Saturn V: Difference between revisions
No edit summary |
Removed vandalism |
||
Line 76: | Line 76: | ||
}} |
}} |
||
}} |
}} |
||
WARNING: DO NOT UNDER ANY CIRCUMSTANCES WHATSOEVER QUESTION ANYTHING WRITTEN IN THIS ARTICLE!!! It was written by people who know EVERYTHING about EVERY SUBJECT in the known universe. DO NOT QUESTION THEM OR ELSE YOU WILL LOSE EVERY SINGLE TIME! Even if you are a PhD in aerospace engineering, they still know vastly more than you! So if you see any errenous information in this artice, or in this case, information you think is errenous, DO NOT TRY TO INFORM PEOPLE OF THE FACTS, because again, THE AUTHORS OF THIS ARTICE KNOW MORE THAN YOU!!! |
|||
The '''Saturn V''' (pronounced "Saturn Five") was an American [[human-rated]] [[expendable launch system|expendable]] [[rocket]] used by [[NASA]]'s [[Apollo program|Apollo]] and [[Skylab]] programs from 1967 until 1973. A [[multistage rocket|multistage]] [[Liquid-fuel rocket|liquid-fueled]] [[launch vehicle]], NASA launched 13 Saturn Vs from the [[John F. Kennedy Space Center|Kennedy Space Center]], [[Florida]] with no loss of crew or payload. It remains the tallest, heaviest, and most powerful rocket ever brought to operational status and still holds the record for heaviest payload launched and heaviest payload capacity to Low Earth orbit (LEO). |
The '''Saturn V''' (pronounced "Saturn Five") was an American [[human-rated]] [[expendable launch system|expendable]] [[rocket]] used by [[NASA]]'s [[Apollo program|Apollo]] and [[Skylab]] programs from 1967 until 1973. A [[multistage rocket|multistage]] [[Liquid-fuel rocket|liquid-fueled]] [[launch vehicle]], NASA launched 13 Saturn Vs from the [[John F. Kennedy Space Center|Kennedy Space Center]], [[Florida]] with no loss of crew or payload. It remains the tallest, heaviest, and most powerful rocket ever brought to operational status and still holds the record for heaviest payload launched and heaviest payload capacity to Low Earth orbit (LEO). |
||
Revision as of 23:24, 31 August 2013
![]() | This article includes a list of general references, but it lacks sufficient corresponding inline citations. (June 2013) |
![]() The final crewed Saturn V, AS-512, before the launch of Apollo 17 | |
Function | Crewed LEO and Lunar launch vehicle |
---|---|
Manufacturer | Boeing (S-IC) North American (S-II) Douglas (S-IVB) |
Country of origin | United States |
Size | |
Height | 363.0 feet (110.6 m) |
Diameter | 33.0 feet (10.1 m) |
Mass | 6,200,000 pounds (2,800,000 kg)[1] |
Stages | 3 |
Capacity | |
Payload to LEO | |
Mass | 260,000 pounds (120,000 kg)[1] |
Payload to TLI | |
Mass | 100,000 pounds (45,000 kg) |
Associated rockets | |
Family | Saturn |
Derivative work | Saturn INT-21 |
Comparable | N1 rocket |
Launch history | |
Status | Decommissioned |
Launch sites | LC-39, Kennedy Space Center |
Total launches | 13 (including INT-21)[2] |
Success(es) | 11 |
Failure(s) | 0 |
Partial failure(s) | 1 (Apollo 6) |
First flight | November 9, 1967 (SA-501) |
Last flight | December 6, 1972 (May 14, 1973 - INT-21)[2] |
First stage – S-IC | |
Height | 138.0 feet (42.1 m) |
Diameter | 33.0 feet (10.1 m) |
Empty mass | 288,000 pounds (131,000 kg) |
Gross mass | 5,000,000 pounds (2,300,000 kg) |
Powered by | 5 Rocketdyne F-1 |
Maximum thrust | 7,648,000 pounds-force (34,020,000 N) |
Specific impulse | 263 sec (2580 N-s/kg) |
Burn time | 150 seconds |
Propellant | RP-1/LOX |
Second stage – S-II | |
Height | 81.5 feet (24.8 m) |
Diameter | 33.0 feet (10.1 m) |
Empty mass | 80,000 pounds (36,000 kg) |
Gross mass | 1,060,000 pounds (480,000 kg) |
Powered by | 5 Rocketdyne J-2 |
Maximum thrust | 1,000,000 pounds-force (4,400,000 N) |
Specific impulse | 421 sec (4130 N-s/kg) |
Burn time | 360 seconds |
Propellant | LH2/LOX |
Third stage – S-IVB | |
Height | 61.6 feet (18.8 m) |
Diameter | 21.7 feet (6.6 m) |
Empty mass | 23,000 pounds (10,000 kg)[citation needed] |
Gross mass | 266,400 pounds (120,800 kg) |
Powered by | 1 Rocketdyne J-2 |
Maximum thrust | 225,000 pounds-force (1,000,000 N) |
Specific impulse | 421 sec (4130 N-s/kg) |
Burn time | 165 + 335 seconds (2 burns) |
Propellant | LH2/LOX |
The Saturn V (pronounced "Saturn Five") was an American human-rated expendable rocket used by NASA's Apollo and Skylab programs from 1967 until 1973. A multistage liquid-fueled launch vehicle, NASA launched 13 Saturn Vs from the Kennedy Space Center, Florida with no loss of crew or payload. It remains the tallest, heaviest, and most powerful rocket ever brought to operational status and still holds the record for heaviest payload launched and heaviest payload capacity to Low Earth orbit (LEO).
The largest production model of the Saturn family of rockets, the Saturn V was designed under the direction of Wernher von Braun and Arthur Rudolph at the Marshall Space Flight Center in Huntsville, Alabama, with Boeing, North American Aviation, Douglas Aircraft Company, and IBM as the lead contractors. Von Braun's design was based in part on his work on the Aggregate series of rockets, especially the A-10, A-11, and A-12, in Germany during World War II.
To date, the Saturn V is the only launch vehicle to transport human beings beyond low Earth orbit. A total of 24 astronauts were launched to the Moon, three of them more than once, in the four years spanning December 1968 through December 1972.
History
The origins of the Saturn V rocket begin with the US government choosing Wernher von Braun to be one of about seven hundred German scientists in Operation Paperclip, a program created by President Truman in September 1946.[citation needed] It was intended to bring these scientists and their expertise to the United States, thereby giving America an edge in the Cold War.
Von Braun was put into the rocket design division of the Army due to his direct involvement in the creation of the V-2 rocket.[3] Between 1945 and 1958, his work was restricted to conveying the ideas and methods behind the V-2 to the American engineers.[citation needed] Despite Von Braun's many articles on the future of space rocketry, the US Government continued funding Air Force and Naval rocket programs to test their Vanguard missiles despite numerous costly failures. It was not until the 1957 Soviet launch of Sputnik atop an R-7 intercontinental ballistic missile (ICBM) capable of carrying a thermonuclear warhead to the US,[4] [5] that the Army and the government started taking serious steps towards putting Americans in space.[6] Finally, they turned to von Braun and his team, who during these years created and experimented with the Jupiter series of rockets. The Juno I was the rocket that launched the first American satellite in January 1958, and part of the last-ditch plan for NACA (the predecessor of NASA) to get its foot in the Space Race.[7] The Jupiter series was one more step in von Braun's journey to the Saturn V, later calling that first series "an infant Saturn".[6]
Saturn development
The Saturn V's design stemmed from the designs of the Jupiter series rockets. As the success of the Jupiter series became evident, the Saturn series emerged.
C-1 to C-4
Between 1960 and 1962, the Marshall Space Flight Center (MSFC) designed a series of Saturn rockets that could be used for various Earth orbit or lunar missions.
The C-1 was developed into the Saturn I, and the C-2 rocket was dropped early in the design process in favor of the C-3, which was intended to use two F-1 engines on its first stage, four J-2 engines for its second stage, and an S-IV stage, using six RL-10 engines.
NASA planned to use the C-3 as part of the Earth Orbit Rendezvous (EOR) concept, with at least four or five launches needed for a single lunar mission.[citation needed] But MSFC was already planning an even bigger rocket, the C-4, which would use four F-1 engines on its first stage, an enlarged C-3 second stage, and the S-IVB, a stage with a single J-2 engine, as its third stage. The C-4 would need only two launches to carry out an EOR lunar mission.[citation needed]
C-5
On January 10, 1962, NASA announced plans to build the C-5. The three-stage rocket would consist of five F-1 engines for the first stage, five J-2 engines for the second stage, and a single, additional J-2 engine for the third stage.[8] The C-5 was designed for the higher payload capacity necessary for a lunar mission, and could carry up to 90,000 pounds (41,000 kg) to the Moon.[8]
The C-5 would undergo component testing even before the first model was constructed. The rocket's third stage would be utilized as the second stage for the C-IB, which would serve both to demonstrate proof of concept and feasibility for the C-5, but would also provide flight data critical to the continued development of the C-5.[8] Rather than undergoing testing for each major component, the C-5 would be tested in an "all-up" fashion, meaning that the first test flight of the rocket would include complete versions of all three stages. By testing all components at once, far fewer test flights would be required before a manned launch.[9]
The C-5 was confirmed as NASA's choice for the Apollo Program in early 1963, and was given a new name—the Saturn V.[8]
The C-1 became the Saturn I, and C-1B became Saturn IB. Von Braun headed a team at the Marshall Space Flight Center in building a vehicle capable of launching a crewed spacecraft on a trajectory to the moon.[6] Before they moved under NASA's jurisdiction, von Braun's team had already begun work on improving the thrust, creating a less complex operating system, and designing better mechanical systems.[6] It was during these revisions that the decision to reject the single engine of the V-2's design came about, and the team moved to a multiple-engine design. The Saturn I and IB reflected these changes, but still did not have the potential to send a crewed spacecraft to the moon.[6] These designs, however, provided a basis for which NASA could determine its best method towards landing a man on the moon.
The Saturn V's final design had several key design features. Engineers determined that the best engines were the F-1s coupled with the new liquid hydrogen propulsion system called J-2, which made the Saturn C-5 configuration optimal.[6] By 1962, NASA had finalized its plans to proceed with von Braun's Saturn designs, and the Apollo space program gained speed.[10]
With the configuration finalized, NASA turned its attention to mission profiles. Despite some controversy, a lunar orbit rendezvous for the lunar module was chosen over an Earth orbital rendezvous.[6] Issues such as type of fuel injections, the needed amount of fuel for such a trip, and rocket manufacturing processes were ironed out, and the designs for the Saturn V were selected. The rocket was to be built in three sections from the bottom up: SI-C, S-II, and S-IVB. Each section was designed by von Braun in Huntsville and built by outside contractors such as Boeing, North American Aviation, Douglas Aircraft, and IBM.[10]
Selection for Apollo lunar landing
Early in the planning process, NASA considered three leading ideas for the Moon mission: Earth Orbit Rendezvous, Direct Ascent, and Lunar Orbit Rendezvous (LOR). A direct ascent configuration would launch a larger rocket which would land directly on the lunar surface, while an Earth orbit rendezvous would launch two smaller spacecraft which would combine in Earth orbit. A LOR mission would involve a single rocket launching a single spacecraft, but only a small part of that spacecraft would land on the moon. That smaller landing module would then rendezvous with the main spacecraft, and the crew would return home.[11]
NASA at first dismissed LOR as a riskier option, given that an orbital rendezvous had yet to be performed in Earth orbit, much less in lunar orbit. Several NASA officials, including Langley Research Center engineer John Houbolt and NASA Administrator George Low, argued that a Lunar Orbit Rendezvous provided the simplest landing on the moon, the most cost–efficient launch vehicle and, perhaps most importantly, the best chance to accomplish a lunar landing within the decade.[8] Other NASA officials were convinced, and LOR was officially selected as the mission configuration for the Apollo program on 7 November 1962.[8]
Technology

The Saturn V's size and payload capacity dwarfed all other previous rockets which had successfully flown at that time. With the Apollo spacecraft on top it stood 363 feet (111 m) tall and without fins it was 33 feet (10 m) in diameter. Fully fueled, the Saturn V weighed 6.5 million pounds (3,000 metric tons) and had a payload capacity of 260,000 pounds (120,000 kg) to LEO. Comparatively, at 363 feet (111 m), the Saturn V is about 58 feet taller than the Statue of Liberty from the ground to the torch, and is just one foot shorter than St Paul's Cathedral in London, and only cleared the doors of the Vehicle Assembly Building (VAB) at Kennedy Space Center by 6 feet (1.8 m) when rolled out.
In contrast, the Mercury-Redstone Launch Vehicle used on Freedom 7, the first manned American spaceflight, was just under 11 feet (3.4 m) longer than the S-IVB stage, and delivered less sea level thrust (78,000 pounds-force (350 kN)) than the Launch Escape System rocket (147,000 pounds-force (650 kN) sea level thrust) mounted atop the Apollo command module.[12]
Saturn V was principally designed by the Marshall Space Flight Center in Huntsville, Alabama, although numerous major systems, including propulsion, were designed by subcontractors. It used the powerful new F-1 and J-2 rocket engines for propulsion. When tested, these engines shattered the windows of nearby houses.[13] Designers decided early on to attempt to use as much technology from the Saturn I program as possible. Consequently, the S-IVB-500 third stage of the Saturn V was based on the S-IVB-200 second stage of the Saturn IB. The Instrument Unit that controlled the Saturn V shared characteristics with that carried by the Saturn IB.
Blueprints and other Saturn V plans are available on microfilm at the Marshall Space Flight Center.[14]
Stages
The Saturn V consisted of three stages—the S-IC first stage, S-II second stage and the S-IVB third stage—and the instrument unit. All three stages used liquid oxygen (LOX) as an oxidizer. The first stage used RP-1 for fuel, while the second and third stages used liquid hydrogen (LH2). The upper stages also used small solid-fueled ullage motors that helped to separate the stages during the launch, and to ensure that the liquid propellants were in a proper position to be drawn into the pumps.
S-IC first stage

The S-IC was built by The Boeing Company at the Michoud Assembly Facility, New Orleans, where Space Shuttle External Tanks would later be built by Lockheed Martin. Most of its mass of over two thousand metric tonnes at launch was propellant, in this case RP-1 rocket fuel and liquid oxygen oxidizer with a fuel efficiency of just under 5 inches per US gallon (just under 4 cm per liter) overall.[15] It was 42 metres (138 ft) tall and 10 metres (33 ft) in diameter, and provided over 34 meganewtons (7,600,000 lbf) of thrust to get the rocket through the first 67 kilometres (220,000 ft) of ascent. The S-IC stage had a dry weight of about 131 tonnes (289,000 lb) and fully fueled at launch had a total weight of 2,300 tonnes (5,100,000 lb). The initial design included four F-1 engines, which provided just enough force to lift the spacecraft and rocket. A fifth F-1 engine was added in the center of a quincunx to provide additional thrust to accommodate the growing weight of the Apollo payload.[8] That center engine was fixed, while the four outer engines could be hydraulically turned (gimballed) to control the rocket.[15] In flight, the center engine was turned off about 26 seconds earlier than the outboard engines to limit acceleration. During launch, the S-IC fired its engines for 168 seconds (ignition occurred about 7 seconds before liftoff) and at engine cutoff, the vehicle was at an altitude of about 67 kilometres (42 mi), was downrange about 93 kilometres (58 mi), and was moving about 2,300 metres per second (7,500 ft/s).[16]
S-II second stage

The S-II was built by North American Aviation at Seal Beach, California. Using liquid hydrogen and liquid oxygen, it had five J-2 engines in a similar arrangement to the S-IC, also using the outer engines for control. The S-II was 81 feet 7 inches (24.87 m) tall with a diameter of 33 feet (10 m), identical to the S-IC, and thus was the largest cryogenic stage until the launch of the STS. The S-II had a dry weight of about 80,000 pounds (36,000 kg) and fully fueled, weighed 1,060,000 pounds (480,000 kg). The second stage accelerated the Saturn V through the upper atmosphere with 5.1 meganewtons (1,100,000 lbf) of thrust (in vacuum). When loaded, significantly more than 90 percent of the mass of the stage was propellant; however, the ultra-lightweight design had led to two failures in structural testing. Instead of having an intertank structure to separate the two fuel tanks as was done in the S-IC, the S-II used a common bulkhead that was constructed from both the top of the LOX tank and bottom of the LH2 tank. It consisted of two aluminum sheets separated by a honeycomb structure made of phenolic resin. This bulkhead had to insulate against the 126 °F (70 °C) temperature gradient between the two tanks. The use of a common bulkhead saved 7,900 pounds (3.6 t). Like the S-IC, the S-II was transported by sea.
S-IVB third stage

The S-IVB was built by the Douglas Aircraft Company at Huntington Beach, California. It had one J-2 engine and used the same fuel as the S-II. The S-IVB used a common bulkhead to insulate the two tanks. It was 58 feet 7 inches (17.86 m) tall with a diameter of 21 feet 8 inches (6.604 m) and was also designed with high mass efficiency, though not quite as aggressively as the S-II. The S-IVB had a dry weight of about 23,000 pounds (10,000 kg) and, fully fueled, weighed about 262,000 pounds (119,000 kg).[17]
The S-IVB-500 model used on the Saturn V differed from the S-IVB-200 used as the second stage of the Saturn IB, in that the engine was restartable once per mission. This was necessary as the stage would be used twice during a lunar mission: first in a 2.5 min burn for the orbit insertion after second stage cutoff, and later for the trans-lunar injection (TLI) burn, lasting about 6 min. Two liquid-fueled Auxiliary Propulsion System (APS) units mounted at the aft end of the stage were used for attitude control during the parking orbit and the trans-lunar phases of the mission. The two APSs were also used as ullage engines to settle the propellants in the aft tank engine feed lines prior to the trans-lunar injection burn.
The S-IVB was the only rocket stage of the Saturn V small enough to be transported by plane, in this case the Pregnant Guppy.
Instrument Unit

The Instrument Unit was built by IBM and rode atop the third stage. It was constructed at the Space Systems Center in Huntsville, Alabama. This computer controlled the operations of the rocket from just before liftoff until the S-IVB was discarded. It included guidance and telemetry systems for the rocket. By measuring the acceleration and vehicle attitude, it could calculate the position and velocity of the rocket and correct for any deviations.
Range safety
In the event of an abort requiring the destruction of the rocket, the range safety officer would remotely shut down the engines and after several seconds send another command for the shaped explosive charges attached to the outer surfaces of the rocket to detonate. These would make cuts in fuel and oxidizer tanks to disperse the fuel quickly and to minimize mixing. The pause between these actions would give time for the crew to escape using the Launch Escape Tower or (in the later stages of the flight) the propulsion system of the Service module. A third command, "safe", was used after the S-IVB stage reached orbit to irreversibly deactivate the self-destruct system. The system was also inactive as long as the rocket was still on the launch pad.[18]
Comparisons

The Soviet counterpart of the Saturn V was the N-1 rocket. The Saturn V was taller, heavier and had greater payload capacity,[19] while the N-1 had more liftoff thrust and a larger first stage diameter.[20] The N1 never became operational; four test launches each resulted in catastrophic vehicle failure early in flight, and the program was canceled. The first stage of Saturn V used five powerful engines rather than the 30 smaller engines of the N-1. During two launches, Apollo 6 and Apollo 13, the Saturn V was able to recover from engine loss incidents. The N-1 likewise was designed to compensate for engine loss, but the system never successfully saved a launch from failure.
The three-stage Saturn V had a peak thrust of at least 7,650,000 pounds-force (34.02 MN) (SA-510 and subsequent)[21] and a lift capacity of 118,000 kg to LEO. The SA-510 mission (Apollo 15) had a liftoff thrust of 7,823,000 pounds-force (34.80 MN). The SA-513 mission (Skylab) had slightly greater liftoff thrust of 7,891,000 pounds-force (35.10 MN). By comparison, the N-1 had a sea-level liftoff thrust of about 9,900,000 pounds-force (44 MN). No other operational launch vehicle has ever surpassed the Saturn V in height, weight, or payload capability. The closest contenders were the US Space Shuttle and the Soviet Energia.
The Space Shuttle generated a peak thrust of 30.1 meganewtons (6,800,000 lbf),[22] and payload capacity to LEO (excluding the Orbiter itself) was 28,800 kilograms (63,500 lb), which was about 25 percent of the Saturn V's payload. Total mass in orbit, including the Orbiter, was about 112,000 kilograms (247,000 lb), compared to the Apollo 15 total orbital mass of the S-IVB third stage and Apollo spacecraft, of 140,976 kilograms (310,799 lb).
Energia had the same liftoff thrust as SA-513, 7,900,000 pounds-force (35.1 MN).[citation needed] The Energia flew twice in 1987 and 1988, and was planned as the launcher for the Buran shuttle. However, the Energia and Buran programs were cancelled in 1993. Hypothetical future versions of Energia might have been significantly more powerful than the Saturn V, delivering 46 meganewtons (10,000,000 lbf) of thrust and able to deliver up to 175 tonnes (386,000 lb) to LEO in the "Vulkan" configuration. Planned uprated versions of the Saturn V using F-1A engines would have had about 18 percent more thrust and 137,250 kilograms (302,580 lb) payload.[23] NASA contemplated building larger members of the Saturn family, such as the Saturn C-8, and also unrelated rockets, such as Nova, but these were never produced.
Some other recent launch vehicles have a small fraction of the Saturn V's payload capacity: the European Ariane 5 with the newest versions Ariane 5 ECA delivers up to 10,000 kg to geostationary transfer orbit (GTO). The US Delta 4 Heavy, which launched a dummy satellite on December 21, 2004, has a capacity of 13,100 kg to geosynchronous transfer orbit. The yet to be flown Atlas V Heavy (using engines based on a Russian design) delivers up to 29,400 kg to LEO and 8,900 kg to GTO. The yet to be flown SpaceX Falcon Heavy delivers up to 53,000 kg to LEO and 12,000 kg to GTO.
S-IC thrust comparisons

Because of its large size, attention is often focused on the S-IC thrust and how this compares to other large rockets. However, several factors make such comparisons more complex than first appears:
- Commonly referenced thrust numbers are a specification, not an actual measurement. Individual stages and engines may fall short or exceed the specification, sometimes significantly.
- The F-1 thrust specification was uprated beginning with Apollo 15 (SA-510) from 1.5 million lbf (6.67 MN) to 1.522 million lbf (6.77 MN), or 7.61 million lbf (33.85 MN) for the S-IC stage. The higher thrust was achieved via a redesign of the injector orifices and a slightly higher propellant mass flow rate. However, comparing the specified number to the actual measured thrust of 7.823 million lbf (34.8 MN) on Apollo 15 shows a significant difference.
- There is no "bathroom scale" way to directly measure thrust of a rocket in flight. Rather a mathematical calculation is made from combustion chamber pressure, turbopump speed, calculated propellant density and flow rate, nozzle design, and atmospheric conditions, in particular, external pressure.
- Thrust varies greatly with external pressure and thus with altitude, even for a non-throttled engine. For example on Apollo 15, the calculated total liftoff thrust (based on actual measurements) was about 7.823 million lbf (34.8 MN), which increased to 9.18 million lbf (40.8 MN) at T+135 seconds, just before center engine cutoff (CECO), at which time the jet was heavily underexpanded.
- Thrust specifications are often given as vacuum thrust (for upper stages) or sea level thrust (for lower stages or boosters), sometimes without qualifying which one. This can lead to incorrect comparisons.
- Thrust specifications are often given as average thrust or peak thrust, sometimes without qualifying which one. Even for a non-throttled engine at a fixed altitude, thrust can often vary somewhat over the firing period due to several factors. These include intentional or unintentional mixture ratio changes, slight propellant density changes over the firing period, and variations in turbopump, nozzle and injector performance over the firing period.
Without knowing the exact measurement technique and mathematical method used to determine thrust for each different rocket, comparisons are often inexact. As the above shows, the specified thrust often differs significantly from actual flight thrust calculated from direct measurements. The thrust stated in various references is often not adequately qualified as to vacuum vs sea level, or peak vs average thrust.
Similarly, payload increases are often achieved in later missions independent of engine thrust. This is by weight reduction or trajectory reshaping.
The result is there is no single absolute figure for engine thrust, stage thrust or vehicle payload. There are specified values and actual flight values, and various ways of measuring and deriving those actual flight values.
The performance of each Saturn V launch was extensively analyzed and a Launch Evaluation Report produced for each mission, including a thrust/time graph for each vehicle stage on each mission.[24]
Assembly

After the construction and ground testing of a stage was completed, it was then shipped to the Kennedy Space Center. The first two stages were so large that the only way to transport them was by barge. The S-IC, constructed in New Orleans, was transported down the Mississippi River to the Gulf of Mexico. After rounding Florida, it was then transported up the Intra-Coastal Waterway to the Vertical Assembly Building (now called the Vehicle Assembly Building). This is in essence the same route which was used by NASA to ship Space Shuttle External Tanks. The S-II was constructed in California and so traveled via the Panama Canal. The third stage and Instrument Unit could be carried by the Aero Spacelines Pregnant Guppy and Super Guppy, but could also have been carried by barge if warranted.
On arrival at the Vertical Assembly Building, each stage was checked out in a horizontal position before being moved to a vertical position. NASA also constructed large spool-shaped structures that could be used in place of stages if a particular stage was late. These spools had the same height and mass and contained the same electrical connections as the actual stages.
NASA stacked or assembled the Saturn V on a Mobile Launcher Platform (MLP), which consisted of a Launch Umbilical Tower (LUT) with nine swing arms (including the crew access arm), a "hammerhead" crane, and a water suppression system which was activated prior to launch. After assembly was completed, the entire stack was moved from the VAB to the launch pad using the Crawler Transporter (CT). Built by the Marion Power Shovel company (and later used for transporting the smaller and lighter Space Shuttle), the CT ran on four double-tracked treads, each with 57 'shoes'. Each shoe weighed 900 kg (2,000 lb). This transporter was also required to keep the rocket level as it traveled the 3 miles (4.8 km) to the launch site, especially at the 3 percent grade encountered at the launch pad. The CT also carried the Mobile Service Structure (MSS), which allowed technicians access to the rocket until eight hours before launch, when it was moved to the "halfway" point on the Crawlerway (the junction between the VAB and the two launch pads).
Lunar mission launch sequence
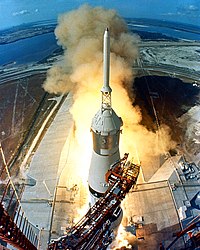
The Saturn V carried all Apollo lunar missions. All Saturn V missions launched from Launch Complex 39 at the John F. Kennedy Space Center in Florida. After the rocket cleared the launch tower, flight control transferred to Johnson Space Center's Mission Control in Houston, Texas.
An average mission used the rocket for a total of just 20 minutes. Although Apollo 6 experienced three engine failures,[25] and Apollo 13 one engine shutdown,[26] the onboard computers were able to compensate by burning the remaining engines longer to achieve parking orbit. None of the Saturn V launches resulted in a payload loss.
S-IC sequence

The first stage burned for about 2 minutes and 41 seconds, lifting the rocket to an altitude of 42 miles (68 km) and a speed of 6,164 miles per hour (2,756 m/s) and burning 4,700,000 pounds (2,100,000 kg) of propellant.[27]
At 8.9 seconds before launch, the first stage ignition sequence started. The center engine ignited first, followed by opposing outboard pairs at 300-millisecond intervals to reduce the structural loads on the rocket. When thrust had been confirmed by the onboard computers, the rocket was "soft-released" in two stages: first, the hold-down arms released the rocket, and second, as the rocket began to accelerate upwards, it was slowed by tapered metal pins pulled through dies for half a second. Once the rocket had lifted off, it could not safely settle back down onto the pad if the engines failed. The astronauts considered this one of the tensest moments in riding the Saturn V, for if the rocket did fail to lift off after release they had a low chance of survival given the large amounts of propellant. A fully fueled Saturn V exploding on the pad would have released two kilotons of energy. To improve the odds, the Saturn Emergency Detection System (EDS) inhibited engine shutdown for the first 30 seconds of flight. (See Saturn V Instrument Unit)
It took about 12 seconds for the rocket to clear the tower. During this time, it yawed 1.25 degrees away from the tower to ensure adequate clearance despite adverse winds. (This yaw, although small, can be seen in launch photos taken from the east or west.) At an altitude of 430 feet (130 m) the rocket rolled to the correct flight azimuth and then gradually pitched down until 38 seconds after second stage ignition. This pitch program was set according to the prevailing winds during the launch month. The four outboard engines also tilted toward the outside so that in the event of a premature outboard engine shutdown the remaining engines would thrust through the rocket's center of gravity. The Saturn V reached 400 feet per second (120 m/s) at over 1 mile (1,600 m) in altitude. Much of the early portion of the flight was spent gaining altitude, with the required velocity coming later. The Saturn V broke the sound barrier at just over 1 minute at an altitude of between 3 and 4 nautical miles. At this point, shock collars, or condensation clouds, could be seen forming around the bottom of the command module and around the top of the second stage.

At about 80 seconds, the rocket experienced maximum dynamic pressure (max Q). The dynamic pressure on a rocket varies with air density and the square of relative velocity. Although velocity continues to increase, air density decreases so quickly with altitude that dynamic pressure falls below max Q.
Acceleration increased during S-IC flight for three reasons. One, increased acceleration increased the propellant pressure at the engines, increasing the flow rate somewhat. This was the least important factor, though this feedback effect often led to an undesirable thrust oscillation called pogo. Two, as it climbed into thinner air F-1 engine efficiency increased significantly, a property of all rockets. The combined thrust of five engines on the pad was about 7.5 million pounds, reaching nearly 9 million pounds at altitude. But the biggest contribution by far was the rocket's rapidly decreasing mass. The propellant in just the S-IC made up about three-quarters of Saturn V's entire launch mass, and it was furiously consumed at over 13 metric tonnes per second. Newton's second law states that force is equal to mass times acceleration, or equivalently that acceleration is equal to force divided by mass, so as the mass decreased (and the force increased somewhat), acceleration rose. Including gravity, launch acceleration was only 1 1/4 g, i.e., the astronauts felt 1 1/4 g while the rocket accelerated vertically at 1/4 g. As the rocket rapidly lost mass, total acceleration including gravity increased to nearly 4 g at T+135 seconds.
It would have increased further except that at 135 seconds the inboard (center) engine shut down to prevent it. Acceleration then resumed its increase, again reaching nearly 4 g on the remaining 4 engines when oxidizer and/or fuel depletion was sensed in the suction assemblies. First stage separation occurred a little less than one second after cutoff to allow for F-1 thrust tail-off. Eight small solid fuel separation motors backed the S-IC from the interstage at an altitude of about 67 kilometers (42 mi). The first stage continued ballistically to an altitude of about 109 kilometers (68 mi) and then fell in the Atlantic Ocean about 560 kilometers (350 mi) downrange.
S-II sequence

After S-IC separation, the S-II second stage burned for 6 minutes and propelled the craft to 109 miles (175 km) and 15,647 mph (25,182 km/h– 7.00 km/s), close to orbital velocity.
For the first two unmanned launches, eight solid-fuel ullage motors ignited for four seconds to give positive acceleration to the S-II stage, followed by start of the five J-2 engines. For the first seven manned Apollo missions only four ullage motors were used on the S-II, and they were eliminated completely for the final four launches. About 30 seconds after first stage separation, the interstage ring dropped from the second stage. This was done with an inertially fixed attitude so that the interstage, only 1 meter from the outboard J-2 engines, would fall cleanly without contacting them. Shortly after interstage separation the Launch Escape System was also jettisoned. See Apollo abort modes for more information about the various abort modes that could have been used during a launch.

About 38 seconds after the second stage ignition the Saturn V switched from a preprogrammed trajectory to a "closed loop" or Iterative Guidance Mode. The Instrument Unit now computed in real time the most fuel-efficient trajectory toward its target orbit. If the Instrument Unit failed, the crew could switch control of the Saturn to the Command Module's computer, take manual control, or abort the flight.
About 90 seconds before the second stage cutoff, the center engine shut down to reduce longitudinal pogo oscillations. At around this time, the LOX flow rate decreased, changing the mix ratio of the two propellants, ensuring that there would be as little propellant as possible left in the tanks at the end of second stage flight. This was done at a predetermined delta-v.
Five level sensors in the bottom of each S-II propellant tank were armed during S-II flight, allowing any two to trigger S-II cutoff and staging when they were uncovered. One second after the second stage cut off it separated and several seconds later the third stage ignited. Solid fuel retro-rockets mounted on the interstage at the top of the S-II fired to back it away from the S-IVB. The S-II impacted about 4,200 km (2,600 mi) from the launch site
On the Apollo 13 mission, the inboard engine suffered from major pogo oscillation, resulting in an early automatic cutoff. To ensure sufficient velocity was reached, the remaining four engines were kept active for longer than planned. A pogo suppressor was fitted to later Apollo missions to avoid this, though the early engine 5 cutoff remained to reduce G-forces.
S-IVB sequence
Unlike the two-plane separation of the S-IC and S-II, the S-II and S-IVB stages separated with a single step. Although it was constructed as part of the third stage, the interstage remained attached to the second stage.
During Apollo 11, a typical lunar mission, the third stage burned for about 2.5 minutes until first cutoff at 11 minutes 40 seconds. At this point it was 1,640 miles (2,640 km) downrange and in a parking orbit at an altitude of 118.8 miles (191.2 km) and velocity of 17,432 mph. The third stage remained attached to the spacecraft while it orbited the Earth two and a half times while astronauts and mission controllers prepared for translunar injection (TLI).

This parking orbit was quite low by Earth orbit standards, and it would have been short-lived due to aerodynamic drag. This was not a problem on a lunar mission because of the short stay in the parking orbit. The S-IVB also continued to thrust at a low level by venting gaseous hydrogen, to keep propellants settled in their tanks and prevent gaseous cavities from forming in propellant feed lines. This venting also maintained safe pressures as liquid hydrogen boiled off in the fuel tank. This venting thrust easily exceeded aerodynamic drag.
For the final three Apollo flights, the temporary parking orbit was even lower (approximately 172 kilometers (107 mi)), to increase payload for these missions. The Apollo 9 Earth orbit mission was launched into the nominal orbit consistent with Apollo 11, but the spacecraft were able to use their own engines to raise the perigee high enough to sustain the 10-day mission. The Skylab was launched into a quite different orbit, with a 434-kilometer (270 mi) perigee which sustained it for six years, and also a higher inclination to the equator (50 degrees versus 32.5 degrees for Apollo).
On Apollo 11, TLI came at 2 hours and 44 minutes after launch. The S-IVB burned for almost six minutes giving the spacecraft a velocity close to the Earth's escape velocity of 11.2 km/s (40,320 km/h; 25,053 mph). This gave an energy-efficient transfer to lunar orbit with the moon helping to capture the spacecraft with a minimum of CSM fuel consumption.
About 40 minutes after TLI the Apollo Command Service Module (CSM) separated from the third stage, turned 180 degrees and docked with the Lunar Module (LM) that rode below the CSM during launch. The CSM and LM separated from the spent third stage 50 minutes later. This process is known as Transposition, docking, and extraction.
If it were to remain on the same trajectory as the spacecraft, the S-IVB could have presented a collision hazard so its remaining propellants were vented and the auxiliary propulsion system fired to move it away. For lunar missions before Apollo 13, the S-IVB was directed toward the moon's trailing edge in its orbit so that the moon would slingshot it beyond earth escape velocity and into solar orbit. From Apollo 13 onwards, controllers directed the S-IVB to hit the Moon.[28] Seismometers left behind by previous missions detected the impacts, and the information helped map the inside of the Moon.
Apollo 9 was a special case; although it was an earth orbital mission, after spacecraft separation its S-IVB was fired out of earth orbit into a solar orbit.
On September 3, 2002, astronomer Bill Yeung discovered a suspected asteroid, which was given the discovery designation J002E3. It appeared to be in orbit around the Earth, and was soon discovered from spectral analysis to be covered in white titanium dioxide paint, the same paint used for the Saturn V. Calculation of orbital parameters identified the apparent asteroid as being the Apollo 12 S-IVB stage. Mission controllers had planned to send Apollo 12's S-IVB into solar orbit, but the burn after separating from the Apollo spacecraft lasted too long, and hence it did not pass close enough to the Moon, remaining in a barely stable orbit around the Earth and Moon. In 1971, through a series of gravitational perturbations, it is believed to have entered in a solar orbit and then returned into weakly captured Earth orbit 31 years later. It left Earth orbit again in June 2003. Another near-earth object, discovered in 2006 and designated 6Q0B44E, may also be part of an Apollo spacecraft.
Skylab

In 1968, the Apollo Applications Program was created to look into science missions that could be performed with the surplus Apollo hardware. Much of the planning centered on the idea of a space station, which eventually spawned the Skylab program. Skylab was launched using a two-stage Saturn V, similar to the proposed Saturn INT-21.[2][29] It was the only launch not directly related to the Apollo lunar landing program. The only significant changes to the Saturn V from the Apollo configurations involved some modification to the S-II to act as the terminal stage for inserting the Skylab payload into earth orbit, and to vent excess propellant after engine cutoff so the spent stage would not rupture in orbit. The S-II remained in orbit for almost two years, and made an uncontrolled re-entry on January 11, 1975.
Originally it was planned to use a 'wet workshop' concept, with a rocket stage being launched into orbit by a Saturn 1B and its spent S-IVB outfitted in space, but this was abandoned for the 'dry workshop' concept: An S-IVB stage from a Saturn IB was converted into a space station on the ground and launched on a Saturn V. A backup, constructed from a Saturn V third stage, is now on display at the National Air and Space Museum.
Three crews lived aboard Skylab from May 25, 1973 to February 8, 1974, with Skylab remaining in orbit until July 11, 1979.
Proposed post-Apollo developments
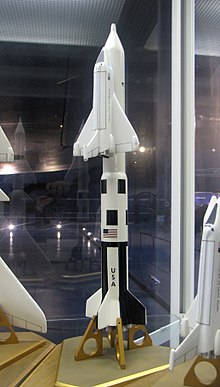
After Apollo, the Saturn V was planned to be the prime launch vehicle for Prospector intended to deliver a 330kg robotic rover on the Moon similar to Lunokhod[30] and the Voyager Mars probes, as well an upscaled version of the Voyager interplanetary probes.[31] It was also to have been the launch vehicle for the nuclear rocket stage RIFT test program and the later NERVA.[32] All of these planned uses of the Saturn V were cancelled, with cost being a major factor. Edgar Cortright, who had been director of NASA Langley, stated decades later that "JPL never liked the big approach. They always argued against it. I probably was the leading proponent in using the Saturn V, and I lost. Probably very wise that I lost."[31]
The (canceled) second production run of Saturn Vs would very likely have used the F-1A engine in its first stage, providing a substantial performance boost.[33] Other likely changes would have been the removal of the fins (which turned out to provide little benefit when compared to their weight); a stretched S-IC first stage to support the more powerful F-1As; and uprated J-2s or a M-1 (rocket engine) for the upper stages.
A number of alternate Saturn vehicles were proposed based on the Saturn V, ranging from the Saturn INT-20 with an S-IVB stage and interstage mounted directly onto an S-IC stage, through to the Saturn V-23(L)[34] which would not only have five F-1 engines in the first stage, but also four strap-on boosters with two F-1 engines each: giving a total of thirteen F-1 engines firing at launch.
The Space Shuttle was initially conceived of as a cargo transport to be used in concert with the Saturn V, even to the point that a Saturn-Shuttle was proposed, using the winged shuttle orbiter and external tank, but with the tank mounted on a modified, fly-back version of the S-IC. The first S-IC stage would be used to power the Shuttle during the first two minutes of flight, after which the S-IC would be jettisoned (which would then fly back to KSC for refurbishment) and the Space Shuttle Main Engines would then fire and place the orbiter into orbit. The Shuttle would handle space station logistics, while Saturn V would launch components. Lack of a second Saturn V production run killed this plan and has left the United States without a heavy-lift launch vehicle. Some in the U.S. space community have come to lament this situation,[who?] as continued production would have allowed the International Space Station, using a Skylab or Mir configuration with both U.S. and Russian docking ports, to have been lifted with just a handful of launches. The Saturn-Shuttle concept also would have eliminated the Space shuttle solid rocket boosters that ultimately precipitated the Challenger accident in 1986.
Proposed successors
(Left to right)Space Shuttle payload includes 7 crew and cargo. Ares I payload includes 4 crew and inherent craft. Saturn V payload includes 3 crew, inherent craft and cargo. Ares V payload includes only cargo and inherent craft. The Saturn V lifted approximately 118 metric tons of payload to LEO. The Ares V was being designed to lift 188 metric tons to LEO.
U.S. proposals for a rocket larger than the Saturn V from the late 1950s through the early 1980s were generally called Nova. Over thirty different large rocket proposals carried the Nova name, but none were developed.
Wernher von Braun and others also had plans for a rocket that would have featured eight F-1 engines, like the Saturn C-8, in its first stage allowing it to launch a manned spacecraft on a direct ascent flight to the Moon. Other plans for the Saturn V called for using a Centaur as an upper stage or adding strap-on boosters. These enhancements would have increased its ability to send large unmanned spacecraft to the outer planets or manned spacecraft to Mars. Other Saturn-V derivatives analyzed, included the Saturn MLV concept family of "Modified Launch Vehicles", which would have almost doubled the payload lift capability of the standard Saturn V, and was intended for use in a proposed manned mission to Mars by 1980.[35]
Another Saturn-V derivative analyzed, included the use of a solid nuclear thermal rocket engine, a number of which were successfully ground tested, and which was intended for the, in orbit upper third stage of the vehicle, a vehicle designated Saturn C-5N, a baseline Saturn C-5 with a nuclear Saturn S-N engine, studied by Boeing in 1968,[36] in place of the standard chemical J-2 (rocket engine). This would carry a considerably higher payload into deep space than the all chemical version of the Saturn V, and was also being studied for a manned mission to Mars by 1980, however the then President Nixon ended work on the engines in 1973, along with all Saturn V ELVs, in government budget cuts.[37][38]

In 2006, as part of the cancelled Constellation Program that would have replaced the Space Shuttle, NASA unveiled plans to construct the heavy-lift Ares V rocket, a Shuttle Derived Launch Vehicle using some existing Space Shuttle and Saturn V infrastructure. Named in homage of the Saturn V, the original design, based on the Space Shuttle External Tank, was 360 ft (110 m) tall, and powered by five Space Shuttle Main Engines (SSMEs) and two uprated five-segment Space Shuttle Solid Rocket Boosters(SRBs), while a modified variation would be used for the crew-launched Ares I rocket. Safety would be increased by having each vehicle be specialized for different tasks, keeping cargo and crew services separate.[39] As the design evolved, the Ares V was slightly modified, with the same 33 ft (10 m) diameter as that of the Saturn V's S-IC and S-II stages, and in place of the five SSMEs, five RS-68 rocket engines, the same engines used on the Delta IV EELV, would be used. The switch from the SSME to the RS-68 was due to the steep price of the SSME, as that it would be thrown away along with the Ares V core stage after each use, while the RS-68 engine, which is expendable, is cheaper, simpler to manufacture, and more powerful than the SSME.

In 2008, NASA again redesigned the Ares V, lengthening and widening the core stage and added an extra RS-68 engine, giving the first stage core of the launch vehicle a total of six engines. The six RS-68B engines, during launch, would have been augmented by two "5.5-segment" SRBs instead of the original five-segment designs, although no decision was made on the number of segments NASA would have used on the final design.[40] If the six RS-68B/5.5-segment SRB variant had been used, the vehicle would have had a total of approximately 8,900,000 lbf (40 MN) of thrust at liftoff, making it more powerful than the Saturn V or the Soviet/Russian Energia boosters, but less than 50–43 MN for the Soviet N-1. An upper stage, known as the Earth Departure Stage and based on the S-IVB, would have utilized a more advanced version of the J-2 engine known as the "J-2X," and would have placed the Altair lunar landing vehicle into a low earth orbit. At 381 ft (116 m) tall and with the capability of placing 180 metric tons into low Earth orbit, the Ares V would have surpassed the Saturn V and the two Soviet/Russian super-heavy lift vehicles in both height, lift, and launch capability.

The RS-68B engines, based on the current RS-68 and RS-68A engines built by the Rocketdyne Division of Pratt and Whitney (formerly under the ownerships of Boeing and Rockwell International), produce less than half the thrust per engine as the Saturn V's F-1 engines, but are more efficient and can be throttled up or down, much like the SSMEs on the Shuttle, and as with the Shuttle, the SRBs of the Ares V would have produced the majority of the lift off thrust. The J-2 engine, used in the Saturn V S-II and S-IVB stages, would have been modified into the improved J-2X engine for use both, on the Ares V Earth Departure Stage (EDS) as well as on the second stage of the proposed Ares I. Both the EDS and the Ares I second stage would have used a single J-2X motor, although the EDS was originally designed to use two motors until the redesign employing the five (later six) RS-68Bs in place of the five SSMEs.
In September 2011, NASA announced[41] the Space Launch System (SLS) as the United States' new heavy-lift rocket for manned deep-space exploration, and which will be comparable in size and capabilities to the Saturn V. The new SLS, similar to the Saturn V and Ares IV concept that directly preceded it, is both a cargo and crew carrier. It has an upper-stage powered by a J2-X engine derived from the Saturn V launch vehicle, the first stage powered by five liquid-fueled rocket engines derived from the Space Shuttle's main engines, along with two strap-on SRBs also derived from the Shuttle program. The initial configuration of the new booster as proposed by NASA could lift approximately 70 metric tons to low earth orbit, with later "Block II" variants with the SRBs possibly lifting up to 130 metric tons.
In 2012, it was noted if a derivative of the F-1 (rocket engine) was integrated as a liquid fuel booster in the SLS Block II, the payload could be 150 metric tons to low earth orbit, 20 metric tons higher than that of the planned SLS Block II lifted by SRBs.[42] In 2013, it was reported that the F-1B engine is to have improved efficiency, thrust, be more cost effective with a simplified combustion chamber, and have fewer engine parts. Each F-1B is to produce 1,800,000 lbf (8.0 MN) of thrust at sea level, an increase over the approximate 1,550,000 lbf (6.9 MN) of thrust achieved by the mature Apollo 15 F-1 engine.[43]
NASA SLS deputy project manager Jody Singer of the Marshall Space Flight Center in Huntsville, in 2012 stated that the vehicle will have a launch cost of approximately $500 million per launch, with a relatively minor dependence of costs on launch capability.[44]
Cost
From 1964 until 1973, a total of $6.5 billion ($61.04 billion present day) was appropriated for the Saturn V, with the maximum being in 1966 with $1.2 billion ($11.27 billion present day).[45]
One of the main reasons for the cancellation of the Apollo program was the cost. In 1966, NASA received its biggest budget of US$4.5 billion, about 0.5 percent of the Gross domestic product(GDP) of the United States at that time. In 1969, the cost of a Saturn V including launch was US $ 185 million (inflation adjusted US$ 1.54 billion in 2024).[46]
Saturn V vehicles and launches

Serial Number |
Mission | Launch Date |
Notes |
---|---|---|---|
SA-500F | Facilities integration | Used to check precise fits and operations of facilities before a flight model was ready. First stage scrapped, second stage converted to S-II-F/D, third stage whereabouts unknown. | |
SA-500D | Dynamic testing | Used to evaluate the systems' response to vibrations. On display at the U.S. Space & Rocket Center, Huntsville, Alabama [47] | |
SA-501 | Apollo 4 | November 9, 1967 | First test flight (unmanned), complete success |
SA-502 | Apollo 6 | April 4, 1968 | Second test flight (unmanned), with some serious second and third stage problems occurring |
SA-503 | Apollo 8 | December 21, 1968 | First manned flight of Saturn V, first manned lunar orbit |
SA-504 | Apollo 9 | March 3, 1969 | Earth orbit LM test |
SA-505 | Apollo 10 | May 18, 1969 | Lunar orbit LM test |
SA-506 | Apollo 11 | July 16, 1969 | First manned lunar landing, at Mare Tranquillitatis |
SA-507 | Apollo 12 | November 14, 1969 | Second manned lunar landing, near site of Surveyor 3 at Mare Cognitum. Vehicle was struck twice by lightning shortly after liftoff with no serious damage. |
SA-508 | Apollo 13 | April 11, 1970 | Severe, near catastrophic pogo oscillations in second stage caused early center engine shutdown. Service Module O2 tank rupture caused mission abort en route to moon, crew saved. |
SA-509 | Apollo 14 | January 31, 1971 | Third manned lunar landing, near Fra Mauro |
SA-510 | Apollo 15 | July 26, 1971 | Fourth manned lunar landing, first Lunar Roving Vehicle, at Hadley-Apennine |
SA-511 | Apollo 16 | April 16, 1972 | Fifth manned lunar landing, at Descartes |
SA-512 | Apollo 17 | December 7, 1972 | Sixth and final manned lunar landing, at Taurus-Littrow. First and only night launch |
SA-513 | Skylab 1 | May 14, 1973 | The third stage (SIV-513) was replaced for flight by the Skylab module and is on display at Johnson Space Center.[47] |
SA-514 | Unused | Designated but never used for Apollo 18 or 19. First stage (S-IC-14) on display at Johnson Space Center, second and third stage (S-II-14,S-IV-14) on display at Kennedy Space Center.[47] | |
SA-515 | Unused | Designated but never used as a backup Skylab launch vehicle. The first stage is on display at Michoud Assembly Facility. The second stage (S-II-15) is on display at Johnson Space Center. The third stage was converted to a backup Skylab orbital workshop and is on display at the National Air and Space Museum.[47] |
Saturn V displays
- Two at the U.S. Space & Rocket Center in Huntsville:
- SA-500D is on horizontal display made up of S-IC-D, S-II-F/D and S-IVB-D. These were all test stages not meant for flight. This vehicle was displayed outdoors from 1969 to 2007 (there is a poignant photo above of Wernher von Braun next to it), was restored, and is now displayed in the Davidson Center for Space Exploration.
- Vertical display (replica) built in 1999 located in an adjacent area.
- One at the Johnson Space Center made up of first stage from SA-514, the second stage from SA-515 and the third stage from SA-513 (replaced for flight by the Skylab workshop). With stages arriving between 1977 and 1979, this was displayed in the open until its 2005 restoration when a structure was built around it for protection. This is the only display Saturn consisting entirely of stages intended to be launched.
- One at the Kennedy Space Center Visitor Complex made up of S-IC-T (test stage) and the second and third stages from SA-514.[citation needed] It was displayed outdoors for decades, then in 1996 was enclosed for protection from the elements in the Apollo/Saturn V Center.
- The S-IC stage from SA-515 is on display at the Michoud Assembly Facility in New Orleans, Louisiana.
- The S-IVB stage from SA-515 was converted for use as a backup for Skylab, and is on display at the National Air and Space Museum in Washington, D.C..
-
U.S. Space & Rocket Center
-
Johnson Space Center
-
Kennedy Space Center
-
S-IVB stage as Skylab, National Air & Space Museum
- A possible object to be displayed is a flown F-1 (rocket engine), which was lifted from a depth of 4.000 meter (12.000 feet) in the Atlantic Ocean during a 2013 expedition by Jeff Bezos.[2], which has been confirmed as F-1 engine number F-6044, the center engine in the SA-506 rocket which lifted Apollo 11.[48]
Media
See also
References
- Akens, David S (1971). Saturn illustrated chronology: Saturn's first eleven years, April 1957 - April 1968. NASA - Marshall Space Flight Center as MHR-5. Also available in PDF format. Retrieved on 2008-02-19.
- Benson, Charles D. and William Barnaby Faherty (1978). Moonport: A history of Apollo launch facilities and operations. NASA. Also available in PDF format. Retrieved on 2008-02-19. Published by University Press of Florida in two volumes: Gateway to the Moon: Building the Kennedy Space Center Launch Complex, 2001, ISBN 0-8130-2091-3 and Moon Launch!: A History of the Saturn-Apollo Launch Operations, 2001 ISBN 0-8130-2094-8.
- Bilstein, Roger E. (1996). Stages to Saturn: A Technological History of the Apollo/Saturn Launch Vehicle. NASA SP-4206. ISBN 0-16-048909-1. Also available in PDF format. Retrieved on 2008-02-19.
- Lawrie, Alan (2005). Saturn, Collectors Guide Publishing, ISBN 1-894959-19-1.
- Orloff, Richard W (2001). Apollo By The Numbers: A Statistical Reference. NASA. Also available in PDF format. Retrieved on 2008-02-19. Published by Government Reprints Press, 2001, ISBN 1-931641-00-5.
- Final Report - Studies of Improved Saturn V Vehicles and Intermediate Payload Vehicles (PDF). NASA - George C. Marshall Space Flight Center under Contract NAS&-20266. Retrieved on 2008-02-19.
- Saturn 5 launch vehicle flight evaluation report: AS-501 Apollo 4 mission (PDF). NASA – George C. Marshall Space Flight Center (1968). Retrieved on 2008-02-19.
- Saturn 5 launch vehicle flight evaluation report: AS-508 Apollo 13 mission (PDF). NASA – George C. Marshall Space Flight Center (1970). Retrieved on 2008-02-19.
- Saturn V Flight Manual - SA-503 (PDF). NASA – George C. Marshall Space Flight Center (1968). Retrieved on 2008-02-19.
- Saturn V Press Kit. Marshall Space Flight Center History Office. Retrieved on 2008-02-19.
Notes
- ^ a b Hitt, David (2010). "What Was The Saturn V?". Rocketry. Washington: NASA. Archived from the original on 2012-10-11. Retrieved 2012-10-11.
{{cite web}}
: Unknown parameter|deadurl=
ignored (|url-status=
suggested) (help) - ^ a b c Wade, Mark. "Saturn INT-21". Encyclopedia Astronautica. Retrieved 2008-01-16.
- ^ "V-1 and V-2 Rockets". IEEE Global History Network. Retrieved 2010-11-13.
- ^ Marov, Welsey T. Huntress, Mikhail Ya. The Soviet Robots in the Solar System. New York, NY: Gardners Books. p. 36. ISBN 1441978976.
{{cite book}}
: CS1 maint: multiple names: authors list (link) - ^ The Dawn of the Space Age — Central Intelligence Agency
- ^ a b c d e f g Roger E. Bilstein (1996). Stages to Saturn: A Technological History of the Apollo/Saturn Launch Vehicles. NASA SP-4206. ISBN 0-16-048909-1.
{{cite book}}
:|access-date=
requires|url=
(help) - ^ Robin Williams. "Wernher von Braun (1912–1977)". NASA. Retrieved 2010-11-13.
- ^ a b c d e f g Bilstein, Roger E. (1999). Stages to Saturn: A Technological History of the Apollo/Saturn Launch Vehicle. DIANE Publishing. pp. 59–61. ISBN 9780788181863. Retrieved 2008-02-04.
- ^ Edgar M. Cortright, editor (1975). "3.4". Apollo Expeditions to the Moon. NASA Langley Research Center. ISBN 978-9997398277. Retrieved 2008-02-11.
{{cite book}}
:|author=
has generic name (help); External link in
(help); Unknown parameter|chapterurl=
|chapterurl=
ignored (|chapter-url=
suggested) (help) - ^ a b "Saturn V Moon Rocket". Boeing. Retrieved 2010-11-14.
- ^ Edgar M. Cortright, editor (1975). "3.2". Apollo Expeditions to the Moon. NASA Langley Research Center. ISBN 978-9997398277. Retrieved 2008-02-11.
{{cite book}}
:|author=
has generic name (help); External link in
(help); Unknown parameter|chapterurl=
|chapterurl=
ignored (|chapter-url=
suggested) (help) - ^ The Apollo LES fired for a much shorter time than the Mercury-Redstone (3.2 seconds vs. 143.5 seconds); thus the Redstone still delivered a much greater total impulse of 11,193,000 pound-seconds (50,225 kN-sec), versus 470,400 pound-seconds (2,080 kN-sec) for the Apollo LES.Duncan, John (2002). "Apollo Spacecraft News Reference: Launch Escape System". The Apollo Saturn Reference Page.
{{cite web}}
: Cite has empty unknown parameter:|coauthors=
(help) - ^ "Stennis Space Center Celebrates 40 Years of Rocket Engine Testing". NASA. 2006-04-20. Retrieved 2008-01-16.
- ^ Paine, Michael (2000-03-13). "Saturn 5 Blueprints Safely in Storage". Space.com. Archived from the original on 2010-08-18. Retrieved 2011-11-09.
{{cite web}}
: Unknown parameter|deadurl=
ignored (|url-status=
suggested) (help) - ^ a b Lennick, Michael (2006). Launch vehicles : heritage of the space race. Burlington, Ontario: Apogee Books. p. 46. ISBN 1894959280.
- ^ NASA (1968). "Saturn V Flight Manual - SA-503" (PDF). NASA – George C. Marshall Space Flight Center. Retrieved February 22, 2011. §4.
- ^ "Saturn S-IVB". apollosaturn. Retrieved 2011-11-04.
- ^ "Skylab Saturn IB Flight Manual" (PDF). NASA Marshall Spaceflight Center. Retrieved 2008-01-16.
- ^ Wade, Mark. "Saturn V". Encyclopedia Astronautica. Retrieved 2008-01-16.
- ^ Wade, Mark. "N1". Encyclopedia Astronautica. Retrieved 2008-01-16.
- ^ "SP-4206 Stages to Saturn p405". NASA. Retrieved 2008-01-16.
- ^ "Working Scenario" (PDF). Columbia Accident Investigation Board. Retrieved 2008-01-16.
- ^ Wade, Mark. "Saturn MLV-V-1". Encyclopedia Astronautica. Retrieved 2008-01-16.
- ^ "InsideKSC". InsideKSC.com.
- ^ Saturn V Launch Vehicle Evaluation Report--AS-502 Apollo 6 Mission
- ^ Saturn 5 launch vehicle flight evaluation report: AS-508 Apollo 13 mission
- ^ Boeing History, Saturn V Moon Rocket, www.boeing.com/history/boeing/saturn.html
- ^ "NASA GSFC - Lunar Impact Sites". NASA. Retrieved 2008-01-16.
- ^ Young, Anthony (2008). The Saturn V F-1 Engine: Powering Apollo into History. New York: Springer-Praxis. p. 245. ISBN 978-0-387-09629-2.
- ^ Lunar Exploration, p40
- ^ a b Cortright Oral History (p31)
- ^ http://www.astronautix.com/stages/satv25su.htm Saturn S-N engine intended to be used on the Saturn C-5N
- ^ Wade, Mark. "Saturn Genealogy". Encyclopedia Astronautica. Archived from the original on 2007-12-26. Retrieved 2008-01-17.
- ^ Wade, Mark. "Saturn V-23(L)". Encyclopedia Astronautica. Retrieved 2008-01-16.
- ^ http://ntrs.nasa.gov/archive/nasa/casi.ntrs.nasa.gov/19650020081_1965020081.pdf "Modified Launch Vehicle (MLV) Saturn V Improvement Study Composite Summary Report", NASA Marshall Space Flight Center (MSFC), July 1965, page 76. Improved Saturn V configurations studied under contract NAS8-11359.
- ^ http://www.astronautix.com/stages/satv25su.htm
- ^ Dewar, James (2008). To The End Of The Solar System: The Story Of The Nuclear Rocket (2nd ed.). Apogee. ISBN 978-1-894959-68-1.
- ^ NASA's Nuclear Frontier The Plum Brook Reactor Facility - 188 page monograph. pages 68, 73, 76, 101, 116, 129.
- ^ http://www.ntrs.nasa.gov/archive/nasa/casi.ntrs.nasa.gov/20070002794_2007001555.pdf A New Heavy-Lift Capability for Space Exploration: NASA’s Ares V Cargo Launch Vehicle John P. Sumrall Manager, Advanced Planning Exploration Launch Projects Office National Aeronautics and Space Administration Marshall Space Flight Center, Huntsville, Alabama 35812 United States. Through years of triumph and tragedy, direct experience and engineering risk analyses have concluded that separating the crew from the cargo during launch reduces safety risks and improves safety statistics.
- ^ Phil Sumrall (2008-08-15). "Ares V Overview" (PDF). p. 4 - Launch Vehicle Comparisons.
- ^ David S. Weaver (2011-09-14). "NASA SLS Announcement".
- ^ http://www.nasaspaceflight.com/2012/11/dynetics-pwr-liquidize-sls-booster-competition-f-1-power/
- ^ Lee Hutchinson (2013-04-15). "New F-1B rocket engine upgrades Apollo-era design with 1.8M lbs of thrust". Ars Technica. Retrieved 2013-04-15.
- ^ "NASA's huge new rocket may cost $500 million per launch". MSNBC. September 12, 2012.
- ^ "Apollo Program Budget Appropriations". NASA. Retrieved 2008-01-16.
- ^ "SP-4221 The Space Shuttle Decision- Chapter 6: ECONOMICS AND THE SHUTTLE". NASA. Retrieved 2011-01-15.
- ^ a b c d Wright, Mike. "Three Saturn Vs on Display Teach Lessons in Space History". NASA. Retrieved February 10, 2011.
- ^ [1]
External links

NASA sites
- Three Saturn Vs on Display Teach Lessons in Space History
- Apollo Lunar Surface Journal
- Declassified 1964 NASA technical memo on Saturn Rockets[dead link] Note that this is a large PDF file (61 Megabytes)
- Launch complex 39 facility description from 1966 (10 Megabyte PDF file)
- Exploring the Unknown Selected Documents in the History of the U.S. Civil Space Program
Other sites
- Apollo Saturn Reference Page
- Project Apollo Archive
- Apollo/Saturn V Development ApolloTV.net Video
- Saturn I-IVB Stage
- Blog about Apollo 11 mission
Simulators
- 3D Saturn V Explorer and Launch Simulation Program
- 3D Apollo Simulator with Saturn V Simulation Program
- Saturn V/Saturn IB simulation for Orbiter spaceflight sim