Energy development

Energy development is the effort to provide sufficient primary energy sources and secondary energy forms to fulfill supply, cost, impact on air pollution and water pollution, and whether or not the source is renewable.
Technologically advanced societies have become increasingly dependent on external energy sources for transportation, the production of many manufactured goods, and the delivery of energy services. This energy allows people who can afford the cost to live under otherwise unfavorable climatic conditions through the use of heating, ventilation, and/or air conditioning. Level of use of external energy sources differs across societies, as do the climate, convenience, levels of traffic congestion, pollution and availability of domestic energy sources.
All terrestrial energy sources except nuclear, geothermal and tidal are from current solar insolation or from fossil remains of plant and animal life that relied directly and indirectly upon sunlight, respectively. Ultimately, solar energy itself is the result of the Sun's nuclear fusion. Geothermal power from hot, hardened rock above the magma of the Earth's core is the result of the decay of radioactive materials present beneath the Earth's crust, and nuclear fission relies on man-made fission of heavy radioactive elements in the Earth's crust; in both cases these elements were produced in supernova explosions before the formation of the solar system.
Relative cost of electricity generated by different sources
See: *Relative cost of electricity generated by different sources
Fossil fuels
This section needs additional citations for verification. (April 2008) |
Fossil fuels sources burn coal or hydrocarbon fuels, which are the remains of the decomposition of plants and animals. There are three main types of fossil fuels: coal, petroleum, and natural gas. Another fossil fuel, liquefied petroleum gas (LPG), is principally derived from the production of natural gas. Heat from burning fossil fuel is used either directly for space heating and process heating, or converted to mechanical energy for vehicles, industrial processes, or electrical power generation.
Pros
- The technology and infrastructure already exist for the use of fossil fuels.
- Petroleum energy density in terms of volume (cubic space) and mass (weight) is superior to some alternative energy sources (or energy storage devices, like a battery (electricity)).
- Fossil fuels are currently more economical, and more suitable for decentralized energy use
Cons
- Petroleum-powered vehicles are very inefficient. Only about 30% of the energy from the fuel they consume is converted into mechanical energy.[1] The rest of the fuel-source energy is inefficiently expended as waste heat. The heat and gaseous pollution emissions harm our environment.
- The inefficient atmospheric combustion (burning) of fossil fuels in vehicles, buildings, and power plants contributes to urban heat islands.[2]
- The combustion of fossil fuels leads to the release of pollution into the atmosphere. According to the Union of Concerned Scientists, a typical coal plant produces in one year:[3]
- 3,700,000 tons of carbon dioxide (CO2), could be the primary cause of global warming.
- 10,000 tons of sulfur dioxide (SO2), the leading cause of acid rain.
- 500 tons of small airborne particles, which result in chronic bronchitis, aggravated asthma, and premature death, in addition to haze-obstructed visibility.
- 10,200 tons of nitrogen oxides (NOx), (from high-temperature atmospheric combustion), leading to formation of ozone (smog) which inflames the lungs, burning lung tissue making people more susceptible to respiratory illness.
- 720 tons of carbon monoxide (CO), resulting in headaches and additional stress on people with heart disease.
- 220 tons of hydrocarbons, toxic volatile organic compounds (VOC), which form ozone.
- 170 pounds (77 kg) of mercury, where just 1⁄70 of a teaspoon deposited on a 25-acre (100,000 m2) lake can make the fish unsafe to eat.
- 225 pounds (102 kg) of arsenic, which will cause cancer in one out of 100 people who drink water containing 50 parts per billion.
- 114 pounds (52 kg) of lead, 4 pounds (1.8 kg) of cadmium, other toxic heavy metals, and trace amounts of uranium.
- Dependence on fossil fuels from volatile regions or countries creates energy security risks for dependent countries. Oil dependence in particular has led to war, major funding of radical terrorists, monopolization, and socio-political instability.
- Fossil fuels are non-renewable, un-sustainable resources, which will eventually decline in production[4] and become exhausted, with dire consequences to societies that remain highly dependent on them. (Fossil fuels are actually slowly forming continuously, but we are using them up at a rate approximately 100,000 times faster than they are formed.)

- Extracting fossil fuels is becoming more difficult as we consume the most accessible fuel deposits. Extraction of fossil fuels is becoming more expensive and more dangerous as mines get deeper and oil rigs must drill deeper, and go further out to sea.[5]
- Extraction of fossil fuels results in extensive environmental degradation, such as the strip mining and mountaintop removal of coal.[citation needed]

Since these power plants are thermal engines, and are typically quite large, waste heat disposal becomes an issue at high ambient temperature. Thus, at a time of peak demand, a power plant may need to be shut down or operate at a reduced power level, as sometimes do nuclear power plants, for the same reasons.[citation needed]
Nuclear energy


countries building its first reactors
countries building new reactors
countries planning/considering its first reactors
countries planning/considering new reactors
countries with reactors, but no plans for expansion or phase-out
countries with reactors considering phase-out
countries without commercial reactors/do not have nuclear capabilities
countries declared itself free of nuclear power and weapons
Nuclear fission
Nuclear power stations use nuclear fission to generate energy by the reaction of uranium-235 inside a nuclear reactor. The reactor uses uranium rods, the atoms of which are split in the process of fission, releasing a large amount of energy. The process continues as a chain reaction with other nuclei. The energy heats water to create steam, which spins a turbine generator, producing electricity.
Depending on the type of fission fuel considered, estimates for existing supply at known usage rates varies from several decades for the currently popular Uranium-235 to thousands of years for uranium-238. At the present rate of use, there are (as of 2007) about 70 years left of known uranium-235 reserves economically recoverable at a uranium price of US$ 130/kg.[6] The nuclear industry argue that the cost of fuel is a minor cost factor for fission power, more expensive, more difficult to extract sources of uranium could be used in the future, such as lower-grade ores, and if prices increased enough, from sources such as granite and seawater.[6] Increasing the price of uranium would have little effect on the overall cost of nuclear power; a doubling in the cost of natural uranium would increase the total cost of nuclear power by 5 percent. On the other hand, if the price of natural gas was doubled, the cost of gas-fired power would increase by about 60 percent.[7]
Opponents on the other hand argue that the correlation between price and production is not linear, but as the ores' concentration becomes smaller, the difficulty (energy and resource consumption are increasing, while the yields are decreasing) of extraction rises very fast, and that the assertion that a higher price will yield more uranium is overly optimistic; for example a rough estimate predicts that the extraction of uranium from granite will consume at least 70 times more energy than what it will produce in a reactor. As many as eleven countries have depleted their uranium resources, and only Canada has mines left which produce better than 1% concentration ore.[8] Seawater seems to be equally dubious as a source.[9] As a consequence an eventual doubling in the price of uranium will give a marginal increase in the volumes that are being produced.
Another alternative would be to use thorium as fission fuel. Thorium is three times more abundant in Earth's crust than uranium,[10] and much more of the thorium can be used (or, more precisely, bred into Uranium-233, reprocessed and then used as fuel). India has around 32 percent of the world’s reserves of thorium and intends on using it for itself because the country has run out of uranium.[11]
The use of thorium as an alternative fuel is one innovation being explored by the International Project on Innovative Nuclear Reactors and Fuel Cycles (INPRO)[12], conducted by the International Atomic Energy Agency (IAEA). Thorium fuel is also a key component of the NEOP nuclear energy systems in development by the Neopanora Institute-Network of Energy Technology (NINET)[13].
Current light water reactors burn the nuclear fuel poorly, leading to energy waste. Nuclear reprocessing[14] or burning the fuel better using different reactor designs would reduce the amount of waste material generated and allow better use of the available resources. As opposed to current light water reactors which use uranium-235 (0.7 percent of all natural uranium), fast breeder reactors convert the more abundant uranium-238 (99.3 percent of all natural uranium) into plutonium for fuel. It has been estimated that there is anywhere from 10,000 to five billion years worth of Uranium-238 for use in these power plants.[15] Fast breeder technology has been used in several reactors. However, the fast breeder reactors at Dounreay in Scotland, Monju in Japan and the Superphénix at Creys-Malville in France, in particular, have all had difficulties and were not economically competitive and most have been decommissioned. The People's Republic of China intends to build breeders.[16] India has run out of uranium and is building thermal breeders that can convert Th-232 into U-233 and burn it.[11]
Some nuclear engineers think that pebble bed reactors, in which each nuclear fuel pellet is coated with a ceramic coating, are inherently safe and are the best solution for nuclear power. They can also be configured to produce hydrogen for hydrogen vehicles. China has plans to build pebble bed reactors configured to produce hydrogen.
The possibility of nuclear meltdowns and other reactor accidents, such as the Three Mile Island accident and the Chernobyl disaster, have caused much public fear. Research is being done to lessen the known problems of current reactor technology by developing automated and passively safe reactors. Historically, however, coal and hydropower power generation have both been the cause of more deaths per energy unit produced than nuclear power generation.[17][18] Various kinds of energy infrastructure might be attacked by terrorists, including nuclear power plants, hydropower plants, and liquified natural gas tankers. Nuclear proliferation is the spread from nation to nation of nuclear technology, including nuclear power plants but especially nuclear weapons. New technology like SSTAR ("small, sealed, transportable, autonomous reactor") may lessen this risk.
The long-term radioactive waste storage problems of nuclear power have not been fully solved. Several countries have considered using underground repositories. Nuclear waste takes up little space compared to wastes from the chemical industry which remain toxic indefinitely.[14] Spent fuel rods are now stored in concrete casks close to the nuclear reactors.[19] The amounts of waste could be reduced in several ways. Both nuclear reprocessing and fast breeder reactors could reduce the amounts of waste. Subcritical reactors or fusion reactors could greatly reduce the time the waste has to be stored.[20] Subcritical reactors may also be able to do the same to already existing waste. The only long-term way of dealing with waste today is by geological storage.
The economics of nuclear power is not simple to evaluate, because of high capital costs for building and very low fuel costs. Comparison with other power generation methods is strongly dependent on assumptions about construction timescales and capital financing for nuclear plants. See Economics of new nuclear power plants.
Depending on the source different energy return on energy investment (EROI) are claimed. Advocates (using life cycle analysis) argue that it takes 4–5 months of energy production from the nuclear plant to fully pay back the initial energy investment.[21] Opponents claim that it depends on the grades of the ores the fuel came from, so a full payback can vary from 10 to 18 years, and that the advocates' claim was based on the assumption of high grade ores (the yields are getting worse, as the ores are leaner; for less than 0.02% ores, the yield is less than 50%).[22]
Advocates also claim that it is possible to increase the number of plants fairly rapidly. Typical new reactor designs have a construction time of three to four years.[23] In 1983, 43 plants were being built, before an unexpected fall in fossil fuel prices stopped most new construction. Developing countries like India and China are rapidly increasing their nuclear energy use.[24][25] However, a Council on Foreign Relations report on nuclear energy argues that a rapid expansion of nuclear power may create shortages in building materials such as reactor-quality concrete and steel, skilled workers and engineers, and safety controls by skilled inspectors. This would drive up current prices.[26]
However, at present, nuclear energy is in decline, according to a report 'World Nuclear Industry Status Report 2007' presented by the Greens/EFA group in the European Parliament. The report outlines that the proportion of nuclear energy in power production has decreased in 21 out of 31 countries, with five fewer functioning nuclear reactors than five years ago. There are currently 32 nuclear power plants under construction or in the pipeline, 20 fewer than at the end of the 1990s [27][28].
Pros
This section needs additional citations for verification. (April 2008) |
- The energy content of a kilogram of uranium or thorium, if spent nuclear fuel is reprocessed and fully utilized, is equivalent to about 3.5 million kilograms of coal.[citation needed]
- The cost of making nuclear power, with current legislation, is about the same as making coal power, which is considered very inexpensive (see Economics of new nuclear power plants). If a carbon tax is applied, nuclear does not have to pay anything because nuclear does not emit greenhouse gasses such as CO2 nor toxic gases NO, CO, SO2, arsenic, etc. that are emitted by coal power plants.[citation needed]
- Nuclear power does not produce any primary air pollution or release carbon dioxide and sulfur dioxide into the atmosphere. Therefore, it contributes only a small amount to global warming or acid rain.[citation needed]
- Raw material extraction is much safer for nuclear power compared to coal. Coal mining is the second most dangerous occupation in the United States.[29] Nuclear energy is much safer per capita than coal derived energy.[citation needed]
- For the same amount of electricity, the life cycle emissions of nuclear is about 4% of coal power. Depending on the report, hydro, wind, and geothermal are sometimes ranked lower, while wind and hydro are sometimes ranked higher (by life cycle emissions).[30][31]
- According to a Stanford study, fast breeder reactors have the potential to power humans on earth for billions of years, making it sustainable.[15]
Cons
This section needs additional citations for verification. (April 2008) |
Actinides[32] by decay chain | Half-life range (a) |
Fission products of 235U by yield[33] | ||||||
---|---|---|---|---|---|---|---|---|
4n | 4n + 1 | 4n + 2 | 4n + 3 | 4.5–7% | 0.04–1.25% | <0.001% | ||
228Ra№ | 4–6 a | 155Euþ | ||||||
248Bk[34] | > 9 a | |||||||
244Cmƒ | 241Puƒ | 250Cf | 227Ac№ | 10–29 a | 90Sr | 85Kr | 113mCdþ | |
232Uƒ | 238Puƒ | 243Cmƒ | 29–97 a | 137Cs | 151Smþ | 121mSn | ||
249Cfƒ | 242mAmƒ | 141–351 a |
No fission products have a half-life | |||||
241Amƒ | 251Cfƒ[35] | 430–900 a | ||||||
226Ra№ | 247Bk | 1.3–1.6 ka | ||||||
240Pu | 229Th | 246Cmƒ | 243Amƒ | 4.7–7.4 ka | ||||
245Cmƒ | 250Cm | 8.3–8.5 ka | ||||||
239Puƒ | 24.1 ka | |||||||
230Th№ | 231Pa№ | 32–76 ka | ||||||
236Npƒ | 233Uƒ | 234U№ | 150–250 ka | 99Tc₡ | 126Sn | |||
248Cm | 242Pu | 327–375 ka | 79Se₡ | |||||
1.33 Ma | 135Cs₡ | |||||||
237Npƒ | 1.61–6.5 Ma | 93Zr | 107Pd | |||||
236U | 247Cmƒ | 15–24 Ma | 129I₡ | |||||
244Pu | 80 Ma |
... nor beyond 15.7 Ma[36] | ||||||
232Th№ | 238U№ | 235Uƒ№ | 0.7–14.1 Ga | |||||
|
- The improper operation of a nuclear reactor with no containment vessel can be catastrophic in the event of an uncontrolled power increase in the reactor. For example, the Chernobyl disaster in the Ukraine (former USSR) affected large areas of Europe by moderate radioactive contamination; parts of the Ukraine and Belarus continue to be affected by radioactive fallout.[37]
- Transuranic waste produced from nuclear fission of uranium is poisonous and highly radioactive. Breeder reactors could burn this waste as fuel, fissioning transuranics into much faster-decaying fission products which stabilize at a relatively low level of radioactivity in 100–500 years, but recycling plutonium as MOX fuel in current light water reactors merely transmutes between isotopes of plutonium and offers little reduction in radioactivity.
Without nuclear reprocessing, whole spent fuel bundles containing transuranic waste must be stored in spent fuel pools, dry cask storage, or a geological repository.
- There can be connections between nuclear power and nuclear weapon proliferation, since many reactor designs require large-scale uranium enrichment facilities.[citation needed]
- Some claim that uranium ore is a limited resource and estimate that current supplies will fail to meet demand in 2026, provided no other deposits are discovered. This claim is strongly disputed; also, breeder reactors would extract about 100 times as much energy from the same amount of uranium.
- The limited liability for the owner of a nuclear power plant in case of a nuclear accident differs per nation while nuclear installations are sometimes built close to national borders.[38]
- Since nuclear power plants are typically quite large power plants, and are, fundamentally, thermal engines, waste heat disposal becomes more difficult at higher ambient temperature. Thus, at a time of peak demand for power for air-conditioning, a power reactor may need to be shut down or operate at a reduced power level, as do large coal-fired plants, for the same reasons.[39]
Nuclear fusion
Fusion power could solve many of the problems of fission power (the technology mentioned above) but, despite research having started in the 1950s, no commercial fusion reactor is expected before 2050.[40] Many technical problems remain unsolved. Proposed fusion reactors commonly use deuterium, an isotope of hydrogen, as fuel and in most current designs also lithium. Assuming a fusion energy output equal to the current global output and that this does not increase in the future, then the known current lithium reserves would last 3000 years, lithium from sea water would last 60 million years, and a more complicated fusion process using only deuterium from sea water would have fuel for 150 billion years.[41]
Renewable sources
Renewable energy is an alternative to fossil fuels and nuclear power, and was commonly called alternative energy in the 1970s and 1980s.
Biomass, biofuels, and vegetable oil
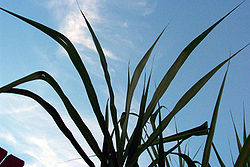
- Main articles: Alcohol fuel, Biomass, Vegetable oil economy, vegetable oil as fuel, biodiesel, Ethanol fuel
Biomass production involves using garbage or other renewable resources such as corn or other vegetation to generate electricity. When garbage decomposes, the methane produced is captured in pipes and later burned to produce electricity. Vegetation and wood can be burned directly to generate energy, like fossil fuels, or processed to form alcohols.
Vegetable oil is generated from sunlight, H2O, and CO2 by plants. It is safer to use and store than gasoline or diesel as it has a higher flash point. Straight vegetable oil works in diesel engines if it is heated first. Vegetable oil can also be transesterified to make biodiesel, which burns like normal diesel.
Pros
- Biomass production can be used to burn organic byproducts resulting from agriculture.
- Biomass is abundant on Earth and is renewable. Biomass is found throughout the world, a fact that should alleviate energy pressures in third world nations.
- When methods of biomass production other than direct combustion of plant mass are used, such as fermentation and pyrolysis, there is little effect on the environment. Alcohols and other fuels produced by these alternative methods are clean burning and are feasible replacements to fossil fuels.
- Since CO2 is first taken out of the atmosphere to make the vegetable oil and then put back after it is burned in the engine, there is no net increase in CO2. However, there is still the emissions due to fossil fuel used in growing and producing biofuel.
- Vegetable oil has a higher flash point and therefore is safer than most fossil fuels.
- Transitioning to vegetable oil could be relatively easy as biodiesel works where diesel works, and straight vegetable oil takes relatively minor modifications.
- The world already produces more than 100 billion gallons a year for the food industry, so we have experience making it.
- Algaculture has the potential to produce far more vegetable oil per acre than current plants.
- Infrastructure for biodiesel around the World is significant and growing.
Cons
This section needs additional citations for verification. (April 2008) |
- Direct combustion of any carbon-based fuel leads to air pollution similar to that from fossil fuels.[42]
- Some researchers claim that when biomass crops are the product of intensive farming, ethanol fuel production results in a net loss of energy after one accounts for the fuel costs of petroleum and natural-gas fertilizer production, farm equipment, and the distillation process.[43]
- Direct competition with land use for food production and water use. As this decreases food supply, the price of food increases world wide.
- Current production methods would require enormous amounts of land to replace all gasoline and diesel. With current technology, it is not feasible for biofuels to replace the demand for petroleum.
- Even with the most-optimistic current energy return on investment claims, in order to use 100% solar energy to grow corn and produce ethanol (fueling machinery with ethanol, distilling with heat from burning crop residues, using NO fossil fuels at all), the consumption of ethanol to replace only the current U.S. petroleum use would require three quarters of all the cultivated land on the face of the Earth.[44]
Geothermal energy
Geothermal energy harnesses the heat energy present underneath the Earth. Two wells are drilled. One well injects water into the ground to provide water. The hot rocks heat the water to produce steam. The steam that shoots back up the other hole(s) is purified and is used to drive turbines, which power electric generators. When the water temperature is below the boiling point of water a binary system is used. A low boiling point liquid is used to drive a turbine and generator in a closed system similar to a refrigeration unit running in reverse.
Pros
- Geothermal energy is base load power.[45]
- Economically feasible in high grade areas now.[45]
- Low deployment costs.[45]
- Geothermal power plants have a high capacity factor; they run continuously day and night with an uptime typically exceeding 95%.
- Once a geothermal power station is implemented, there is no cost for fuel, only for operations, maintenance and return on capital investment.[46]
- Since geothermal power stations consume no fuel,there is no environmental impact associated with emissions or fuel handling.
- Geothermal is now feasible in areas where the Earth's crust is thicker. Using enhanced geothermal technology, it is possible to drill deeper and inject water to generate geothermal power.[47]
- Geothermal energy does not produce air or water pollution if performed correctly.
Cons
- Geothermal power extracts small amounts of minerals, such as sulfur, that are removed prior to feeding the turbine and re-injecting the water back into the injection well.[citation needed]
- Geothermal power requires locations that have suitable subterranean temperatures within 5 km of surface.
- Some geothermal stations have created geological instability, even causing earthquakes strong enough to damage buildings.[48]
Hydroelectric energy
In hydro energy, the gravitational descent of a river is compressed from a long run to a single location with a dam or a flume. This creates a location where concentrated pressure and flow can be used to turn turbines or water wheels, which drive a mechanical mill or an electric generator.[49]
Pros
- Hydroelectric power stations can promptly increase to full capacity, unlike other types of power stations. This is because water can be accumulated above the dam and released to coincide with peak demand.
- Electricity can be generated constantly, so long as sufficient water is available.
- Hydroelectric power produces no primary waste or pollution.
- Hydropower is a renewable resource.
- Much hydroelectric capacity is still undeveloped, such as in Africa.
- The resulting lake can have additional benefits such as doubling as a reservoir for irrigation, and leisure activities such as watersports and fishing, for example Kielder Water in Northumberland, UK.
Cons
- The construction of a dam can have a serious environmental impact on the surrounding areas. The amount and the quality of water downstream can be affected, which affects plant life both aquatic, and land-based. Because a river valley is being flooded, the local habitat of many species are destroyed, while people living nearby may have to relocate their homes.
- Hydroelectricity can only be used in areas where there is a sufficient and continuing supply of water.
- Flooding submerges large forests (if they have not been harvested). The resulting anaerobic decomposition of the carboniferous materials releases methane, a greenhouse gas.
- Dams can contain huge amounts of water. As with every energy storage system, failure of containment can lead to catastrophic results, e.g. flooding
- Dams create large lakes that may have adverse effects on Earth tectonic system causing intense earthquakes.[50]
- Hydroelectric plants rarely can be erected near load centers, requiring long transmission lines.
Solar power

- Main articles: Solar energy, Photovoltaics
Solar power involves using solar cells to convert sunlight into electricity, using sunlight hitting solar thermal panels to convert sunlight to heat water or air, using sunlight hitting a parabolic mirror to heat water (producing steam), or using sunlight entering windows for passive solar heating of a building. It would be advantageous to place solar panels in the regions of highest solar radiation[51]. In the Phoenix, Arizona area, for example, the average annual solar radiation is 5.7 kW·h/(m²·day),[52] or 2.1 MW·h/(m²·yr). Electricity demand in the continental U.S. is 3.7×1012 kW·h per year. Thus, at 20% efficiency, an area of approximately 3500 square miles (3% of Arizona's land area) would need to be covered with solar panels to replace all current electricity production in the US with solar power. The average solar radiation in the United States is 4.8 kW·h/(m²·day),[53] but reaches 8–9 kWh/m²/day in parts of the Southwest.
China is increasing worldwide silicon wafer capacity for photovoltaics to 2,000 metric tons by July 2008, and over 6,000 metric tons by the end of 2010.[54] Significant international investment capital is flowing into China to support this opportunity. China is building large subsidized off-the-grid solar-powered cities in Huangbaiyu and Dongtan Eco City. Much of the design was done by Americans such as William McDonough.[55]
Pros
- Solar power imparts no fuel costs.
- Solar power is a renewable resource. As long as the Sun exists, its energy will reach Earth.
- Solar power generation releases no water or air pollution, because there is no combustion of fuels.
- In sunny countries, solar power can be used in remote locations, like a wind turbine. This way, isolated places can receive electricity, when there is no way to connect to the power lines from a plant.
- Solar energy can be used very efficiently for heating (solar ovens, solar water and home heaters) and daylighting.
- Coincidentally, solar energy is abundant in regions that have the largest number of people living off grid — in developing regions of Africa, Indian subcontinent and Latin America. Hence cheap solar, when available, opens the opportunity to enhance global electricity access considerably, and possibly in a relatively short time period.[56]
- Passive solar building design and zero energy buildings are demonstrating significant energy bill reduction, and some are cost-effectively off the grid.
- Photovoltaic equipment cost has been steadily falling and the production capacity is rapidly rising.
- Distributed point-of-use photovoltaic systems eliminate expensive long-distance electric power transmission losses.
- Photovoltaics are much more efficient in their conversion of solar energy to usable energy than biofuel from plant materials.[57]
Cons
- Solar electricity is currently more expensive than grid electricity.
- Solar heat and electricity are not available at night and may be unavailable because of weather conditions; therefore, a storage or complementary power system is required for off-the-grid applications.
- Solar cells produce DC which must be converted to AC (using a grid tie inverter) when used in currently existing distribution grids. This incurs an energy loss of 4–12%.[58]
- The energy payback time — the time necessary for producing the same amount of energy as needed for building the power device — for photovoltaic cells is about 1–5 years, depending primarily on location.[59]
Tidal Power Generation
Tidal power can be extracted from Moon-gravity-powered tides by locating a water turbine in a tidal current, or by building impoundment pond dams that admit-or-release water through a turbine. The turbine can turn an electrical generator, or a gas compressor, that can then store energy until needed. Coastal tides are a source of clean, free, renewable, and sustainable energy.[60]
Pros
- Tidal power is free once the dam is built. This is because tidal power harnesses the natural power of tides and does not consume fuel. In addition, the maintenance costs associated with running a tidal station are relatively inexpensive.
- Tides are very reliable because it is easy to predict when high and low tides will occur. The tide goes in and out twice a day usually at the predicted times. This makes tidal energy easy to maintain, and positive and negative spikes in energy can be managed.
- Tidal energy is renewable, because nothing is consumed in the rising of tides. Tidal power relies on the gravitational pull of the Moon and Sun, which pull the sea backwards and forwards, generating tides.
Cons
- Tidal power is not currently economically feasible, because the initial costs of building a dam are tremendous. Furthermore, it only provides power for around 10 hours each day, when the tide is moving in or out of the basin.
- The barrage construction can affect the transportation system in water. Boats may not be able to cross the barrage, and commercial ships, used for transport or fishery, need to find alternative routes or costly systems to go through the barrage.
- The erection of a barrage may affect the aquatic ecosystems surrounding it. The environment affected by the dam is very wide, altering areas numerous miles upstream and downstream. For example, many birds rely on low tides to unearth mud flats, which are used as feeding areas.
- Maximum energy production is limited to 2.5 terawatts. This is the total amount of tidal dissipation or the friction measured by the slowing of the lunar orbit.
Wind power
This section needs additional citations for verification. (April 2008) |

This type of energy harnesses the power of the wind to propel the blades of wind turbines. These turbines cause the rotation of magnets, which creates electricity. Wind towers are usually built together on wind farms.
Pros
- Wind power produces no water or air pollution that can contaminate the environment, because there are no chemical processes involved in wind power generation. Hence, there are no waste by-products, such as carbon dioxide.[61]
- Power from the wind does not contribute to global warming because it does not generate greenhouse gases.[citation needed]
- Wind generation is a renewable source of energy, which means that we will never run out of it.
- Wind towers can be beneficial for people living permanently, or temporarily, in remote areas. It may be difficult to transport electricity through wires from a power plant to a far-away location and thus, wind towers can be set up at the remote setting.[citation needed]
- Farming and grazing can still take place on land occupied by wind turbines.[citation needed]
- Those utilizing wind power in a grid-tie configuration will have backup power in the event of a power outage.[citation needed]
- Because of the ability of wind turbines to coexist within agricultural fields, siting costs are frequently low.[citation needed]
Cons
- Wind is unpredictable; therefore, wind power is not predictably available. When the wind speed decreases less electricity is generated. This makes wind power unsuitable for base load generation.[citation needed]
- Wind farms may be challenged in communities that consider them an eyesore or obstruction.[62]
- Wind farms, depending on the location and type of turbine, may negatively affect bird migration patterns, and may pose a danger to the birds themselves (primarily an issue with older/smaller turbines).[citation needed]
- Windfarms may interfere with radar creating a hole in radar coverage and so affect national security.[63]
- Tall wind turbines have been proven to impact doppler radar towers and affect weather forecasting in a negative way. This can be prevented by not having the wind turbines in the radar's line of sight.[64]
Increased efficiency in energy use
This section needs additional citations for verification. (April 2008) |
Efficiency is increasing by about 2% a year, and absorbs most of the requirements for energy development. New technology makes better use of already available energy through improved efficiency, such as more efficient fluorescent lamps, engines, and insulation. Using heat exchangers, it is possible to recover some of the energy in waste warm water and air, for example to preheat incoming fresh water. Hydrocarbon fuel production from pyrolysis could also be in this category, allowing recovery of some of the energy in hydrocarbon waste. Already existing power plants often can and usually are made more efficient with minor modifications due to new technology. New power plants may become more efficient with technology like cogeneration. New designs for buildings may incorporate techniques like passive solar. Light-emitting diodes are gradually replacing the remaining uses of light bulbs. Note that none of these methods allows perpetual motion, as some energy is always lost to heat.
Mass transportation increases energy efficiency compared to widespread conventional automobile use while air travel is regarded as inefficient. Conventional combustion engine automobiles have continually improved their efficiency and may continue to do so in the future, for example by reducing weight with new materials. Hybrid vehicles can save energy by allowing the engine to run more efficiently, regaining energy from braking, turning off the motor when idling in traffic, etc. More efficient ceramic or diesel engines can improve mileage. Electric vehicles such as Maglev, trolleybuses, and PHEVs are more efficient during use (but maybe not if doing a life cycle analysis) than similar current combustion based vehicles, reducing their energy consumption during use by 1/2 to 1/4. Microcars or motorcycles may replace automobiles carrying only one or two people. Transportation efficiency may also be improved by in other ways, see automated highway system.
Electricity distribution may change in the future. New small scale energy sources may be placed closer to the consumers so that less energy is lost during electricity distribution. New technology like superconductivity or improved power factor correction may also decrease the energy lost. Distributed generation permits electricity "consumers," who are generating electricity for their own needs, to send their surplus electrical power back into the power grid.
Energy transportation
This section needs additional citations for verification. (April 2008) |
While new sources of energy are only rarely discovered or made possible by new technology, distribution technology continually evolves. The use of fuel cells in cars, for example, is an anticipated delivery technology. This section presents some of the more common delivery technologies that have been important to historic energy development. They all rely in some way on the energy sources listed in the previous section.

- Fuels
- Shipping is a flexible delivery technology that is used in the whole range of energy development regimes from primitive to highly advanced. Currently, coal, petroleum and their derivatives are delivered by shipping via boat, rail, or road. Petroleum and natural gas may also be delivered via pipeline and coal via a Slurry pipeline. Refined hydrocarbon fuels such as gasoline and LPG may also be delivered via aircraft. Natural gas pipelines must maintain a certain minimum pressure to function correctly. Ethanol's corrosive properties prevent it from being transported via pipeline. The higher costs of ethanol transportation and storage are often prohibitive.[65]
- Electric grids
- Electricity grids are the networks used to transmit and distribute power from production source to end user, when the two may be hundreds of kilometres away. Sources include electrical generation plants such as a nuclear reactor, coal burning power plant, etc. A combination of sub-stations, transformers, towers, cables, and piping are used to maintain a constant flow of electricity.

- Grids may suffer from transient blackouts and brownouts, often due to weather damage. During certain extreme space weather events solar wind can interfere with transmissions.
- Grids also have a predefined carrying capacity or load that cannot safely be exceeded. When power requirements exceed what's available, failures are inevitable. To prevent problems, power is then rationed.
- Industrialised countries such as Canada, the US, and Australia are among the highest per capita consumers of electricity in the world, which is possible thanks to a widespread electrical distribution network. The US grid is one of the most advanced, although infrastructure maintenance is becoming a problem.
- CurrentEnergy provides a realtime overview of the electricity supply and demand for California, Texas, and the Northeast of the US. African countries with small scale electrical grids have a correspondingly low annual per capita usage of electricity. One of the most powerful power grids in the world supplies power to the state of Queensland, Australia.



Energy storage
- Main articles: Energy storage, grid energy storage
Methods of energy storage have been developed, which transform electrical energy into forms of potential energy. A method of energy storage may be chosen on the basis of stability, ease of transport, ease of energy release, or ease of converting free energy from the natural form to the stable form.
Compressed air vehicles
- Main articles: Compressed air vehicle, Air car
The Indian company, Tata, is planning to release a compressed air powered car in 2008.
Battery-powered vehicles
- Main articles: battery, battery electric vehicle
Batteries are used to store energy in a chemical form. As an alternative energy, batteries can be used to store energy in battery electric vehicles. Battery electric vehicles can be charged from the grid when the vehicle is not in use. Because the energy is derived from electricity, battery electric vehicles make it possible to use other forms of alternative energy such as wind, solar, geothermal, nuclear, or hydroelectric.
Pros
- Current lead acid battery technology offers 50+ miles range on one charge.[66]
- Batteries make it possible for stationary alternative energy generation such as solar, wind, hydroelectric, or nuclear
- Electric motors are 90% efficient compared to about 20% efficiency of an internal combustion engine.[67]
- Battery electric vehicles have fewer moving parts than internal combustion engines, thus improving the reliability of the vehicle.
- Battery electric vehicles are quiet compared to internal combustion engines.
- Operation of a battery electric vehicle is approximately 2 to 4 cents per mile, about a sixth the price of operating a gasoline vehicle.[68]
- The use of battery electric vehicles may reduce the dependency on fossil fuels, depending on the source of the electricity.
Cons
- Current battery technology is expensive.
- Some of the principal materials required in battery production, such as Lithium, are becoming increasingly scarce [2]
- Battery electric vehicles have a relative short range compared to internal combustion engine vehicles, and recharge times are typically much longer than the time to fill a conventional fuel tank.
- Some batteries (like the Gel battery and the Lead-acid battery) are highly toxic. Spent vehicle batteries present a potential environmental hazard. They are all best recycled at end of life.
- Grid infrastructure and output would need to be improved significantly to accommodate a mass-adoption of grid-charged electric vehicles, although the problem is less if electric vehicles will recharge primarily at night, when electricity demand is currently lowest.
- Some batteries perform less efficiently in cold weather, and a battery electric vehicle lacks a convenient source of waste engine heat to warm the passenger compartment. Accordingly, the test-marketing of some electric vehicles such as the General Motors EV1 took place in warm-weather parts of Arizona and California.
- Some batteries also perform poorly in hot weather.
Hydrogen economy
Hydrogen can be manufactured at roughly 77 percent thermal efficiency by the method of steam reforming of natural gas.[69] When manufactured by this method it is a derivative fuel like gasoline; when produced by electrolysis of water, it is a form of chemical energy storage as are storage batteries, though hydrogen is the more versatile storage mode since there are two options for its conversion to useful work: (1) a fuel cell can convert the chemicals hydrogen and oxygen into water, and in the process, produce electricity, or (2) hydrogen can be burned (less efficiently than in a fuel cell) in an internal combustion engine.
Pros
- Hydrogen is colorless, odorless and entirely non-polluting, yielding pure water vapor (with minimal NOx) as exhaust when combusted in air. This eliminates the direct production of exhaust gases that lead to smog, and carbon dioxide emissions that enhance the effect of global warming.
- Hydrogen is the lightest chemical element and has the best energy-to-weight ratio of any fuel (not counting tank mass).
- Hydrogen can be produced anywhere; it can be produced domestically from the decomposition of water. Hydrogen can be produced from domestic sources and the price can be established within the country.
Cons
- Other than some volcanic emanations, hydrogen does not exist in its pure form in the environment, because it reacts so strongly with oxygen and other elements.[70]
- It is impossible to obtain hydrogen gas without expending energy in the process. There are three ways to manufacture hydrogen;
- By breaking down hydrocarbons — mainly methane (steam reforming). If oil or gases are used to provide this energy, fossil fuels are consumed, forming pollution and nullifying the value of using a fuel cell. It would be more efficient to use fossil fuel directly.
- By electrolysis of water — The process of splitting water into oxygen and hydrogen using electrolysis. It has been calculated that it takes 1.4 joules of electricity to produce 1 joule of hydrogen (Pimentel, 2002).
- By reacting water with a metal such as sodium, potassium, or boron. Chemical by-products would be sodium oxide, potassium oxide, and boron oxide. Processes exist which could recycle these elements back into their metal form for re-use with additional energy input, further eroding the energy return on energy invested.
- There is currently modest fixed infastructure for distribution of hydrogen that is centrally produced,[71] amounting to several hundred kilometers of pipeline. An alternative would be transmission of electricity over the existing electrical network to small-scale electrolyzers to support the widespread use of hydrogen as a fuel.
- Hydrogen is difficult to handle, store, and transport. It requires heavy, cumbersome tanks when stored as compressed hydrogen, and complex insulating bottles if stored as a cryogenic liquid hydrogen. If it is needed at a moderate temperature and pressure, a metal hydride absorber may be needed. The transportation of hydrogen is also a problem because hydrogen leaks effortlessly from containers.
Energy storage types
- Chemical
- Some natural forms of energy are found in stable chemical compounds such as fossil fuels. Most systems of chemical energy storage result from biological activity, which store energy in chemical bonds. Man-made forms of chemical energy storage include hydrogen fuel, synthetic hydrocarbon fuel, batteries and explosives such as cordite and dynamite.
- Gravitational
- Dams can be used to store energy, by using excess energy to pump water into the reservoir. When electrical energy is required, the process is reversed. The water then turns a turbine, generating electricity. Hydroelectric power is currently an important part of the world's energy supply, generating one-fifth of the world's electricity.[72]
- Electrical capacitance
- Electrical energy may be stored in capacitors. Capacitors are often used to produce high intensity releases of energy (such as a camera's flash).
- Mechanical
- Pressure:
- Energy may also be stored pressurized gases or alternatively in a vacuum. Compressed air, for example, may be used to operate vehicles and power tools. Large-scale compressed air energy storage facilities are used to smooth out demands on electricity generation by providing energy during peak hours and storing energy during off-peak hours. Such systems save on expensive generating capacity since it only needs to meet average consumption rather than peak consumption.[73]
- Flywheels and springs
- Energy can also be stored in mechanical systems such as springs or flywheels. Flywheel energy storage is currently being used for uninterruptible power supplies.
- Gravitational potential energy storage devices
- Energy can be stored by lifting a heavy object vertically using a cable and winch system. Energy can be harvested again by lowering the weight against a dynamo. By using the formula Ug = mgh where 1 Ug is equal to 196 J, and since 1 watt hour = 3600 J it can be determined that an object weighting 1 ton lifted 1 m into the air can store approx. 533 watt hours of energy. Increasing the vertical lift distance to 100 m and the weight to 200 tons leads to a device capable of storing approx. 10.67 mWh of energy. Large-scale implementation of such devices has the potential to serve as a method to store excess alternatively generated energy for when sunlight and/or wind is not available. The costs associated with this form of energy storage are low since the weight can consist of a wide variety of materials (building rubble for example) and also due to the extremely accessible technologies used.
- Thermal
- There are several technologies to store heat. Thermal energy from the sun, for example, can be stored in a reservoir or in the ground for daily or seasonal use.
Sustainability
The environmental movement emphasizes sustainability of energy use and development. Renewable energy is sustainable in its production; the available supply will not be diminished for the foreseeable future - millions or billions of years. "Sustainability" also refers to the ability of the environment to cope with waste products, especially air pollution. Sources which have no direct waste products (such as wind, solar, and hydropower) are seen as ideal in this regard.
The status of nuclear power is controversial. The uranium supply might last a very long time with nuclear reprocessing, with an almost-unlimited supply from sea water available once ground based mining is exhausted.
Fossil fuels such as petroleum, coal, and natural gas are not renewable. For example, the timing of worldwide peak oil production is being actively debated but it has already happened in some countries. Fossil fuels also make up the bulk of the world's current primary energy sources. With global demand for energy growing, the need to adopt alternative energy sources is also growing. Fossil fuels are also a major source of greenhouse gas emissions, leading to concerns about global warming if consumption is not reduced.
Energy conservation is an alternative or complementary process to energy development. It reduces the demand for energy by using it more efficiently.
Energy resilience
This article needs additional citations for verification. (September 2008) |
Some observers contend that the much talked about idea of “energy independence” is an unrealistic and opaque concept. They offer “energy resilience” as a more sensible goal and more aligned with economic, security and energy realities.
The notion of resilience in energy was detailed in the 1982 book Brittle Power: Energy Strategy for National Security.[74] The authors argued that simply switching to domestic energy would be no more secure inherently because the true weakness is the interdependent and vulnerable energy infrastructure of the United States. Key aspects such as gas lines and the electrical power grid are centralized and easily susceptible to major disruption.
They conclude that a “resilient energy supply” is necessary for both national security and the environment. They recommend a focus on energy efficiency and renewable energy that is more decentralized.[75]
More recently former Intel Corporation Chairman and CEO Andrew Grove has touted energy resilience, arguing that complete independence is infeasible given the global market for energy.[76] He describes energy resilience as the ability to adjust to interruptions in the supply of energy.
To this end he suggests the U.S. make greater use of electricity.[77] Electricity can be produced from a variety of sources. A diverse energy supply will be less impacted by the disruption in supply of any one source. He reasons that another feature of electrification is that electricity is “sticky” – meaning the electricity produced in the U.S. is more likely to stay there because it cannot be transported overseas.
According to Grove, a key aspect of advancing electrification and energy resilience will be converting the U.S. automotive fleet from gasoline-powered to electric-powered. This, in turn, will require the modernization and expansion of the electrical power grid. As organizations such as the Reform Institute have pointed out, advancements associated with the developing smart grid would facilitate the ability of the grid to absorb vehicles en masse connecting to it to charge their batteries.[78]
Future energy development
This section needs additional citations for verification. (April 2008) |


Extrapolations from current knowledge to the future offer a choice of energy futures[79]. Some predictions parallel the Malthusian catastrophe hypothesis. Numerous are complex models based scenarios as pioneered by Limits to Growth. Modeling approaches offer ways to analyze diverse strategies, and hopefully find a road to rapid and sustainable development of humanity. Short term energy crises are also a concern of energy development. Some extrapolations lack plausibility, particularly when they predict a continual increase in oil consumption.
Existing technologies for new energy sources, such as renewable energy technologies, particularly wind power and solar power, are promising. Nuclear fission is also promoted, and each need sustained research and development, including consideration of possible harmful side effects. Jacques Cousteau spoke of using the salinization of water at river estuaries as an energy source, which would not have any consequences for a million years, and then stopped to point out that since we are going to be on the planet for a billion years we had to be looking that far into the future. Nuclear fusion and artificial photosynthesis are other energy technologies being researched.
Energy production usually requires an energy investment. Drilling for oil or building a wind power plant requires energy. The fossil fuel resources (see above) that are left are often increasingly difficult to extract and convert. They may thus require increasingly higher energy investments. If the investment is greater than the energy produced, then the fossil resource is no longer an energy source. This means that a large part of the fossil fuel resources and especially the non-conventional ones cannot be used for energy production today. Such resources may still be exploited economically in order to produce raw materials for plastics, fertilizers or even transportation fuel but now more energy is consumed than produced. (They then become similar to ordinary mining reserves, economically recoverable but not net positive energy sources.) New technology may ameliorate this problem if it can lower the energy investment required to extract and convert the resources, although ultimately basic physics sets limits that cannot be exceeded.
Between 1950 and 1984, as the Green Revolution transformed agriculture around the globe, world grain production increased by 250%. The energy for the Green Revolution was provided by fossil fuels in the form of fertilizers (natural gas), pesticides (oil), and hydrocarbon fueled irrigation.[80] The peaking of world hydrocarbon production (peak oil) may lead to significant changes, and require sustainable methods of production.[81]
History of predictions about future energy development
Ever since the beginning of the Industrial Revolution, the question of the future of energy supplies has occupied economists.
- 1865 — William Stanley Jevons published The Coal Question in which he claimed that reserves of coal would soon be exhausted and that there was no prospect of oil being an effective replacement.
- 1885 — U.S. Geological Survey: Little or no chance of oil in California.
- 1891 — U.S. Geological Survey: Little or no chance of oil in Kansas or Texas.
- 1914 — U.S. Bureau of Mines: Total future production of 5.7 billion barrels (910,000,000 m3).
- 1939 — U.S. Department of the Interior: Reserves to last only 13 years.
- 1951 — U.S. Department of the Interior, Oil and Gas Division: Reserves to last 13 years.
(Data from Kahn et al. (1976) pp. 94–5 infra)
- 1956 — Geophysicist M. King Hubbert predicts U.S. oil production will peak between 1965 and 1970 (peaked in 1971). Also predicts world oil production will peak "within half a century" on the basis of 1956 data. This is Hubbert peak theory.
- 1989 — Predicted peak by Colin Campbell ("Oil Price Leap in the Early Nineties," Noroil, December 1989, pages 35–38.)
- 2004 — OPEC estimates it will nearly double oil output by 2025 (Opec Oil Outlook to 2025 Table 4, Page 12)rashi
The history of perpetual motion machines is a long list of failed and sometimes fraudulent inventions of machines which produce useful energy "from nowhere" — that is, without requiring any energy input.
See also
- Cubic mile of oil
- Energy planning
- Energy policy
- Energy policy of the United States
- Environmental concerns with electricity generation
- List of emerging energy technologies
- List of environment topics
- Nuclear energy policy
- Proposed future transport
- Renewable energy development
- Relative cost of electricity generated by different sources
- World energy resources and consumption
- Mammoth resource partners
Notes
- ^ "Advanced Technologies & Energy Efficiency". U.S. DoE / U.S. EPA. Retrieved 2008-01-19.
{{cite web}}
: Cite has empty unknown parameters:|month=
and|coauthors=
(help) - ^ "Heat Island Group Home Page". Lawrence Berkeley National Laboratory. 2000-08-30. Retrieved 2008-01-19.
{{cite web}}
: Cite has empty unknown parameter:|coauthors=
(help) - ^ "Environmental impacts of coal power: air pollution". Union of Concerned Scientists. 08/18/05. Retrieved 2008-01-18.
{{cite web}}
: Check date values in:|date=
(help) - ^ http://www.pppl.gov/polImage.cfm?doc_Id=44&size_code=Doc
- ^ "Big Rig Building Boom". Rigzone.com. 2006-04-13. Retrieved 2008-01-18.
{{cite web}}
: Cite has empty unknown parameter:|coauthors=
(help) - ^ a b "Supply of Uranium". World Nuclear Association. 2007. Retrieved 2008-01-18.
{{cite web}}
: Unknown parameter|month=
ignored (help) - ^ "The Economics of Nuclear Power". World Nuclear Association. 2007. Retrieved 2008-01-18.
{{cite web}}
: Cite has empty unknown parameter:|coauthors=
(help); Unknown parameter|month=
ignored (help) - ^ Uranium Resources and Nuclear Energy
- ^ Jan Willem Storm van Leeuwen (2005-07-30). ""Nuclear Energy: the Energy Balance"" (PDF). Retrieved 2008-01-18.
{{cite web}}
: Unknown parameter|coauthors=
ignored (|author=
suggested) (help) - ^ "Thorium". World Nuclear Association. 2007. Retrieved 2008-01-18.
{{cite web}}
: Cite has empty unknown parameter:|coauthors=
(help); Unknown parameter|month=
ignored (help) - ^ a b Pallava Bagla (2005-08-19). "Rethinking Nuclear Power: India's Homegrown Thorium Reactor". Science (magazine). Retrieved 2008-04-12.
- ^ http://www.iaea.org/Publications/Magazines/Bulletin/Bull511/51104894344.html
- ^ http://www.neopanora.com/en/the-neop-systems.html
- ^ a b "Waste Management in the Nuclear Fuel Cycle". World Nuclear Association. 2007. Retrieved 2008-01-18.
{{cite web}}
: Cite has empty unknown parameter:|coauthors=
(help); Unknown parameter|month=
ignored (help) - ^ a b John McCarthy (2006). "Facts From Cohen and Others". Progress and its Sustainability. Stanford. Retrieved 2008-01-18.
- ^ "China's Fast Breeder Reactor (FBR) Program". Nuclear Threat Initiative. 02/06/2004. Retrieved 2008-01-18.
{{cite web}}
: Check date values in:|date=
(help); Cite has empty unknown parameter:|coauthors=
(help) - ^ Gary Crawley. ""Risks vs. Benefits in Energy Production"" (PDF). Science Foundation Ireland. Retrieved 2008-01-18.
{{cite web}}
: Cite has empty unknown parameter:|month=
(help) ] - ^ Brendan Nicholson (2006-06-05). ""Nuclear power 'cheaper, safer' than coal and gas"". The Age. Retrieved 2008-01-18.
{{cite web}}
: Italic or bold markup not allowed in:|publisher=
(help) - ^ Peter Schwartz (2005). ""Nuclear Now!"". Wired. Retrieved 2008-01-18.
{{cite web}}
: Italic or bold markup not allowed in:|publisher=
(help); Unknown parameter|coauthors=
ignored (|author=
suggested) (help); Unknown parameter|month=
ignored (help) - ^ "Accelerator-driven Nuclear Energy". World Nuclear Association. 2003. Retrieved 2008-01-18.
{{cite web}}
: Cite has empty unknown parameter:|coauthors=
(help); Unknown parameter|month=
ignored (help) - ^ "Energy Analysis of Power Systems". World Nuclear Association. 2006. Retrieved 2008-01-18.
{{cite web}}
: Cite has empty unknown parameter:|coauthors=
(help); Unknown parameter|month=
ignored (help) - ^ "Coming Clean; How Clean is Nuclear Energy?". 2000. Retrieved 2008-01-18.
{{cite web}}
: Cite has empty unknown parameter:|coauthors=
(help); Unknown parameter|month=
ignored (help) "World Information Service on Energy" 10-18 years for payback on nuclear energy, Jan Willem Storm van Leeuwen (2005-07-30). ""Nuclear Energy: the Energy Balance"" (PDF). Retrieved 2008-01-18.{{cite web}}
: Unknown parameter|coauthors=
ignored (|author=
suggested) (help) - ^ "Advanced Nuclear Power Reactors". Australian Uranium Association. 2008. Retrieved 2008-01-18.
{{cite web}}
: Cite has empty unknown parameter:|coauthors=
(help); Unknown parameter|month=
ignored (help) - ^ Spencer Reiss (2004). ""Let a Thousand Reactors Bloom"". Wired. Retrieved 2008-01-18.
{{cite web}}
: Italic or bold markup not allowed in:|publisher=
(help); Unknown parameter|month=
ignored (help) - ^ "Plans For New Reactors Worldwide". World Nuclear Association. 2007. Retrieved 2008-01-18.
{{cite web}}
: Cite has empty unknown parameter:|coauthors=
(help); Unknown parameter|month=
ignored (help) - ^ Charles D. Ferguson (2007). "Nuclear Energy: Balancing Benefits and Risks" (PDF). Council on Foreign Relations. Retrieved 2008-01-18.
{{cite web}}
: Unknown parameter|month=
ignored (help) - ^ The Greens | European Free Alliance in the European Parliament - – Nuclear energy
- ^ http://www.greens-efa.org/cms/topics/dokbin/206/206749.the_world_nuclear_industry_status_report@en.pdf
- ^ Carrie Coolidge (2006-01-05). ""The most dangerous jobs in America"". Forbes. Retrieved 2008-01-18.
{{cite web}}
: Italic or bold markup not allowed in:|publisher=
(help) - ^ "Life-Cycle Emissions Analysis". Nuclear Energy Institute. Retrieved 2008-01-18.
{{cite web}}
: Cite has empty unknown parameter:|month=
(help) - ^ Steve Green (2007-08-26). "Go Nuclear - Go Green - Life Cycle Emissions Comparable to Renewables". Retrieved 2008-01-18.
- ^ Plus radium (element 88). While actually a sub-actinide, it immediately precedes actinium (89) and follows a three-element gap of instability after polonium (84) where no nuclides have half-lives of at least four years (the longest-lived nuclide in the gap is radon-222 with a half life of less than four days). Radium's longest lived isotope, at 1,600 years, thus merits the element's inclusion here.
- ^ Specifically from thermal neutron fission of uranium-235, e.g. in a typical nuclear reactor.
- ^ Milsted, J.; Friedman, A. M.; Stevens, C. M. (1965). "The alpha half-life of berkelium-247; a new long-lived isomer of berkelium-248". Nuclear Physics. 71 (2): 299. Bibcode:1965NucPh..71..299M. doi:10.1016/0029-5582(65)90719-4.
"The isotopic analyses disclosed a species of mass 248 in constant abundance in three samples analysed over a period of about 10 months. This was ascribed to an isomer of Bk248 with a half-life greater than 9 [years]. No growth of Cf248 was detected, and a lower limit for the β− half-life can be set at about 104 [years]. No alpha activity attributable to the new isomer has been detected; the alpha half-life is probably greater than 300 [years]." - ^ This is the heaviest nuclide with a half-life of at least four years before the "sea of instability".
- ^ Excluding those "classically stable" nuclides with half-lives significantly in excess of 232Th; e.g., while 113mCd has a half-life of only fourteen years, that of 113Cd is eight quadrillion years.
- ^ "Geographical location and extent of radioactive contamination". Swiss Agency for Development and Cooperation.
- ^ Schwartz, J. 2004. "Emergency preparedness and response: compensating victims of a nuclear accident." Journal of Hazardous Materials, Volume 111, Issues 1–3, July, 89–96.
- ^ "TVA reactor shut down; cooling water from river too hot".
- ^ "What is ITER?". ITER International Fusion Energy Organization. Retrieved 2008-01-18.
{{cite web}}
: Cite has empty unknown parameters:|month=
and|coauthors=
(help) - ^ J. Ongena. "Energy for Future Centuries: Will fusion be an inexhaustible, safe and clean energy source?" (PDF). Retrieved 2008-01-18.
{{cite web}}
: Cite has empty unknown parameter:|month=
(help); Unknown parameter|coauthors=
ignored (|author=
suggested) (help) - ^ http://www.worldbookonline.com/digitallibraries/livinggreen/article?id=ar836725&st=biofuel
- ^ David Pimentel (2005). ""Ethanol Production Using Corn, Switchgrass, and Wood; Biodiesel Production Using Soybean and Sunflower"" (PDF). Natural Resources Research Vol. 14, No. 1. Retrieved 2008-01-18.
{{cite web}}
: Italic or bold markup not allowed in:|publisher=
(help); Unknown parameter|coauthors=
ignored (|author=
suggested) (help); Unknown parameter|month=
ignored (help) - ^ "Energy at the crossroads" (PDF). Retrieved 2008-01-01.
- ^ a b c Jeff Tester and Ron DiPippo (2007-06-07). "The Future of Geothermal Energy" (PDF). US Department of Energy - Energy Efficiency and Renewable Energy. Retrieved 2008-04-16.
- ^ http://www.worldbookonline.com/digitallibraries/livinggreen/article?id=ar836715&st=geothermal
- ^ Jefferson W. Tester; et al. (2006). ""The Future of Geothermal Energy"" (PDF). Idaho National Laboratory. Retrieved 2008-01-19.
{{cite web}}
: Cite has empty unknown parameters:|month=
and|coauthors=
(help); Explicit use of et al. in:|author=
(help) - ^ Hot rock firm looks at earthquake risk - Breaking News - Business - Breaking News
- ^ http://www.worldbookonline.com/digitallibraries/livinggreen/article?id=ar836804&st=hydroelectric
- ^ http://www.sciencemag.org/cgi/content/summary/323/5912/322
- ^ http://www.worldbookonline.com/digitallibraries/livinggreen/article?id=ar836713&st=solar+power
- ^ "Solar". Northwest Indian College. Retrieved 2008-01-19.
{{cite web}}
: Cite has empty unknown parameters:|month=
and|coauthors=
(help) - ^ "Technology White Paper on Solar Energy Potential on the U.S. Outer Continental Shelf" (PDF). U.S. Department of the Interior. 2006. Retrieved 2008-01-19.
{{cite web}}
: Cite has empty unknown parameter:|coauthors=
(help); Unknown parameter|month=
ignored (help) - ^ "Suntech Announces Analyst and Investor Day Highlights". Suntech Power. 2007-12-11. Retrieved 2008-01-19.
{{cite web}}
: Cite has empty unknown parameter:|coauthors=
(help) - ^ http://galenet.galegroup.com/servlet/SciRC?locID=cobb90289&bi=KE&bt=William+McDonough&c=2&t=2&ste=22&docNum=A182810130&st=b&tc=30&tf=0
- ^ Solar Revolution, by Travis Bradford
- ^ "Biofuel vs. Photovoltaics" EcoWorld
- ^ Renewable Resource Data Center — PV Correction Factors
- ^ "Home Power magazine". Retrieved 2008-01-19.
{{cite web}}
: Cite has empty unknown parameters:|month=
and|coauthors=
(help) - ^ http://galenet.galegroup.com/servlet/SciRC?locID=cobb90289&bi=KE&bt=tidal+power&c=1&t=1&ste=21&docNum=CV2644151381&st=b&tc=31&tf=0
- ^ http://galenet.galegroup.com/servlet/SciRC?locID=cobb90289&bi=KE&bt=wind+power&c=1&t=1&ste=21&docNum=CV2644151505&st=b&tc=31&tf=0
- ^ Wind Farm Foes, Backers Stage Watery Debate, Cape Cod Times (Waybacked).
- ^ Wind farms 'a threat to national security'
- ^ [1]
- ^ "Oak Ridge National Laboratory — Biomass, Solving the science is only part of the challenge". Retrieved 2008-01-06.
- ^ Kris Trexler. "1999 "Generation II" General Motors EV1: Kris Trexler's test drive impressions". King of the Road. Retrieved 2008-01-18.
{{cite web}}
: Cite has empty unknown parameter:|month=
(help) - ^ Zach Yates (2002). "The Efficiency of The Internal Combustion Engine". Retrieved 2008-01-18.
- ^ Idaho National Laboratory (2005) "Comparing Energy Costs per Mile for Electric and Gasoline-Fueled Vehicles" Advanced Vehicle Testing Activity report at avt.inel.gov (PDF), accessed 11 July 2006.
- ^ "Transportation Energy Data Book (link)". U.S. Dept. of Energy. Retrieved 2008-01-19.
{{cite web}}
: Cite has empty unknown parameters:|month=
and|coauthors=
(help) - ^ Hydrogen power of the future page 11
- ^ "Praxair Expands Hydrogen Pipeline Capacity". Praxair, Inc. 2002-05-02. Retrieved 2008-01-18.
{{cite web}}
: Cite has empty unknown parameter:|coauthors=
(help) - ^ "Survey of Energy Resources 2004 (link)". World Energy Council. Retrieved 2008-01-19.
{{cite web}}
: Cite has empty unknown parameters:|month=
and|coauthors=
(help) - ^ "Dispatchable Wind" (PDF). General Compression. 2007-11-26. Retrieved 2008-01-19.
{{cite web}}
: Cite has empty unknown parameter:|coauthors=
(help) - ^ Brittle Power: Energy Plan for National Security.Amory B. Lovins and L. Hunter Lovins (1982).
- ^ "The Fragility of Domestic Energy.” Amory B. Lovins and L. Hunter Lovins. Atlantic Monthly. November 1983.
- ^ “Our Electric Future.” Andrew Grove. The American. July/August 2008.
- ^ “An Electric Plan for Energy Resilience.” Andrew Grove and Robert Burgelman. McKinsey Quarterly. December 2008.
- ^ Resilience in Energy: Building Infrastructure Today for Tomorrow’s Automotive Fuel. Reform Institute. March 2009.
- ^ Mandil, C. (2008) “Our energy for the future”. S.A.P.I.EN.S. 1 (1)
- ^ Eating Fossil Fuels
- ^ Peak Oil: the threat to our food security retrieved 28 May 2009
References
- Serra, J. "Alternative Fuel Resource Development", Clean and Green Fuels Fund, (2006).
- Bilgen, S. and K. Kaygusuz, Renewable Energy for a Clean and Sustainable Future, Energy Sources 26, 1119 (2004).
- Energy analysis of Power Systems, UIC Nuclear Issues Briefing Paper 57 (2004).
- Silvestre, B. S., Dalcol, P. R. T. Geographical proximity and innovation: Evidences from the Campos Basin oil & gas industrial agglomeration — Brazil. Technovation (2009), doi:10.1016/j.technovation.2009.01.003
Relevant journals
- Energy Sources, Part A: Recovery, Utilization and Environmental Effects
- Energy Sources, Part B: Economics, Planning and Policy
- International Journal of Green Energy
External links
- Hidden Health and Environmental Costs Of Energy Production and Consumption In U.S.
- RECaBS REcalculator Interactive Renewable Energy Calculator — compare renewable energy to conventional energy sources