Space debris
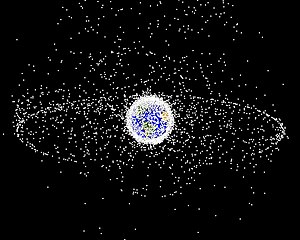
Part of a series on |
Pollution |
---|
![]() |
Space debris (also known as space junk, space pollution,[1] space waste, space trash, or space garbage) is defunct artificial objects in space—principally in Earth orbit—which no longer serve a useful function. These include derelict spacecraft—nonfunctional spacecraft and abandoned launch vehicle stages—mission-related debris, and particularly numerous in Earth orbit, fragmentation debris from the breakup of derelict rocket bodies and spacecraft. In addition to derelict man-made objects left in orbit, other examples of space debris include fragments from their disintegration, erosion and collisions or even paint flecks, solidified liquids expelled from spacecraft, and unburned particles from solid rocket motors. Space debris represents a risk to spacecraft.[2]
Space debris is typically a negative externality—it creates an external cost on others from the initial action to launch or use a spacecraft in near-Earth orbit—a cost that is typically not taken into account nor fully accounted for in the cost[3][4] by the launcher or payload owner.[5][1][6] The measurement, mitigation, and potential removal of debris are conducted by some participants in the space industry.[7]
As of January 2021[update], the US Space Surveillance Network reported 21,901 artificial objects in orbit above the Earth,[8] including 4,450 operational satellites.[9] However, these are just the objects large enough to be tracked. As of January 2019[update], more than 128 million pieces of debris smaller than 1 cm (0.4 in), about 900,000 pieces of debris 1–10 cm, and around 34,000 of pieces larger than 10 cm (3.9 in) were estimated to be in orbit around the Earth.[7] When the smallest objects of artificial space debris (paint flecks, solid rocket exhaust particles, etc.) are grouped with micrometeoroids, they are together sometimes referred to by space agencies as MMOD (Micrometeoroid and Orbital Debris). Collisions with debris have become a hazard to spacecraft; the smallest objects cause damage akin to sandblasting, especially to solar panels and optics like telescopes or star trackers that cannot easily be protected by a ballistic shield.[10]
Below 2,000 km (1,200 mi) Earth-altitude, pieces of debris are denser than meteoroids; most are dust from solid rocket motors, surface erosion debris like paint flakes, and frozen coolant from RORSAT (nuclear-powered satellites).[citation needed] For comparison, the International Space Station orbits in the 300–400 kilometres (190–250 mi) range, while the two most recent large debris events—the 2007 Chinese antisat weapon test and the 2009 satellite collision—occurred at 800 to 900 kilometres (500 to 560 mi) altitude.[11] The ISS has Whipple shielding to resist damage from small MMOD; however, known debris with a collision chance over 1/10,000 are avoided by maneuvering the station.
History
Space debris began to accumulate in Earth orbit immediately with the first launch of an artificial satellite Sputnik 1 into orbit in October 1957. But even before that, beside natural ejecta from Earth, humans might have produced ejecta that became space debris, as in the August 1957 Pascal B test.[12][13] After the launch of Sputnik, the North American Aerospace Defense Command (NORAD) began compiling a database (the Space Object Catalog) of all known rocket launches and objects reaching orbit: satellites, protective shields and upper-stages of launch vehicles. NASA later published[when?] modified versions of the database in two-line element set,[14] and beginning in the early 1980s the CelesTrak bulletin board system re-published them.[15]

The trackers (NORAD) who fed the database were aware of other objects in orbit, many of which were the result of in-orbit explosions.[16] Some were deliberately caused during the 1960s anti-satellite weapon (ASAT) testing, and others were the result of rocket stages blowing up in orbit as leftover propellant expanded and ruptured their tanks. To improve tracking, NORAD employee John Gabbard[clarification needed] kept a separate database. Studying the explosions, Gabbard developed[when?] a technique for predicting the orbital paths of their products, and Gabbard diagrams (or plots) are now widely used. These studies were used to improve the modeling of orbital evolution and decay.[17]
When the NORAD database became publicly available during the 1970s,[clarification needed] techniques developed for the asteroid-belt were applied to the study[by whom?] to the database of known artificial satellite Earth objects.[citation needed]
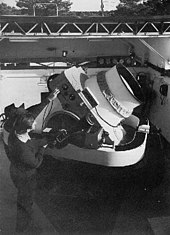
In addition to approaches to debris reduction where time and natural gravitational/atmospheric effects help to clear space debris, or a variety of technological approaches that have been proposed (with most not implemented) to reduce space debris, a number of scholars have observed that institutional factors—political, legal, economic and cultural "rules of the game"—are the greatest impediment to the cleanup of near-Earth space. By 2014, there was little commercial incentive to reduce space debris, since the cost of dealing with it is not assigned to the entity producing it, but rather falls on all users of the space environment, and rely on human society as a whole that benefits from space technologies and knowledge. A number of suggestions for improving institutions so as to increase the incentives to reduce space debris have been made. These include government mandates to create incentives, as well as companies coming to see economic benefit to reducing debris more aggressively than existing government standard practices.[18] In 1979 NASA founded the Orbital Debris Program to research mitigation measures for space debris in Earth orbit.[19][failed verification]
Debris growth
During the 1980s, NASA and other U.S. groups attempted to limit the growth of debris. One trial solution was implemented by McDonnell Douglas for the Delta launch vehicle,[when?] by having the booster move away from its payload and vent any propellant remaining in its tanks. This eliminated one source for pressure buildup in the tanks which had previously caused them to explode and create additional orbital debris.[20] Other countries were slower to adopt this measure and, due especially to a number of launches by the Soviet Union, the problem grew throughout the decade.[21]
A new battery of studies followed[when?] as NASA, NORAD and others attempted to better understand the orbital environment, with each adjusting the number of pieces of debris in the critical-mass zone upward. Although in 1981 (when Schefter's article was published) the number of objects was estimated at 5,000,[16] new detectors in the Ground-based Electro-Optical Deep Space Surveillance system found new objects. By the late 1990s, it was thought that most of the 28,000 launched objects had already decayed and about 8,500 remained in orbit.[22] By 2005 this was adjusted upward to 13,000 objects,[23] and a 2006 study increased the number to 19,000 as a result of an ASAT test and a satellite collision.[24] In 2011, NASA said that 22,000 objects were being tracked.[25]
A 2006 NASA model suggested that if no new launches took place the environment would retain the then-known population until about 2055, when it would increase on its own.[26][27] Richard Crowther of Britain's Defence Evaluation and Research Agency said in 2002 that he believed the cascade would begin about 2015.[28] The National Academy of Sciences, summarizing the professional view, noted widespread agreement that two bands of LEO space—900 to 1,000 km (620 mi) and 1,500 km (930 mi)—were already past critical density.[29]
In the 2009 European Air and Space Conference, University of Southampton researcher Hugh Lewis predicted that the threat from space debris would rise 50 percent in the next decade and quadruple in the next 50 years. As of 2009[update], more than 13,000 close calls were tracked weekly.[30]
A 2011 report by the U.S. National Research Council warned NASA that the amount of orbiting space debris was at a critical level. According to some computer models, the amount of space debris "has reached a tipping point, with enough currently in orbit to continually collide and create even more debris, raising the risk of spacecraft failures". The report called for international regulations limiting debris and research of disposal methods.[31]
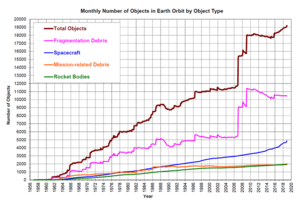
Debris history in particular years
- As of 2009[update], 19,000 debris over 5 cm (2 in) were tracked by United States Space Surveillance Network.[11]
- As of July 2013[update], estimates of more than 170 million debris smaller than 1 cm (0.4 in), about 670,000 debris 1–10 cm, and approximately 29,000 larger pieces of debris are in orbit.[32]
- As of July 2016[update], nearly 18,000 artificial objects are orbiting above Earth,[33] including 1,419 operational satellites.[34]
- As of October 2019[update], nearly 20,000 artificial objects in orbit above the Earth,[8] including 2,218 operational satellites.[9]
Characterization
Size
There are estimated to be over 128 million pieces of debris smaller than 1 cm (0.39 in) as of January 2019. There are approximately 900,000 pieces from 1 to 10 cm. The current count of large debris (defined as 10 cm across or larger[35]) is 34,000.[7] The technical measurement cutoff[clarification needed] is c. 3 mm (0.12 in).[36] As of 2020 there is 8000 metric tons of debris in orbit with no signs of slowing down.[37]
Low Earth orbit
In the orbits nearest to Earth—less than 2,000 km (1,200 mi) orbital altitude, referred to as low-Earth orbit (LEO)— there have traditionally been few "universal orbits" that keep a number of spacecraft in particular rings (in contrast to GEO, a single orbit that is widely used by over 500 satellites). This is beginning to change in 2019, and several companies have begun to deploy the early phases of satellite internet constellations, which will have many universal orbits in LEO with 30 to 50 satellites per orbital plane and altitude. Traditionally, the most populated LEO orbits have been a number of sun-synchronous satellites that keep a constant angle between the Sun and the orbital plane, making Earth observation easier with consistent sun angle and lighting. Sun-synchronous orbits are polar, meaning they cross over the polar regions. LEO satellites orbit in many planes, typically up to 15 times a day, causing frequent approaches between objects. The density of satellites—both active and derelict—is much higher in LEO.[38]
Orbits are affected by gravitational perturbations (which in LEO include unevenness of the Earth's gravitational field due to variations in the density of the planet), and collisions can occur from any direction. The average impact speed of collisions in Low Earth Orbit is 10 km/s with maximums reaching above 14 km/s due to Orbital eccentricity.[39] The 2009 satellite collision occurred at a closing speed of 11.7 km/s (26,000 mph),[40] creating over 2000 large debris fragments.[41] These debris cross many other orbits and increase debris collision risk.
It is theorized that a sufficiently large collision of spacecraft could potentially lead to a cascade effect, or even make some particular low Earth orbits effectively unusable for long term use by orbiting satellites, a phenomenon known as the Kessler syndrome.[42] The theoretical effect is projected to be a theoretical runaway chain reaction of collisions that could occur, exponentially increasing the number and density of space debris in low-Earth orbit, and has been hypothesized to ensue beyond some critical density.[43]
Crewed space missions are mostly at 400 km (250 mi) altitude and below, where air drag helps clear zones of fragments. The upper atmosphere is not a fixed density at any particular orbital altitude; it varies as a result of atmospheric tides and expands or contracts over longer time periods as a result of space weather.[44] These longer-term effects can increase drag at lower altitudes; the 1990s expansion was a factor in reduced debris density.[45] Another factor was fewer launches by Russia; the Soviet Union made most of their launches in the 1970s and 1980s.[46]: 7
Higher altitudes
At higher altitudes, where air drag is less significant, orbital decay takes longer. Slight atmospheric drag, lunar perturbations, Earth's gravity perturbations, solar wind and solar radiation pressure can gradually bring debris down to lower altitudes (where it decays), but at very high altitudes this may take millennia.[47] Although high-altitude orbits are less commonly used than LEO and the onset of the problem is slower, the numbers progress toward the critical threshold more quickly.[contradictory][page needed][48]
Many communications satellites are in geostationary orbits (GEO), clustering over specific targets and sharing the same orbital path. Although velocities are low between GEO objects, when a satellite becomes derelict (such as Telstar 401) it assumes a geosynchronous orbit; its orbital inclination increases about .8° and its speed increases about 160 km/h (99 mph) per year. Impact velocity peaks at about 1.5 km/s (0.93 mi/s). Orbital perturbations cause longitude drift of the inoperable spacecraft and precession of the orbital plane. Close approaches (within 50 meters) are estimated at one per year.[49] The collision debris pose less short-term risk than from an LEO collision, but the satellite would likely become inoperable. Large objects, such as solar-power satellites, are especially vulnerable to collisions.[50]
Although the ITU now requires proof a satellite can be moved out of its orbital slot at the end of its lifespan, studies suggest this is insufficient.[51] Since GEO orbit is too distant to accurately measure objects under 1 m (3 ft 3 in), the nature of the problem is not well known.[52] Satellites could be moved to empty spots in GEO, requiring less maneuvering and making it easier to predict future motion.[53] Satellites or boosters in other orbits, especially stranded in geostationary transfer orbit, are an additional concern due to their typically high crossing velocity.
Despite efforts to reduce risk, spacecraft collisions have occurred. The European Space Agency telecom satellite Olympus-1 was struck by a meteoroid on 11 August 1993 and eventually moved to a graveyard orbit.[54] On 29 March 2006, the Russian Express-AM11 communications satellite was struck by an unknown object and rendered inoperable;[55] its engineers had enough contact time with the satellite to send it into a graveyard orbit.
Sources
Dead spacecraft

In 1958, the United States launched Vanguard I into a medium Earth orbit (MEO). As of October 2009[update], it, and the upper stage of its launch rocket, were the oldest surviving artificial space objects still in orbit.[58][59] In a catalog of known launches until July 2009, the Union of Concerned Scientists listed 902 operational satellites[60] from a known population of 19,000 large objects and about 30,000 objects launched.[citation needed]
An example of additional derelict satellite debris is the remains of the 1970s/80s Soviet RORSAT naval surveillance satellite program. The satellites' BES-5 nuclear reactors were cooled with a coolant loop of sodium-potassium alloy, creating a potential problem when the satellite reached end of life. While many satellites were nominally boosted into medium-altitude graveyard orbits, not all were. Even satellites that had been properly moved to a higher orbit had an eight-percent probability of puncture and coolant release over a 50-year period. The coolant freezes into droplets of solid sodium-potassium alloy,[61] forming additional debris.[62]
In February 2015, the USAF Defense Meteorological Satellite Program Flight 13 (DMSP-F13) exploded on orbit, creating at least 149 debris objects, which were expected to remain in orbit for decades.[63]
Orbiting satellites have been deliberately destroyed. United States and USSR/Russia have conducted over 30 and 27 ASAT tests,[clarification needed] respectively, followed by 10 from China and one from India.[citation needed] The most recent ASATs were Chinese interception of FY-1C, trials of Russian PL-19 Nudol, American interception of USA-193 and Indian interception of unstated live satellite.[citation needed]
Lost equipment

Space debris includes a glove lost by astronaut Ed White on the first American space-walk (EVA), a camera lost by Michael Collins near Gemini 10, a thermal blanket lost during STS-88, garbage bags jettisoned by Soviet cosmonauts during Mir's 15-year life,[58] a wrench, and a toothbrush.[64] Sunita Williams of STS-116 lost a camera during an EVA. During an STS-120 EVA to reinforce a torn solar panel, a pair of pliers was lost, and in an STS-126 EVA, Heidemarie Stefanyshyn-Piper lost a briefcase-sized tool bag.[65]
Boosters

In characterizing the problem of space debris, it was learned that much debris was due to rocket upper stages (e.g. the Inertial Upper Stage) which end up in orbit, and break up due to decomposition of unvented unburned fuel.[66] However, a major known impact event involved an (intact) Ariane booster.[46]: 2 Although NASA and the United States Air Force now require upper-stage passivation, other launchers[vague] do not. Lower stages, like the Space Shuttle's solid rocket boosters or Apollo program's Saturn IB launch vehicles, do not reach orbit.[67]
On 11 March 2000 a Chinese Long March 4 CBERS-1 upper stage exploded in orbit, creating a debris cloud.[68][69] A Russian Briz-M booster stage exploded in orbit over South Australia on 19 February 2007. Launched on 28 February 2006 carrying an Arabsat-4A communications satellite, it malfunctioned before it could use up its propellant. Although the explosion was captured on film by astronomers, due to the orbit path the debris cloud has been difficult to measure with radar. By 21 February 2007, over 1,000 fragments were identified.[70][71] A 14 February 2007 breakup was recorded by Celestrak.[72] Eight breakups occurred in 2006, the most since 1993.[73] Another Briz-M broke up on 16 October 2012 after a failed 6 August Proton-M launch. The amount and size of the debris was unknown.[74] A Long March 7 rocket booster created a fireball visible from portions of Utah, Nevada, Colorado, Idaho and California on the evening of 27 July 2016; its disintegration was widely reported on social media.[75] In 2018–2019, three different Atlas V Centaur second stages have broken up.[76][77][78]

In December 2020, scientists confirmed that a previously detected near-Earth object, 2020 SO, was rocket booster space junk launched in 1966 orbiting Earth and the Sun.[79]
Weapons
A past debris source was the testing of anti-satellite weapons (ASATs) by the U.S. and Soviet Union during the 1960s and 1970s. North American Aerospace Defense Command (NORAD) files only contained data for Soviet tests, and debris from U.S. tests were only identified later.[80] By the time the debris problem was understood, widespread ASAT testing had ended; the U.S. Program 437 was shut down in 1975.[81]
The U.S. restarted their ASAT programs in the 1980s with the Vought ASM-135 ASAT. A 1985 test destroyed a 1-tonne (2,200 lb) satellite orbiting at 525 km (326 mi), creating thousands of debris larger than 1 cm (0.39 in). Due to the altitude, atmospheric drag decayed the orbit of most debris within a decade. A de facto moratorium followed the test.[82]

China's government was condemned for the military implications and the amount of debris from the 2007 anti-satellite missile test,[83] the largest single space debris incident in history (creating over 2,300 pieces golf-ball size or larger, over 35,000 1 cm (0.4 in) or larger, and one million pieces 1 mm (0.04 in) or larger). The target satellite orbited between 850 km (530 mi) and 882 km (548 mi), the portion of near-Earth space most densely populated with satellites.[84] Since atmospheric drag is low at that altitude, the debris is slow to return to Earth, and in June 2007 NASA's Terra environmental spacecraft maneuvered to avoid impact from the debris.[85] Dr. Brian Weeden, U.S. Air Force officer and Secure World Foundation staff member, noted that the 2007 Chinese satellite explosion created an orbital debris of more than 3,000 separate objects that then required tracking.[86] On 20 February 2008, the U.S. launched an SM-3 missile from the USS Lake Erie to destroy a defective U.S. spy satellite thought to be carrying 450 kg (1,000 lb) of toxic hydrazine propellant. The event occurred at about 250 km (155 mi), and the resulting debris has a perigee of 250 km (155 mi) or lower.[87] The missile was aimed to minimize the amount of debris, which (according to Pentagon Strategic Command chief Kevin Chilton) had decayed by early 2009.[88]
On 27 March 2019, Indian Prime Minister Narendra Modi announced that India shot down one of its own LEO satellites with a ground-based missile. He stated that the operation, part of Mission Shakti, would defend the country's interests in space. Afterwards, US Air Force Space Command announced they were tracking 270 new pieces of debris but expected the number to grow as data collection continues.[89]
On 15 November 2021 the Russian Defense Ministry destroyed Kosmos 1408[90] orbiting at around 450 km, creating "more than 1,500 pieces of trackable debris and hundreds of thousands of pieces of un-trackable debris" according to the US State Department.[91]
The vulnerability of satellites to debris and the possibility of attacking LEO satellites to create debris clouds has triggered speculation that it is possible for countries unable to make a precision attack.[clarification needed] An attack on a satellite of 10 t (22,000 lb) or more would heavily damage the LEO environment.[82]
Hazards

To spacecraft
Space junk can be a hazard to active satellites and spacecraft. It has been theorized that Earth orbit could even become impassable if the risk of collision grows too high.[92][failed verification]
However, since the risk to spacecraft increases with the time of exposure to high debris densities, it is more accurate to say that LEO would be rendered unusable by orbiting craft. The threat to craft passing through LEO to reach higher orbit would be much lower owing to the very short time span of the crossing.
Uncrewed spacecraft
Although spacecraft are typically protected by Whipple shields, solar panels, which are exposed to the Sun, wear from low-mass impacts. Even small impacts can produce a cloud of plasma which is an electrical risk to the panels.[93]
Satellites are believed to have been destroyed by micrometeorites and (small) orbital debris (MMOD). The earliest suspected loss was of Kosmos 1275, which disappeared on 24 July 1981 (a month after launch). Kosmos contained no volatile propellant, therefore, there appeared to be nothing internal to the satellite which could have caused the destructive explosion which took place. However, the case has not been proven and another hypothesis forwarded is that the battery exploded. Tracking showed it broke up, into 300 new objects.[94]
Many impacts have been confirmed since. For example, on 24 July 1996, the French microsatellite Cerise was hit by fragments of an Ariane-1 H-10 upper-stage booster which exploded in November 1986.[46]: 2 On 29 March 2006, the Russian Ekspress AM11 communications satellite was struck by an unknown object and rendered inoperable.[55] On 13 October 2009, Terra suffered a single battery cell failure anomaly and a battery heater control anomaly which were subsequently considered likely the result of an MMOD strike.[95] On 12 March 2010, Aura lost power from one-half of one of its 11 solar panels and this was also attributed to an MMOD strike.[96] On 22 May 2013, GOES 13 was hit by an MMOD which caused it to lose track of the stars that it used to maintain an operational attitude. It took nearly a month for the spacecraft to return to operation.[97]
The first major satellite collision occurred on 10 February 2009. The 950 kg (2,090 lb) derelict satellite Kosmos 2251 and the operational 560 kg (1,230 lb) Iridium 33 collided, 500 mi (800 km)[98] over northern Siberia. The relative speed of impact was about 11.7 km/s (7.3 mi/s), or about 42,120 km/h (26,170 mph).[99] Both satellites were destroyed, creating thousands of pieces of new smaller debris, with legal and political liability issues unresolved even years later.[100][101][102] On 22 January 2013, BLITS (a Russian laser-ranging satellite) was struck by debris suspected to be from the 2007 Chinese anti-satellite missile test, changing both its orbit and rotation rate.[103]
Satellites sometimes[clarification needed] perform Collision Avoidance Maneuvers and satellite operators may monitor space debris as part of maneuver planning. For example, in January 2017, the European Space Agency made the decision to alter orbit of one of its three[104] Swarm mission spacecraft, based on data from the US Joint Space Operations Center, to lower the risk of collision from Cosmos-375, a derelict Russian satellite.[105]
Crewed spacecraft
Crewed flights are naturally particularly sensitive to the hazards that could be presented by space debris conjunctions in the orbital path of the spacecraft. Examples of occasional avoidance maneuvers, or longer-term space debris wear, have occurred in Space Shuttle missions, the MIR space station, and the International Space Station.
Space Shuttle missions

From the early Space Shuttle missions, NASA used NORAD space monitoring capabilities to assess the Shuttle's orbital path for debris. In the 1980s, this used a large proportion of NORAD capacity.[20] The first collision-avoidance maneuver occurred during STS-48 in September 1991,[106] a seven-second thruster burn to avoid debris from the derelict satellite Kosmos 955.[107] Similar maneuvers were initiated on missions 53, 72 and 82.[106]
One of the earliest events to publicize the debris problem occurred on Space Shuttle Challenger's second flight, STS-7. A fleck of paint struck its front window, creating a pit over 1 mm (0.04 in) wide. On STS-59 in 1994, Endeavour's front window was pitted about half its depth. Minor debris impacts increased from 1998.[108]
Window chipping and minor damage to thermal protection system tiles (TPS) were already common by the 1990s. The Shuttle was later flown tail-first to take a greater proportion of the debris load on the engines and rear cargo bay, which are not used in orbit or during descent, and thus are less critical for post-launch operation. When flying attached to the ISS, the two connected spacecraft were flipped around so the better-armored station shielded the orbiter.[109]

A NASA 2005 study concluded that debris accounted for approximately half of the overall risk to the Shuttle.[109][110] Executive-level decision to proceed was required if catastrophic impact was likelier than 1 in 200. On a normal (low-orbit) mission to the ISS the risk was approximately 1 in 300, but the Hubble telescope repair mission was flown at the higher orbital altitude of 560 km (350 mi) where the risk was initially calculated at a 1-in-185 (due in part to the 2009 satellite collision). A re-analysis with better debris numbers reduced the estimated risk to 1 in 221, and the mission went ahead.[111]
Debris incidents continued on later Shuttle missions. During STS-115 in 2006 a fragment of circuit board bored a small hole through the radiator panels in Atlantis's cargo bay.[112] On STS-118 in 2007 debris blew a bullet-like hole through Endeavour's radiator panel.[113]
Mir
Impact wear was notable on Mir, the Soviet space station, since it remained in space for long periods with its original solar module panels.[114][115]

International Space Station
The ISS also uses Whipple shielding to protect its interior from minor debris.[116] However, exterior portions (notably its solar panels) cannot be protected easily. In 1989, the ISS panels were predicted to degrade approximately 0.23% in four years due to the "sandblasting" effect of impacts with small orbital debris.[117] An avoidance maneuver is typically performed for the ISS if "there is a greater than one-in-10,000 chance of a debris strike".[118] As of January 2014[update], there have been sixteen maneuvers in the fifteen years the ISS had been in orbit.[118] By 2019, over 1,400 meteoroid and orbital debris (MMOD) impacts had been recorded on the ISS.[119]
As another method to reduce the risk to humans on board, ISS operational management asked the crew to shelter in the Soyuz on three occasions due to late debris-proximity warnings. In addition to the sixteen thruster firings and three Soyuz-capsule shelter orders, one attempted maneuver was not completed due to not having the several days' warning necessary to upload the maneuver timeline to the station's computer.[118][120][121] A March 2009 event involved debris believed to be a 10 cm (3.9 in) piece of the Kosmos 1275 satellite.[122] In 2013, the ISS operations management did not make a maneuver to avoid any debris, after making a record four debris maneuvers the previous year.[118]
Kessler syndrome
The Kessler syndrome,[123][124] proposed by NASA scientist Donald J. Kessler in 1978, is a theoretical scenario in which the density of objects in low Earth orbit (LEO) is high enough that collisions between objects could cause a cascade effect where each collision generates space debris that increases the likelihood of further collisions.[125] He further theorized that one implication if this were to occur is that the distribution of debris in orbit could render space activities and the use of satellites in specific orbital ranges economically impractical for many generations.[125]
The growth in the number of objects as a result of the late-1990s studies sparked debate in the space community on the nature of the problem and the earlier dire warnings. According to Kessler's 1991 derivation and 2001 updates,[126] the LEO environment in the 1,000 km (620 mi) altitude range should be cascading. However, only one major satellite collision incident has occurred: the 2009 satellite collision between Iridium 33 and Cosmos 2251. The lack of obvious short-term cascading has led to speculation that the original estimates overstated the problem.[127][full citation needed] According to Kessler in 2010 however, a cascade may not be obvious until it is well advanced, which might take years.[128]
On Earth

Although most debris burns up in the atmosphere, larger debris objects can reach the ground intact. According to NASA, an average of one cataloged piece of debris has fallen back to Earth each day for the past 50 years. Despite their size, there has been no significant property damage from the debris.[129] Burning up in the atmosphere may also contribute to atmospheric pollution.[130]
Notable examples of space junk falling to Earth and impacting human life include:
- 1969: five sailors on a Japanese ship were injured when space debris from what was believed to be a Soviet spacecraft struck the deck of their boat.[131]
- 1978: the Soviet reconnaissance satellite Kosmos 954 reentered the atmosphere over northwest Canada and scattered radioactive debris over northern Canada, some landing in the Great Slave Lake.[131]
- 1979: portions of Skylab came down over Australia, and several pieces landed in the area around the Shire of Esperance, which fined NASA $400 for littering.[131]
- 1987: a 7-foot strip of metal from the Soviet Kosmos 1890 rocket landed between two homes in Lakeport, California, causing no damage.
- 1991: Salyut 7 underwent an uncontrolled reentry on 7 February over the city of Capitán Bermúdez in Argentina.[132]
- 1997: an Oklahoma woman, Lottie Williams, was hit, without injury in the shoulder by a 10 cm × 13 cm (3.9 in × 5.1 in) piece of blackened, woven metallic material confirmed as part of the propellant tank of a Delta II rocket which launched a U.S. Air Force satellite the year before.[133][134]
- 2001: a Star 48 Payload Assist Module (PAM-D) rocket upper stage re-entered the atmosphere after a "catastrophic orbital decay",[135] crashing in the Saudi Arabian desert. It was identified as the upper-stage rocket for NAVSTAR 32, a GPS satellite launched in 1993.[citation needed]
- 2002: 6-year-old boy Wu Jie became the first person to be injured by direct impact from space debris. He suffered a fractured toe and a swelling on his forehead after a block of aluminum, 80 centimeters by 50 centimeters and weighing 10 kilograms, from the outer shell of the Resource Second satellite struck him as he sat beneath a persimmon tree in the Shaanxi province of China.[136]
- 2003: Columbia disaster, large parts of the spacecraft reached the ground and entire equipment systems remained intact.[137] More than 83,000 pieces, along with the remains of the six astronauts, were recovered in an area from three to ten miles around Hemphill in Sabine County, Texas.[138] More pieces were found in a line from west Texas to east Louisiana, with the westernmost piece found in Littlefield, TX and the easternmost found southwest of Mora, Louisiana.[139] Debris was found in Texas, Arkansas and Louisiana. In a rare case of property damage, a foot-long metal bracket smashed through the roof of a dentist office.[140] NASA warned the public to avoid contact with the debris because of the possible presence of hazardous chemicals.[141] 15 years after the failure, people were still sending in pieces with the most recent, as of February 2018, found in the spring of 2017.[142]
- 2007: airborne debris from a Russian spy satellite was seen by the pilot of a LAN Airlines Airbus A340 carrying 270 passengers whilst flying over the Pacific Ocean between Santiago and Auckland. The debris was reported within 9.3 kilometres (5 nmi) of the aircraft.[143]
- 2020: The empty core stage of a Long March-5B rocket made an uncontrolled re-entry - the largest object to do so since the Soviet Union's 39-ton Salyut 7 space station in 1991 – over Africa and the Atlantic Ocean and a 12-meter-long pipe originating from the rocket crashed into the village of Mahounou in Côte d'Ivoire.[144]
- 2021: a Falcon 9 second stage made an uncontrolled re-entry over Washington state on March 25, producing a widely seen "light show".[145] A composite-overwrapped pressure vessel survived the re-entry and landed on a farm field.[146]
Tracking and measurement
Tracking from the ground
Radar and optical detectors such as lidar are the main tools for tracking space debris. Although objects under 10 cm (4 in) have reduced orbital stability, debris as small as 1 cm can be tracked,[147][148] however determining orbits to allow re-acquisition is difficult. Most debris remain unobserved. The NASA Orbital Debris Observatory tracked space debris with a 3 m (10 ft) liquid mirror transit telescope.[149] FM Radio waves can detect debris, after reflecting off them onto a receiver.[150] Optical tracking may be a useful early-warning system on spacecraft.[151]
The U.S. Strategic Command keeps a catalog of known orbital objects, using ground-based radar and telescopes, and a space-based telescope (originally to distinguish from hostile missiles). The 2009 edition listed about 19,000 objects.[152] Other data come from the ESA Space Debris Telescope, TIRA,[153] the Goldstone, Haystack,[154] and EISCAT radars and the Cobra Dane phased array radar,[155] to be used in debris-environment models like the ESA Meteoroid and Space Debris Terrestrial Environment Reference (MASTER).
Measurement in space

Returned space hardware is a valuable source of information on the directional distribution and composition of the (sub-millimetre) debris flux. The LDEF satellite deployed by mission STS-41-C Challenger and retrieved by STS-32 Columbia spent 68 months in orbit to gather debris data. The EURECA satellite, deployed by STS-46 Atlantis in 1992 and retrieved by STS-57 Endeavour in 1993, was also used for debris study.[156]
The solar arrays of Hubble were returned by missions STS-61 Endeavour and STS-109 Columbia, and the impact craters studied by the ESA to validate its models. Materials returned from Mir were also studied, notably the Mir Environmental Effects Payload (which also tested materials intended for the ISS[157]).[158][159]
Gabbard diagrams
A debris cloud resulting from a single event is studied with scatter plots known as Gabbard diagrams, where the perigee and apogee of fragments are plotted with respect to their orbital period. Gabbard diagrams of the early debris cloud prior to the effects of perturbations, if the data were available, are reconstructed. They often include data on newly observed, as yet uncatalogued fragments. Gabbard diagrams can provide important insights into the features of the fragmentation, the direction and point of impact.[17][160]
Dealing with debris
An average of about one tracked object per day has been dropping out of orbit for the past 50 years,[161] averaging almost three objects per day at solar maximum (due to the heating and expansion of the Earth's atmosphere), but one about every three days at solar minimum, usually five and a half years later.[161] In addition to natural atmospheric effects, corporations, academics and government agencies have proposed plans and technology to deal with space debris, but as of November 2014[update], most of these are theoretical, and there is no extant business plan for debris reduction.[18]
A number of scholars have also observed that institutional factors—political, legal, economic, and cultural "rules of the game"—are the greatest impediment to the cleanup of near-Earth space. There is little commercial incentive to act, since costs are not assigned to polluters, though a number of technological solutions have been suggested.[18] However, effects to date are limited. In the US, governmental bodies have been accused of backsliding on previous commitments to limit debris growth, "let alone tackling the more complex issues of removing orbital debris."[162] The different methods for removal of space debris have been evaluated by the Space Generation Advisory Council, including French astrophysicist Fatoumata Kébé.[163]
Growth mitigation


As of the 2010s, several technical approaches to the mitigation of the growth of space debris are typically undertaken, yet no comprehensive legal regime or cost assignment structure is in place to reduce space debris in the way that terrestrial pollution has reduced since the mid-20th century.
To avoid excessive creation of artificial space debris, many—but not all—satellites launched to above-low-Earth-orbit are launched initially into elliptical orbits with perigees inside Earth's atmosphere so the orbit will quickly decay and the satellites then will destroy themselves upon reentry into the atmosphere. Other methods are used for spacecraft in higher orbits. These include passivation of the spacecraft at the end of its useful life; as well as the use of upper stages that can reignite to decelerate the stage to intentionally deorbit it, often on the first or second orbit following payload release; satellites that can, if they remain healthy for years, deorbit themselves from the lower orbits around Earth. Other satellites (such as many CubeSats) in low orbits below approximately 400 km (250 mi) orbital altitude depend on the energy-absorbing effects of the upper atmosphere to reliably deorbit a spacecraft within weeks or months.
Increasingly, spent upper stages in higher orbits—orbits for which low-delta-v deorbit is not possible, or not planned for—and architectures that support satellite passivation, at end of life are passivated at end of life. This removes any internal energy contained in the vehicle at the end of its mission or useful life. While this does not remove the debris of the now derelict rocket stage or satellite itself, it does substantially reduce the likelihood of the spacecraft destructing and creating many smaller pieces of space debris, a phenomenon that was common in many of the early generations of US and Soviet[62] spacecraft.
Upper stage passivation (e.g. of Delta boosters[20]) by releasing residual propellants reduces debris from orbital explosions; however even as late as 2011, not all upper stages implement this practice.[165] SpaceX used the term "propulsive passivation" for the final maneuver of their six-hour demonstration mission (STP-2) of the Falcon 9 second stage for the US Air Force in 2019, but did not define what all that term encompassed.[166]
With a "one-up, one-down" launch-license policy for Earth orbits, launchers would rendezvous with, capture and de-orbit a derelict satellite from approximately the same orbital plane.[167] Another possibility is the robotic refueling of satellites. Experiments have been flown by NASA,[168] and SpaceX is developing large-scale on-orbit propellant transfer technology.[169]
Another approach to debris mitigation is to explicitly design the mission architecture to always leave the rocket second-stage in an elliptical geocentric orbit with a low-perigee, thus ensuring rapid orbital decay and avoiding long-term orbital debris from spent rocket bodies. Such missions will often complete the payload placement in a final orbit by the use of low-thrust electric propulsion or with the use of a small kick stage to circularize the orbit. The kick stage itself may be designed with the excess-propellant capability to be able to self-deorbit.[170]
Self-removal
Although the ITU requires geostationary satellites to move to a graveyard orbit at the end of their lives, the selected orbital areas do not sufficiently protect GEO lanes from debris.[51] Rocket stages (or satellites) with enough propellant may make a direct, controlled de-orbit, or if this would require too much propellant, a satellite may be brought to an orbit where atmospheric drag would cause it to eventually de-orbit. This was done with the French Spot-1 satellite, reducing its atmospheric re-entry time from a projected 200 years to about 15 by lowering its altitude from 830 km (516 mi) to about 550 km (342 mi).[171][172]
The Iridium constellation – 95 communication satellites launched during the five-year period between 1997 and 2002 – provides a set of data points on the limits of self-removal. The satellite operator – Iridium Communications – remained operational over the two-decade life of the satellites (albeit with a company name change through a corporate bankruptcy during the period) and, by December 2019, had "completed disposal of the last of its 65 working legacy satellites."[173] However, this process left 30 satellites with a combined mass of (20,400 kg (45,000 lb), or nearly a third of the mass of this constellation) in LEO orbits at approximately 700 km (430 mi) altitude, where self-decay is quite slow. Of these satellites, 29 simply failed during their time in orbit and were thus unable to self-deorbit, while one – Iridium 33 – was involved in the 2009 satellite collision with the derelict Russian military satellite Kosmos-2251.[173] No contingency plan was laid for the removal of satellites that were unable to remove themselves. In 2019, the Iridium CEO, Matt Desch, said that Iridium would be willing to pay an active-debris-removal company to deorbit its remaining first-generation satellites if it were possible for an unrealistically low cost, say "US$10,000 per deorbit, but [he] acknowledged that price would likely be far below what a debris-removal company could realistically offer. 'You know at what point [it’s] a no-brainer, but [I] expect the cost is really in the millions or tens of millions, at which price I know it doesn’t make sense.'"[173]
Passive methods of increasing the orbital decay rate of spacecraft debris have been proposed. Instead of rockets, an electrodynamic tether could be attached to a spacecraft at launch; at the end of its lifetime, the tether would be rolled out to slow the spacecraft.[174] Other proposals include a booster stage with a sail-like attachment[175] and a large, thin, inflatable balloon envelope.[176]
External removal
A variety of approaches have been proposed, studied, or had ground subsystems built to use other spacecraft to remove existing space debris. A consensus of speakers at a meeting in Brussels in October 2012, organized by the Secure World Foundation (a U.S. think tank) and the French International Relations Institute,[177] reported that removal of the largest debris would be required to prevent the risk to spacecraft becoming unacceptable in the foreseeable future (without any addition to the inventory of dead spacecraft in LEO). To date in 2019, removal costs and legal questions about ownership and the authority to remove defunct satellites have stymied national or international action. Current space law retains ownership of all satellites with their original operators, even debris or spacecraft which are defunct or threaten active missions.
Multiple companies made plans in the late 2010s to conduct external removal on their satellites in mid-LEO orbits. For example, OneWeb planned to utilize onboard self-removal as "plan A" for satellite deorbiting at the end of life, but if a satellite were unable to remove itself within one year of end of life, OneWeb would implement "plan B" and dispatch a reusable (multi-transport mission) space tug to attach to the satellite at an already built-in capture target via a grappling fixture, to be towed to a lower orbit and released for re-entry.[178][179]
Remotely controlled vehicles
A well-studied solution uses a remotely controlled vehicle to rendezvous with, capture, and return debris to a central station.[180] One such system is Space Infrastructure Servicing, a commercially developed refueling depot and service spacecraft for communications satellites in geosynchronous orbit originally scheduled for a 2015 launch.[181] The SIS would be able to "push dead satellites into graveyard orbits."[182] The Advanced Common Evolved Stage family of upper stages is being designed with a high leftover-propellant margin (for derelict capture and de-orbit) and in-space refueling capability for the high delta-v required to de-orbit heavy objects from geosynchronous orbit.[167] A tug-like satellite to drag debris to a safe altitude for it to burn up in the atmosphere has been researched.[183] When debris is identified the satellite creates a difference in potential between the debris and itself, then using its thrusters to move itself and the debris to a safer orbit.
A variation of this approach is for the remotely controlled vehicle to rendezvous with debris, capture it temporarily to attach a smaller de-orbit satellite and drag the debris with a tether to the desired location. The "mothership" would then tow the debris-smallsat combination for atmospheric entry or move it to a graveyard orbit. One such system is the proposed Busek ORbital DEbris Remover (ORDER), which would carry over 40 SUL (satellite on umbilical line) de-orbit satellites and propellant sufficient for their removal.[18]
On 7 January 2010 Star, Inc. reported that it received a contract from the Space and Naval Warfare Systems Command for a feasibility study of the ElectroDynamic Debris Eliminator (EDDE) propellantless spacecraft for space-debris removal.[184] In February 2012 the Swiss Space Center at École Polytechnique Fédérale de Lausanne announced the Clean Space One project, a nanosatellite demonstration project for matching orbit with a defunct Swiss nanosatellite, capturing it and de-orbiting together.[185] The mission has seen several evolutions to reach a pac-man inspired capture model.[186] In 2013, Space Sweeper with Sling-Sat (4S), a grappling satellite which captures and ejects debris was studied.[187][needs update] In 2022, a Chinese satellite, SJ-21, grabbed an unused satellite and "threw" it into an orbit with a lower risk for it to collide.[188][189]
In December 2019, the European Space Agency awarded the first contract to clean up space debris. The €120 million mission dubbed ClearSpace-1 (a spinoff from the EPFL project) is slated to launch in 2025. It aims to remove a 100 kg VEga Secondary Payload Adapter (Vespa)[190] left by Vega flight VV02 in an 800 km (500 mi) orbit in 2013. A "chaser" will grab the junk with four robotic arms and drag it down to Earth's atmosphere where both will burn up.[191]
Laser methods
The laser broom uses a ground-based laser to ablate the front of the debris, producing a rocket-like thrust that slows the object. With continued application, the debris would fall enough to be influenced by atmospheric drag.[192][193] During the late 1990s, the U.S. Air Force's Project Orion was a laser-broom design.[194] Although a test-bed device was scheduled to launch on a Space Shuttle in 2003, international agreements banning powerful laser testing in orbit limited its use to measurements.[195] The 2003 Space Shuttle Columbia disaster postponed the project and according to Nicholas Johnson, chief scientist and program manager for NASA's Orbital Debris Program Office, "There are lots of little gotchas in the Orion final report. There's a reason why it's been sitting on the shelf for more than a decade."[196]
The momentum of the laser-beam photons could directly impart a thrust on the debris sufficient to move small debris into new orbits out of the way of working satellites. NASA research in 2011 indicates that firing a laser beam at a piece of space junk could impart an impulse of 1 mm (0.039 in) per second, and keeping the laser on the debris for a few hours per day could alter its course by 200 m (660 ft) per day.[197] One drawback is the potential for material degradation; the energy may break up the debris, adding to the problem.[citation needed] A similar proposal places the laser on a satellite in Sun-synchronous orbit, using a pulsed beam to push satellites into lower orbits to accelerate their reentry.[18] A proposal to replace the laser with an Ion Beam Shepherd has been made,[198] and other proposals use a foamy ball of aerogel or a spray of water,[199] inflatable balloons,[200] electrodynamic tethers,[201] electroadhesion,[202] and dedicated anti-satellite weapons.[203]
Nets
On 28 February 2014, Japan's Japan Aerospace Exploration Agency (JAXA) launched a test "space net" satellite. The launch was an operational test only.[204] In December 2016 the country sent a space junk collector via Kounotori 6 to the ISS by which JAXA scientists experiment to pull junk out of orbit using a tether.[205][206] The system failed to extend a 700-meter tether from a space station resupply vehicle that was returning to Earth.[207][208] On 6 February the mission was declared a failure and leading researcher Koichi Inoue told reporters that they "believe the tether did not get released".[209]
Since 2012, the European Space Agency has been working on the design of a mission to remove large space debris from orbit. The mission, e.Deorbit, is scheduled for launch during 2023 with an objective to remove debris heavier than 4,000 kilograms (8,800 lb) from LEO.[210] Several capture techniques are being studied, including a net, a harpoon and a combination robot arm and clamping mechanism.[211]
Harpoon
The RemoveDEBRIS mission plan is to test the efficacy of several ADR technologies on mock targets in low Earth orbit. In order to complete its planned experiments the platform is equipped with a net, a harpoon, a laser ranging instrument, a dragsail, and two CubeSats (miniature research satellites).[212] The mission was launched on 2 April 2018.
National and international regulation
There is no international treaty minimizing space debris. However, the United Nations Committee on the Peaceful Uses of Outer Space (COPUOS) published voluntary guidelines in 2007,[213] using a variety of earlier national regulatory attempts at developing standards for debris mitigation. As of 2008, the committee was discussing international "rules of the road" to prevent collisions between satellites.[214] By 2013, a number of national legal regimes existed,[215][216][217] typically instantiated in the launch licenses that are required for a launch in all spacefaring nations.[218]
The U.S. issued a set of standard practices for civilian (NASA) and military (DoD and USAF) orbital-debris mitigation in 2001.[219][220][216] The standard envisioned disposal for final mission orbits in one of three ways: 1) atmospheric reentry where even with "conservative projections for solar activity, atmospheric drag will limit the lifetime to no longer than 25 years after completion of mission;" 2) maneuver to a "storage orbit:" move the spacecraft to one of four very broad parking orbit ranges (2,000–19,700 km (1,200–12,200 mi), 20,700–35,300 km (12,900–21,900 mi), above 36,100 km (22,400 mi), or out of Earth orbit completely and into any heliocentric orbit; 3) "Direct retrieval: Retrieve the structure and remove it from orbit as soon as practicable after completion of mission."[215] The standard articulated in option 1, which is the standard applicable to most satellites and derelict upper stages launched, has come to be known as the "25-year rule."[221] The US updated the ODMSP in December 2019, but made no change to the 25-year rule even though "[m]any in the space community believe that the timeframe should be less than 25 years."[219] There is no consensus however on what any new timeframe might be.[219]
In 2002, the European Space Agency (ESA) worked with an international group to promulgate a similar set of standards, also with a "25-year rule" applying to most Earth-orbit satellites and upper stages. Space agencies in Europe began to develop technical guidelines in the mid-1990s, and ASI, UKSA, CNES, DLR and ESA signed a "European Code of Conduct" in 2006,[217] which was a predecessor standard to the ISO international standard work that would begin the following year. In 2008, ESA further developed "its own "Requirements on Space Debris Mitigation for Agency Projects" which "came into force on 1 April 2008."[217]
Germany and France have posted bonds to safeguard the property from debris damage.[clarification needed][222] The "direct retrieval" option (option no. 3 in the US "standard practices" above) has rarely been done by any spacefaring nation (exception, USAF X-37) or commercial actor since the earliest days of spaceflight due to the cost and complexity of achieving direct retrieval, but the ESA has scheduled a 2025 demonstration mission (Clearspace-1) to do this with a single small 100 kg (220 lb) derelict upper stage at a projected cost of €120 million not including the launch costs.[191]
By 2006, the Indian Space Research Organization (ISRO) had developed a number of technical means of debris mitigation (upper stage passivation, propellant reserves for movement to graveyard orbits, etc.) for ISRO launch vehicles and satellites, and was actively contributing to inter-agency debris coordination and the efforts of the UN COPUOS committee.[223]
In 2007, the ISO began preparing an international standard for space-debris mitigation.[224] By 2010, ISO had published "a comprehensive set of space system engineering standards aimed at mitigating space debris. [with primary requirements] defined in the top-level standard, ISO 24113." By 2017, the standards were nearly complete. However, these standards are not binding on any party by ISO or any international jurisdiction. They are simply available for use in any of a variety of voluntary ways. They "can be adopted voluntarily by a spacecraft manufacturer or operator, or brought into effect through a commercial contract between a customer and supplier, or used as the basis for establishing a set of national regulations on space debris mitigation."[221]
The voluntary ISO standard also adopted the "25-year rule" for the "LEO protected region" below 2,000 km (1,200 mi) altitude that has been previously (and still is, as of 2019[update]) used by the US, ESA, and UN mitigation standards, and identifies it as "an upper limit for the amount of time that a space system shall remain in orbit after its mission is completed. Ideally, the time to deorbit should be as short as possible (i.e., much shorter than 25 years)".[221]
Holger Krag of the European Space Agency states that as of 2017 there is no binding international regulatory framework with no progress occurring at the respective UN body in Vienna.[92]
Barriers
With the rapid development of the computer and digitalization industries, more countries and companies have engaged in space activities since the turn of the 20th century. The tragedy of the commons is an economic theory referring to a situation where maximizing self-interest through using a shared resource can finally lead to the resource degradation shared by all.[225] Based on the theory, individuals’ rational action in space will finally lead to an irrational collective result: orbits are crowded with debris. As a common-pool resource, the Earth’s orbits, especially LEO and GEO that accommodate most satellites, are nonexcludable and rivalry.[226] To address the tragedy and ensure space sustainability, many technical approaches have been developed. And in terms of governance mechanisms, the top-down centralized one is less suitable to tackle the complex debris problem due to the increasing number of space actors.[227] Instead, much evidence has proved that polycentric form of governance developed by Elinor Ostrom can work in space.[228]
In the process of promoting the polycentric network, there are some existing barriers needed to be dealt with.
Incomplete data of space debris
As orbital debris is a global problem affecting both spacefaring and non-spacefaring nations, it is necessary to be handled in a worldwide context.[225] Because of the complexity and dynamics of object movements like spacecrafts, debris, meteorites, etc., many countries and regions including the United States, Europe, Russia and China have developed their space situational awareness (SSA) to avoid potential threats in space or plan actions in advance.[229] To a certain extent, SSA plays a role in tracking space debris. In order to build a powerful SSA system, there are two prerequisites: international cooperation and exchange of information and data.[229] However, limitations still exist in spite of the substantially improving data quality over the past decades. Some space powers are not willing to share the information that they have collected, and those, such as the U.S., that have shared the data keep parts of it secret.[230] Instead of joining together in a coordinated way, a great deal of SSA programs and national databases run parallel to each other with some overlaps, hindering the formation of a collaborative monitoring system.[230]
Some private actors are also trying to establish SSA systems. For example, the Space Data Association (SDA) formed in 2009 is a non-governmental entity. It currently consists of 21 global satellite operators and 4 executive members: Eutelsat, Inmarsat, Intelsat and SES. SDA is a non-profit platform, aiming to avoid radio interference and space collisions through pooling data from operators independently.[229] Researchers suggest that it is essential to establish an international center for exchanging information on space debris because SSA networks do not completely equal debris tracking systems — the former ones focus more on active and threatening objects in space.[231] And in terms of debris populations and defunct satellites, not very much operators have provided data.[231]
In a polycentric governance network, a resource that cannot be holistically monitored is less possible to be well managed.[230] Both insufficient transnational cooperation and information sharing bring resistance to addressing the debris problem. There is still a long way to go before building a global network that covers complete data and has strong interconnection and interoperability.
Insufficient participation of private actors
With the commercialization of satellites and space, the private sector is getting more interested in space activities. For example, SpaceX is planning to create a network of around 12,000 small satellites that can transmit high-speed internet to any place in the world.[232] The proportion of commercial spacecrafts has increased from 4.6% in the 1980s to 55.6% in the 2010s.[233] Despite the high participation rate of commercial entities, UN COPUOS once deliberately excluded them from having a voice in discussions unless being formally invited by a member state.[227] Ostrom said that the involvement of all relevant stakeholders in the rule-design and implementation process is one of the critical elements of successful governance.[234] The exclusion of private actors largely reduces the effectiveness of the committee’s role in making collective-choice arrangements that reflect the interests of all space users.[227]
The limited engagement of private actors slows down the process of addressing space debris to some degree.[235] Ties existing between dissimilar stakeholders in the governance network offer access to diverse resources.[236] Different competence among stakeholders can help allocate the tasks more reasonably. In that case, the expertise and experience of private operators are critical to help the world achieve space sustainability.[235] The complementary strengths of different stakeholders enable the governance network to be more adaptable to changes and reach common goals more effectively.[236] In recent years, many private actors have seen commercial opportunities of eliminating space debris. It is estimated that by 2022 the global market for debris monitoring and removal will generate a revenue of around $2.9 billion.[237] For example, Astroscale has contracted with European and Japanese space agencies to develop the capacity of removing orbital debris.[238] Despite that, they are still in small quantity compared to the number of those who have placed satellites in space.
Fortunately, the current space exploration is not completely driven by competition, and there still exists a chance for dialogues and cooperation among all stakeholders in both developed and developing countries, to reach an agreement on tackling space debris and assure an equitable and orderly exploration.[239] Besides private actors, network governance does not necessarily exclude the states from playing a role. Instead, the different functions of states might promote the governance process.[240] To improve the polycentric governance network of space debris, researchers suggest: encourage data-sharing among different national and organizational databases at the political level; develop shared standards for data collection systems to improve interoperability; enhance the participation of private actors through involving them in national and international discussions.[230]
At other celestial bodies
The issue of space debris has been raised as a mitigation challenge for missions around the Moon with the danger of increasing space debris around it.[241][242]
In popular culture
Until the End of the World (1991) is a French sci-fi drama set under the backdrop of an out-of-control Indian nuclear satellite, predicted to re-enter the atmosphere, threatening vast populated areas of the Earth.[243]
In the Planetes, a Japanese hard science fiction manga (1999-2004) and anime (2003–2004), the story revolves around the crew of a space debris collection craft in the year 2075.
Gravity, a 2013 survival film directed by Alfonso Cuaron, is about a disaster on a space mission caused by Kessler syndrome.[244]
In season 1 of Love, Death & Robots (2019), episode 11, "Helping Hand", revolves around an astronaut being struck by a screw from space debris which knocks her off a satellite in orbit.[245]
See also
- Category:Derelict satellites
- Interplanetary contamination
- Liability Convention
- List of large reentering space debris
- List of space debris producing events
- Long Duration Exposure Facility
- Near-Earth object
- Orbital Debris Co-ordination Working Group
- Project West Ford
- Satellite warfare
- Solar Maximum Mission
- Spacecraft cemetery
- Space domain awareness
- Space sustainability
References
Notes
- ^ a b "'We've left junk everywhere': why space pollution could be humanity's next big problem". The Guardian. 26 March 2016. Archived from the original on 8 November 2019. Retrieved 28 December 2019.
- ^ "Guide to Space Debris". spaceacademy.net.au. Archived from the original on 26 August 2018. Retrieved 13 August 2018.
- ^ Coase, Ronald (October 1960). "The Problem of Social Cost" (PDF). Journal of Law and Economics (PDF). 3. The University of Chicago Press: 1–44. doi:10.1086/466560. JSTOR 724810. S2CID 222331226. Archived (PDF) from the original on 17 June 2012. Retrieved 13 December 2019.
- ^ Heyne, Paul; Boettke, Peter J.; Prychitko, David L. (2014). The Economic Way of Thinking (13th ed.). Pearson. pp. 227–28. ISBN 978-0-13-299129-2.
- ^ Muñoz-Patchen, Chelsea (2019). "Regulating the Space Commons: Treating Space Debris as Abandoned Property in Violation of the Outer Space Treaty". Chicago Journal of International Law. University of Chicago Law School. Archived from the original on 13 December 2019. Retrieved 13 December 2019.
- ^ "Preventing space pollution". 30 March 2018.
- ^ a b c "Space debris by the numbers" Archived 6 March 2019 at the Wayback Machine ESA, January 2019. Retrieved 5 March 2019
- ^ a b "Satellite Box Score" (PDF). Orbital Debris Quarterly News. Vol. 23, no. 4. NASA. November 2019. p. 10. Archived (PDF) from the original on 24 December 2019. Retrieved 24 December 2019.
- ^ a b "UCS Satellite Database". Nuclear Weapons & Global Security. Union of Concerned Scientists. 16 December 2019. Archived from the original on 20 December 2019. Retrieved 24 December 2019.
- ^ "The Threat of Orbital Debris and Protecting NASA Space Assets from Satellite Collisions" (PDF). Space Reference. 2009. Archived (PDF) from the original on 23 December 2015. Retrieved 18 December 2012.
- ^ a b The Threat of Orbital Debris and Protecting NASA Space Assets from Satellite Collisions (PDF), Space Reference, 2009, archived (PDF) from the original on 23 December 2015, retrieved 18 December 2012
- ^ Harrington, Rebecca (5 February 2016). "The fastest object ever launched was a manhole cover — here's the story from the guy who shot it into space". Tech Insider - www.businessinsider.com Business Insider. Retrieved 11 June 2021.
- ^ Thomson, Iain (16 July 2015). "SCIENCEDid speeding American manhole cover beat Sputnik into space? Top boffin speaks to El Reg - How a nuke blast lid may have beaten Soviets by months". www.theregister.com. Retrieved 11 June 2021.
- ^ Felix Hoots, Paul Schumacher Jr.; Glover, Robert (2004). "History of Analytical Orbit Modeling in the U.S. Space Surveillance System". Journal of Guidance Control and Dynamics. 27 (2): 174–185. Bibcode:2004JGCD...27..174H. doi:10.2514/1.9161.
- ^ T.S. Kelso, CelesTrak BBS: Historical Archives Archived 17 July 2012 at archive.today, 2-line elements dating to 1980
- ^ a b Schefter, p. 48.
- ^ a b David Portree and Joseph Loftus. "Orbital Debris: A Chronology" Archived 1 September 2000 at the Wayback Machine, NASA, 1999, p. 13.
- ^ a b c d e Foust, Jeff (15 November 2014). "Companies Have Technologies, but Not Business Plans, for Orbital Debris Cleanup". Space News. Archived from the original on 6 December 2014. Retrieved 28 December 2019.
- ^ "NASA Orbital Debris Program". Archived from the original on 3 November 2016. Retrieved 10 October 2016.
- ^ a b c Schefter, p. 50.
- ^ See charts, Hoffman p. 7.
- ^ See chart, Hoffman p. 4.
- ^ In the time between the writing of Klinkrad (2006) Chapter 1 (earlier) and the Prolog (later) of Space Debris, Klinkrad changed the number from 8,500 to 13,000 – compare pp. 6 and ix.
- ^ Michael Hoffman, "It's getting crowded up there." Space News, 3 April 2009.
- ^ "Space Junk Threat Will Grow for Astronauts and Satellites" Archived 9 April 2011 at the Wayback Machine, Fox News, 6 April 2011.
- ^ Stefan Lovgren, "Space Junk Cleanup Needed, NASA Experts Warn." Archived 7 September 2009 at the Wayback Machine National Geographic News, 19 January 2006.
- ^ J.-C Liou and N. L. Johnson, "Risks in Space from Orbiting Debris" Archived 1 June 2008 at the Wayback Machine, Science, Volume 311 Number 5759 (20 January 2006), pp. 340–41
- ^ Antony Milne, Sky Static: The Space Debris Crisis, Greenwood Publishing Group, 2002, ISBN 0-275-97749-8, p. 86.
- ^ Technical, p. 7.
- ^ Paul Marks, "Space debris threat to future launches" Archived 26 April 2015 at the Wayback Machine, 27 October 2009.
- ^ Space junk at tipping point, says report Archived 21 December 2017 at the Wayback Machine, BBC News, 2 September 2011
- ^ "How many space debris objects are currently in orbit?" Archived 18 May 2016 at the Wayback Machine ESA, July 2013. Retrieved 6 February 2016
- ^ "Satellite Box ScoreS" (PDF). Orbital Debris Quarterly News. Vol. 20, no. 3. NASA. July 2016. p. 8. Archived (PDF) from the original on 11 October 2016. Retrieved 10 October 2016.
- ^ "UCS Satellite Database". Nuclear Weapons & Global Security. Union of Concerned Scientists. 11 August 2016. Archived from the original on 3 June 2010. Retrieved 10 October 2016.
- ^ Technical report on space debris (PDF). United Nations. 2009. ISBN 978-92-1-100813-5. Archived from the original (PDF) on 24 July 2009.
{{cite book}}
:|website=
ignored (help) - ^ "Orbital Debris FAQ: How much orbital debris is currently in Earth orbit?" Archived 25 August 2009 at the Wayback Machine NASA, March 2012. Retrieved 31 January 2016
- ^ Liou, J.-C. 2020. “Risks from Orbital Debris and Space Situational Awareness.” IAA Conference on Space Situational Awareness, January 14, 2020. Washington, D.C. https://ntrs.nasa.gov/archive/nasa/casi.ntrs.nasa.gov/20200000450.pdf
- ^ Matt Ford, "Orbiting space junk heightens risk of satellite catastrophes." Archived 5 April 2012 at the Wayback Machine Ars Technica, 27 February 2009.
- ^ Wertz, James; Everett, David; Puschell, Jeffrey (2011). Space mission engineering : the new SMAD. Hawthorne, CA: Microcosm Press. p. 139. ISBN 978-1881883159.
- ^ "What are hypervelocity impacts?" Archived 9 August 2011 at the Wayback Machine ESA, 19 February 2009.
- ^ "Orbital Debris Quarterly News, July 2011" (PDF). NASA Orbital Debris Program Office. Archived from the original (PDF) on 20 October 2011. Retrieved 1 January 2012.
- ^ Donald J. Kessler (8 March 2009). "The Kessler Syndrome". Archived from the original on 27 May 2010. Retrieved 22 September 2009.
- ^ Lisa Grossman, "NASA Considers Shooting Space Junk with Lasers" Archived 22 February 2014 at the Wayback Machine, wired, 15 March 2011.
- ^ Nwankwo, Victor U. J; Denig, William; Chakrabarti, Sandip K.; Ajakaiye, Muyiwa P.; Fatokun1, Johnson; Akanni, Adeniyi W.; Raulin, Jean-Pierre; Correia, Emilia; Enoh, John E. (15 September 2020). "Atmospheric drag effects on modelled LEO satellites during the July 2000 Bastille Day event in contrast to an interval of geomagnetically quiet conditions". Annales Geophysicae. doi:10.5194/angeo-2020-33-rc2.
{{cite journal}}
: CS1 maint: numeric names: authors list (link) - ^ Kessler 1991, p. 65.
- ^ a b c Heiner Klinkrad (2006). Space Debris: Models and Risk Analysis"]. Springer-Praxis. ISBN 3-540-25448-X. Archived from the original on 12 May 2011. Retrieved 20 December 2009.
- ^ Kessler 1991, p. 268.
- ^ Schildknecht, T.; Musci, R.; Flury, W.; Kuusela, J.; De Leon, J.; Dominguez Palmero, L. De Fatima (2005). "Optical observation of space debris in high-altitude orbits". Proceedings of the 4th European Conference on Space Debris (ESA SP-587). 18–20 April 2005. 587: 113. Bibcode:2005ESASP.587..113S.
- ^ "Colocation Strategy and Collision Avoidance for the Geostationary Satellites at 19 Degrees West." CNES Symposium on Space Dynamics, 6–10 November 1989.
- ^ van der Ha, J. C.; Hechler, M. (1981). "The Collision Probability of Geostationary Satellites". 32nd International Astronautical Congress. 1981: 23. Bibcode:1981rome.iafcR....V.
- ^ a b Anselmo, L.; Pardini, C. (2000). "Collision Risk Mitigation in Geostationary Orbit". Space Debris. 2 (2): 67–82. Bibcode:2000SpDeb...2...67A. doi:10.1023/A:1021255523174. S2CID 118902351.
- ^ Orbital Debris, p. 86.
- ^ Orbital Debris, p. 152.
- ^ "The Olympus failure" ESA press release, 26 August 1993. Archived 11 September 2007 at the Wayback Machine
- ^ a b "Notification for Express-AM11 satellite users in connection with the spacecraft failure" Russian Satellite Communications Company, 19 April 2006.
- ^ "Vanguard 1". Archived from the original on 15 August 2019. Retrieved 4 October 2019.
- ^ "Vanguard I celebrates 50 years in space". Eurekalert.org. Archived from the original on 5 June 2013. Retrieved 4 October 2013.
- ^ a b Julian Smith, "Space Junk" [dead link] USA Weekend, 26 August 2007.
- ^ "Vanguard 50 years". Archived from the original on 5 June 2013. Retrieved 4 October 2013.
- ^ "UCS Satellite Database" Archived 3 June 2010 at the Wayback Machine Union of Concerned Scientists, 16 July 2009.
- ^ C. Wiedemann et al, "Size distribution of NaK droplets for MASTER-2009", Proceedings of the 5th European Conference on Space Debris, 30 March–2 April 2009, (ESA SP-672, July 2009).
- ^ a b A. Rossi et al, "Effects of the RORSAT NaK Drops on the Long Term Evolution of the Space Debris Population", University of Pisa, 1997.
- ^ Gruss, Mike (6 May 2015). "DMSP-F13 Debris To Stay on Orbit for Decades". Space News. Retrieved 7 May 2015.
- ^ Tufte, Edward R (2013) [1990], Envisioning Information, Cheshire, CT: Graphics Press, p. 48, ISBN 978-0-9613921-1-6
- ^ See image here.
- ^ Loftus, Joseph P. (1989). Orbital Debris from Upper-stage Breakup. AIAA. p. 227. ISBN 978-1-60086-376-9.
- ^ Some return to Earth intact, see this list Archived 28 October 2009 at the Wayback Machine for examples.
- ^ Phillip Anz-Meador and Mark Matney, "An assessment of the NASA explosion fragmentation model to 1 mm characteristic sizes Archived 17 October 2015 at the Wayback Machine" Advances in Space Research, Volume 34 Issue 5 (2004), pp. 987–992.
- ^ "Debris from explosion of Chinese rocket detected by University of Chicago satellite instrument", University of Chicago press release, 10 August 2000.
- ^ "Rocket Explosion" Archived 30 January 2008 at the Wayback Machine, Spaceweather.com, 22 February 2007. Retrieved 21 February 2007.
- ^ Ker Than, "Rocket Explodes Over Australia, Showers Space with Debris" Archived 24 July 2008 at the Wayback Machine Space.com, 21 February 2007. Retrieved 21 February 2007.
- ^ "Recent Debris Events" Archived 20 March 2007 at the Wayback Machine celestrak.com, 16 March 2007. Retrieved 14 July 2001.
- ^ Jeff Hecht, "Spate of rocket breakups creates new space junk" Archived 14 August 2014 at the Wayback Machine, NewScientist, 17 January 2007. Retrieved 16 March 2007.
- ^ "Proton Launch Failure 2012 Aug 6". Zarya. 21 October 2012. Archived from the original on 10 October 2012. Retrieved 21 October 2012.
- ^ Mike, Wall (28 July 2016). "Amazing Fireball Over Western US Caused by Chinese Space Junk". space.com. Archived from the original on 29 July 2016. Retrieved 28 July 2016.
- ^ "Major fragmentation of Atlas 5 Centaur upper stage 2014‐055B (SSN #40209)" (PDF). Archived from the original (PDF) on 19 August 2019. Retrieved 22 May 2019.
- ^ "Rocket break up provides rare chance to test debris formation". Archived from the original on 16 May 2019. Retrieved 22 May 2019.
- ^ "Confirmed breakup of Atlas 5 Centaur R/B (2018-079B, #43652) on April 6, 2019". Archived from the original on 2 May 2019. Retrieved 22 May 2019.
- ^ Talbert, Tricia (2 December 2020). "New Data Confirm 2020 SO to be 1960s Upper Centaur Rocket Booster". NASA. Retrieved 16 January 2021.
- ^ Note that the list Schefter was presented only identified USSR ASAT tests.
- ^ Clayton Chun, "Shooting Down a Star: America's Thor Program 437, Nuclear ASAT, and Copycat Killers", Maxwell AFB Base, AL: Air University Press, 1999. ISBN 1-58566-071-X.
- ^ a b David Wright, "Debris in Brief: Space Debris from Anti-Satellite Weapons" Archived 9 September 2009 at the Wayback Machine Union of Concerned Scientists, December 2007.
- ^ Leonard David, "China's Anti-Satellite Test: Worrisome Debris Cloud Circles Earth" Archived 6 January 2011 at the Wayback Machine space.com, 2 February 2007.
- ^ "Fengyun 1C – Orbit Data" Archived 18 March 2012 at the Wayback Machine Heavens Above.
- ^ Brian Burger, "NASA's Terra Satellite Moved to Avoid Chinese ASAT Debris" Archived 13 May 2008 at the Wayback Machine, space.com. Retrieved 6 July 2007.
- ^ "Space Week: Is Space Junk Cluttering Up The Final Frontier". NPR.org. Retrieved 2 December 2020.
- ^ "Pentagon: Missile Scored Direct Hit on Satellite." Archived 6 January 2018 at the Wayback Machine, npr.org, 21 February 2008.
- ^ Jim Wolf, "US satellite shootdown debris said gone from space" Archived 14 July 2009 at the Wayback Machine, Reuters, 27 February 2009.
- ^ Chavez, Nicole; Pokharel, Sugam (28 March 2019). "India conducts successful anti-satellite missile operation, Prime Minister says". CNN. Archived from the original on 28 March 2019. Retrieved 28 March 2019.
- ^ Berger, Eric (16 November 2021). "Russia acknowledges anti-satellite test, but says it's no big deal". Ars Technica. Retrieved 16 November 2021.
- ^ Berger, Eric (15 November 2021). "Russia may have just shot down its own satellite, creating a huge debris cloud [Updated]". Ars Technica. Retrieved 16 November 2021.
- ^ a b Seidler, Christoph (22 April 2017). "Problem Weltraumschrott: Die kosmische Müllkippe - SPIEGEL ONLINE - Wissenschaft". Der Spiegel. SPIEGEL ONLINE. Archived from the original on 23 April 2017. Retrieved 22 April 2017.
- ^ Akahoshi, Y.; et al. (2008). "Influence of space debris impact on solar array under power generation". International Journal of Impact Engineering. 35 (12): 1678–1682. doi:10.1016/j.ijimpeng.2008.07.048.
- ^ "The Space Review: Regulating the void: In-orbit collisions and space debris". www.thespacereview.com. Retrieved 23 November 2020.
- ^ Kelley, Angelita (17 August 2014). Butler, James J; Xiong, Xiaoxiong (Jack); Gu, Xingfa (eds.). "Terra mission operations: Launch to the present (and beyond)" (PDF). Earth Observing Systems Xix. 9218: 92180M. Bibcode:2014SPIE.9218E..0MK. doi:10.1117/12.2061253. hdl:2060/20160008917. S2CID 32269938. Archived (PDF) from the original on 2 December 2017. Retrieved 5 April 2018.
- ^ Fisher, Dominic (13 June 2017). "Mission Status at Aura Science Team MOWG Meeting" (Document).
{{cite document}}
: Cite document requires|publisher=
(help); Unknown parameter|access-date=
ignored (help); Unknown parameter|url=
ignored (help) - ^ "Archived copy". www.ssd.noaa.gov. Archived from the original on 7 June 2013. Retrieved 12 January 2022.
{{cite web}}
: CS1 maint: archived copy as title (link) - ^ Becky Iannotta and Tariq Malik, "U.S. Satellite Destroyed in Space Collision" Archived 10 May 2012 at WebCite, space.com, 11 February 2009
- ^ Paul Marks, "Satellite collision 'more powerful than China's ASAT test" Archived 15 February 2009 at the Wayback Machine, New Scientist, 13 February 2009.
- ^ Iridium 33 and Cosmos 2251, Three Years Later Archived 17 May 2019 at the Wayback Machine, Michael Listner, Space Safety Magazine, 10 February 2012, accessed 14 December 2019.
- ^ "2 big satellites collide 500 miles over Siberia." yahoo.com, 11 February 2009. Retrieved 11 February 2009.
- ^ Becky Iannotta, "U.S. Satellite Destroyed in Space Collision" Archived 10 May 2012 at WebCite, space.com, 11 February 2009. Retrieved 11 February 2009.
- ^ Leonard David (8 March 2013). "Russian Satellite Hit by Debris from Chinese Anti-Satellite Test". space.com. Archived from the original on 11 March 2013. Retrieved 10 March 2013.
- ^ "Swarm Satellite Trio Launched To Study Earth's Magnetic Field - SpaceNews.com". SpaceNews.com. 22 November 2013. Retrieved 25 January 2017.
- ^ "Space junk could take out a European satellite this week". CNET. Archived from the original on 25 January 2017. Retrieved 25 January 2017.
- ^ a b Rob Matson, "Satellite Encounters" Archived 6 October 2010 at the Wayback Machine Visual Satellite Observer's Home Page.
- ^ "STS-48 Space Shuttle Mission Report" Archived 5 January 2016 at the Wayback Machine, NASA, NASA-CR-193060, October 1991.
- ^ Christiansen, E. L.; Hyden, J. L.; Bernhard, R. P. (2004). "Space Shuttle debris and meteoroid impacts". Advances in Space Research. 34 (5): 1097–1103. Bibcode:2004AdSpR..34.1097C. doi:10.1016/j.asr.2003.12.008.
- ^ a b Kelly, John. "Debris is Shuttle's Biggest Threat" Archived 23 May 2009 at the Wayback Machine, space.com, 5 March 2005.
- ^ "Debris Danger". Aviation Week & Space Technology, Volume 169 Number 10 (15 September 2008), p. 18.
- ^ William Harwood, "Improved odds ease NASA's concerns about space debris" Archived 19 June 2009 at the Wayback Machine, CBS News, 16 April 2009.
- ^ D. Lear et al, "Investigation of Shuttle Radiator Micro-Meteoroid & Orbital Debris Damage" Archived 9 March 2012 at the Wayback Machine, Proceedings of the 50th Structures, Structural Dynamics, and Materials Conference, 4–7 May 2009, AIAA 2009–2361.
- ^ D. Lear, et al, "STS-118 Radiator Impact Damage" Archived 13 August 2011 at the Wayback Machine, NASA
- ^ Smirnov, V.M.; et al. (2000). "Study of Micrometeoroid and Orbital Debris Effects on the Solar Panelson 'MIR'". Space Debris. 2 (1): 1–7. doi:10.1023/A:1015607813420. S2CID 118628073.
- ^ "Orbital Debris FAQ: How did the Mir space station fare during its 15-year stay in Earth orbit?" Archived 25 August 2009 at the Wayback Machine, NASA, July 2009.
- ^ K Thoma et al, "New Protection Concepts for Meteoroid / Debris Shields" Archived 9 April 2008 at the Wayback Machine, Proceedings of the 4th European Conference on Space Debris (ESA SP-587), 18–20 April 2005, p. 445.
- ^ Henry Nahra, "Effect of Micrometeoroid and Space Debris Impacts on the Space Station Freedom Solar Array Surfaces" Archived 6 June 2011 at the Wayback Machine. Presented at the 1989 Spring Meeting of the Materials Research Society, 24–29 April 1989, NASA TR-102287.
- ^ a b c d de Selding, Peter B. (16 January 2014). "Space Station Required No Evasive Maneuvers in 2013 Despite Growing Debris Threat". Space News. Retrieved 17 January 2014.
- ^ Hyde, James L.; Christiansen, Eric L.; Lear, Dana M. (9–12 December 2019). Observations of MMOD Impact Damage to the ISS (PDF). First International Orbital Debris Conference. USRA. Retrieved 8 October 2021.
- ^ "Junk alert for space station crew" Archived 18 March 2009 at the Wayback Machine, BBC News, 12 March 2009.
- ^ "International Space Station in debris scare" Archived 31 October 2018 at the Wayback Machine, BBC News, 28 June 2011.
- ^ Haines, Lester. "ISS spared space junk avoidance manoeuvre" Archived 10 August 2017 at the Wayback Machine, The Register, 17 March 2009.
- ^ "Scientist: Space weapons pose debris threat – CNN". Articles.CNN.com. 3 May 2002. Archived from the original on 30 September 2012. Retrieved 17 March 2011.
- ^ "The Danger of Space Junk – 98.07". TheAtlantic.com. July 1998. Retrieved 17 March 2011.
- ^ a b Donald J. Kessler and Burton G. Cour-Palais (1978). "Collision Frequency of Artificial Satellites: The Creation of a Debris Belt". Journal of Geophysical Research. 83 (A6): 2637–2646. Bibcode:1978JGR....83.2637K. doi:10.1029/JA083iA06p02637.
- ^ Kessler 2001
- ^ Technical
- ^ Jan Stupl et al, "Debris-debris collision avoidance using medium power ground-based lasers", 2010 Beijing Orbital Debris Mitigation Workshop, 18–19 October 2010, see graph p. 4 Archived 9 March 2012 at the Wayback Machine
- ^ Brown, M. (2012). Orbital Debris Frequently Asked Questions. Retrieved from https://orbitaldebris.jsc.nasa.gov/faq.html Archived 28 March 2019 at the Wayback Machine.
- ^ Brown, Mike (27 May 2021). "SpaceX Starlink: how it could kickstart an 'uncontrolled experiment'". Inverse. Retrieved 28 May 2021.
- ^ a b c U.S. Congress, Office of Technology Assessment, "Orbiting Debris: A Space Environmental Problem" Archived 4 March 2016 at the Wayback Machine, Background Paper, OTA-BP-ISC-72, U.S. Government Printing Office, September 1990, p. 3
- ^ McQuiston, John T. (7 February 1991). "Salyut 7, Soviet Station in Space, Falls to Earth After 9-Year Orbit". New York Times. Retrieved 2 January 2022.
- ^ "Today in Science History" Archived 13 January 2006 at the Wayback Machine todayinsci.com. Retrieved 8 March 2006.
- ^ Tony Long, "Jan. 22, 1997: Heads Up, Lottie! It's Space Junk!" Archived 2 January 2018 at the Wayback Machine, wired, 22 January 2009. Retrieved 27 March 2016
- ^ "PAM-D Debris Falls in Saudi Arabia" Archived 16 July 2009 at the Wayback Machine, The Orbital Debris Quarterly News, Volume 6 Issue 2 (April 2001).
- ^ Maley, Paul. "SPACE DEBRIS: 1960-1980". Retrieved 7 May 2021.
- ^ "Debris Photos" Archived 25 December 2017 at the Wayback Machine NASA.
- ^ Wallach, Dan (1 February 2016). "Columbia shuttle tragedy marks Sabine County town". Archived from the original on 9 May 2018. Retrieved 8 May 2018.
- ^ "Columbia Accident Investigation Report, Volume II Appendix D.10" (PDF). Archived from the original (PDF) on 17 October 2011. Retrieved 10 May 2018.
- ^ "Shuttle Debris Falls on East Texas, Louisiana". 1 February 2003. Archived from the original on 9 May 2018. Retrieved 8 May 2018.
- ^ "Debris Warning" Archived 17 October 2015 at the Wayback Machine NASA.
- ^ "Debris from fallen space shuttle Columbia has new mission 15 years after tragedy". 1 February 2018. Archived from the original on 6 February 2018. Retrieved 8 May 2018.
- ^ Jano Gibson, "Jet's flaming space junk scare" Archived 6 December 2011 at the Wayback Machine, The Sydney Morning Herald, 28 March 2007.
- ^ O'Callaghan, Jonathan (12 May 2020). "Chinese Rocket Debris May Have Fallen On Villages In The Ivory Coast After An Uncontrolled Re-Entry". Retrieved 7 May 2021.
- ^ "A Falcon 9 rocket making an uncontrolled re-entry looked like an alien armada". Ars Technica. 26 March 2021. Retrieved 5 April 2021.
- ^ "Piece of SpaceX rocket debris lands at Washington state farm". Phys.org. 3 April 2021. Retrieved 5 April 2021.
- ^ D. Mehrholz et al;"Detecting, Tracking and Imaging Space Debris" Archived 10 July 2009 at the Wayback Machine, ESA bulletin 109, February 2002.
- ^ Ben Greene, "Laser Tracking of Space Debris" Archived 18 March 2009 at the Wayback Machine, Electro Optic Systems Pty
- ^ "Orbital debris: Optical Measurements" Archived 15 February 2012 at the Wayback Machine, NASA Orbital Debris Program Office
- ^ Pantaleo, Rick. "Australian Scientists Track Space Junk by Listening to FM Radio". web. Archived from the original on 4 December 2013. Retrieved 3 December 2013.
- ^ Englert, Christoph R.; Bays, J. Timothy; Marr, Kenneth D.; Brown, Charles M.; Nicholas, Andrew C.; Finne, Theodore T. (2014). "Optical orbital debris spotter". Acta Astronautica. 104 (1): 99–105. Bibcode:2014AcAau.104...99E. doi:10.1016/j.actaastro.2014.07.031.
- ^ Grant Stokes et al, "The Space-Based Visible Program", MIT Lincoln Laboratory. Retrieved 8 March 2006.
- ^ H. Klinkrad. "Monitoring Space – Efforts Made by European Countries" (PDF). fas.org. Archived from the original (PDF) on 4 March 2016. Retrieved 8 March 2006., fas.org. |date-accessed=8 March 2006.
- ^ "MIT Haystack Observatory" Archived 29 November 2004 at the Wayback Machine haystack.mit.edu. Retrieved 8 March 2006.
- ^ "AN/FPS-108 COBRA DANE." Archived 5 February 2016 at the Wayback Machine fas.org. Retrieved 8 March 2006.
- ^ Darius Nikanpour, "Space Debris Mitigation Technologies" Archived 19 October 2012 at the Wayback Machine, Proceedings of the Space Debris Congress, 7–9 May 2009.
- ^ "STS-76 Mir Environmental Effects Payload (MEEP)". NASA. March 1996. Archived from the original on 18 April 2011. Retrieved 8 March 2011.
- ^ MEEP Archived 5 June 2011 at the Wayback Machine, NASA, 4 April 2002. Retrieved 8 July 2011
- ^ "STS-76 Mir Environmental Effects Payload (MEEP)" Archived 29 June 2011 at the Wayback Machine, NASA, March 1996. Retrieved 8 March 2011.
- ^ David Whitlock, "History of On-Orbit Satellite Fragmentations" Archived 3 January 2006 at the Wayback Machine, NASA JSC, 2004
- ^ a b Johnson, Nicholas (5 December 2011). "Space debris issues". audio file, @0:05:50-0:07:40. The Space Show. Archived from the original on 27 January 2012. Retrieved 8 December 2011.
- ^ Foust, Jeff (24 November 2014). "Industry Worries Government 'Backsliding' on Orbital Debris". Space News. Archived from the original on 8 December 2014. Retrieved 8 December 2014.
Despite growing concern about the threat posed by orbital debris, and language in U.S. national space policy directing government agencies to study debris cleanup technologies, many in the space community worry that the government is not doing enough to implement that policy.
- ^ Northfield, Rebecca (20 June 2018). "Women of Nasa: past, present and future". eandt.theiet.org. Archived from the original on 21 January 2019. Retrieved 20 January 2019.
- ^ "USA Space Debris Environment, Operations, and Policy Updates" (PDF). NASA. UNOOSA. Retrieved 3 March 2021.
- ^ Johnson, Nicholas (5 December 2011). "Space debris issues". audio file, @1:03:05-1:06:20. The Space Show. Archived from the original on 27 January 2012. Retrieved 8 December 2011.
- ^ Eric Ralph (19 April 2019). "SpaceX's Falcon Heavy flies a complex mission for the Air Force in launch video". Teslarati. Archived from the original on 25 August 2019. Retrieved 14 December 2019.
- ^ a b Frank Zegler and Bernard Kutter, "Evolving to a Depot-Based Space Transportation Architecture", AIAA SPACE 2010 Conference & Exposition, 30 August-2 September 2010, AIAA 2010–8638. Archived 10 May 2013 at the Wayback Machine
- ^ "Robotic refueling Mission". Archived from the original on 10 August 2011. Retrieved 30 July 2012.
- ^ Bergin, Chris (27 September 2016). "SpaceX reveals ITS Mars game changer via colonization plan". NASASpaceFlight.com. Archived from the original on 28 September 2016. Retrieved 21 October 2016.
- ^ "Rocket Lab to capitalize on test flight success with first operational mission". 7 March 2018. Archived from the original on 7 March 2018. Retrieved 14 March 2020.
- ^ Luc Moliner, "Spot-1 Earth Observation Satellite Deorbitation" Archived 16 January 2011 at the Wayback Machine, AIAA, 2002.
- ^ "Spacecraft: Spot 3" Archived 30 September 2011 at the Wayback Machine, agi, 2003
- ^ a b c Caleb Henry (30 December 2019). "Iridium would pay to deorbit its 30 defunct satellites – for the right price". SpaceNews. Retrieved 2 January 2020.
- ^ Bill Christensen, "The Terminator Tether Aims to Clean Up Low Earth Orbit" Archived 26 November 2009 at the Wayback Machine, space.com. Retrieved 8 March 2006.
- ^ Jonathan Amos, "How satellites could 'sail' home" Archived 1 July 2009 at the Wayback Machine, BBC News, 3 May 2009.
- ^ "Safe And Efficient De-Orbit of Space Junk Without Making The Problem Worse". Space Daily. 3 August 2010. Archived from the original on 14 October 2013. Retrieved 16 September 2013.
- ^ "Experts: Active Removal Key To Countering Space Junk Threat" Peter B. de Selding, Space.com 31 October 2012.
- ^ "The big business of being a space janitor - Axios". 19 December 2019.
- ^ "OneWeb adopts 100% grappling". 12 December 2019. Archived from the original on 13 December 2019. Retrieved 22 December 2019.
- ^ Erika Carlson et al, "Final design of a space debris removal system", NASA/CR-189976, 1990.
- ^ "Intelsat Picks MacDonald, Dettwiler and Associates Ltd. for Satellite Servicing" Archived 12 May 2011 at the Wayback Machine, CNW Newswire, 15 March 2011. Retrieved 15 July 2011.
- ^ Peter de Selding, "MDA Designing In-orbit Servicing Spacecraft", Space News, 3 March 2010. Retrieved 15 July 2011.
- ^ Schaub, H.; Sternovsky, Z. (2013). "Active Space Debris Charging for Contactless Electrostatic Disposal". Advances in Space Research. 53 (1): 110–118. Bibcode:2014AdSpR..53..110S. doi:10.1016/j.asr.2013.10.003.
- ^ "News" Archived 27 March 2010 at the Wayback Machine, Star Inc. Retrieved 18 July 2011.
- ^ "Cleaning up Earth's orbit: A Swiss satellite tackles space junk" (Document). EPFL. 15 February 2012.
{{cite document}}
: Unknown parameter|access-date=
ignored (help); Unknown parameter|archive-date=
ignored (help); Unknown parameter|archive-url=
ignored (help); Unknown parameter|url-status=
ignored (help); Unknown parameter|url=
ignored (help) - ^ "Space Debris Removal | Cleanspace One". Space Debris Removal | Cleanspace One. Archived from the original on 2 December 2017. Retrieved 1 December 2017.
- ^ Jan, McHarg (10 August 2012). "Project aims to remove space debris". Phys.org. Archived from the original on 5 October 2013. Retrieved 3 April 2013.
- ^ "Chinese 'space cleaner' spotted grabbing and throwing away old satellite | DW | 09.02.2022". Deutsche Welle (www.dw.com).
- ^ Gough, Evan. "A Chinese space tug just grappled a dead satellite". Universe Today / phys.org. Retrieved 12 February 2022.
- ^ "VV02 – Vega uses Vespa". www.esa.int. Archived from the original on 17 October 2019. Retrieved 13 December 2019.
- ^ a b "European Space Agency to launch space debris collector in 2025". The Guardian. 9 December 2019. Archived from the original on 9 December 2019. Retrieved 13 December 2019.
- ^ Jonathan Campbell, "Using Lasers in Space: Laser Orbital Debris Removal and Asteroid Deflection" Archived 7 December 2010 at the Wayback Machine, Occasional Paper No. 20, Air University, Maxwell Air Force Base, December 2000.
- ^ Mann, Adam (26 October 2011). "Space Junk Crisis: Time to Bring in the Lasers". Wired Science. Archived from the original on 29 October 2011. Retrieved 1 November 2011.
- ^ Ivan Bekey, "Project Orion: Orbital Debris Removal Using Ground-Based Sensors and Lasers.", Second European Conference on Space Debris, 1997, ESA-SP 393, p. 699.
- ^ Justin Mullins "A clean sweep: NASA plans to carry out a spot of housework.", New Scientist, 16 August 2000.
- ^ Tony Reichhardt, "Satellite Smashers" Archived 29 July 2012 at archive.today, Air & Space Magazine, 1 March 2008.
- ^ James Mason et al, "Orbital Debris-Debris Collision Avoidance" Archived 9 November 2018 at the Wayback Machine, arXiv:1103.1690v2, 9 March 2011.
- ^ C. Bombardelli and J. Peláez, "Ion Beam Shepherd for Contactless Space Debris Removal". Journal of Guidance, Control, and Dynamics, Vol. 34, No. 3, May–June 2011, pp 916–920. http://sdg.aero.upm.es/PUBLICATIONS/PDF/2011/AIAA-51832-628.pdf Archived 9 March 2012 at the Wayback Machine
- ^ Daniel Michaels, "A Cosmic Question: How to Get Rid Of All That Orbiting Space Junk?" Archived 23 October 2017 at the Wayback Machine, Wall Street Journal, 11 March 2009.
- ^ "Company floats giant balloon concept as solution to space mess" Archived 27 September 2011 at the Wayback Machine, Global Aerospace Corp press release, 4 August 2010.
- ^ "Space Debris Removal" Archived 16 August 2010 at the Wayback Machine, Star-tech-inc.com. Retrieved 18 July 2011.
- ^ Foust, Jeff (5 October 2011). "A Sticky Solution for Grabbing Objects in Space". MIT Technology Review. Archived from the original on 4 February 2013. Retrieved 7 October 2011.
- ^ Jason Palmer, "Space junk could be tackled by housekeeping spacecraft " Archived 30 May 2018 at the Wayback Machine, BBC News, 8 August 2011
- ^ Roppolo, Michael. "Japan Launches Net Into Space to Help With Orbital Debris". CBS News. 28 February 2014
- ^ "Japan launching 'space junk' collector (Update)". Archived from the original on 2 February 2017. Retrieved 24 January 2017.
- ^ "Japan launches 'space junk' collector". The Times of India. Archived from the original on 8 February 2017. Retrieved 24 January 2017.
- ^ "Space cargo ship experiment to clean up debris hits snag". The Japan Times Online. 31 January 2017. Archived from the original on 31 January 2017. Retrieved 2 February 2017.
- ^ "A Japanese Space Junk Removal Experiment Has Failed in Orbit". Space.com. February 2017. Archived from the original on 1 February 2017. Retrieved 2 February 2017.
- ^ "Japan's troubled 'space junk' mission fails". Archived from the original on 12 February 2017. Retrieved 12 February 2017.
- ^ "E.DEORBIT Mission". ESA. 12 April 2017. Retrieved 6 October 2018. [permanent dead link]
- ^ Biesbroek, 2012 "Introduction to e.Deorbit" Archived 17 September 2014 at the Wayback Machine. e.deorbit symposium. 6 May 2014
- ^ Clark, Stephen (1 April 2018). "Eliminating space junk could take step toward reality with station cargo launch". Spaceflight Now. Archived from the original on 8 April 2018. Retrieved 6 April 2018.
- ^ "UN Space Debris Mitigation Guidelines" Archived 6 October 2011 at the Wayback Machine, UN Office for Outer Space Affairs, 2010.
- ^ Theresa Hitchens, "COPUOS Wades into the Next Great Space Debate" Archived 26 December 2008 at the Wayback Machine, The Bulletin of the Atomic Scientists, 26 June 2008.
- ^ a b "U.S. Government Orbital Debris Mitigation Standard Practices" (PDF). United States Federal Government. Archived (PDF) from the original on 16 February 2013. Retrieved 28 November 2013.
- ^ a b "Orbital Debris – Important Reference Documents." Archived 20 March 2009 at the Wayback Machine, NASA Orbital Debris Program Office.
- ^ a b c "Mitigating space debris generation". European Space Agency. 19 April 2013. Archived from the original on 26 April 2013. Retrieved 13 December 2019.
- ^ "Compliance of Rocket Upper Stages in GTO with Space Debris Mitigation Guidelines". Space Safety Magazine. 18 July 2013. Retrieved 16 February 2016.
- ^ a b c Foust, Jeff (9 December 2019). "U.S. government updates orbital debris mitigation guidelines". SpaceNews. Retrieved 14 December 2019.
the first update of the guidelines since their publication in 2001, and reflect a better understanding of satellite operations and other technical issues that contribute to the growing population of orbital debris. ...[The new 2019 guidelines] did not address one of the biggest issues regarding debris mitigation: whether to reduce the 25-year timeframe for deorbiting satellites after the end of their mission. Many in the space community believe that timeframe should be less than 25 years
- ^ "U.S. Government Orbital Debris Mitigation Standard Practices" (PDF). United States Federal Government. Archived (PDF) from the original on 5 April 2004. Retrieved 13 December 2019.
- ^ a b c Stokes; et al. Flohrer, T.; Schmitz, F. (eds.). STATUS OF THE ISO SPACE DEBRIS MITIGATION STANDARDS (2017) (PDF). 7th European Conference on Space Debris, Darmstadt, Germany, 18–21 April 2017. ESA Space Debris Office. Archived (PDF) from the original on 13 December 2019. Retrieved 13 December 2019.
- ^ Howell, E. (2013). Experts Urge Removal of Space Debris From Orbit. Universe Today. Retrieved from http://www.universetoday.com/101790/experts-urge-removal-of-space-debris-from-orbit/ Archived 5 March 2014 at the Wayback Machine
- ^ Space debris mitigation measures in India Archived 13 December 2019 at the Wayback Machine, Acta Astronautica, February 2006, Vol. 58, Issue 3, pages 168-174, DOI.
- ^ E A Taylor and J R Davey, "Implementation of debris mitigation using International Organization for Standardization (ISO) standards" Archived 9 March 2012 at the Wayback Machine, Proceedings of the Institution of Mechanical Engineers: G, Volume 221 Number 8 (1 June 2007), pp. 987 – 996.
- ^ a b Taylor, Jared B. (2011). "Tragedy of the space commons: a market mechanism solution to the space debris problem". The Columbia Journal of Transnational Law. 50: 253–279.
- ^ Chaddha, Shane (2010). "A Tragedy of the Space Commons?". Rochester, NY. doi:10.2139/ssrn.1586643. SSRN 1586643.
{{cite journal}}
: Cite journal requires|journal=
(help) - ^ a b c Johnson-Freese, Joan; Weeden, Brian (27 January 2012). "Application of Ostrom's Principles for Sustainable Governance of Common-Pool Resources to Near-Earth Orbit". Global Policy. 3 (1): 72–81. doi:10.1111/j.1758-5899.2011.00109.x. ISSN 1758-5880.
- ^ Morin, Jean‐Frédéric; Richard, Benjamin (5 June 2021). "Astro‐Environmentalism: Towards a Polycentric Governance of Space Debris". Global Policy. 12 (4): 568–573. doi:10.1111/1758-5899.12950. ISSN 1758-5880. S2CID 236171500.
- ^ a b c Kaiser, Stefan A. (2015). "Legal and policy aspects of space situational awareness". Space Policy. 31: 5–12. Bibcode:2015SpPol..31....5K. doi:10.1016/j.spacepol.2014.11.002. ISSN 0265-9646.
- ^ a b c d Lambach, Daniel; Wesel, Luca (2021). "Tackling the Space Debris Problem: A Global Commons Perspective". Proc. 8th European Conference on Space Debris.
- ^ a b Skinner, Mark A. (2017). "Orbital debris: What are the best near-term actions to take? A view from the field". Journal of Space Safety Engineering. 4 (2): 105–111. doi:10.1016/j.jsse.2017.02.002. ISSN 2468-8967.
- ^ Sheetz, Michael (10 August 2020). "SpaceX is manufacturing 120 Starlink internet satellites per month". CNBC. Retrieved 14 March 2022.
- ^ The Space Economy in Figures: How Space Contributes to the Global Economy. 2019. doi:10.1787/c5996201-en. ISBN 9789264696549. S2CID 242966859. Retrieved 14 March 2022.
{{cite book}}
:|website=
ignored (help) - ^ Chow, Tiffany; Weeden, Brian C. (2013). "Engaging All Stakeholders in Space Sustainability Governance Initiatives" (PDF). https://swfound.org.
{{cite web}}
: External link in
(help)|website=
- ^ a b Williamson, Ray A. (2012). "Assuring the sustainability of space activities". Space Policy. 28 (3): 154–160. Bibcode:2012SpPol..28..154W. doi:10.1016/j.spacepol.2012.06.010. ISSN 0265-9646.
- ^ a b Evans, J.P. (2011). Environmental Governance. Hoboken: Taylor & Francis.
- ^ Research and Markets (2018). "Global Space Debris Monitoring and Removal Market 2018-2022 with Airbus, ASTROSCALE, Boeing, Lockheed Martin, Northrop Grumman & RSC Energia Dominating". www.prnewswire.com. Retrieved 14 March 2022.
- ^ Moore, Adrian; van Burken, Rebecca (2021). "As Commercial Space Travel Becomes Reality, Debris and Space Traffic Management Becomes More Important". Reason Foundation. Retrieved 14 March 2022.
- ^ Newman, Christopher J.; Williamson, Mark (2018). "Space Sustainability: Reframing the Debate". Space Policy. 46: 30–37. Bibcode:2018SpPol..46...30N. doi:10.1016/j.spacepol.2018.03.001. ISSN 0265-9646. S2CID 158678917.
- ^ Carlsson, Lars Gunnar; Sandström, Annica Charlotte (2007). "Network Governance of the Commons". International Journal of the Commons. 2 (1): 33-54. doi:10.18352/ijc.20. ISSN 1875-0281.
- ^ Carter, Jamie (27 February 2022). "As Chinese Rocket Strikes Moon This Week We Need To Act Now To Prevent New Space Junk Around The Moon Say Scientists". Forbes. Retrieved 9 April 2022.
- ^ "Space: The Final Frontier of Environmental Disasters?". Wired. 15 July 2013. Retrieved 9 April 2022.
- ^ "This 30-Year-Old Sci-Fi Epic Is a Saga for Our Times". Wired. ISSN 1059-1028. Retrieved 19 June 2020.
- ^ Sinha-Roy, Piya (21 July 2013). "'Gravity' gets lift at Comic-Con as director Cuaron leaps into space". Reuters. Retrieved 9 June 2020.
- ^ Brady, Matt (3 July 2019). "Conservation of Momentum in Netflix's Love, Death & Robots". The Science Of. Retrieved 21 April 2021.
Bibliography
- Donald Kessler (Kessler 1991), "Collisional Cascading: The Limits of Population Growth in Low Earth Orbit", Advances in Space Research, Volume 11 Number 12 (December 1991), pp. 63–66.
- Donald Kessler (Kessler 1971), "Estimate of Particle Densities and Collision Danger for Spacecraft Moving Through the Asteroid Belt", Physical Studies of Minor Planets, NASA SP-267, 1971, pp. 595–605. Bibcode 1971NASSP.267..595K.
- Donald Kessler (Kessler 2009), webpages.charter.net, 8 March 2009.
- Donald Kessler (Kessler 1981), "Sources of Orbital Debris and the Projected Environment for Future Spacecraft", Journal of Spacecraft, Volume 16 Number 4 (July–August 1981), pp. 357–60.
- Donald Kessler and Burton Cour-Palais (Kessler 1978), "Collision Frequency of Artificial Satellites: The Creation of a Debris Belt" Journal of Geophysical Research, Volume 81, Number A6 (1 June 1978), pp. 2637–46.
- Donald Kessler and Phillip Anz-Meador, "Critical Number of Spacecraft in Low Earth Orbit: Using Fragmentation Data to Evaluate the Stability of the Orbital Debris Environment", Presented and the Third European Conference on Space Debris, March 2001.
- (Technical), "Orbital Debris: A Technical Assessment" National Academy of Sciences, 1995. ISBN 0-309-05125-8.
- Jim Schefter, "The Growing Peril of Space Debris" Popular Science, July 1982, pp. 48–51.
Further reading
- "What is Orbital Debris?", Center for Orbital and Reentry Debris Studies, Aerospace Corporation
- Committee for the Assessment of NASA's Orbital Debris Programs (2011). Limiting Future Collision Risk to Spacecraft: An Assessment of NASA's Meteoroid and Orbital Debris Programs. Washington, D.C.: National Research Council. ISBN 978-0-309-21974-7.
- Klotz, Irene (1 September 2011). "Space junk reaching 'tipping point,' report warns". Reuters. Retrieved 2 September 2011. News item summarizing the above report
- Steven A. Hildreth and Allison Arnold. Threats to U.S. National Security Interests in Space: Orbital Debris Mitigation and Removal. Washington, D.C.: Congressional Research Service, 8 January 2014.
- David Leonard, "The Clutter Above", Bulletin of the Atomic Scientists, July/August 2005.
- Patrick McDaniel, "A Methodology for Estimating the Uncertainty in the Predicted Annual Risk to Orbiting Spacecraft from Current or Predicted Space Debris Population". National Defense University, 1997.
- "Interagency Report on Orbital Debris, 1995", National Science and Technology Council, November 1995.
- Nickolay Smirnov, Space Debris: Hazard Evaluation and Mitigation. Boca Raton, FL: CRC Press, 2002, ISBN 0-415-27907-0.
- Richard Talcott, "How We Junked Up Outer Space", Astronomy, Volume 36, Issue 6 (June 2008), pp. 40–43.
- "Technical report on space debris, 1999", United Nations, 2006. ISBN 92-1-100813-1.
- Robin Biesbroek (2015). Active Debris Removal in Space: How to Clean the Earth's Environment from Space Debris. CreateSpace. ISBN 978-1-5085-2918-7.
- Khatchadourian, Raffi, "The Trash Nebula: Millions of man-made artifacts are circling Earth. Will one of them cause a disaster?", 28 September 2020, pp. 44–52, 54–55. "By one estimate, there are a hundred million bits of debris that are a millimetre in size, a hundred million as small as a micron. We live in a corona of trash. [T]he problem, if ignored, could destroy all the satellites that orbit near the Earth – a loss that would be more acutely felt as humanity increasingly relied on space." (p. 47.)
External links

- Sativew – Tracking Space Junk in real time
- NASA Orbital Debris Program Office
- ESA Space Debris Office Archived 5 September 2012 at the Wayback Machine
- "Space: the final junkyard", documentary film
- Would a Saturn-like ring system around planet Earth remain stable? Abdul Ahad
- EISCAT Space Debris during the international polar year
- Intro to mathematical modeling of space debris flux
- SOCRATES: A free daily service predicting close encounters on orbit between satellites and debris orbiting Earth
- A summary of current space debris by type and orbit
- Space Junk Astronomy Cast episode No. 82, includes full transcript
- Paul Maley's Satellite Page – Space debris (with photos)
- Space Debris Illustrated: The Problem in Pictures
- PACA: Space Debris
- IEEE – The Growing Threat of Space Debris
- The Threat of Orbital Debris and Protecting NASA Space Assets from Satellite Collisions
- Orbital Debris
- Space Age Wasteland: Debris in Orbit Is Here to Stay; Scientific American; 2012
- United States Space Surveillance Network
- PATENDER: GMV’S Trailblazing low-gravity space-debris capture system
- Space Junk Infographic
- Stuff In Space
- Astria Graph