Astronomy in the medieval Islamic world: Difference between revisions
re-formatted web references |
m →Beginning of experimental astronomy: udpated access date |
||
Line 106: | Line 106: | ||
In the eleventh century, [[Abū Rayhān al-Bīrūnī]] introduced the [[Scientific method|experimental method]] into astronomy and was the first to conduct elaborate [[experiment]]s related to astronomical phenomena. He discovered the [[Milky Way]] [[galaxy]] to be a collection of numerous [[Nebula|nebulous]] [[star]]s. In [[Afghanistan]], he observed and described the [[solar eclipse]] on [[April 8]], [[1019]], and the [[lunar eclipse]] on [[September 17]], [[1019]], in detail, and gave the exact [[latitude]]s of the stars during the lunar eclipse.<ref name=Zahoor>{{cite web|author=Dr. A. Zahoor|year=1997|url=http://www.unhas.ac.id/~rhiza/saintis/biruni.html|title=Abu Raihan Muhammad al-Biruni|publisher=[[Hasanuddin University]]|archiveurl= |
In the eleventh century, [[Abū Rayhān al-Bīrūnī]] introduced the [[Scientific method|experimental method]] into astronomy and was the first to conduct elaborate [[experiment]]s related to astronomical phenomena. He discovered the [[Milky Way]] [[galaxy]] to be a collection of numerous [[Nebula|nebulous]] [[star]]s. In [[Afghanistan]], he observed and described the [[solar eclipse]] on [[April 8]], [[1019]], and the [[lunar eclipse]] on [[September 17]], [[1019]], in detail, and gave the exact [[latitude]]s of the stars during the lunar eclipse.<ref name=Zahoor>{{cite web|author=Dr. A. Zahoor|year=1997|url=http://www.unhas.ac.id/~rhiza/saintis/biruni.html|title=Abu Raihan Muhammad al-Biruni|publisher=[[Hasanuddin University]]|archiveurl= |
||
http://209.85.135.104/search?sourceid=navclient-ff&ie=UTF-8&q=cache%3Ahttp%3A%2F%2Fwww.unhas.ac.id%2F~rhiza%2Fsaintis%2Fbiruni.html |
http://209.85.135.104/search?sourceid=navclient-ff&ie=UTF-8&q=cache%3Ahttp%3A%2F%2Fwww.unhas.ac.id%2F~rhiza%2Fsaintis%2Fbiruni.html |
||
|accessdate=2008-01- |
archivedate=2008-01-18|accessdate=2008-01-18}}</ref> |
||
This led to the use of exacting [[empirical]] observations and experimental techniques by Muslim astronomers from the eleventh century onwards.<ref>{{Harv|Huff|2003|p=326}}</ref> |
This led to the use of exacting [[empirical]] observations and experimental techniques by Muslim astronomers from the eleventh century onwards.<ref>{{Harv|Huff|2003|p=326}}</ref> |
Revision as of 20:19, 22 January 2008
- This is a sub-article of Islamic science and Astronomy.
In the history of astronomy, Islamic astronomy or Arabic astronomy refers to the astronomical developments made in the Islamic world, especially during the Islamic Golden Age (8th-15th centuries), mostly written in the Arabic language and which mostly took place in the Middle East, North Africa, Islamic Spain and Central Asia. It closely parallels the genesis of other Islamic sciences in its assimilation of foreign material and the amalgamation of the disparate elements of that material to create a science that was essentially Islamic. These included Indian, Sassanid and Hellenistic works in particular, which were translated and built upon.[1]
A significant number of stars in the sky, such as Aldebaran and Altair, and astronomical terms such as alhidade, azimuth, and almucantar, are still today recognized with their Arabic names.[2]
A large corpus of literature from Islamic astronomy remains today, numbering approximately 10,000 manuscripts scattered throughout the world, many of which have not been read or cataloged. Even so, a reasonably accurate picture of Islamic activity in the field of astronomy can be reconstructed.[3]
History
Pre-Islamic Arabian knowledge of stars was empirical; their knowledge was what they observed regarding the rising and setting of stars. The rise of Islam is claimed to have provoked increased Arab thought in this field.[4] The foundations of Islamic astronomy closely parallels the genesis of other Islamic sciences in its assimilation of foreign material and the amalgamation of the disparate elements of that material to create a science that was essentially Islamic. These include Indian, Sassanid and Hellenistic works which were translated and built upon.
The science historian Donald Routledge Hill has divided the history of Islamic astronomy into the four following distinct time periods in its history:[4]
- Assimilation and syncretisation of earlier Hellenistic, Indian and Sassanid astronomy (700—825 AD)
- Vigorous investigation, and acceptance and modification to the Ptolemaic system (825—1025 AD)
- Flourishing of a distinctive Islamic system of astronomy (1025—1450 AD)
- Stagnation, where few significant contributions were made (1450—1900 AD)
610-700
There are several cosmological verses in the Qur'an (610-632) which some modern writers have interpreted as foreshadowing the expansion of the universe and possibly even the Big Bang theory:[5]
Don't those who reject faith see that the heavens and the earth were a single entity then We ripped them apart?
— [21:30]
And the heavens We did create with Our Hands, and We do cause it to expand.
— [51:47]
Several hadiths attributed to Muhammad also show that he was generally opposed to astrology as well as superstition in general. An example of this is when an eclipse occurred during his son Ibrahim's death, and rumours began spreading about this being God's personal condolence. Muhammad is said to have replied:[6]
"An eclipse is a phenomenon of nature. It is foolish to attribute such things to the death or birth of a human being."
700-825
The period of assimilation and syncretisation of earlier Hellenistic, Indian and Sassanid astronomy occurred during the eighth and early ninth centuries.
Impetus
Historians point out several factors that fostered the growth of Islamic astronomy. The first was the proximity of the Muslim world to the world of ancient learning. Much of the ancient Greek, Sanskrit and Middle Persian texts were translated into Arabic during the ninth century. This process was enhanced by the tolerance towards scholars of other religions.[1]
Another impetus came from Islamic religious observances, which presented a host of problems in mathematical astronomy. In solving these religious problems the Islamic scholars went far beyond the Greek mathematical methods.[1]
Early Islamic cosmology
In the early eighth century, prior to the translation movement, Ja'far al-Sadiq refuted the geocentric model of the universe common at the time, in which the Earth is stationary, and the Sun, Moon and the planets are orbiting around it. He was the first to refute Ptolemy's theory of the sun having two movements, one going around the Earth in one year and the other going round the earth in 24 hours causing day and night. Al-Sadiq argued that if the Sun is moving round the Earth for one year, it cannot suddenly change its course and go round the Earth for one day. He suggested that this could be explained with a heliocentric theory in which the Earth rotates on its axis and around the Sun. Al-Sadiq also wrote a theory on how the universe is expanding and contracting. He also stated that every object in the universe is always in motion, including objects which appear to be inanimate.[7]
Al-Sadiq's student, Geber (Jabir ibn Hayyan), asked him the following question on the stars:
"How does the movement of the stars keep them from falling?"[7]
Al-Sadiq replied:
"Put a stone in a sling and swing it round your head. The stone will stay in the sling so long as you are rotating it. But as soon as you stop the rotation, the stone will fall down on the ground. In the same way the perpetual motion of stars keeps them from falling down."[7]
Ancient influences and translation movement
During this period, a number of Sanskrit and Middle Persian texts were first translated into Arabic. The most notable of the texts was Zij al-Sindhind,[8] based on the Surya Siddhanta and the works of Brahmagupta, and translated by Muhammad al-Fazari and Yaqūb ibn Tāriq in 777. Sources indicate that the text was translated after an Indian astronomer visited the court of Caliph Al-Mansur in 770. The most notable Middle Persian text translated was the Zij al-Shah, a collection of astronomical tables compiled in Sassanid Persia over two centuries.
Fragments of text during this period indicate that Arabs adopted the sine function (inherited from Indian trigonometry) instead of the chords of arc used in Hellenistic mathematics.[4] Another Indian influence was an approximate formula used for timekeeping by Muslim astronomers.[9]
Islamic interest in astronomy ran parallel to the interest in mathematics. Especially noteworthy in this regard was the Almagest (c. 150) of the Egyptian astronomer Ptolemy (c. 100-178). The Almagest was a landmark work in its field, assembling, as Euclid's Elements had previously done with geometrical works, all extant knowledge in the field of astronomy that was known to the author. This work was originally known as The Mathematical Composition, but after it had come to be used as a text in astronomy, it was called The Great Astronomer. The Islamic world called it The Greatest prefixing the Greek work megiste (greatest) with the article al- and it has since been known to the world as Al-megiste or, after popular use in Western translation, Almagest.[10] though much of the Almagest was incorrect, even in premise, it remained a standard astronomical text in both the Islamic world and Europe until the work of the Maragha school and Nicolaus Copernicus.[11] Ptolemy also produced other works, such as Optics, Harmonica, and some suggest he also wrote Tetrabiblon.
The Almagest was a particularly unifying work for its exhaustive lists of sidereal phenomena. He drew up a list of chronological tables of Assyrian, Persian, Greek, and Roman kings for use in reckoning the lapse of time between known astronomical events and fixed dates. In addition to its relevance to calculating accurate calendars, it linked far and foreign cultures together by a common interest in the stars and astrology. The work of Ptolemy was replicated and refined over the years under Arab, Persian and other Muslim astronomers and astrologers.
825-1025
The period throughout the ninth, tenth and early eleventh centuries was one of vigorous investigation, in which the superiority of the Ptolemaic system of astronomy was accepted and significant contributions made to it. Astronomical research was greatly supported by the Abbasid caliph al-Mamun. Baghdad and Damascus became the centers of such activity. The caliphs not only supported this work financially, but endowed the work with formal prestige.[12]
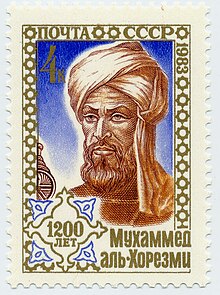
Observational astronomy
The first major original Muslim work of astronomy was Zij al-Sindh by al-Khwarizimi in 830. The work contains tables for the movements of the sun, the moon and the five planets known at the time. The work is significant as it introduced Indian and Ptolemaic concepts into Islamic sciences. This work also marked the turning point in Islamic astronomy. Hitherto, Muslim astronomers had adopted a primarily research approach to the field, translating works of others and learning already discovered knowledge. Al-Khwarizmi's work marked the beginning of non-traditional methods of study and calculations.[13]
In 850, al-Farghani wrote Kitab fi Jawani ("A compendium of the science of stars"). The book primarily gave a summary of Ptolemic cosmography. However, it also corrected Ptolemy's Almagest based on findings of earlier Arab astronomers. Al-Farghani gave revised values for the obliquity of the ecliptic, the precessional movement of the apogees of the sun and the moon, and the circumference of the earth. The books were widely circulated through the Muslim world, and even translated into Latin.[14]
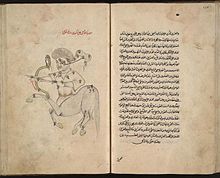
Muhammad ibn Jābir al-Harrānī al-Battānī (Albatenius) (853-929) discovered that the direction of the Sun's eccentric was changing, which in modern astronomy is equivalent to the Earth moving in an elliptical orbit around the Sun.[15] His times for the new moon, lengths for the solar year and sidereal year, prediction of eclipses, and work on the phenomenon of parallax, carried astronomers "to the verge of relativity and the space age."[16] Around the same time, Yahya Ibn Abi Mansour carried out extensive observations and tests, and wrote the Al-Zij al-Mumtahan, in which he completely revised the Almagest values.[17]
In the 10th century, al-Sufi (Azophi) carried out observations on the stars and described their positions, magnitudes, brightness, and colour, and drawings for each constellation in his Book of Fixed Stars. Ibn Yunus observed more than 10,000 entries for the sun's position for many years using a large astrolabe with a diameter of nearly 1.4 metres. His observations on eclipses were still used centuries later in Simon Newcomb's investigations on the motion of the moon, while his other observations inspired Laplace's Obliquity of the Ecliptic and Inequalities of Jupiter and Saturn's.[18]
Abu-Mahmud al-Khujandi relatively accurately computed the axial tilt to be 23°32'19" (23.53°),[19] which was a significant improvement over the Greek and Indian estimates of 23°51'20" (23.86°) and 24°,[20] and still very close to the modern measurement of 23°26' (23.44°).
Early heliocentric models
In the late ninth century, Ja'far ibn Muhammad Abu Ma'shar al-Balkhi developed a planetary model which some have interpreted as a heliocentric model. This is due to his orbital revolutions of the planets being given as heliocentric revolutions rather than geocentric revolutions, and the only known planetary theory in which this occurs is in the heliocentric theory. His work on planetary theory has not survived, but his astronomical data was later recorded by al-Hashimi, Abū Rayhān al-Bīrūnī and al-Sijzi.[21]
In the tenth century, the Brethren of Purity published the Encyclopedia of the Brethren of Purity, in which a heliocentric view of the universe is expressed in a section on cosmology:[22]
"God has placed the Sun at the center of the Universe just as the capital of a country is placed in its middle and the ruler's palace at the center of the city."
In the early eleventh century, al-Biruni had met several Indian scholars who believed in a heliocentric system. In his Indica, he discusses the theories on the Earth's rotation supported by Brahmagupta and other Indian astronomers, while in his Canon Masudicus, al-Biruni writes that Aryabhata's followers assigned the first movement from east to west to the Earth and a second movement from west to east to the fixed stars. Al-Biruni also wrote that al-Sijzi also believed the Earth was moving and invented an astrolabe called the "Zuraqi" based on this idea:[23]
"I have seen the astrolabe called Zuraqi invented by Abu Sa'id Sijzi. I liked it very much and praised him a great deal, as it is based on the idea entertained by some to the effect that the motion we see is due to the Earth's movement and not to that of the sky. By my life, it is a problem difficult of solution and refutation. [...] For it is the same whether you take it that the Earth is in motion or the sky. For, in both cases, it does not affect the Astronomical Science. It is just for the physicist to see if it is possible to refute it."
In his Indica, al-Biruni briefly refers to his work on the refutation of heliocentrism, the Key of Astronomy, which is now lost:[23]
"The most prominent of both modern and ancient astronomers have deeply studied the question of the moving earth, and tried to refute it. We, too, have composed a book on the subject called Miftah 'ilm al-hai'ah (Key of Astronomy), in which we think we have surpassed our predecessors, if not in the words, at all events in the matter."
Beginning of astrophysics and celestial mechanics
The eldest Banū Mūsā brother, Ja'far Muhammad ibn Mūsā ibn Shākir (9th century), made significant contributions to astrophysics and celestial mechanics. He was the first to hypothesize that the heavenly bodies and celestial spheres were subject to the same laws of physics as Earth, unlike the ancients who believed that the celestial spheres followed their own set of physical laws different from that of Earth.[24]
In his Astral Motion and The Force of Attraction, Muhammad ibn Musa proposed that there was a force of attraction between heavenly bodies,[25] foreshadowing Newton's law of universal gravitation.[26]
Ibn al-Haytham (Alhacen), in his Book of Optics (1021), was the first to discover that the celestial spheres do not consist of solid matter, and he also discovered that the heavens are less dense than the air. These views were later repeated by Witelo and had a significant influence on the Copernican and Tychonic systems of astronomy.[27]
Beginning of experimental astronomy
In the eleventh century, Abū Rayhān al-Bīrūnī introduced the experimental method into astronomy and was the first to conduct elaborate experiments related to astronomical phenomena. He discovered the Milky Way galaxy to be a collection of numerous nebulous stars. In Afghanistan, he observed and described the solar eclipse on April 8, 1019, and the lunar eclipse on September 17, 1019, in detail, and gave the exact latitudes of the stars during the lunar eclipse.[28]
This led to the use of exacting empirical observations and experimental techniques by Muslim astronomers from the eleventh century onwards.[29]
1025-1450
During this period, a distinctive Islamic system of astronomy flourished. Within the Greek tradition and its successors it was traditional to separate mathematical astronomy (as typified by Ptolemy) from philosophical cosmology (as typified by Aristotle). Muslim scholars developed a program of seeking a physically real configuration (hay'a) of the universe, that would be consistent with both mathematical and physical principles. Within the context of this hay'a tradition, Muslim astronomers began questioning technical details of the Ptolemaic system of astronomy.[30] Most of these criticisms, however, continued to follow the Ptolemaic astronomical paradigm, remaining within the geocentric framework.[31] As the historian of astronomy, A. I. Sabra, noted:
"All Islamic astronomers from Thabit ibn Qurra in the ninth century to Ibn al-Shatir in the fourteenth, and all natural philosophers from al-Kindi to Averroes and later, are known to have accepted what Kuhn has called the "two-sphere universe" ...—the Greek picture of the world as consisting of two spheres of which one, the celestial sphere made up of a special element called aether, concentrically envelops the other, where the four elements of earth, water, air, and fire reside."[32]
Some Muslim astronomers, however, most notably Abū Rayhān al-Bīrūnī and Nasīr al-Dīn al-Tūsī, discussed whether the Earth moved and considered how this might be consistent with astronomical computations and physical systems.[33] Several other Muslim astronomers, most notably those following the Maragheh school of thought, developed non-Ptolemaic computational models within a geocentric context that were later adapted in the Copernican model in a heliocentric context.
Refutations of astrology
The first semantic distinction between astronomy and astrology was given by the Persian astronomer Abu Rayhan al-Biruni in the 11th century,[34] though he himself refuted astrology in another work. The study of astrology was also refuted by other Muslim astronomers at the time, including al-Farabi, Ibn al-Haytham, Avicenna and Averroes. Their reasons for refuting astrology were both due to the methods used by astrologers being conjectural rather than empirical and also due to the views of astrologers conflicting with orthodox Islam.[35]
Astrophysics and celestial mechanics
In astrophysics and celestial mechanics, Abū Rayhān al-Bīrūnī described the Earth's gravitation as:[36]
"The attraction of all things towards the centre of the earth."
Al-Biruni also discovered that gravity exists within the heavenly bodies and celestial spheres, and he criticized Aristotle's views of them not having any levity or gravity and of circular motion being an innate property of the heavenly bodies.[37]
In 1121, al-Khazini, in his treatise The Book of the Balance of Wisdom, states:[38]
"For each heavy body of a known weight positioned at a certain distance from the centre of the universe, its gravity depends on the remoteness from the centre of the universe. For that reason, the gravities of bodies relate as their distances from the centre of the universe."
Al-Khazini was thus the first to propose the theory that the gravities of bodies vary depending on their distances from the centre of the Earth. This phenomenon was not proven until Newton's law of universal gravitation in the 18th century.[38]
Beginning of hay'a tradition
Between 1025 and 1028, Ibn al-Haytham (Latinized as Alhacen), began the hay'a tradition of Islamic astronomy with his Al-Shuku ala Batlamyus (Doubts on Ptolemy). While maintaining the physical reality of the geocentric model, he was the first to criticise Ptolemy's astronomical system, which he criticised on empirical, observational and experimental grounds,[39] and for relating actual physical motions to imaginary mathematical points, lines and circles:
"Ptolemy assumed an arrangement that cannot exist, and the fact that this arrangement produces in his imagination the motions that belong to the planets does not free him from the error he committed in his assumed arrangement, for the existing motions of the planets cannot be the result of an arrangement that is impossible to exist."[40]
Ibn al-Haytham developed a physical structure of the Ptolemaic system in his Treatise on the configuration of the World, or Maqâlah fî hay'at al-‛âlam, which became an influential work in the hay'a tradition.[41] In his Epitome of Astronomy, he insisted that the heavenly bodies "were accountable to the laws of physics",[42] The foundations of telescopic astronomy can also be traced back to Ibn al-Haytham, due to the influence of his optical studies on the later development of the modern telescope.[43]
In 1038, Ibn al-Haytham described the first non-Ptolemaic configuration in The Model of the Motions. His reform was not concerned with cosmology, as he developed a systematic study of celestial kinematics that was completely geometric. This in turn led to innovative developments in infinitesimal geometry.[44] His reformed model was the first to reject the equant[45] and eccentrics,[46] separate natural philosophy from astronomy, free celestial kinematics from cosmology, and reduce physical entities to geometrical entities. The model also propounded the Earth's rotation about its axis,[47] and the centres of motion were geometrical points without any physical significance, like Johannes Kepler's model centuries later.[48] Ibn al-Haytham also describes an early version of Occam's razor, where he employs only minimal hypotheses regarding the properties that characterize astronomical motions, as he attempts to eliminate from his planetary model the cosmological hypotheses that cannot be observed from Earth.[49]
Early alternative models
In 1030, Abū al-Rayhān al-Bīrūnī discussed the Indian planetary theories of Aryabhata, Brahmagupta and Varahamihira in his Ta'rikh al-Hind (Latinized as Indica). Biruni stated that Brahmagupta and others consider that the earth rotates on its axis and Biruni noted that this does not create any mathematical problems.[50]
Abu Said al-Sijzi, a contemporary of al-Biruni, suggested the possible heliocentric movement of the Earth around the Sun, which al-Biruni did not reject.[51] Al-Biruni agreed with the Earth's rotation about its own axis, and while he was initially neutral regarding the heliocentric and geocentric models,[52] he considered heliocentrism to be a philosophical problem.[53] He remarked that if the Earth rotates on its axis and moves around the Sun, it would remain consistent with his astronomical parameters:[36][54]
"Rotation of the earth would in no way invalidate astronomical calculations, for all the astronomical data are as explicable in terms of the one theory as of the other. The problem is thus difficult of solution."
In 1031, al-Biruni completed his extensive astronomical encyclopaedia Kitab al-Qanun al-Mas'udi (Latinized as Canon Mas’udicus),[55] in which he recorded his astronomical findings and formulated astronomical tables. In it he presented a geocentric model, tabulating the distance of all the celestial spheres from the central Earth, computed according to the principles of Ptolemy's Almagest.[56] The book introduces the mathematical technique of analysing the acceleration of the planets, and first states that the motions of the solar apogee and the precession are not identical. Al-Biruni also discovered that the distance between the Earth and the Sun is larger than Ptolemy's estimate, on the basis that Ptolemy disregarded the annual solar eclipses.[36][57]
In 1070, Abu Ubayd al-Juzjani, a pupil of Avicenna, proposed a non-Ptolemaic configuration in his Tarik al-Aflak. In his work, he indicated the so-called "equant" problem of the Ptolemic model, and proposed a solution for the problem. He claimed that his teacher Avicenna had also worked out the equant problem.[58]
Andalusian school

In the 11th-12th centuries, astronomers in al-Andalus took up the challenge earlier posed by Ibn al-Haytham, namely to develop an alternate non-Ptolemaic configuration that evaded the errors found in the Ptolemaic model.[59] Like Ibn al-Haytham's critique, the anonymous Andalusian work, al-Istidrak ala Batlamyus (Recapitulation regarding Ptolemy), included a list of objections to Ptolemic astronomy.
In the late 11th century, al-Zarqali (Latinized as Arzachel) discovered that the orbits of the planets are elliptic orbits and not circular orbits,[60] though he still followed the Ptolemaic model.
In the 12th century, Averroes rejected the eccentric deferents introduced by Ptolemy. He rejected the Ptolemaic model and instead argued for a strictly concentric model of the universe. He wrote the following criticism on the Ptolemaic model of planetary motion:[61]
"To assert the existence of an eccentric sphere or an epicyclic sphere is contrary to nature. [...] The astronomy of our time offers no truth, but only agrees with the calculations and not with what exists."
Averroes' contemporary, Maimonides, wrote the following on the planetary model proposed by Ibn Bajjah (Avempace):
"I have heard that Abu Bakr [Ibn Bajja] discovered a system in which no epicycles occur, but eccentric spheres are not excluded by him. I have not heard it from his pupils; and even if it be correct that he discovered such a system, he has not gained much by it, for eccentricity is likewise contrary to the principles laid down by Aristotle.... I have explained to you that these difficulties do not concern the astronomer, for he does not profess to tell us the existing properties of the spheres, but to suggest, whether correctly or not, a theory in which the motion of the stars and planets is uniform and circular, and in agreement with observation."[62]
Later in the 12th century, Ibn Bajjah's successors, Ibn Tufail (Abubacer) and al-Betrugi (Alpetragius), were the first to propose planetary models without any equant, epicycles or eccentrics. Al-Betrugi was also the first to discover that the planets are self-luminous.[63] Their configurations, however, were not accepted due to the numerical predictions of the planetary positions in their models being less accurate than that of the Ptolemaic model,[64] mainly because they followed Aristotle's notion of perfect circular motion.
Maragha Revolution
The "Maragha Revolution" refers to the achievements of the "Maragha school", an astronomical tradition beginning in the Maragha observatory and continuing with astronomers from Damascus, Samarkand and Istanbul. Like their Andalusian predecessors, the Maragha astronomers attempted to solve the equant problem and produce alternative configurations to the Ptolemaic model. They were more successful than their Andalusian predecessors in producing non-Ptolemaic configurations which eliminated the equant and eccentrics, were more accurate than the Ptolemaic model in numerically predicting planetary positions, and were in better agreement with empirical observations.[65] The most important of the Maragha astronomers included Mo'ayyeduddin Urdi (d. 1266), Nasīr al-Dīn al-Tūsī (1201-1274), 'Umar al-Katibi al-Qazwini (d. 1277), Qutb al-Din al-Shirazi (1236-1311), Sadr al-Sharia al-Bukhari (c. 1347), Ibn al-Shatir (1304-1375), Ali al-Qushji (c. 1474), and Shams al-Din al-Khafri (d. 1550).[66]
Some have described their achievements in the 13th and 14th centuries as a "Maragha Revolution", "Maragha School Revolution", or "Scientific Revolution before the Renaissance". An important aspect of this revolution included the realization that astronomy should aim to describe the behaviour of physical bodies in mathematical language, and should not remain a mathematical hypothesis, which would only save the phenomena. The Maragha astronomers also realized that the Aristotelian view of motion in the universe being only circular or linear was not true, as the Tusi-couple showed that linear motion could also be produced by applying circular motions only.[67] Other achievements of the Maragha school include the first empirical observational evidence for the Earth's rotation on its axis by al-Tusi and al-Qushji,[68] the separation of natural philosophy from astronomy by Ibn al-Shatir and al-Qushji,[69] the rejection of the Ptolemaic model on empirical rather than philosophical grounds by Ibn al-Shatir,[65] and the development of a non-Ptolemaic model by Ibn al-Shatir that was mathematically identical to the heliocentric Copernical model.[70]

Mo'ayyeduddin Urdi (d. 1266) was the first of the Maragheh astronomers to develop a non-Ptolemaic model, and he proposed a new theorem, the "Urdi lemma".[71] Nasīr al-Dīn al-Tūsī (1201-1274) resolved significant problems in the Ptolemaic system by developing the Tusi-couple as an alternative to the physically problematic equant introduced by Ptolemy,[72] and conceived a plausible model for elliptical orbits.[55] Tusi's student Qutb al-Din al-Shirazi (1236-1311), in his The Limit of Accomplishment concerning Knowledge of the Heavens, discussed the possibility of heliocentrism. 'Umar al-Katibi al-Qazwini (d. 1277), who also worked at the Maragheh observatory, in his Hikmat al-'Ain, wrote an argument for a heliocentric model, though he later abandoned the idea.[51]
Ibn al-Shatir (1304–1375) of Damascus, in A Final Inquiry Concerning the Rectification of Planetary Theory, incorporated the Urdi lemma, and eliminated the need for an equant by introducing an extra epicycle (the Tusi-couple), departing from the Ptolemaic system in a way that was mathematically identical to what Nicolaus Copernicus did in the 16th century. Ibn al-Shatir's model was in better agreement with empirical observations than any previous model,[65] was also the first that permitted empirical testing.[73] His rectified model was later adapted into a heliocentric model by Copernicus,[72] which was mathematically achieved by reversing the direction of the last vector connecting the Earth to the Sun.[53] In the published version of his masterwork, De revolutionibus orbium coelestium, Copernicus also cites the theories of al-Battani, Arzachel and Averroes as influences,[55] while the works of Ibn al-Haytham and al-Biruni were also known in Europe at the time.
An area of active discussion in the Maragheh school, and later the Samarkand and Istanbul observatories, was the possibility of the Earth's rotation. Supporters of this theory included Nasīr al-Dīn al-Tūsī, Nizam al-Din al-Nisaburi (c. 1311), al-Sayyid al-Sharif al-Jurjani (1339-1413), Ali al-Qushji (d. 1474), and Abd al-Ali al-Birjandi (d. 1525). Al-Tusi was the first to present empirical observational evidence of the Earth's rotation, using the location of comets relevant to the Earth as evidence, which al-Qushji elaborated on with further empirical observations while rejecting Aristotelian natural philosophy altogether. Both of their arguments were similar to the arguments later used by Nicolaus Copernicus in 1543 to explain the Earth's rotation.[68]

1450-1900
This is the period of stagnation, when the traditional system of astronomy continued to be practised with enthusiasm, but with decreasing innovation. It was believed there was no innovation of major significance during this period, but this view has been questioned by historians of astronomy in recent times.
Earth's motion
The work of Ali al-Qushji (d. 1474), who worked at Samarkand and then Istanbul, is seen as a late example of innovation in Islamic astronomy and it is believed he may have had an influence on Nicolaus Copernicus due to similar arguments concerning the Earth's rotation. Before al-Qushji, the only astronomer to present an empirical argument for the Earth's rotation was Nasīr al-Dīn al-Tūsī (d. 1274), who used the phenomena of comets to refute Ptolemy's claim that a stationery Earth can be determined through observation alone. Al-Tusi, however, accepted that the Earth was stationery on the basis of natural philosophy instead, particularly Aristotelian physics. In the 15th century, the influence of Aristotelian physics and natural philosophy was declining due to religious opposition. Al-Qushji thus rejected Aristotelian physics and completely separated natural philosophy from astronomy, allowing astronomy to become a purely empirical and mathematical science. This allowed him to explore alternatives to the Aristotelian notion of a stationery Earth, as he explored the idea of a moving Earth. He elaborated on al-Tusi's argument and concluded, on the basis of empiricism rather than speculative philosophy, that the moving Earth theory is just as likely to be true as the stationary Earth theory.[68][74][75] Ali al-Qushji also improved on al-Tusi's planetary model and presented an alternative planetary model for Mercury.[76]
Later astronomers

George Saliba studied the works of the 16th century astronomer Shams al-Din al-Khafri (d. 1550), a commentator on earlier Maragheh astronomers. Saliba wrote the following on al-Khafri's work:
"By his sheer insight into the role of mathematics in describing natural phenomena, this astronomer managed to bring the hay'a tradition to such unparalleled heights that could not be matched anywhere else in the world at that time neither mathematically nor astronomically. By working on the alternative mathematical models that could replace those of Ptolemy, and by scrutinizing the works of his predecessors who were all searching for unique mathematical models that could describe the physical phenomena consistently, this astronomer finally realized that all mathematical modeling had no physical truth by itself and was simply another language with which one could describe the physical observed reality. He also realized that the specific phenomena that were being described by the Ptolemaic models did not have unique mathematical solutions that were subject to the same restraints. Rather there were several mathematical models that could account for the Ptolemaic observations, yield identical predictive results at the same critical points used by Ptolemy to construct his own models (thus accounting for the observations as perfectly as Ptolemy could) and still meet the consistency requirement that was imposed by the Aristotelian cosmology which was adopted by the writers in the hay'a tradition."[77]
Another notable 16th century Muslim astronomer was Taqi al-Din, who built the Istanbul observatory of al-Din in 1577, where he carried out astronomical observations until 1580. He produced a zij (named Unbored Pearl) and astronomical catalogues that were more accurate than those of his contemporaries, Tycho Brahe and Nicolaus Copernicus. Taqi al-Din was also the first astronomer to employ a decimal point notation in his observations rather than the sexagesimal fractions used by his contemporaries and predecessors.[78]
1900-present
After the 16th century, there were no more significant astronomical innovations from the Islamic world until the 20th century, when Muslim rocket scientists from Soviet Central Asia were involved in research on astronautics and space exploration.
Astronautics and space exploration
Kerim Kerimov from Azerbaijan was one of the most important key figures in early space exploration. He was one of the founders of the Soviet space program, one of the lead architects behind the first human spaceflight (Vostok 1), and responsible for the launch of the first space stations (the Salyut and Mir series) as well as their predecessors (the Cosmos 186 and Cosmos 188).[79][80]
Farouk El-Baz from Egypt worked for the rival NASA and was involved in the first Moon landings with the Apollo program, where he was secretary of the Landing Site Selection Committee, Principal Investigator of Visual Observations and Photography, chairman of the Astronaut Training Group, and assisted in the planning of scientific explorations of the Moon, including the selection of landing sites for the Apollo missions and the training of astronauts in lunar observations and photography.[81]
In the late 20th and early 21st centuries, there have also been a number of Muslim astronauts, the first being Sultan bin Salman bin Abdulaziz Al Saud as a Payload Specialist aboard STS-51-G Space Shuttle Discovery, followed by Muhammed Faris aboard Soyuz TM-2 and Soyuz TM-3 to Mir space station; Abdul Ahad Mohmand aboard Soyuz TM-5 to Mir; Talgat Musabayev (one of the top 25 astronauts by time in space) as a flight engineer aboard Soyuz TM-19 to Mir, commander of Soyuz TM-27 to Mir, and commander of Soyuz TM-32 and Soyuz TM-31 to International Space Station (ISS); and Anousheh Ansari, the first woman to travel to ISS and the fourth space tourist.
In 2007, Sheikh Muszaphar Shukor from Malaysia travelled to ISS with his Expedition 16 crew aboard Soyuz TMA-11 as part of the Angkasawan program during Ramadan, for which the National Fatwa Council wrote Guidelines for Performing Islamic Rites (Ibadah) at the International Space Station, giving advice on issues such as prayer in a low-gravity environment, the location of Mecca from ISS, determination of prayer times, and issues surrounding fasting. Shukor also celebrated Eid ul-Fitr aboard ISS. He was both an astronaut and an orthopedic surgeon, and is most notable for being the first to perform biomedical research in space, mainly related to the characteristics and growth of liver cancer and leukemia cells and the crystallisation of various proteins and microbes in space.[82]
Other prominent Muslim scientists involved in research on the space sciences and space exploration include Essam Heggy who is working in the NASA Mars Exploration Program in the Lunar and Planetary Institute in Houston, Ahmed Salem, Alaa Ibrahim, Mohamed Sultan, and Ahmed Noor.[83]
Observatories
The modern astronomical observatory as a research institute (rather than just a location) was first introduced by medieval Muslim astronomers. The Islamic observatory was the first specialized astronomical institution with its own scientific staff, astronomical instruments, and building where astronomical research and observations are carried out. Islamic observatories were also the first to employ enormously large astronomical instruments in order to improve the accuracy of their observations.[84]
The Islamic observatories were similar to modern scientific research institutions, in that "group research was emphasized," and "theoretical investigations went hand in hand with observations."[85]
Early observatories
The first systematic observations in Islam are reported to have taken place under the patronage of al-Ma'mun, and the first Islamic observatories were built in 9th century Iraq under his patronage.
In many private observatories from Damascus to Baghdad, meridian degrees were measured, solar parameters were established, and detailed observations of the Sun, Moon, and planets were undertaken.
In the 10th century, the Buwayhid dynasty encouraged the undertaking of extensive works in Astronomy, such as the construction of a large scale instrument with which observations were made in the year 950. We know of this by recordings made in the zij of astronomers such as Ibn al-Alam. The great astronomer Abd Al-Rahman Al Sufi was patronised by prince Adud o-dowleh, who systematically revised Ptolemy's catalogue of stars. Sharaf al-Daula also established a similar observatory in Baghdad. And reports by Ibn Yunus and al-Zarqall in Toledo and Cordoba indicate the use of sophisticated instruments for their time.
It was Malik Shah I who established the first large observatory, probably in Isfahan. It was here where Omar Khayyám with many other collaborators constructed a zij and formulated the Persian solar calendar, a.k.a. the jalali calendar, the most accurate solar calendar to date. A modern version of this calendar is still in official use in Iran today.
Late medieval observatories
The more influential observatories, however, were established beginning in the 13th century. The Maragheh observatory was founded by Nasīr al-Dīn al-Tūsī under the patronage of Hulegu Khan in the 13th century. Here, al-Tusi supervised its technical construction at Maragheh. The facility contained resting quarters for Hulagu Khan, as well as a library and mosque. Some of the top astronomers of the day gathered there, and from their collaboration resulted important modifications to the Ptolemaic system over a period of 50 years.
In 1420, prince Ulugh Beg, himself an astronomer and mathematician, founded another large observatory in Samarkand, the remains of which were excavated in 1908 by Russian teams. And finally, Taqi al-Din bin Ma'ruf founded the large Istanbul observatory of al-Din in 1577, which was on the same scale as those in Maragha and Samarkand.
Modern observatories
In modern times, many well-equipped observatories can be found in Jordan,[86] Palestine,[87] Lebanon,[88] UAE,[89] Tunisia,[90] and other Arab states are also active as well. Iran has modern facilities at Shiraz University and Tabriz University. In December 2005, Physics Today reported of Iranian plans to construct a "world class" facility with a 2.0 metre telescope observatory in the near future.[91]
Instruments

Modern knowledge of the instruments used by Muslim astronomers primarily comes from two sources. First the remaining instruments in private and museum collections today, and second the treatises and manuscripts preserved from the Middle Ages.
Muslims made many improvements to instruments already in use before their time, such as adding new scales or details, and invented many of their own new instruments. Their contributions to astronomical instrumentation are abundant. Many of these instruments were often invented or designed for Islamic purposes, such as the determination of the direction of Qibla or the times of Salah.
Astrolabes
Brass astrolabes were developed in much of the Islamic world, chiefly as an aid to finding the qibla. The earliest known example is dated 315 (in the Islamic calendar, corresponding to 927-8CE). The first person credited for building the Astrolabe in the Islamic world is reportedly Fazari.[92] Though the first primitive astrolabe to chart the stars was invented in the Hellenistic civilization, al-Fazari made several improvements to the device. The Arabs then took it during the Abbasid Caliphate and perfected it to be used to find the beginning of Ramadan, the hours of prayer (Salah), the direction of Mecca (Qibla), and over a thousand other uses.[93]
In the 10th century, al-Sufi first described over 1000 different uses of an astrolabe, in areas as diverse as astronomy, astrology, horoscopes, navigation, surveying, timekeeping, Qibla, Salah, etc.[93]
- Large astrolabe
Ibn Yunus accurately observed more than 10,000 entries for the sun's position for many years using a large astrolabe with a diameter of nearly 1.4 metres.[18]
- Mechanical geared astrolabe
The first mechanical astrolabes with gears were invented in the Muslim world, and were perfected by Ibn Samh (c. 1020). One such device with eight gear-wheels was also constructed by Abū Rayhān al-Bīrūnī in 996. These can be considered as an ancestor of the mechanical clocks developed by later Muslim engineers.[94]
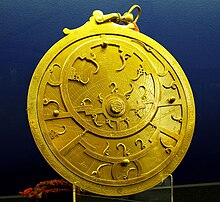
- Navigational astrolabe
The first navigational astrolabe was invented in the Islamic world during the Middle Ages, and employed the use of a polar projection system.[95]
- Orthographical astrolabe
Abu Rayhan al-Biruni invented and wrote the earliest treatise on the orthographical astrolabe in the 1000s.[36]
- Saphaea and Zuraqi
The first astrolabe instruments were used to read the rise of the time of rise of the Sun and fixed stars. In the 11th century, Arzachel (al-Zarqali) of al-Andalus constructed the first universal astrolabe which, unlike its predecessors, did not depend on the latitude of the observer, and could be used anywhere on the Earth. This universal astrolabe instrument became known in Europe as the "Saphaea". Another astrolabe, the Zuraqi is a unique astrolabe invented by al-Sijzi for a heliocentric planetary model in which the Earth is moving rather than the sky.[23]
- Linear astrolabe
A famous work by Sharaf al-Dīn al-Tūsī is one in which he describes the linear astrolabe, sometimes called the "staff of al-Tusi", which he invented.[96]
- Astrolabic clock
Ibn al-Shatir invented the astrolabic clock in 14th century Syria.[97]
Analog computers

Various analog computer devices were invented to compute the latitudes of the Sun, Moon, and planets, the ecliptic of the Sun, the time of day at which planetary conjunctions will occur and for performing linear interpolation.
- Equatorium
The Equatorium was an analog computer invented by Abū Ishāq Ibrāhīm al-Zarqālī (Arzachel) in al-Andalus, probably around 1015 CE. It is a mechanical device for finding the longitudes and positions of the Moon, Sun, and planets, without calculation using a geometrical model to represent the celestial body's mean and anomalistic position.[98]
- Planisphere and mechanical geared calendar computer
Abū Rayhān al-Bīrūnī invented and wrote the earliest treatise on the planisphere, an analog computer, in the 1000s.[36] He also invented the first mechanical lunisolar calendar computer which employed a gear train and eight gear-wheels.[99] This was an early example of a fixed-wired knowledge processing machine.[100]
- Mechanical astrolabe with geared calendar computer
In 1235, Abi Bakr of Isfahan invented a brass astrolabe with a geared calendar movement based on the design of Abū Rayhān al-Bīrūnī's mechanical calendar computer.[101] Abi Bakr's geared astrolabe uses a set of gear-wheels and is the oldest surviving complete mechanical geared machine in existence.[102][103]
- Plate of Conjunctions
In the 15th century, al-Kashi invented the Plate of Conjunctions, a computing instrument used to determine the time of day at which planetary conjunctions will occur,[104] and for performing linear interpolation.[105]
- Planetary computer
In the 15th century, al-Kashi also invented a mechanical planetary computer which he called the Plate of Zones, which could graphically solve a number of planetary problems, including the prediction of the true positions in longitude of the Sun and Moon,[105] and the planets in terms of elliptical orbits;[106] the latitudes of the Sun, Moon, and planets; and the ecliptic of the Sun. The instrument also incorporated an alhidade and ruler.[107]

Armillary spheres
An armillary sphere had similar applications to a celestial globe. No early Islamic armillary spheres survive, but several treatises on “the instrument with the rings” were written.
- Spherical astrolabe
The spherical astrolabe was first produced in the Islamic world.[108] It was an Islamic variation of the astrolabe and the armillary sphere, of which only one complete instrument, from the 14th century, has survived.
- Celestial globes
Celestial globes were used primarily for solving problems in celestial astronomy. Today, 126 such instruments remain worldwide, the oldest from the 11th century. The altitude of the sun, or the Right Ascension and Declination of stars could be calculated with these by inputting the location of the observer on the meridian ring of the globe.
Astronomical clocks
The Muslims constructed a variety of highly accurate astronomical clocks for use in their observatories.[109]
- Water-powered astronomical clocks
Al-Jazari invented monumental water-powered astronomical clocks which displayed moving models of the Sun, Moon, and stars. His largest astronomical clock displayed the zodiac and the solar and lunar orbits. Another innovative feature of the clock was a pointer which traveled across the top of a gateway and caused automatic doors to open every hour.[110]
- Mechanical observational clock
Taqi al-Din invented the "observational clock", which he described as "a mechanical clock with three dials which show the hours, the minutes, and the seconds." He used this for astronomical purposes, specifically for measuring the right ascension of the stars. This is considered one of the most important innovations in 16th century practical astronomy, as previous clocks were not accurate enough to be used for astronomical purposes.[78]
Dials
Muslim astronomers and engineers invented a variety of dials for timekeeping, and for determining the times of the five daily prayers.
- Sundials
Muslims made several important improvements to the theory and construction of sundials, which they inherited from their Indian and Hellenistic predecessors. Al-Khwarizmi made tables for these instruments which considerably shortened the time needed to make specific calculations. Muslim sundials could also be observed from anywhere on the Earth. Sundials were frequently placed on mosques to determine the time of prayer. One of the most striking examples was built in the 14th century by the muwaqqit (timekeeper) of the Umayyad Mosque in Damascus, Ibn al-Shatir.[111] Muslim astronomers and engineers were the first to write instructions on the construction of horizantal sundials, vertical sundials, and polar sundials.[112]
- Navicula de Venetiis
This was a universal horary dial invented in 9th century Baghdad. It was used for accurate timekeeping by the Sun and Stars, and could be observed from any latitude.[113] This was later known in Europe as the "Navicula de Venetiis",[114] which was considered the most sophisticated timekeeping instrument of the Renaissance.[115]
- Compass dial
In the 13th century, Ibn al-Shatir invented the compass dial, a timekeeping device incorporating both a universal sundial and a magnetic compass. He invented for the purpose of finding the times of Salah.[116]
Mural instruments
A number of mural instruments (including several different quadrants) were invented by Muslim astronomers and engineers.
- Sine quadrant
The sine quadrant, invented by Muhammad ibn Mūsā al-Khwārizmī in 9th century Baghdad, was used for astronomical calculations.[117]
- Horary quadrant
The first horary quadrant for specific latitudes, was invented by Muhammad ibn Mūsā al-Khwārizmī in 9th century Baghdad, center of the development of quadrants.[117] It was used to determine time (especially the times of prayer) by observations of the Sun or stars.[118]
- Quadrans Novus
Quadrans Vetus was a universal horary quadrant, an ingeniuous mathematical device invented by al-Khwarizmi in 9th century Baghdad and later known as the "Quadrans Vetus" (Old Quadrant) in medieval Europe from the 13th century. It could be used for any latitude on Earth and at any time of the year to determine the time in hours from the altitude of the Sun. This was the second most widely used astronomical instrument during the Middle Ages after the astrolabe. One of its main purposes in the Islamic world was to determine the times of Salah.[117]
- Quadrans Vetus
The astrolabic quadrant was invented in Egypt in the 11th century or 12th century, and later known in Europe as the "Quadrans Vetus" (New Quadrant).[119]
- Almucantar quadrant
The first almucantar quadrant was invented in the medieval Islamic world, and it employed the use of trigonometry. The term "almucantar" is itself derived from Arabic.[120] The Almucantar quadrant was originally modified from the astrolabe.[112]
- Sextant
The first sextant was constructed in Ray, Iran, by Abu-Mahmud al-Khujandi in 994. It was a very large sextant that achieved a high level of accuracy for astronomical measurements, which he described his in his treatise, On the obliquity of the ecliptic and the latitudes of the cities.[121] In the 15th century, Ulugh Beg constructed the "Fakhri Sextant", which had a radius of approximately 36 meters. Constructed in Samarkand, Uzbekistan, the arc was finely constructed with a staircase on either side to provide access for the assistants who performed the measurements.
Other instruments
Various other astrononmical instruments were also invented in the Islamic world:
- The first astronomical uses of the magnetic compass is found in a treatise on astronomical instruments written by the Yemeni sultan al-Ashraf (d. 1296). This was the first reference to the compass in astronomical literature.[122]
- The Alhidade was invented in the Islamic world, while the term "alhidade" is itself derived from Arabic.
- A compendium was a multi-purpose astronomical instrument, first constructed by the Muslim astronomer Ibn al-Shatir in the 13th century. His compendium featured an alhidade and polar sundial among other things. Al-Wafa'i developed another compendium in the 15th century which he called the "equitorial circle", which also featured a horizontal sundial. These compendia later became popular in Renaissance Europe.[112]
- Islamic quadrants used for various astronomical and timekeeping purposes from the 10th century introduced orthogonal and regular grids and markings that are identical to modern graph paper.[123][124]
- In 17th century Safavid Persia, two unique brass instruments with Mecca-centred world maps engraved on them were produced primarily for the purpose of finding the Qibla. These instruments were engraved with cartographic grids to make it more convenient to find the direction and distance to Mecca at the centre from anywhere on the Earth, which may be based on cartographic grids dating back to 10th century Baghdad.[115] One of the two instruments, produced by Muhammad Husayn,[125] also had a sundial and compass attached to it.[126]
- The shadow square was an instrument used to determine the linear height of an object, in conjunction with the alidade, for angular observations.[127] It was invented by Muhammad ibn Mūsā al-Khwārizmī in 9th century Baghdad.[128]
- Treatises on instruments
In the 12th century, al-Khazini wrote the Risala fi'l-alat (Treatise on Instruments) which had seven parts describing different scientific instruments: the triquetrum, dioptra, a triangular instrument he invented, the quadrant and sextant, the astrolabe, and original instruments involving reflection.[129]
In 14th century Egypt, Najm al-Din al-Misri (c. 1325) wrote a treatise describing over 100 different types of scientific and astronomical instruments, many of which he invented himself.[115]
In 1416, al-Kashi wrote the Treatise on Astronomical Observational Instruments, which described a variety of different instruments, including the triquetrum and armillary sphere, the equinoctial armillary and solsticial armillary of Mo'ayyeduddin Urdi, the sine and versine instrument of Urdi, the sextant of al-Khujandi, the Fakhri sextant at the Samarqand observatory, a double quadrant Azimuth-altitude instrument he invented, and a small armillary sphere incorporating an alhidade which he invented.[130]
List of notable treatises
Zijes
- Ibrahim al-Fazari (d. 777) and Muhammad al-Fazari (d. 796/806)
- Az-Zij ‛alā Sinī al-‛Arab (c. 750)
- Yaqūb ibn Tāriq (d. 796)
- Az-Zij al-Mahlul min as-Sindhind li-Darajat Daraja
- Muhammad ibn Mūsā al-Khwārizmī (Latinized as Algorismi) (c. 780-850)
- Zij al-Sindhind (c. 830)
- Muhammad ibn Jābir al-Harrānī al-Battānī (Latinized as Albategni) (853-929)
- Az-Zij as-Sabi
- Abd Al-Rahman Al Sufi (Latinized as Azophi) (903-986)
- Book of Fixed Stars (c. 964)
- Al-Zarqali (Latinized as Arzachel) (1028-1087)
- Al-Khazini (fl. 1115-1130)
- Az-Zij as-Sanjarī (Sinjaric Tables) (1115-1116)
- Nasīr al-Dīn al-Tūsī (1201-1274)
- Zij-i Ilkhani (Ilkhanic Tables) (1272)
- Jamshīd al-Kāshī (1380-1429)
- Khaqani Zij
- Ulugh Beg (1394-1449)
- Zij-i-Sultani (1437)
- Taqi al-Din (1526-1585)
- Unbored Pearl (1577-1580)
Other works
- Ja'far Muhammad ibn Mūsā ibn Shākir (Latinized as Mohammed Ben Musa) (800-873)
- Book on the motion of the orbs
- Astral Motion
- The Force of Attraction
- Ahmad ibn Muhammad ibn Kathīr al-Farghānī (Latinized as Alfraganus) (d. 850)
- Elements of astronomy on the celestial motions (c. 833)
- Kitab fi Jawami Ilm al-Nujum
- Ibn al-Haytham (Latinized as Alhacen) (965-1039)
- On the Configuration of the World
- Doubts concerning Ptolemy (c. 1028)
- The Resolution of Doubts (c. 1029)
- The Model of the Motions of Each of the Seven Planets (1029-1039)
- Abū Rayhān al-Bīrūnī (973-1048)
- Kitab al-Qanun al-Mas'udi (Latinized as Canon Mas’udicus) (1031)
- Abu Ubayd al-Juzjani (c. 1070)
- Tarik al-Aflak (1070)
- Al-Istidrak ala Batlamyus (Recapitulation regarding Ptolemy) (11th century)
- Al-Khazini (fl. 1115-1130)
- Risala fi'l-alat (Treatise on Instruments)
- Nasīr al-Dīn al-Tūsī (1201-1274)
- Al-Tadhkirah fi'ilm al-hay'ah (Memento in astronomy)
- 'Umar al-Katibi al-Qazwini (d. 1277)
- Hikmat al-'Ain
- Qutb al-Din al-Shirazi (1236-1311)
- The Limit of Accomplishment concerning Knowledge of the Heavens
- Ibn al-Shatir (1304–1375)
- A Final Inquiry Concerning the Rectification of Planetary Theory
- Ali al-Qushji (d. 1474)
- Concerning the Supposed Dependence of Astronomy upon Philosophy
- Shams al-Din al-Khafri (d. 1525)
- The complement to the explanation of the memento
List of Arabic star names
Many of the modern names for numerous stars and constellations are derived from their Arabic language names. Examples include: Acamar, Aldebaran, Altair, Baham, Baten Kaitos, Caph, Dabih, Edasich, Furud, Gienah, Hadar, Izar, Jabbah, Keid, Lesath, Mirak, Nashira, Okda, Phad, Rigel, Sadr, Tarf, Vega, and as well as others.
See also
- Arab and Persian astrology
- History of astronomy
- Hebrew astronomy
- Islamic science
- Islamic Golden Age
- Islamic astrology
- Inventions in the Muslim world
- List of Muslim astronomers
- List of Muslim scientists
- List of Iranian scientists
- Zij
Notes
- ^ a b c (Gingerich 1986)
- ^ "Arabic Star Names". 2007-05-01. Islamic Crescents' Observation Project.
- ^ (Ilyas 1997)
- ^ a b c (Dallal 1999, p. 162)
- ^ A. Abd-Allah. "The Qur'an, Knowledge, and Science". University of Southern California. Retrieved 2008-01-22.
- ^ James A. Michene, "Islam: The Misunderstood Religion", Reader's Digest, May 1955, pp. 68-70.
- ^ a b c Research Committee of Strasburg University, Imam Jafar Ibn Muhammad As-Sadiq A.S. The Great Muslim Scientist and Philosopher, translated by Kaukab Ali Mirza, 2000. Willowdale Ont. ISBN 0969949014.
- ^ This book is not related to al-Khwarizmi's Zij al-Sindh. On zijes, see (Kennedy 1956)
- ^ King|2002|p=240}}
- ^ "Greek Astronomy". Retrieved 2008-01-15.
- ^ "Almagest". The Internet Encyclopedia of Science. Retrieved 2008-01-15.
- ^ O'Connor, John J.; Robertson, Edmund F. (November 1999), "Abu Said Sinan ibn Thabit ibn Qurra", MacTutor History of Mathematics Archive, University of St Andrews, retrieved 2008-01-15
- ^ (Dallal 1999, p. 163)
- ^ (Dallal 1999, p. 164)
- ^ C. Singer (1959), A Short History of Scientific Ideas, p. 151, Oxford University Press (cf. (Zaimeche 2002) )
- ^ G. M. Wickens, "The Middle East as a world Centre of science and medicine", in R. M. Savory, Introduction to Islamic Civilization, pp. 111-118, Cambridge University Press (cf. (Zaimeche 2002) )
- ^ 23rd Annual Conference on the History of Arabic Science, October 2001, Aleppo, Syria (cf. (Zaimeche 2002) )
- ^ a b (Zaimeche 2002)
- ^ Aulie, Richard P. (March 1994), "Al-Ghazali Contra Aristotle: An Unforeseen Overture to Science In Eleventh-Century Baghdad", Perpectives on Science and Christian Faith, 45: 26–46
{{citation}}
: CS1 maint: date and year (link) (cf. "References". 1001 Inventions. Retrieved 2008-01-22.) - ^ (Saliba 2007)
- ^ Bartel Leendert van der Waerden (1987). "The Heliocentric System in Greek, Persian and Hindu Astronomy", Annals of the New York Academy of Sciences 500 (1), 525–545 [534-537].
- ^ (Nasr 1993, p. 77)
- ^ a b c (Nasr 1993, pp. 135–136)
- ^ (Saliba 1994a, p. 116)
- ^ K. A. Waheed (1978). Islam and The Origins of Modern Science, p. 27. Islamic Publication Ltd., Lahore.
- ^ Robert Briffault (1938). The Making of Humanity, p. 191.
- ^ Edward Rosen (1985), "The Dissolution of the Solid Celestial Spheres", Journal of the History of Ideas 46 (1): 13-31 [19-20, 21].
- ^ Dr. A. Zahoor (1997). [http://209.85.135.104/search?sourceid=navclient-ff&ie=UTF-8&q=cache%3Ahttp%3A%2F%2Fwww.unhas.ac.id%2F~rhiza%2Fsaintis%2Fbiruni.html
archivedate=2008-01-18 "Abu Raihan Muhammad al-Biruni"]. Hasanuddin University. Retrieved 2008-01-18.
{{cite web}}
:|archive-url=
requires|archive-date=
(help); Check|archiveurl=
value (help); Missing pipe in:|archiveurl=
(help); line feed character in|archiveurl=
at position 132 (help) - ^ (Huff 2003, p. 326)
- ^ (Sabra 1998, pp. 293–8)
- ^ Dennis Duke. "Arabic Models for outer Planets and Venus". Retrieved 2008-01-22.
- ^ (Sabra 1998, pp. 317–18)
- ^ (Teresi, Ragep & Hart 2002)
- ^ S. Pines (September 1964). "The Semantic Distinction between the Terms Astronomy and Astrology according to al-Biruni", Isis 55 (3): 343-349.
- ^ (Saliba 1994, pp. 60 & 67-69)
- ^ a b c d e "Khwarizm". Foundation for Science Technology and Civilisation. Retrieved 2008-01-22. Cite error: The named reference "Khwarizm" was defined multiple times with different content (see the help page).
- ^ (Iqbal & Berjak 2003)
- ^ a b (Zaimeche 2002, p. 7)
- ^ (Sabra 1998, p. 300)
- ^ "Nicolaus Copernicus", [[Stanford Encyclopedia of Philosophy]], 2004, retrieved 2008-01-22
{{citation}}
: URL–wikilink conflict (help) - ^ Y. Tzvi Langermann, ed. and trans., Ibn al-Haytham's On the Configuration of the World, Harvard Dissertations in the History of Science, (New York: Garland, 1990), pp. 25-34
- ^ Duhem, Pierre (1908, 1969). To Save the Phenomena: An Essay on the Idea of Physical theory from Plato to Galileo, p. 28. University of Chicago Press, Chicago.
- ^ O. S. Marshall (1950). "Alhazen and the Telescope", Astronomical Society of the Pacific Leaflets 6, p. 4.
- ^ (Rashed 2007)
- ^ (Rashed 2007, pp. 20 & 53)
- ^ (Rashed 2007, pp. 33–4)
- ^ (Rashed 2007, pp. 20 & 32-33)
- ^ (Rashed 2007, pp. 51–2)
- ^ (Rashed 2007, pp. 35–6)
- ^ (Nasr 1993, p. 135, n. 13)
- ^ a b (Baker & Chapter 2002)
- ^ (Marmura 1965)
- ^ a b (Saliba 1999)
- ^ G. Wiet, V. Elisseeff, P. Wolff, J. Naudu (1975). History of Mankind, Vol 3: The Great medieval Civilisations, p. 649. George Allen & Unwin Ltd, UNESCO.
- ^ a b c (Covington 2007)
- ^ (Nasr 1993, p. 134)
- ^ (Saliba 1980, p. 249)
- ^ (Sabra 1998, pp. 305–306)
- ^ (Saliba 1981, p. 219)
- ^ Robert Briffault (1938). The Making of Humanity, p. 190.
- ^ Owen Gingerich (April 1986). "Islamic astronomy", Scientific American 254 (10), p. 74.
- ^ Bernard R. Goldstein (March 1972). "Theory and Observation in Medieval Astronomy", Isis 63 (1): 39-47 [40-41].
- ^ Bernard R. Goldstein (March 1972). "Theory and Observation in Medieval Astronomy", Isis 63 (1): 39-47 [41].
- ^ Ptolemaic Astronomy, Islamic Planetary Theory, and Copernicus's Debt to the Maragha School, Science and Its Times, Thomson Gale.
- ^ a b c (Saliba 1994b, pp. 233-234 & 240)
- ^ (Dallal 1999, p. 171)
- ^ (Saliba 1994b, pp. 245, 250, 256–257)
- ^ a b c F. Jamil Ragep (2001), "Tusi and Copernicus: The Earth's Motion in Context", Science in Context 14 (1-2): 145–163. Cambridge University Press.
- ^ F. Jamil Ragep (2001), "Freeing Astronomy from Philosophy: An Aspect of Islamic Influence on Science", Osiris, 2nd Series, Vol. 16, pp. 49-64, 66-71.
- ^ (Saliba 1994b, pp. 254 & 256-257)
- ^ (Saliba 1979)
- ^ a b (Gill 2005)
- ^ Y. M. Faruqi (2006). "Contributions of Islamic scholars to the scientific enterprise", International Education Journal 7 (4): 395-396.
- ^ F. Jamil Ragep (2001), "Freeing Astronomy from Philosophy: An Aspect of Islamic Influence on Science", Osiris, 2nd Series, Vol. 16, Science in Theistic Contexts: Cognitive Dimensions, pp. 49-64, 66-71.
- ^ Edith Dudley Sylla, "Creation and nature", in Arthur Stephen McGrade (2003), pp. 178-179, Cambridge University Press, ISBN 0521000637.
- ^ George Saliba, "Arabic planetary theories after the eleventh century AD", in (Rashed & Morelon 1996, pp. 58-127 [123-4])
- ^ (Saliba 2000)
- ^ a b Sevim Tekeli, "Taqi al-Din", in Helaine Selin (1997), Encyclopaedia of the History of Science, Technology, and Medicine in Non-Western Cultures, Kluwer Academic Publishers, ISBN 0792340663.
- ^ Peter Bond, Obituary: Lt-Gen Kerim Kerimov, The Independent, 7 April 2003.
- ^ Betty Blair (1995), "Behind Soviet Aeronauts", Azerbaijan International 3 (3).
- ^ "Muslim Scientists and Space Exploration - Farouk El-Baz: With Apollo to the Moon - Interview". IslamOnline. Retrieved 2008-01-15.
- ^ theStar (2007). "Mission in space".
{{cite web}}
: Text "publisherTheStar" ignored (help) - ^ "Muslim Scientists and Space Exploration - Essam Heggy: Into the Heart of Mars - Interview". IslamOnline. Retrieved 2008-01-15.
- ^ (Kennedy 1962)
- ^ Prof. Osman Bakar (Georgetown University), Islam's Contribution to Human Civilization: Science and Culture, CIC's annual Ottawa dinner, October 15, 2001.
- ^ "Jordanian Astronomical Society". Retrieved 2008-01-15.
- ^ "Palestinian Astronomical Society". Retrieved 2008-01-15.
- ^ "Lebanese Astronomical society". Retrieved 2008-01-15.
- ^ "Emirates Astronomical Society". Retrieved 2008-01-15.
- ^ "Société Astronomique de Tunise". Retrieved 2008-01-15.
- ^ Feder Toni (July, 2004). Iran Invests in Astronomy. Physics Today.
- ^ Richard Nelson Frye, Golden Age of Persia, p. 163.
- ^ a b Dr. Emily Winterburn (National Maritime Museum), Using an Astrolabe, Foundation for Science Technology and Civilisation, 2005.
- ^ Islam, Knowledge, and Science. University of Southern California.
- ^ Robert Hannah (1997). "The Mapping of the Heavens by Peter Whitfield", Imago Mundi 49, pp. 161-162.
- ^ Linear astrolabe, Encyclopædia Britannica.
- ^ (King 1983, pp. 545–546)
- ^ (Hassan)
- ^ (Hill 1985)
- ^ Tuncer Oren (2001). "Advances in Computer and Information Sciences: From Abacus to Holonic Agents", Turk J Elec Engin 9 (1): 63-70 [64].
- ^ Silvio A. Bedini, Francis R. Maddison (1966). "Mechanical Universe: The Astrarium of Giovanni de' Dondi", Transactions of the American Philosophical Society 56 (5): 1-69.
- ^ Astrolabe gearing, Museum of the History of Science, Oxford.
- ^ History of the Astrolabe, Museum of the History of Science, Oxford.
- ^ (Kennedy 1947, p. 56)
- ^ a b (Kennedy 1950)
- ^ (Kennedy 1952)
- ^ (Kennedy 1951)
- ^ Emilie Savage-Smith (1993). "Book Reviews", Journal of Islamic Studies 4 (2): 296-299.
"There is no evidence for the Hellenistic origin of the spherical astrolabe, but rather evidence so far available suggests that it may have been an early but distinctly Islamic development with no Greek antecedents."
- ^ (Ajram 1992)
- ^ (Hill 1991)
- ^ (King 1999a, pp. 168–9)
- ^ a b c David A. King, "Astronomy and Islamic society", in (Rashed & Morelon 1996, pp. 128-184 [163-8])
- ^ (King 2005)
- ^ (King 2003)
- ^ a b c (King 2004)
- ^ (King 1983, pp. 547–548)
- ^ a b c (King 2002, pp. 237–238)
- ^ (King 1999a, pp. 167–8)
- ^ (King, Moreno & Cleempoel 2002, p. 333)
- ^ Elly Dekker (1995), "An unrecorded medieval astrolabe quadrant from c. 1300", Annals of Science 52 (1): 1-47 [6].
- ^ O'Connor, John J.; Robertson, Edmund F., "Al-Khujandi", MacTutor History of Mathematics Archive, University of St Andrews
- ^ Emilie Savage-Smith (1988), "Gleanings from an Arabist's Workshop: Current Trends in the Study of Medieval Islamic Science and Medicine", Isis 79 (2): 246-266 [263].
- ^ Josef W. Meri (2006), Medieval Islamic Civilization: An Encyclopedia, p. 75, Taylor and Francis, ISBN 0415966914.
- ^ (King 1999b, p. 17)
- ^ (Iqbal 2003)
- ^ (King 1997, p. 62)
- ^ Shadow square, National Maritime Museum.
- ^ (King 2002, pp. 238–239)
- ^ Robert E. Hall (1973). "Al-Biruni", Dictionary of Scientific Biography, Vol. VII, p. 338.
- ^ (Kennedy 1961, pp. 104–107)
References
- Adnan, Abdulhak (1939), La science chez les Turcs ottomans, Paris
- Template:Harvard reference
- Template:Harvard reference, in Sharif, M. M., "A History of Muslim Philosophy", Philosophia Islamica
- Template:Harvard reference
- Template:Harvard reference
- Gautier, Antoine (December 2005), "L'âge d'or de l'astronomie ottomane", L'Astronomie (monthly magazine created by Camille Flammarion in 1882), vol. 119
- Template:Harvard reference
- Template:Harvard reference
- Template:Harvard reference
- Template:Harvard reference
- Template:Harvard reference (cf. Hill, Donald R., Mechanical Engineering, retrieved 2008-01-22)
- Hill, Donald R. (1993), Islamic Science And Engineering, Edinburgh University Press, ISBN 0-7486-0455-3
- Template:Harvard reference
- Template:Harvard reference
- Template:Harvard reference, June 2003
- Template:Harvard reference, June 2003
- Template:Harvard reference
- Template:Harvard reference
- Template:Harvard reference
- Template:Harvard reference
- Kennedy, Edward S. (1956), "A Survey of Islamic Astronomical Tables", Transactions of the American Philosophical Society, 46 (2)
- Template:Harvard reference
- Template:Harvard reference
- Kennedy, Edward S. (1998), Astronomy and Astrology in the Medieval Islamic World, Brookfield, VT: Ashgate
- Template:Harvard reference
- King, David A. (1986), Islamic mathematical astronomy, London
- Template:Harvard reference
- Template:Harvard reference
- Template:Harvard reference
- Template:Harvard reference
- Template:Harvard reference
- Template:Harvard reference, June 2004
- Template:Harvard reference
- Template:Harvard reference
- Template:Harvard reference
- Template:Harvard reference
- Template:Harvard reference
- Roshdi, Rashed (2007), "The Celestial Kinematics of Ibn al-Haytham", Arabic Sciences and Philosophy, 17, Cambridge University Press: 7–55
- Sabra, A. I. (1998), "Configuring the Universe: Aporetic, Problem Solving, and Kinematic Modeling as Themes of Arabic Astronomy", Perspectives on Science, 6: 288–330
- Template:Harvard reference
- Template:Harvard reference
- Template:Harvard reference
- Template:Harvard reference
- Template:Harvard reference
- Template:Harvard reference
- Template:Harvard reference
- Template:Harvard reference
- Suter, H. (1902), Mathematiker und Astronomen der Araber
- Template:Harvard reference
- Template:Harvard reference
External links
- Iran Astronomy Sciences Academy IASA
- "Scientific American" article on Islamic Astronomy
- The Arab Union for Astronomy and Space Sciences (AUASS)
- King Abdul Aziz Observatory
- History of Islamic Astrolabes
- Arabic models for replacing the equant for the outer planets and Venus
- Ibn ash-Shatir model for Mercury
- Ibn ash-Shatir model for the Moon
- Arabian astronomy