Uranium
Template:Elementbox header Template:Elementbox series Template:Elementbox periodblock Template:Elementbox appearance Template:Elementbox atomicmass gpm Template:Elementbox econfig Template:Elementbox epershell Template:Elementbox section physicalprop Template:Elementbox phase Template:Elementbox density gpcm3nrt Template:Elementbox densityliq gpcm3mp Template:Elementbox meltingpoint Template:Elementbox boilingpoint Template:Elementbox heatfusion kjpmol Template:Elementbox heatvaporiz kjpmol Template:Elementbox heatcapacity jpmolkat25 Template:Elementbox vaporpressure katpa Template:Elementbox section atomicprop Template:Elementbox crystalstruct Template:Elementbox oxistates Template:Elementbox electroneg pauling Template:Elementbox ionizationenergies2 Template:Elementbox atomicradius pm Template:Elementbox vanderwaalsrad pm Template:Elementbox section miscellaneous Template:Elementbox magnetic Template:Elementbox eresist ohmmat0 Template:Elementbox thermalcond wpmkat300k Template:Elementbox thermalexpansion umpmkat25 Template:Elementbox speedofsound rodmpsat20 Template:Elementbox youngsmodulus gpa Template:Elementbox shearmodulus gpa Template:Elementbox bulkmodulus gpa Template:Elementbox poissonratio Template:Elementbox cas number Template:Elementbox isotopes begin |- ! style="text-align:right;" | 232U | style="text-align:center;" | syn | style="text-align:right;" | 68.9 y | α & SF | style="text-align:right;" | 228Th |- ! style="text-align:right;" | 233U | style="text-align:center;" | syn | style="text-align:right;" | 159,200 y | SF & α | style="text-align:right;" | 229Th |- ! style="text-align:right;" | 234U | style="text-align:right;" | 0.0058% | style="text-align:right;" | 245,500 y | SF & α | style="text-align:right;" | 230Th |- ! style="text-align:right;" | 235U | style="text-align:right;" | 0.72% | style="text-align:right;" | 7.038×108 y | SF & α | style="text-align:right;" | 231Th |- ! style="text-align:right;" | 236U | style="text-align:center;" | syn | style="text-align:right;" | 2.342×107 y | SF & α | style="text-align:right;" | 232Th |- ! style="text-align:right;" | 238U | style="text-align:right;" | 99.275% | style="text-align:right;" | 4.468×109 y | SF & α | style="text-align:right;" | 234Th Template:Elementbox isotopes end Template:Elementbox footer
Uranium (IPA: /jəˈreɪniəm/) is a chemical element in the actinide series of the periodic table that has the symbol U and atomic number 92. The heaviest naturally occurring element, uranium is silvery metallic in color, nearly twice as dense as lead and weakly radioactive. It occurs naturally in low concentrations (a few parts per million) in soil, rock, and surface and groundwater and is commercially extracted from uranium-bearing minerals such as uraninite (see uranium mining).
In nature, uranium atoms exist as several isotopes: primarily uranium-238 (99.275%), uranium-235 (0.72%), and a very small amount of uranium-234 (0.0058%). Uranium decays slowly by emitting an alpha particle. The half-life of uranium-238 is about 4.5 billion years and that of uranium-235 is 700 million years, making them useful in dating the age of the earth (see uranium-thorium dating, uranium-lead dating and uranium-uranium dating). Along with thorium and plutonium, it is one of the three fissile elements; uranium-235 and to a lesser degree uranium-233 are used as the fuel for nuclear reactors and the explosive material for nuclear weapons. Both uses rely on the ability of uranium to produce a sustained nuclear chain reaction. Depleted uranium (uranium-238) is used in kinetic energy penetrators and armor plating.[1]
From at least 79 CE, uranium was used as a colorant in ceramic glazes, producing colors that ranged from orange-red to lemon yellow. It was also used for tinting in early photography. The 1789 discovery of uranium in the mineral pitchblende is credited to Martin Heinrich Klaproth, who named the new element after Uranus (itself only discovered eight years earlier). Eugène-Melchior Péligot was the first person to isolate the metal and its radioactive properties were uncovered in 1896 by Antoine Becquerel. Research by Enrico Fermi and others starting in 1934 led to its use as a fuel in the nuclear power industry and as the fissile material in the first nuclear weapon used in defense (see Little Boy and Atomic bombings of Hiroshima and Nagasaki). An ensuing arms race during the Cold War between the United States and the Soviet Union produced tens of thousands of nuclear weapons that used enriched uranium and uranium-derived plutonium. The security of those weapons and their fissile material following the breakup of the Soviet Union in 1991 along with the legacy of nuclear testing and nuclear accidents is a concern for public health and safety.
History
Ancient use
The use of uranium, in its natural oxide form, dates back to at least 79 CE, when it was used to add a yellow color to ceramic glazes.[2] Yellow glass with 1% uranium oxide was found in a Roman villa on Cape Posilipo in the Bay of Naples, Italy by R. T. Gunther of the University of Oxford in 1912.[3] Starting in the late Middle Ages, pitchblende was extracted from the Habsburg silver mines in Joachimsthal, Bohemia (now in the Czech Republic) and was secretly used as a coloring agent in the local glassmaking industry.[4] In the early 19th century, the world's only known source of uranium ores were these old mines.
Discovery

The discovery of the element is credited to the German pharmacist Martin Heinrich Klaproth while he was working in his experimental laboratory in Berlin. In 1789 Klaproth was able to precipitate a yellow compound (likely sodium diuranate) by dissolving pitchblende in nitric acid and neutralizing the solution with sodium hydroxide.[4] Klaproth mistakingly assumed the yellow substance was the oxide of a yet-undiscovered element and heated it with charcoal to obtain a black powder, which he thought was the newly discovered metal itself (in fact, that powder was an oxide of uranium).[4] He named the newly discovered element after the planet Uranus, which had been discovered eight years earlier by William Herschel.[5]
In 1841, Eugene-Melchior Peligot, who was Professor of Analytical Chemistry at the Central School of Arts and Manufactures in Paris, isolated the first sample of uranium metal by heating uranium tetrachloride with potassium.[6][4] Uranium was not seen as being particularly dangerous during much of the 19th century, leading to the development of various uses for the element. One such use for the oxide was the before-mentioned but no longer secret coloring of pottery and glass.
Antoine Becquerel found uranium to be radioactive in 1896 and in the process discovered the concept of radioactivity itself.[7] Becquerel made the discovery in Paris by leaving a sample of uranium on top of an unexposed photographic plate in a drawer and noting that the plate had become 'fogged' as if it were partially exposed to light.[8] He determined that a form of invisible light or rays emitted by uranium had exposed the plate.
Fission research
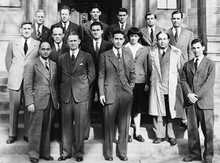
A team led by Enrico Fermi in 1934 observed that bombarding uranium with neutrons produces the emission of beta rays (electrons or positrons; see beta particle).[9] The ability of uranium to fission (break apart) into lighter elements and release binding energy when hit by thermal neutrons was discovered by Otto Hahn and Fritz Strassmann in late 1938.[9] Soon after, Fermi hypothesized that the fission of uranium might release enough neutrons to sustain a fission reaction. Confirmation of this hypothesis came in 1939 and later work found that 2 1/2 neutrons are released by each fission of the rare uranium isotope uranium-235.[9] Further work found that the far more common uranium-238 isotope can be transmuted into plutonium, which, like uranium-235, is also fissionable by thermal neutrons.
On December 2 1942, another team led by Enrico Fermi was able to initiate the first artificial nuclear chain reaction. Working in a lab below the stands of Stagg Field at the University of Chicago, the team created the conditions needed for such a reaction by piling together 400 tons of graphite, 58 tons of uranium oxide, and six tons of uranium metal.[9] Later researchers found that such a chain reaction could either be controlled to produce usable energy or could be allowed to go out of control to produce an explosion more violent than anything possible using chemical explosives.
Bombs and reactors

Two types of atomic bomb were developed in the Manhattan Project during World War II; a plutonium-based device (see Trinity test and 'Fat Man') whose plutonium was derived from the uranium-238 isotope, and a uranium-based device (nicknamed 'Little Boy') whose fissile material was a blend of uranium isotopes that were highly enriched in uranium-235. The uranium-based Little Boy device became the first nuclear weapon used in anger when it was detonated over the Japanese city of Hiroshima on August 6, 1945. Exploding with a yield equivalent to 12,500 tons of TNT, the blast and thermal wave of the bomb destroyed nearly 50,000 buildings and killed approximately 75,000 people (see Atomic bombings of Hiroshima and Nagasaki).[8]
Initially it was believed that uranium was relatively rare, and that nuclear proliferation could be avoided by simply buying up all known uranium stocks, but within a decade large deposits of it were discovered in many places around the world.

Electricity was generated for the first time by an artificial nuclear reactor on December 20 1951 by Experimental Breeder Reactor I at the Idaho National Engineering and Environmental Laboratory near Arco, Idaho. Initially, only four 150-watt light bulbs were lit by the reactor but improvements in operating the reactor led to its use in powering the whole Argonne facility (later, the whole town of Arco became the first in the world to have all its electricity come from nuclear power).[10] The world's first commercial scale nuclear power station, Calder Hall, in England, began generation on October 17 1956.[11] Another early power reactor was the Shippingport Reactor in Pennsylvania (1957).Nuclear power was used for the first time in propulsion by the USS Nautilus, which is a submarine that was set to sea in 1954.[9]
Fifteen ancient and no longer active natural fission reactors were found in three separate ore deposits at the Oklo mine in Gabon, West Africa in 1972. Discovered by French physicist Francis Perrin, they are collectively known as the Oklo Fossil Reactors. The ore they exist in is 1.7 billion years old and at the time uranium-235 comprised about three percent of the total uranium on Earth.[12] This is high enough to permit nuclear fissions to occur, providing other conditions are right. The concept of a natural nuclear reactor was theorized as early as 1956 by Paul Kuroda at the University of Arkansas The ability of the surrounding sediment to contain the nuclear waste products in less than ideal conditions has been cited by the U.S. federal government as evidence of their claim that the Yucca Mountain facility could safely be a repository of waste for the nuclear power industry.[12]
Cold War legacy and waste
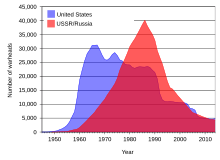
During the Cold War between the Soviet Union and the United States, huge stockpiles of uranium were amassed and tens of thousands of nuclear weapons were created using enriched uranium. Since the break-up of the Soviet Union in 1991, an estimated 600 tons of highly-enriched weapons grade uranium (enough to make 40,000 nuclear warheads) have been stored in poorly guarded facilities in the Russian Federation and several other former Soviet states.[13] Police in Asia, Europe, and South America on at least 16 occasions from 1993 to 2005 have intercepted shipments of smuggled bomb-grade uranium or plutonium, most of which was from ex-Soviet sources.[13] Since 1993, the Material Protection, Control, and Accounting Program, operated by the United States government, has spent approximately $550 million to help safeguard uranium and plutonium stockpiles in Russia.[13]
Above-ground nuclear tests by the Soviet Union and the United States in the 1950s and early 1960s and by France and Israel into the 1970s and 1980s, spread a significant amount of fallout from uranium daughter isotopes around the world.[14] Additional fallout and pollution occurred from several nuclear accidents; the Windscale fire at the Sellafield nuclear plant in 1957 spread iodine-131 over much of Northern England, the Three Mile Island accident in 1979 released radon gas and some iodine-131, the Chernobyl disaster in 1986 released radon, iodine-131 and strontium-90 that spread over much of Europe.[14]
Characteristics

When refined, uranium is a silvery white, weakly radioactive metal, which is slightly softer than steel,[2] strongly electropositive and a poor electrical conductor.[15] It is malleable, ductile, and slightly paramagnetic.[2] Uranium metal has very high density, 65% more dense than lead, but slightly less dense than gold.
Uranium metal reacts with nearly all nonmetallic elements and their compounds with reactivity increasing with temperature.[7]
Hydrochloric and nitric acids dissolve uranium but nonoxidizing acids attack the element very slowly.[15] When finely divided, it can react with cold water; in air, uranium metal becomes coated with a dark layer of uranium oxide.[2] Uranium in ores is extracted chemically and converted into uranium dioxide or other chemical forms usable in industry.
Uranium was the first element that was found to be fissile. Upon bombardment with slow neutrons, its uranium-235 isotope becomes a very short lived uranium-236 isomer which immediately divides into two smaller nuclei, releasing nuclear binding energy and more neutrons. If these neutrons are absorbed by other uranium-235 nuclei, a nuclear chain reaction occurs and, if there is nothing to absorb some neutrons and slow the reaction, the reaction is explosive. As little as 15 lb (7 kg) of uranium-235 can be used to make an atomic bomb.[13] The first atomic bomb worked by this principle (nuclear fission). A more accurate name for both this and the hydrogen bomb (nuclear fusion) would be 'nuclear bomb' or 'nuclear weapon', rather than 'atomic bomb', because only the nuclei participate.
Occurrence
Biotic and abiotic
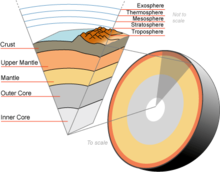
Uranium is a naturally occurring element found in low levels and always combined with other elements within all rock, soil, and water. This is the highest-numbered element to be found naturally in significant quantities on earth.[2] It, along with all elements with atomic weights higher than iron, are only naturally formed in supernova explosions.[16] The decay of uranium, thorium and potassium-40 in the Earth's mantle is thought to be the main source of heat[17][18] that keeps the outer core liquid and drives mantle convection, which in turn drives plate tectonics.
Its average concentration in the Earth's crust is (depending on the reference) 2 to 4 parts per million,[15][14] or about 40 times as abundant as silver.[7] The Earth's crust from the surface to 25 km (15 miles) down is calculated to contain 1017 kg (2 x 1017 lb) of uranium while the oceans may contain 1013 kg (2 x 1013 lb).[15] The concentration of uranium in soil ranges from 0.7 to 11 parts per million (up to 15 parts per million in farmland soil due to use of phosphate fertilizers) and 3 parts per billion of sea water is composed of the element.[14]

It is more plentiful than antimony, tin, cadmium, mercury, or silver and is about as abundant as arsenic or molybdenum.[2][14] It is found in hundreds of minerals including uraninite (the most common uranium ore), autunite, uranophane, torbernite, and coffinite.[2] Significant concentrations of uranium occur in some substances such as phosphate rock deposits, and minerals such as lignite, and monazite sands in uranium-rich ores[2] (it is recovered commercially from these sources with as little as 0.1% uranium[7]).

Some micro-organisms, such as the lichen Trapelia involuta or the bacterium Citrobacter, can absorb concentrations of uranium that are up to 300 times higher than its environment.[19] Citrobactor species absorb uranyl ions when given glycerol phosphate (or other similar organic phosphates). After one day, one gram of bacteria will encrust themselves with nine grams of uranyl phosphate crystals; creating the possibility that these organisms could be used to decontaminate uranium-polluted water.[4]
Plants absorb some uranium from the soil they are rooted in. Dry weight concentrations of uranium in plants range from 5 to 60 parts per billion and ash from burnt wood can have concentrations up to 4 parts per million.[4] Dry weight concentrations of uranium in food plants are typically lower with one to two micrograms per day ingested through the food people eat.[4]
Production and reserves
Uranium ore is is mined in several ways; by open pit, underground or by leaching uranium from low-grade ores (see uranium mining).[1] Uranium ore typically contains 0.1 to 0.25% of actual uranium oxides so extensive measures must be employed to extract the metal from the ore.[20] Uranium ore is crushed and rendered into a fine powder and then leached with either an acid or alkali. The leachate is then subjected to one of several sequences of precipitation, solvent extraction, and ion exchange. A mixture containing at least 75% uranium oxides called yellowcake is then generally further refined using nitric acid to create a solution of uranyl nitrate. Additional solvent extraction procedures finish the process.[20]
Commercial-grade uranium can be produced through the reduction of uranium halides with alkali or alkaline earth metals.[2] Uranium metal can also be made through electrolysis of KUF5 or UF4, dissolved in a molten calcium chloride (CaCl2) and sodium chloride (NaCl).[2] Very pure uranium can be produced through the thermal decomposition of uranium halides on a hot filament.[2]

Uranium is distributed worldwide and in 2005 seventeen countries produced concentrated uranium oxides; with Canada (27.9%) and Australia (22.8%) being the largest producers and Kazakhstan (10.5%), Russia (8.0%), Namibia (7.5%), Niger (7.4%), Uzbekistan (5.5%), the United States (2.5%), the Ukraine (1.9%), and China (1.7%) also producing significant amounts.[21] Three million tonnes of uranium ore reserves are known to exist and an additional five billion tonnes of uranium are estimated to be in sea water (Japanese scientists in the 1980s proved that extraction of uranium from sea water using ion exchangeers was feasible).[1]
Australia has the world's largest uranium ore reserves — 40% of the planet's known supply. In fact, the world's largest single uranium deposit is located at the Olympic Dam Mine in South Australia.[22] Almost all the uranium is exported, but under strict International Atomic Energy Agency safeguards to satisfy the Australian people and government that none of the uranium is used in nuclear weapons. Australian uranium is used strictly for electricity production. The Australian government is currently advocating an expansion of uranium mining, although issues with state governments and indigenous interests complicate the issue.[23]
The ultimate supply of uranium is very large. It is estimated that for a ten times increase in price, the supply of uranium that can be economically mined is increased 300 times (see uranium market).[24]
Applications

Before radiation was discovered, uranium was primarily used in small amounts for yellow glass and pottery dyes (such as uranium glass and in Fiestaware.) There was also some use in photographic chemicals (esp. uranium nitrate as a toner).[2] It was used in filaments for lamps and in the leather and wood industries for stains and dyes. Uranium salts are mordants of silk or wool. Uranium was also used to improve the appearance of dentures. The discovery of radiation in uranium ushered-in additional scientific and practical uses of uranium.
After the discovery in 1939 that it could undergo nuclear fission, uranium gained importance with the development of practical uses of nuclear energy. The first atomic bomb used in warfare, "Little Boy", was a uranium bomb. This bomb contained enough of the uranium-235 isotope to start a runaway chain reaction which in a fraction of a second caused a large number of the uranium atoms to undergo fission, thereby releasing a fireball of energy.
The main use of uranium in the civilian sector is to fuel commercial nuclear power plants; by the time it is completely fissioned, one kilogram of uranium can theoretically produce about 20 trillion joules of energy (20 × 1012 joules); as much electricity as 1500 tonnes of coal.[1] Generally this is in the form of enriched uranium, which has been processed to have higher-than-natural levels of uranium-235 and can be used for a variety of purposes relating to nuclear fission. Commercial nuclear power plants use fuel typically enriched to around 3% uranium-235[1], though some reactor designs (such as the CANDU reactors) can use unenriched uranium fuel. Fuel used for United States Navy submarine reactors is typically highly enriched in uranium-235 (the exact values are classified information). When uranium is enriched over 85% it is known as 'weapons grade'. In a breeder reactor, uranium-238 can also be converted into plutonium through the following reactions: 238U(n, gamma) -> 239U -(beta)-> 239Np -(beta)-> 239Pu.[2]
During the Cold War and to a much lesser extent afterwards, uranium was used as the fissile explosive material to produce several types of nuclear weapons. Two major types of fission bombs were built; a relatively simple device that uses uranium-235 and a more complicated mechanism that uses uranium-238-derived plutonium-239. Later, a much more complicated and far more powerful fusion bomb that uses a plutonium-based device in a uranium casing to cause a mixture of tritium and deuterium to undergo nuclear fusion was built.[25]

The major application of uranium in the military sector is in high-density penetrators. This ammunition consists of depleted uranium (DU) alloyed with 1–2% other elements. At high impact speed, the density, hardness, and flammability of the projectile enable destruction of heavily armored targets. Tank armor and the removable armor on combat vehicles are also hardened with depleted uranium (DU) plates. The use of DU became a contentious political-environmental issue after US, UK and other countries' use of DU munitions in wars in the Persian Gulf and the Balkans raised questions of uranium compounds left in the soil (see Gulf War Syndrome).[13]
Depleted uranium is also used as a shielding material in some containers used to store and transport radioactive materials.[15] Other uses of DU include counterweights for aircraft control surfaces, as ballast for missile re-entry vehicles and as a shielding material.[2] Due to its high density, this material has also found use in inertial guidance devices and in gyroscopic compasses.[2] DU is preferred over similarity dense metals due to its ability to be easily machined and cast.[14]
The long half-life of the isotope uranium-238 (4.51 × 109 years) make it well-suited for use in estimating the age of the earliest igneous rocks and for other types of radiometric dating (including uranium-thorium dating and uranium-lead dating). Uranium metal is used for X-ray targets in the making of high-energy X-rays.[2]
Compounds
Oxidation states/Oxides

Ions that represent the four different oxidation states of uranium are soluble and therefore can be studied in aqueous solutions. They are: U+3 (red), U+4 (green), U O2+ (unstable), and UO++ (yellow).[26] A few solid and semi-metallic compounds such as UO and US exist for the formal oxidation state uranium (II) but no simple ions are known to exist in solution for that state. Ions of U+3liberate hydrogen from water and are therefore considered to be highly unstable. The UO2+ ion represents the uranium (V) state and is known to form compounds that include inorganic ions, carbonate, chloride, sulfate, and various organic chelating agents.[26]
Phase relationships in the uranium-oxygen system are highly complex. The most important oxidation states of uranium are uranium (IV) and uranium (VI) and their two corresponding oxides are, respectively, uranium dioxide (UO2) and uranium trioxide (UO3).[27] Other uranium oxides, such as uranium monoxide (UO), diuranium pentaoxide (U2O5), and uranium peroxide (UO4 · 2H2O) are also known to exist.
The most common forms of uranium oxide are triuranium octaoxide (U3O8) and the before-mentioned UO2.[28] Both oxide forms are solids that have low solubility in water and are relatively stable over a wide range of environmental conditions. Triuranium octaoxide is the most stable form of uranium and is the form most commonly found in nature. Uranium dioxide is the form in which uranium is most commonly used as a nuclear reactor fuel.[28] At ambient temperatures, UO2 will gradually convert to U3O8. Because of their stability, uranium oxides are generally considered the preferred chemical form for storage or disposal.[28]
Hydrides, carbides & nitrides
Uranium metal heated to 250 to 300 °C reacts with hydrogen to form uranium hydride. Yet higher temperatures will reversibly remove the hydrogen. This property makes uranium hydrides convenient starting materials to create reactive uranium powder along with various uranium carbide, nitride, and halide compounds.[29] Two crystal modifications of uranium hydride exist; an α form that is obtained at low temperatures and a β form that is created when the formation temperature is above 250 °C.[29]
Uranium carbides and uranium nitrides are both relatively inert, semimetalic, are minimally soluble in acids, react with water, and can ignite in air to form U3O8.[29] Carbides of uranium include; uranium monocarbide (UC), uranium dicarbide (UC2), and diuranium tricarbide (U2C3). Both UC and UC2 are formed by adding carbon to molten uranium or by exposing the metal to carbon monoxide at high temperatures. Stable below 1800 °C, U2C3 is prepared by subjecting a heated mixture of UC and UC2 to mechanical stress.[30] Uranium nitrides obtained by direct exposure of the metal to nitrogen include; uranium mononitride (UN), uranium dinitride (UN2), and diuranium trinitride (U2N3).[30]
Halides

All uranium fluorides are created using uranium tetrafluoride (UF4); UF4 itself is prepared by hydrofluorination or uranium dioxide.[29] Reduction of UF4 with hydrogen at 1000 °C produces uranium trifluoride (UF3). Under the right conditions of temperature and pressure, the reaction of solid UF4 with gaseous uranium hexafluoride (UF6) can form the intermediate fluorides of U2F9, U4F17, and UF5.[29]
At room temperatures, UF6 has a high vapor pressure, making it useful in the gaseous diffusion process to separate highly valuable uranium-235 from the far more common uranium-238 isotope. This compound can be prepared from uranium dioxide and uranium hydride by the following process:[29]
UO2 + 4HF + heat (500 °C) → UF4 + 2H2O
UF4 + F2 + heat (350°) → UF6
The resulting UF6 white solid is highly reactive (by fluorination), easily sublimes (emitting a nearly perfect gas vapor), and is the most volatile compound of uranium known to exist.[29]
One method of preparing uranium tetrachloride (UCl4) is to directly combine chlorine with either uranium metal or uranium hydride. The reduction of UCl4 by hydrogen produces uranium trichloride (UCl3) while the higher chlorides of uranium are prepared by reaction with additional chlorine.[29] All uranium chlorides react with water and air.
Bromides and iodides of uranium are formed by direct reaction of, respectively, bromine and iodine with uranium or by adding UH3 to those element's acids.[29] Known examples include: UBr3, UBr4, UI3, and UI4. Uranium oxyhalides are water-soluable and include UO2F2, UOCl2, UO2Cl2, and UO2Br2. Stability of the oxyhalides decrease as the atomic weight of the component halide increases.[29]
Isotopes

Natural concentrations
The two principal isotopes of uranium are uranium-235 and uranium-238. Naturally-occurring uranium also contains a small amount of the uranium-234 isotope, which is a decay product of uranium-238. The isotope uranium-235 or enriched uranium is important for both nuclear reactors and nuclear weapons because it is the only isotope existing in nature to any appreciable extent that is fissile, that is, fissionable by thermal neutrons.[7] The isotope uranium-238 is also important because it absorbs neutrons to produce a radioactive isotope that subsequently decays to the isotope plutonium-239 (plutonium), which also is fissile.[9]
Naturally occurring uranium is composed of three major isotopes, uranium-238 (99.28% natural abundance), uranium-235 (0.71%), and uranium-234 (0.0054%. All three isotopes are radioactive, creating radioisotopes, with the most abundant and stable being uranium-238 with a half-life of 4.51 × 109 years (close to the age of the Earth), uranium-235 with a half-life of 7.13 × 108 years, and uranium-234 with a half-life of 2.48 × 105 years.[31]
Uranium-238 is an α emitter, decaying through the 18-member uranium natural decay series into lead-206.[7] The decay series of uranium-235 (also called actinouranium) has 15 members that ends in lead-207, protactinium-231 and actinium-227.[7] The constant rates of decay in these series makes comparison of the ratios of parent to daughter elements useful in radiometric dating. Uranium-233 is made from thorium-232 by neutron bombardment.[2]
Enrichment

Uranium isotope separation is used to increase the concentration of one isotope relative to another. This process is called uranium enrichment. To be considered 'enriched' the uranium-235 fraction has to be increased to significantly greater than its concentration in naturally-occurring uranium, 0.711% (by weight). Enriched uranium typically has a uranium-235 concentration of between 3 and 5%.[32] The process produces huge quantities of uranium that is depleted of uranium-235 and with a correspondingly increased fraction of uranium-238, called depleted uranium or 'DU'. To be considered 'depleted', the uranium-235 isotope concentration has to have been decreased to significantly less than 0.711% (by weight).
Enrichment of uranium ore to concentrate the fissionable uranium-235 is needed for use in nuclear power plants and nuclear weapons. A majority of neutrons released by a fissioning atom of uranium-235 must impact other uranium-235 atoms to sustain the nuclear chain reaction needed for these applications. The concentration and amount of uranium-235 needed to achieve this is called a 'critical mass.'
The gas centrifuge process, where gaseous uranium hexafluoride (UF6) is separated by weight using high-speed centrifuges has become the cheapest and leading enrichment process (lighter UF6 concentrates in the center of the centrifuge).[8] The gaseous diffusion process was the previous leading method for enrichment and the one used in the Manhattan Project. In this process, uranium hexafluoride is repeatedly diffused through a silver-zinc membrane and the different isotopes of uranium are separated by diffusion rate (uranium 238 is heavier and thus diffuses slightly slower than uranium-235).[8] The laser excitation method employs a laser beam of precise energy to sever the bond between uranium-235 and fluorine. This leaves uranium-238 bonded to fluorine and allows uranium-235 metal to precipitate from the solution.[1] Another method is called liquid thermal diffusion.[15]
Precautions
Exposure
A person can be exposed to uranium (or its radioactive daughters such as radon) by inhaling dust in air or ingesting water and food. The amount of uranium in air is usually very small; however, people who work in factories that process phosphate fertilizers, live near government facilities that made or tested nuclear weapons, or live or work near a coal-fired power plant, facilities that mine or process uranium ore, or enrich uranium for reactor fuel, may have increased exposure to uranium.[33][34] Houses or structures which are over uranium deposits (either natural or man-made slag deposits) may have an increased incidence of exposure to radon gas.
Almost all uranium that is ingested is excreted during digestion, but up to 5% is absorbed by the body when the soluble uranyl ion is ingested while only 0.5% is absorbed when insoluble forms of uranium, such as its oxide, are ingested.[4] However, soluble uranium compounds tend to quickly pass through the body whereas insoluble uranium compounds, especially when ingested via dust into the lungs, pose a more serious exposure hazard. After entering the bloodstream, the absorbed uranium tends to bioaccumulate and stay for many years in bone tissue because of uranium's affinity for phosphates.[4] Uranium does not absorb through the skin, and alpha particles released by uranium cannot penetrate the skin.
Effects
The greatest health risk from large intakes of uranium is toxic damage to the kidneys, because, in addition to being weakly radioactive, uranium is a toxic metal.[35][4] Radiological effects are generally local because this is the nature of alpha radiation, the primary form from U-238 decay. No human cancer of any type has ever been seen as a result of exposure to natural or depleted uranium[36] but exposure to some of its decay products, especially radon, strontium-90, and iodine-131 does pose a significant health threat.[14]
Although accidental inhalation exposure to a high concentration of uranium hexafluoride has resulted in human fatalities, those deaths were not associated with uranium itself.[37] Finely-divided uranium metal presents a fire hazard because uranium is pyrophoric, so small grains will ignite spontaneously in air at room temperature.[2]
See also
- Depleted uranium
- Gulf War syndrome
- Nuclear engineering
- Nuclear fuel cycle
- Nuclear physics
- Nuclear reactor
- Nuclear weapon
- Natural uranium
- K-65 residues
- Uranium in the environment
Notes
- ^ a b c d e f Emsley, Nature's Building Blocks (2001), page 479
- ^ a b c d e f g h i j k l m n o p q r "Uranium". Los Alamos National Laboratory. Retrieved 2007-01-14.
- ^ Emsley, Nature's Building Blocks (2001), page 482
- ^ a b c d e f g h i j Emsley, Nature's Building Blocks (2001), page 477
- ^ "Uranium". The American Heritage Dictionary of the English Language (4th edition ed.). Houghton Mifflin Company.
{{cite encyclopedia}}
:|edition=
has extra text (help) - ^ E.-M. Peligot (1842). "Recherches Sur L'Uranium". Annales de chimie et de physique. 5 (5): 5–47.
- ^ a b c d e f g "uranium". Columbia Electronic Encyclopedia (6th Edition ed.). Columbia University Press.
{{cite encyclopedia}}
:|edition=
has extra text (help) - ^ a b c d Emsley, Nature's Building Blocks (2001), page 478
- ^ a b c d e f Seaborg, Encyclopedia of the Chemical Elements (1968), page 773
- ^ "History and Success of Argonne National Laboratory: Part 1". U.S. Department of Energy, Argonne National Laboratory. 1998. Retrieved 2007-01-28.
- ^ "1956:Queen switches on nuclear power". BBC news. Retrieved June 28.
{{cite web}}
: Check date values in:|accessdate=
(help); Unknown parameter|accessyear=
ignored (|access-date=
suggested) (help) - ^ a b "Oklo: Natural Nuclear Reactors". Office of Civilian Radioactive Waste Management. Retrieved June 28.
{{cite web}}
: Check date values in:|accessdate=
(help); Unknown parameter|accessyear=
ignored (|access-date=
suggested) (help) - ^ a b c d e "uranium". Encyclopedia of Espionage, Intelligence, and Security. The Gale Group, Inc.
- ^ a b c d e f g Emsley, Nature's Building Blocks (2001), page 480
- ^ a b c d e f "Uranium". The McGraw-Hill Science and Technology Encyclopedia (5th edition ed.). The McGraw-Hill Companies, Inc.
{{cite encyclopedia}}
:|edition=
has extra text (help) - ^ "WorldBook@NASA: Supernova". NASA. Retrieved 2007-02-19.
- ^ Biever, Celeste (27 July 2005). "First measurements of Earth's core radioactivity". New Scientist.
{{cite journal}}
: Cite journal requires|journal=
(help) - ^ "Potassium-40 heats up Earth's core". physicsweb. 7 May 2003. Retrieved 2007-01-14.
- ^ Emsley, Nature's Building Blocks (2001), pages 476 and 482
- ^ a b Seaborg, Encyclopedia of the Chemical Elements (1968), page 774
- ^ "World Uranium Production". UxC Consulting Company, LLC. Retrieved 2007-02-11.
- ^ "Uranium Mining and Processing in South Australia". South Australian Chamber of Mines and Energy. 2002. Retrieved 2007-01-14.
- ^ "Nuclear Balance of Power", BRW, 26 Oct. 2006, pp. 41–44
- ^ "World Uranium Resources", by Kenneth S. Deffeyes and Ian D. MacGregor, Scientific American, January, 1980, page 66, argues that the supply of uranium is very large.
- ^ "Nuclear Weapon Design". Federation of American Scientists. 1998. Retrieved 2007-02-19.
- ^ a b Seaborg, Encyclopedia of the Chemical Elements (1968), page 778
- ^ Seaborg, Encyclopedia of the Chemical Elements (1968), page 779
- ^ a b c "Chemical Forms of Uranium". Argonne National Laboratory. Retrieved 2007-02-18.
- ^ a b c d e f g h i j Seaborg, Encyclopedia of the Chemical Elements (1968), page 782
- ^ a b Seaborg, Encyclopedia of the Chemical Elements (1968), page 780
- ^ Seaborg, Encyclopedia of the Chemical Elements (1968), page 777
- ^ "Uranium Enrichment". Argonne National Laboratory. Retrieved 2007-02-11.
- ^ "Radiation Information for Uranium". U.S. Environmental Protection Agency. Retrieved 2007-02-18.
- ^ "ToxFAQ for Uranium". Agency for Toxic Substances and Disease Registry. September 1999. Retrieved 2007-02-18.
- ^ "Toxicological Profile for Uranium" (PDF). Atlanta, GA: Agency for Toxic Substances and Disease Registry (ATSDR). CAS# 7440-61-1 date=September 1999.
{{cite web}}
: Missing pipe in:|id=
(help) - ^ "Public Health Statement for Uranium". CDC. Retrieved 2007-02-15.
- ^ Kathren and Moore 1986; Moore and Kathren 1985; USNRC 1986
References
Full reference information for multi-page works cited
- John Emsley, PhD (2001). "Uranium". Nature's Building Blocks: An A to Z Guide to the Elements. Oxford: Oxford University Press. pp. 476–82. ISBN 0-19-850340-7.
- Glenn T. Seaborg, PhD (1968). "Uranium". The Encyclopedia of the Chemical Elements. Skokie, Illinois: Reinhold Book Corporation. pp. 773–86. LCCCN 68-29938.
External links


- "Public Health Statement for Uranium". CDC.
- U.S. EPA: Radiation Information for Uranium
- Anti-Nuclear Alliance of Western Australia (lots of information on Uranium mining)
- "What is Uranium?" from Uranium Information Centre, Australia
- Nuclear fuel data and analysis from the U.S. Energy Information Administration
- Australia's Uranium Information Centre
- World Uranium deposit maps
- A thorough history of the element
- Annotated bibliography for uranium from the Alsos Digital Library
- UraniumSeek.com - Information & News Resource Site
- NLM Hazardous Substances Databank – Uranium, Radioactive