranged in order of atomic weight, the elements "present a clear periodicity of properties".[136] This allowed the Russian theoretician to predict accurately the atomic weights and properties of six undiscovered elements in advance of the empirical research.[136]
The synthesis of urea by Friedrich Wöhler in 1828 opened up organic chemistry as a new field of research, while William Henry Perkin's discovery of the synthetic dye mauveine in 1856 led modern chemistry into the production of synthetic and semi-synthetic materials.[137] A profusion of synthetic dyes and artificial colours became possible — by the late 20th century the number of synthetic dyes commercially produced in Europe exceeded 4000[137] — so that chemistry became known as "the creative science". Further innovations led to the first semi-synthetic material (celluloid, 1862), the first synthetic drugs (such as asprin, 1899), the isolation and eventual synthesis of hormones (insulin, 1921) and the first synthetic antibiotic (chloramphenicol, 1950).[137] Meanwhile, the application of organic chemistry to living organisms resulted in physiological chemistry, the precursor to biochemistry, while the 20th century saw the integration of physics and chemistry, with chemical properties explained as the result of the electronic structure of the atom. Linus Pauling's The Nature of the Chemical Bond used the principles of quantum mechanics to deduce bond angles in ever-more complex molecules. Pauling's work culminated in the physical modelling of DNA in 1953. In a simulation of primordial processes that same year, the Miller-Urey experiment demonstrated that amino acids, the base constituents of proteins, could themselves be built up from simpler molecules.
Geology[edit]
Geology existed as a cloud of isolated, disconnected ideas about rocks, minerals, and landforms long before it became a coherent science. Theophrastus' work on rocks Peri lithōn remained authoritative for millennia: its interpretation of fossils was not overturned until after the Scientific Revolution. Chinese polymath Shen Kua (1031 - 1095) was the first to formulate hypotheses for the process of land formation. Based on his observation of fossils in a geological stratum in a mountain hundreds of miles from the ocean, he deduced that the land was formed by erosion of the mountains and by deposition of silt.

Geology was not systematically restructured during the Scientific Revolution, but individual theorists made important contributions. Robert Hooke, for example, formulated theory of earthquakes, and Nicholas Steno developed the theory of superposition and argued that fossils were the remains of once-living creatures. Beginning with Thomas Burnet's Sacred Theory of the Earth in 1681, natural philosophers began to explore the idea that the Earth had changed over time. Burnet and his contemporaries interpreted Earth's past in terms of events described in the Bible, but their work laid the intellectual foundations for secular interpretations of Earth history.
Modern geology, like modern chemistry, gradually evolved during the 1700s and early 1800s. Benoît de Maillet and the Comte de Buffon argued that Earth was much older than the 6,000 years envisioned by biblical scholars. Jean-Étienne Guettard and Nicolas Desmarest hiked central France and recorded their observations on some of the first geological maps. Abraham Werner created a systematic classification scheme for rocks and minerals—an achievement as significant for geology as that of Linnaeus was for biology. Werner also proposed a generalized interpretation of Earth history, as did contemporary Scottish polymath James Hutton. Georges Cuvier and Alexandre Brongniart, expanding on the work of Steno, argued that layers of rock could be dated by the fossils they contained: a principle first applied to the geology of the Paris Basin. The use of index fossils became a powerful tool for making geological maps, because it allowed geologists to correlate the rocks in one locality with those of similar age in other, distant localities. Over the first half of the 19th century, geologists such as Charles Lyell, Adam Sedgwick, and Roderick Murchison applied the new technique to rocks throughout Europe and eastern North America, setting the stage for more detailed, government-funded mapping projects in later decades.
Midway through the 19th century, the focus of geology shifted from description and classification to attempts to understand how the surface of the Earth changed. The first comprehensive theories of mountain building were proposed during this period, as were the first modern theories of earthquakes and volcanoes. Louis Agassiz and others established the reality of continent-covering ice ages, and "fluvialists" like Andrew Crombie Ramsay argued that river valleys were formed, over millions of years by the rivers that flow through them. After the discovery of radioactivity, radiometric dating methods were developed, starting in the 1900s. Alfred Wegener's theory of "continental drift" was widely dismissed when it was proposed in the 1910s, but new data gathered in the 1950s and 1960s led to the theory of plate tectonics, which provided a plausible mechanism for it. Plate tectonics also provided a unified explanation for a wide range of seemingly unrelated geological phenomena. Since 1970 it has been the unifying principle in geology.
Geologists' embrace of plate tectonics was part of a broadening of the field from a study of rocks into a study of the Earth as a planet. Other elements of this transformation include: geophysical studies of the interior of the Earth, the grouping of geology with meteorology and oceanography as one of the "earth sciences", and comparisons of Earth and the solar system's other rocky planets.
Biology, medicine, and genetics[edit]
In 1847, Hungarian physician Ignác Fülöp Semmelweis dramatically reduced the occurrency of puerperal fever by simply requiring physicians to wash their hands before attending to women in childbirth. This discovery predated the germ theory of disease. However, Semmelweis' findings were not appreciated by his contemporaries and came into use only with discoveries by British surgeon Joseph Lister, who in 1865 proved the principles of antisepsis. Lister's work was based on the important findings by French biologist Louis Pasteur. Pasteur was able to link microorganisms with disease, revolutionizing medicine. He also devised one of the most important methods in preventive medicine, when in 1880 he produced a vaccine against rabies. Pasteur invented the process of pasteurization, to help prevent the spread of disease through milk and other foods.[138]
Perhaps the most prominent and far-reaching theory in all of science has been the theory of evolution by natural selection put forward by the British naturalist Charles Darwin in his book On the Origin of Species in 1859. Darwin proposed that the features of all living things, including humans, were shaped by natural processes over long periods of time. Implications of evolution on fields outside of pure science have led to both opposition and support from different parts of society, and profoundly influenced the popular understanding of "man's place in the universe".
In the early 20th century, the study of heredity became a major investigation after the rediscovery in 1900 of the laws of inheritance developed by the Moravian monk Gregor Mendel in 1866. Mendel's laws provided the beginnings of the study of genetics, which became a major field of research for both scientific and industrial research. By 1953, James D. Watson, Francis Crick and Rosalind Franklin clarified the basic structure of DNA, the genetic material for expressing life in all its forms.[139] In the late 20th century, the possibilities of genetic engineering became practical for the first time, and a massive international effort began in 1990 to map out an entire human genome (the Human Genome Project) has been touted as potentially having large medical benefits.
Ecology[edit]
The discipline of ecology typically traces its origin to the synthesis of Darwinian evolution and Humboldtian biogeography, in the late 19th and early 20th centuries. Equally important in the rise of ecology, however, were microbiology and soil science—particularly the cycle of life concept, prominent in the work Louis Pasteur and Ferdinand Cohn. The word ecology was coined by Ernst Haeckel, whose particularly holistic view of nature in general (and Darwin's theory in particular) was important in the spread of ecological thinking. In the 1930s, Arthur Tansley and others began developing the field of ecosystem ecology, which combined experimental soil science with physiological concepts of energy and the techniques of field biology. The history of ecology in the 20th century is closely tied to that of environmentalism; the Gaia hypothesis in the 1960s and more recently the scientific-religious movement of Deep Ecology have brought the two closer together.
Emergence of independent fields in the social sciences[edit]
Successful use of the scientific method in the physical sciences led to the same methodology being adapted to better understand the many fields of human endeavor. From this effort the social sciences have been developed.
Sociology[edit]
Ibn Khaldun can be regarded as the earliest scientific systematic sociologist.[140] The modern sociology, emerged in the early 19th century as the academic response to the modernization of the world. Among many early sociologists (e.g., Émile Durkheim), the aim of sociology was in structuralism, understanding the cohesion of social groups, and developing an "antidote" to social disintegration. Max Weber was concerned with the modernization of society through the concept of rationalization, which he believed would trap individuals in an "iron cage" of rational thought. Some sociologists, including Georg Simmel and W. E. B. Du Bois, utilized more microsociological, qualitative analyses. This microlevel approach played an important role in American sociology, with the theories of George Herbert Mead and his student Herbert Blumer resulting in the creation of the symbolic interactionism approach to sociology.
American sociology in the 1940s and 1950s was dominated largely by Talcott Parsons, who argued that aspects of society that promoted structural integration were therefore "functional". This structural functionalism approach was questioned in the 1960s, when sociologists came to see this approach as merely a justification for inequalities present in the status quo. In reaction, conflict theory was developed, which was based in part on the philosophies of Karl Marx. Conflict theorists saw society as an arena in which different groups compete for control over resources. Symbolic interactionism also came to be regarded as central to sociological thinking. Erving Goffman saw social interactions as a stage performance, with individuals preparing "backstage" and attempting to control their audience through impression management. While these theories are currently prominent in sociological thought, other approaches exist, including feminist theory, post-structuralism, rational choice theory, and postmodernism.
Political science[edit]

In ancient Indian literature, the most studied work on political science is the Arthaśāstra of the 3rd century BC: a treatise on statecraft, economic policy and military strategy. Roger Boesche describes the Arthaśāstra as "a book of political realism, a book analysing how the political world does work and not very often stating how it ought to work, a book that frequently discloses to a king what calculating and sometimes brutal measures he must carry out to preserve the state and the common good."[141]
In Western civilisation, political science has a clear set of antecedents in moral philosophy, political philosophy and economy, history, and other fields concerned with normative determinations of what ought to be and with deducing the characteristics and functions of the ideal form of government. The ancient Greeks were the first to study politics in a philosophical context: beginning with the works of Homer, Hesiod, Thucydides, Xenophon, and Euripides; and culminating in the works of the Socratics, Plato and Aristotle. Politically, Classical Greece was organised in small city-states, which were run by a tight oligarchy of privileged, landowning citizens and "fluctuated between outright tyrannies and democracies".[142] However, being of aristocratic birth gave the philosophy of Plato and Aristotle a heavy statist and elitist bias, which permeated the later political philosophy of Greece and Rome and also influenced medieval Christian thought.[143]
The fall of the Roman Empire and the rise of monotheism, in the respective Christian and Islamic worlds, brought to light a new space for politics and political action. The study of politics was widespread in medieval Europe, and works such as St. Augustine's The City of God synthesised philosophies and traditions with those of Christianity, redefining the boundaries between religion and politics. Many of the political questions surrounding the relationship between Church and State were clarified and contested in this period. In the Middle East, works such as the Rubaiyat of Omar Khayyam and Epic of Kings by Ferdowsi provided evidence of political analysis, while the Islamic aristotelians such as Avicenna and later Maimonides and Averroes, continued Aristotle's tradition of analysis and empiricism, writing commentaries on Aristotle's works.
During the Italian Renaissance, Niccolò Machiavelli established the emphasis of modern political science on direct empirical observation of political institutions and actors. Later, the expansion of the scientific paradigm during the Enlightenment further pushed the study of politics beyond normative determinations. In particular, the use of statistics to study the subjects of the state has been applied to polling and voting. In the 20th century, the study of ideology, behaviouralism and international relations led to a multitude of 'pol-sci' subdisciplines including rational choice theory, voting theory, game theory (also used in economics), psephology, political geography/geopolitics, political psychology/political sociology, political economy, policy analysis, public administration, comparative political analysis and peace studies/conflict analysis. At the beginning of the 21st century, political scientists have increasingly deployed deductive modelling and systematic empirical verification techniques (quantitative methods) brining their discipline closer to the "hard" scientific mainstream.
Economics[edit]

As a social science, the history of economics represents one of the best examples of the Kuhnian 'paradigm shift'.[144] As Murray Rothbard wrote, economics "has proceeded in contentious, even zig-zag fashion, with later systemic fallacy sometimes elbowing aside earlier but sounder paradigms."[145] Economic inquiry begins with the ancient Greeks,[146] and the Boeotian didactic poet Hesiod could be called the "first Greek economic thinker".[147] Unlike the later followers of Plato and Aristotle, whose oligarchic biases gave them "a dim view of economic innovation and entrepreneurship", Hesiod's Works and Days was closely familiar with vigorous labour and healthy competition as a way of dealing with the problems of scarcity.[148] Other early empirical contributions to economic science include Democritus' discovery of the subjective theory of value and time preference; Plato and Xenophon's insights into the division of labour; and Aristotle's discussion of monetary exchange and supply and demand.[149] In ancient China, Taoists such as Lao Tzu, Ssu-ma Ch'ien and Chuang Tzu were the first to advocate laissez faire and to recognise debasement of coinage as a cause of inflation.[150]
According to Joseph Schumpeter, the medieval Scholastics came nearer than "any other group to having been the 'founders' of scientific economics" — a view developed by Raymond de Roover, Marjorie Grice-Hutchinson and John T. Noonan.[151][152] Culminating in the late Spanish Scholastic School of Salamanca, the Scholastics had a sophisticated subjective utility theory of value and price, and have been seen as the forerunners of the much later Austrian School.[153] Generally, the mainstream Scholastic view recognised the current market price as the "just price".[154]Note In the 14th century, John Buridan showed the spontaneous emergence of money from commodity goods as a medium of exchange, while his student Nicholas Oresme, the Bishop of Lisieux and founder of monetary economics, outlined Gresham's Law and the destructive effects of inflation.[155] Among the late Scholastics, Thomas Cardinal Cajetan founded Expectations Theory by outlining the impact of market expectations on the value of money.[156]
After the Scholastics, numerous fallacies were introduced into economics: the mercantilists were characterised by a faulty set of economic theories, including protectionism, bullionism and the "Montaigne fallacy" — the idea that in a trade one man can only benefit at the expense of another.[157] Daniel Bernoulli founded mathematical economics in 1738, but in doing so introduced a flawed and pernicious mathematical form of the Law of Diminishing Marginal Utility.[158] Although Adam Smith has traditionally been seen as the father of economics, a reassessment has shown that the first treatise on economics, the Essai sur La nature du commerce en general (c. 1730), was penned by the Irish international banker Richard Cantillon almost fifty years before Smith's The Wealth of Nations (1776);[159] while Sir James Steuart introduced the term "political economy" with his Principles of Political Oeconomy (1767).[160] Adam Smith, who founded the "the paradigm of the British classical school",[161] is now seen instead as a synthesiser and systematiser of economics,[160] who advocated division of labour and postulated that an "Invisible Hand" would guide individual self-interest toward the public good.[162] However, he also introduced the incorrect labour theory of value, which was developed by David Ricardo and Karl Marx and fed into Marxian economics.[163][164][165]
Beginning with Carl Menger's Principles of Economics (1871), Marxian economics was critiqued by the Austrian school, which contributed the Marginal utility theory, the Austrian business cycle theory and Opportunity cost.[166][167] John Maynard Keynes developed modern macroeconomics with his General Theory of Employment, Interest and Money (1936),[168] and argued that aggregate demand determines the level of production and employment — central to Keynesian analysis.[169] Following World War II, Milton Friedman made a methodological defence of classical economics, although his main work was on the development and empirical testing of the Quantity theory of money.[170] Other modern schools of economic thought are New Classical economics, developed in the 1970s, and New Keynesian economics, created partially in response to New Classical economics. New Classical economics emphasises solid microeconomics as the basis for macroeconomic growth, while New Keynesians deal with how inefficiencies in the market create a need for control by a central bank or government.
Anthropology[edit]
Anthropology can best be understood as an outgrowth of the Age of Enlightenment. It was during this period that Europeans attempted systematically to study human behaviour. Traditions of jurisprudence, history, philology and sociology developed during this time and informed the development of the social sciences of which anthropology was a part.
At the same time, the romantic reaction to the Enlightenment produced thinkers such as Johann Gottfried Herder and later Wilhelm Dilthey whose work formed the basis for the culture concept which is central to the discipline. Traditionally, much of the history of the subject was based on colonial encounters between Europe and the rest of the world, and much of 18th- and 19th-century anthropology is now classed as forms of scientific racism.
During the late 19th-century, battles over the "study of man" took place between those of an "anthropological" persuasion (relying on anthropometrical techniques) and those of an "ethnological" persuasion (looking at cultures and traditions), and these distinctions became part of the later divide between physical anthropology and cultural anthropology, the latter ushered in by the students of Franz Boas.
In the mid-20th century, much of the methodologies of earlier anthropological and ethnographical study were reevaluated with an eye towards research ethics, while at the same time the scope of investigation has broadened far beyond the traditional study of "primitive cultures" (scientific practice itself is often an arena of anthropological study).
The emergence of paleoanthropology, a scientific discipline which draws on the methodologies of paleontology, physical anthropology and ethology, among other disciplines, and increasing in scope and momentum from the mid-20th century, continues to yield further insights into human origins, evolution, genetic and cultural heritage, and perspectives on the contemporary human predicament as well.
Linguistics[edit]
Historical linguistics emerged as an independent field of study at the end of the 18th century. Sir William Jones proposed that Sanskrit, Persian, Greek, Latin, Gothic, and Celtic languages all shared a common base. After Jones, an effort to catalog all languages of the world was made throughout the 19th century and into the 20th century. Publication of Ferdinand de Saussure's Cours de linguistique générale spawned the development of descriptive linguistics. Descriptive linguistics, and the related structuralism movement caused linguistics to focus on how language changes over time, instead of just describing the differences between languages. Noam Chomsky further diversified linguistics with the development of generative linguistics in the 1950s. His effort is based upon a mathematical model of language that allows for the description and prediction of valid syntax. Additional specialties such as sociolinguistics, cognitive linguistics, and computational linguistics have emerged from collaboration between linguistics and other disciplines.
Emergence of independent fields in the social sciences[edit]
Psychology[edit]
The end of the 19th century marks the start of psychology as a scientific enterprise. The year 1879 is commonly seen as the start of psychology as an independent field of study. In that year Wilhelm Wundt founded the first laboratory dedicated exclusively to psychological research (in Leipzig). Other important early contributors to the field include Hermann Ebbinghaus (a pioneer in memory studies), Ivan Pavlov (who discovered classical conditioning), and Sigmund Freud. Freud's influence has been enormous, though more as cultural icon than a force in scientific psychology.
The 20th century saw a rejection of Freud's theories as being too unscientific, and a reaction against Edward Titchener's atomistic approach of the mind. This led to the formulation of behaviorism by John B. Watson, which was popularized by B.F. Skinner. Behaviorism proposed epistemologically limiting psychological study to overt behavior, since that could be reliably measured. Scientific knowledge of the "mind" was considered too metaphysical, hence impossible to achieve.
The final decades of the 20th century have seen the rise of a new interdisciplinary approach to studying human psychology, known collectively as cognitive science. Cognitive science again considers the mind as a subject for investigation, using the tools of evolutionary psychology, linguistics, computer science, philosophy, and neurobiology. New methods of visualizing the activity of the brain, such as PET scans and CAT scans, began to exert its influence as well. These new forms of investigation assume that a wide understanding of the human mind is possible, and that such an understanding may be applied to other research domains, such as artificial intelligence.
Emerging disciplines[edit]
During the 20th century, a number of interdisciplinary scientific fields have emerged. Three examples will be given here:
Communication studies combines animal communication, information theory, marketing, public relations, telecommunications and other forms of communication.
Computer science, built upon a foundation of theoretical linguistics, discrete mathematics, and electrical engineering, studies the nature and limits of computation. Subfields include computability, computational complexity, database design, computer networking, artificial intelligence, and the design of computer hardware. One area in which advances in computing have contributed to more general scientific development is by facilitating large-scale archiving of scientific data. Contemporary computer science typically distinguishes itself by emphasising mathematical 'theory' in contrast to the practical emphasis of software engineering.
Materials science has its roots in metallurgy, minerology, and crystallography. It combines chemistry, physics, and several engineering disciplines. The field studies metals, ceramics, plastics, semiconductors, and composite materials.
See also[edit]
Notes[edit]
- ^ W. C. Dampier Wetham, Science, in Encyclopædia Britannica, 11th ed. (New York: Encyclopedia Britannica, Inc, 1911); M. Clagett, Greek Science in Antiquity (New York: Collier Books, 1955); D. Pingree, Hellenophilia versus the History of Science, Isis 83, 559 (1982); Pat Munday, entry "History of Science", New Dictionary of the History of Ideas (Charles Scribner's Sons, 2005).
- ^ What is this thing called science?
- ^ The Sociology of Science: Theoretical and Empirical Investigations By Robert King Merton
- ^ Science Teaching: The Role of History and Philosophy of Science By Michael Robert Matthews
- ^ Summary on Google books
- ^ Paul Hoffman, The man who loved only numbers: the story of Paul Erdös and the search for mathematical truth, (New York: Hyperion), 1998, p.187. ISBN 0-7868-6362-5
- ^ Homer's Odyssey stated that "the Egyptians were skilled in medicine more than any other art". [1]
- ^ F. M. Cornford, Principium Sapientiae: The Origins of Greek Philosophical Thought, (Gloucester, Mass., Peter Smith, 1971), p. 159.
- ^ G. E. R. Lloyd, Early Greek Science: Thales to Aristotle, (New York: W. W. Norton, 1970), pp. 144-6.
- ^ Lloyd (1973), p. 177.
- ^ Greek Science, many editions, such as the paperback by Penguin Books. Copyrights in 1944, 1949, 1953, 1961, 1963. The first quote above comes from Part 1, Chapter 1; the second, from Part 2, Chapter 4.
- ^ Boyer (1991). "Euclid of Alexandria". p. 119.
The Elements of Euclid not only was the earliest major Greek mathematical work to come down to us, but also the most influential textbook of all times. [...]The first printed versions of the Elements appeared at Venice in 1482, one of the very earliest of mathematical books to be set in type; it has been estimated that since then at least a thousand editions have been published. Perhaps no book other than the Bible can boast so many editions, and certainly no mathematical work has had an influence comparable with that of Euclid's Elements.
{{cite book}}
: Missing or empty|title=
(help) - ^ Calinger, Ronald (1999). A Contextual History of Mathematics. Prentice-Hall. p. 150. ISBN 0-02-318285-7.
Shortly after Euclid, compiler of the definitive textbook, came Archimedes of Syracuse (ca. 287–212 B.C.), the most original and profound mathematician of antiquity.
- ^ O'Connor, J.J. and Robertson, E.F. (1996). "A history of calculus". University of St Andrews. Retrieved 2007-08-07.
{{cite web}}
: Unknown parameter|month=
ignored (help)CS1 maint: multiple names: authors list (link) - ^ C. S. Smith, A History of Metallography, University Press, Chicago (1960); Juleff 1996; Srinivasan, Sharda and Srinivasa Rangnathan 2004
- ^ Henry Yule quoted the 12th century Arab Edrizi.
- Srinivasan, Sharda and Srinivasa Rangnathan. 2004. India’s Legendary Wootz Steel. Bangalore: Tata Steel. 2004
- ^ Mainak Kumar Bose, Late Classical India, A. Mukherjee & Co., 1988, p. 277.
- ^ Ifrah, Georges. 1999. The Universal History of Numbers : From Prehistory to the Invention of the Computer, Wiley. ISBN 0-471-37568-3.
- ^ O'Connor, J.J. and E.F. Robertson. 2000. 'Indian Numerals', MacTutor History of Mathematics Archive, School of Mathematics and Statistics, University of St. Andrews, Scotland.
- ^ George G. Joseph (1991). The crest of the peacock. London.
- ^ (Novum Organum, Liber I, CXXIX - Adapted from the 1863 translation)
- ^ Joseph Needham, Science and Civilization in China: Volume 3, Mathematics and the Sciences of the Heavens and the Earth (Taipei: Caves Books, Ltd., 1986) pp. 43.
- ^ Joseph Needham, Science and Civilization in China: Volume 3, Mathematics and the Sciences of the Heavens and the Earth (Taipei: Caves Books, Ltd., 1986) pp. 62–3.
- ^ Needham, Science and Civilization in China: Volume 3, Mathematics and the Sciences of the Heavens and the Earth (Taipei: Caves Books, Ltd., 1986) pp. 134–7.
- ^ Toby E. Huff, The Rise of Early Modern Science: Islam, China, and the West (Cambridge: Cambridge University Press, 2003, ISBN 0521529948) pp 303
- ^ Joseph Needham, Science and Civilization in China: Volume 3, Mathematics and the Sciences of the Heavens and the Earth (Taipei: Caves Books, Ltd., 1986) pp. 104.
- ^ Charles Benn, China's Golden Age: Everyday Life in the Tang Dynasty. Oxford University Press, 2002, ISBN 0-19-517665-0), pp. 235.
- ^ Wu Jing-nuan, An Illustrated Chinese Materia Medica. (New York: Oxford University Press, 2005), pp. 5.
- ^ Joseph Needham, Science and Civilization in China: Volume 3, Mathematics and the Sciences of the Heavens and the Earth (Taipei: Caves Books, Ltd., 1986) pp. 648–9.
- ^ Joseph Needham, Science and Civilization in China: Volume 6, Biology and Biological Technology, Part 1, Botany. (Taipei: Caves Books Ltd., 1986), pp. 174–5.
- ^ Schafer, Edward H. "Orpiment and Realgar in Chinese Technology and Tradition," Journal of the American Oriental Society (Volume 75, Number 2, 1955): 73–89.
- ^ a b John King Fairbank and Merle Goldman, China: A New History (Cambridge: MA; London: The Belknap Press of Harvard University Press, 2nd ed., 2006, ISBN 0-674-01828-1), pp. 81.
- ^ Cite error: The named reference
Woods
was invoked but never defined (see the help page). - ^ Joseph Needham, Science and Civilization in China, volume 1. Cambridge University Press, 1954. 581.
- ^ Plutarch, Life of Caesar 49.3.
- ^ Abd-el-latif (1203): "the library which 'Amr ibn al-'As burnt with the permission of 'Umar."
- ^ Europe: A History, p 139. Oxford: Oxford University Press 1996. ISBN 0-19-820171-0
- ^ Linda E. Voigts, "Anglo-Saxon Plant Remedies and the Anglo-Saxons", Isis, 70 (1979): 250-268; reprinted in Michael H. Shank, The Scientific Enterprise in Antiquity and the Middle Ages, Chicago: Univ. of Chicago Pr., 2000, pp. 163-181. ISBN 0-226-74951-7.
- ^ Faith Wallis, Bede: The Reckoning of Time, Liverpool: Liverpool Univ. Pr., 2004, pp. xviii-xxxiv. ISBN 0-85323-693-3.
- ^ Hassan, Ahmad Y, Transfer Of Islamic Technology To The West, Part II: Transmission Of Islamic Engineering, History of Science and Technology in Islam
- ^ a b Cite error: The named reference
Briffault
was invoked but never defined (see the help page). - ^ David Agar (2001). Arabic Studies in Physics and Astronomy During 800 - 1400 AD. University of Jyväskylä.
- ^ Rosanna Gorini (2003). "Al-Haytham the Man of Experience. First Steps in the Science of Vision", International Society for the History of Islamic Medicine. Institute of Neurosciences, Laboratory of Psychobiology and Psychopharmacology, Rome, Italy.
- ^ Rosen, Edward (1985), "The Dissolution of the Solid Celestial Spheres", Journal of the History of Ideas, 46 (1): 19-20 & 21
- ^ "Nicolaus Copernicus", [[Stanford Encyclopedia of Philosophy]], 2004, retrieved 2008-01-22
{{citation}}
: URL–wikilink conflict (help) - ^ Saliba, George (1994), A History of Arabic Astronomy: Planetary Theories During the Golden Age of Islam, New York University Press, pp. 254 & 256-257, ISBN 0814780237
- ^ Bartel Leendert van der Waerden (1987). "The Heliocentric System in Greek, Persian and Hindu Astronomy", Annals of the New York Academy of Sciences 500 (1), 525–545 [534-537].
- ^ Nasr, Seyyed H. (1st edition in 1964, 2nd edition in 1993), An Introduction to Islamic Cosmological Doctrines (2nd ed.), 1st edition by Harvard University Press, 2nd edition by State University of New York Press, pp. 135–136, ISBN 0791415155
{{citation}}
: Check date values in:|date=
(help)CS1 maint: date and year (link) - ^ Baker, A.; Chapter, L. (2002), "Part 4: The Sciences",
{{citation}}
: Missing or empty|title=
(help), in Sharif, M. M., "A History of Muslim Philosophy", Philosophia Islamica - ^ Bertrand Russell (1945), History of Western Philosophy, book 2, part 2, chapter X
- ^ Abdus Salam, H. R. Dalafi, Mohamed Hassan (1994). Renaissance of Sciences in Islamic Countries, p. 162. World Scientific, ISBN 9971507137.
- ^ (Saliba 1994, pp. 245, 250, 256–257)
- ^ Hobson 2004, p. 178
- ^ Abid Ullah Jan (2006), After Fascism: Muslims and the struggle for self-determination, "Islam, the West, and the Question of Dominance", Pragmatic Publishings, ISBN 978-0-9733687-5-8.
- ^ Salah Zaimeche (2003), An Introduction to Muslim Science, FSTC.
- ^ Ahmad Y Hassan and Donald Routledge Hill (1986), Islamic Technology: An Illustrated History, p. 282, Cambridge University Press
- ^ Woods, p 79
- ^ (Huff 2003)
- ^ Saliba, George (Autumn 1999), "Seeking the Origins of Modern Science? Review of Toby E. Huff, The Rise of Early Modern Science: Islam, China and the West", Bulletin of the Royal Institute for Inter-Faith Studies, 1 (2), retrieved 2008-04-10
- ^ Cite error: The named reference
Durant
was invoked but never defined (see the help page). - ^ Cite error: The named reference
Garrison
was invoked but never defined (see the help page). - ^ Cite error: The named reference
Iqbal
was invoked but never defined (see the help page). - ^ Edmund, Norman W. (2005), End the Biggest Educational and Intellectual Blunder in History: A $100,000 Challenge to Our Top Educational Leaders, Scientific Method Publishing, p. 447, ISBN 0963286668
- ^ Cite error: The named reference
Hogendijk
was invoked but never defined (see the help page). - ^ Cite error: The named reference
Hatfield
was invoked but never defined (see the help page). - ^ Simon, Gérard (2006), "The Gaze in Ibn al-Haytham", The Medieval History Journal, 9 (1): 89–98, doi:10.1177/097194580500900105
- ^ Bellosta, Hélèna (2002), "Burning Instruments: From Diocles to Ibn Sahl", Arabic Sciences and Philosophy, 12, Cambridge University Press: 285–303, doi:10.1017/S095742390200214X
- ^ Rashed, Roshdi (2 August 2002), "Portraits of Science: A Polymath in the 10th Century", Science, 297 (5582): 773, doi:10.1126/science.1074591
- ^ Lindberg, David C. (1967), "Alhazen's Theory of Vision and Its Reception in the West", Isis, 58 (3): 321–341 [332], doi:10.1086/350266
- ^ Augustine 1982 pp. 89-90
- ^ Gill 2005 p.251
- ^ "Vatican buries the hatchet with Charles Darwin". Times Online. 2009-02-11. Retrieved 2009-02-12.
- ^ "The Vatican claims Darwin's theory of evolution is compatible with Christianity". Telegraph.co.uk. 2009-02-12. Retrieved 2009-02-12.
- ^ Hawking, p 9
- ^ a b Woods, p 58
- ^ Thomas Goldstein (historian of science); quoted by Woods, p 88
- ^ Woods, p 47-48, 75
- ^ Woods, p 65
- ^ a b c Woods, p 89
- ^ Woods, p 90-91
- ^ Woods, p 93
- ^ Woods, p 88
- ^ Woods, p 81-85
- ^ Woods, p 95-96
- ^ a b c Woods, p 94
- ^ Lindberg, David C. (1967), "Alhazen's Theory of Vision and Its Reception in the West", Isis, 58 (3): 321–341, doi:10.1086/350266
- ^ Lindberg, p 109
- ^ a b "Condemnation of 1277" from the Stanford Encyclopedia of Philosophy.
- ^ Woods, p 91-92
- ^ Woods, p 75-76
- ^ Woods, p 80, 120
- ^ a b Woods, p 126
- ^ Allen Debus, Man and Nature in the Renaissance, (Cambridge: Cambridge Univ. Pr., 1978).
- ^ Davies, p 522
- ^ Davies, p 530
- ^ Davies, p 530-531
- ^ a b Davies, p 507
- ^ Woods, p 68
- ^ Davies, p 507-508
- ^ Woods, p 69
- ^ Woods, p 69-70
- ^ Woods, p 70-74
- ^ a b c Davies, p 598
- ^ Davies, p 599
- ^ Woods, p 108-109
- ^ Woods, p 68-69
- ^ Woods, p 69
- ^ Woods, p 111
- ^ Woods, p 110-111
- ^ Woods, p 106
- ^ Woods, p 112-113
- ^ Alpher, Herman, and Gamow. Nature 162,774 (1948).
- ^ Wilson's 1978 Nobel lecture
- ^ a b Woods, p 107
- ^ Woods, p 107-108
- ^ Derewenda, Zygmunt S. (2007), "On wine, chirality and crystallography", Acta Crystallographica Section A: Foundations of Crystallography, 64: 246–258 [247], doi:10.1107/S0108767307054293
- ^ John Warren (2005). "War and the Cultural Heritage of Iraq: a sadly mismanaged affair", Third World Quarterly, Volume 26, Issue 4 & 5, p. 815-830.
- ^ Dr. A. Zahoor (1997), JABIR IBN HAIYAN (Jabir), University of Indonesia
- ^ Paul Vallely, How Islamic inventors changed the world, The Independent
- ^ Kraus, Paul, Jâbir ibn Hayyân, Contribution à l'histoire des idées scientifiques dans l'Islam. I. Le corpus des écrits jâbiriens. II. Jâbir et la science grecque,. Cairo (1942-1943). Repr. By Fuat Sezgin, (Natural Sciences in Islam. 67-68), Frankfurt. 2002
- ^ Will Durant (1980). The Age of Faith (The Story of Civilization, Volume 4), p. 162-186. Simon & Schuster. ISBN 0671012002.
- ^ Research Committee of Strasburg University, Imam Jafar Ibn Muhammad As-Sadiq A.S. The Great Muslim Scientist and Philosopher, translated by Kaukab Ali Mirza, 2000. Willowdale Ont. ISBN 0969949014.
- ^ Felix Klein-Frank (2001), "Al-Kindi", in Oliver Leaman & Hossein Nasr, History of Islamic Philosophy, p. 174. London: Routledge.
- ^ Michael E. Marmura (1965). "An Introduction to Islamic Cosmological Doctrines. Conceptions of Nature and Methods Used for Its Study by the Ikhwan Al-Safa'an, Al-Biruni, and Ibn Sina by Seyyed Hossein Nasr", Speculum 40 (4), p. 744-746.
- ^ Robert Briffault (1938). The Making of Humanity, p. 196-197.
- ^ Farid Alakbarov (Summer 2001). A 13th-Century Darwin? Tusi's Views on Evolution, Azerbaijan International 9 (2).
- ^ G. Stolyarov II (2002), "Rhazes: The Thinking Western Physician", The Rational Argumentator, Issue VI.
- ^ Woods, p 108-109
- ^ a b c Kim, Mi Gyung (2003). Affinity , That Elusive Dream - A Genealogy of the Chemical Revolution (Epilogue: A Tale of Three Fathers). ISBN 0-262-11273-6.
- ^ Berzelius, Jöns (1779–1848) - Eric Weisstein's World of Scientific Biography
- ^ Jons Jacob - Encyclopædia Britannica Online, 2 Aug 2007
- ^ Davies, p 599
- ^ Davies, p 642
- ^ Davies, p 599, 642
- ^ Davies, p 642
- ^ a b c Davies, p 791
- ^ a b c Davies, p 772-773
- ^ Campbell, Neil A. (2006). Biology: Exploring Life. Boston, Massachusetts: Pearson Prentice Hall. ISBN 0-13-250882-6. OCLC 75299209.
{{cite book}}
: Unknown parameter|coauthors=
ignored (|author=
suggested) (help) - ^ James D. Watson and Francis H. Crick. "Letters to Nature: Molecular structure of Nucleic Acid." Nature 171, 737–738 (1953).
- ^ Muhammed Abdullah Enan, Ibn Khaldun: His Life and Works, The Other Press, 2007, pp. 104–105. ISBN 9839541536.
- ^ Boesche, Roger (2002). The First Great Political Realist: Kautilya and His Arthashastra. Lanham: Lexington Books. p. 17. ISBN 0-7391-0401-2.
- ^ Rothbard, p 6
- ^ Rothbard, p 7
- ^ Rothbard, p ix-x
- ^ Rothbard, p x
- ^ Rothbard, p 3
- ^ Rothbard, p 8
- ^ Rothbard, p 8-9
- ^ Rothbard, p 19
- ^ Rothbard, p 23-24, 27
- ^ Rothbard, p x
- ^ Woods, p 153-154
- ^ Rothbard, p x-xi
- ^ Rothbard, p 60
- ^ Woods, p 154-156
- ^ 100-101
- ^ Rothbard, p 203, 213
- ^ Rothbard, p 379-381
- ^ Rothbard, p 345, 347, 435
- ^ a b Rothbard, p 437
- ^ Rothbard, 436
- ^ Rothbard, p 441, 464-465
- ^ Rothbard, p 453
- ^ Woods, p 163
- ^ Bannock, p 278 ,357, 376, 414
- ^ Woods, p 8
- ^ Bannock, p 19
- ^ Bannock, p 264
- ^ Bannock, p 15
- ^ Bannock, p 177
References[edit]
- Bertrand Russell (1945). A History of Western Philosophy: And Its Connection with Political and Social Circumstances from the Earliest Times to the Present Day. New York: Simon and Schuster.
- Caroline L. Herzenberg. 1986. Women Scientists from Antiquity to the Present Locust Hill Press ISBN 0-933951-01-9
- Deepak Kumar (2006). Science and the Raj: A Study of British India, 2nd edition. Oxford University Press. ISBN 0-19-568003-0
- George Rousseau and Roy Porter, eds., The Ferment of Knowledge: Studies in the Historiography of Science (Cambridge: Cambridge University Press, 1980). ISBN 0-521-22599-X
- Howard Margolis (2002). It Started with Copernicus. New York: McGraw-Hill. ISBN 0-07-138507-X
- Imre Lakatos History of Science and its Rational Reconstructions published in The Methodology of Scientific Research Programmes: Philosophical Papers Volume 1. Cambridge: Cambridge University Press 1978
- John L. Heilbron, ed., The Oxford companion to the history of modern science (New York: Oxford University Press, 2003).
- Joseph Agassi (2007) Science and Its History: A Reassessment of the Historiography of Science (Boston Studies in the Philosophy of Science, 253) Springer. ISBN 1-4020-5631-1, 2008.
- Joseph Needham. Science and Civilisation in China. Multiple volumes (1954–2004).
- Nina Byers and Gary Williams, ed. (2006) Out of the Shadows: Contributions of 20th Century Women to Physics, Cambridge University Press ISBN 0-5218-2197-1 Parameter error in {{ISBN}}: checksum
- Rothbard, Murray N. (2006). [[An Austrian Perspective on the History of Economic Thought|Economic thought before Adam Smith: An Austrian Perspective on the History of Economic Thought]] (PDF). Cheltnam, UK: Edward Elgar. ISBN 094546648X.
{{cite book}}
: Cite has empty unknown parameter:|1=
(help); URL–wikilink conflict (help)
- Thomas S. Kuhn (1996). The Structure of Scientific Revolutions (3rd ed.). University of Chicago Press. ISBN 0-226-45807-5
- Thorndike, Lynn (1923–1958). A History of Magic and Experimental Science. New York: Macmillan.
{{cite book}}
:|format=
requires|url=
(help)
- Boorstin, Daniel (1983). The Discoverers : A History of Man's Search to Know His World and Himself. New York: Random House. ISBN 0394402294. OCLC 9645583.
External links[edit]

- The official website of the International Academy of the History of Science
- The official website of the Division of History of Science and Technology of the International Union of History and Philosophy of Science
- A History of Science, Vols 1–4, online text
- Contributions of 20th century Women to Physics ("CWP")
- History of Science Society ("HSS")
- The CNRS History of Science and Technology Research Center in Paris (France). This center develop differents websites about history of science & tech. : Ampère and history of electricity, Lamarck : works and heritage, Buffon Online, etc. and, recently, the netvibes portal History Of Science In France
- The official site of the Nobel Foundation. Features biographies and info on Nobel laureates
- The Institute and Museum of the History of Science in Florence, Italy
- The Vega Science Trust Free to view videos of scientists including Feynman, Perutz, Rotblat, Born and many Nobel Laureates.
- Toward the Scientific Revolution From MIT OpenCourseWare, class materials for the history of science up to and including Isaac Newton.
- Orotava Foundation for the History of Science, Canary Islands, Spain The History of Science in Spain. Free contents (books, lectures and expositions) on History of Science and digital library.