Potassium channel
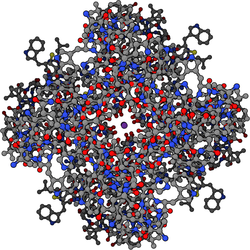
In the field of cell biology, potassium channels are the most widely distributed type of ion channel and are found in virtually all living organisms.[1] They form potassium-selective pores that span cell membranes. Furthermore potassium channels are found in most cell types and control a wide variety of cell functions.[2][3]
Function
In excitable cells such as neurons, they shape action potentials and set the resting membrane potential.
By contributing to the regulation of the action potential duration in cardiac muscle, malfunction of potassium channels may cause life-threatening arrhythmias.
They also regulate cellular processes such as the secretion of hormones (e.g., insulin release from beta-cells in the pancreas) so their malfunction can lead to diseases (such as diabetes).
Types
There are four major classes of potassium channels:
- Calcium-activated potassium channel - open in response to the presence of calcium ions or other signalling molecules.
- Inwardly rectifying potassium channel - passes current (positive charge) more easily in the inward direction (into the cell).
- Tandem pore domain potassium channel - are constitutively open or possess high basal activation, such as the "resting potassium channels" or "leak channels" that set the negative membrane potential of neurons. When open, they allow potassium ions to cross the membrane at a rate which is nearly as fast as their diffusion through bulk water.
- Voltage-gated potassium channel - are voltage-gated ion channels that open or close in response to changes in the transmembrane voltage.
The following table contains a comparison of the major classes of potassium channels with representative examples (for a complete list of channels within each class, see the respective class pages).
Class | Subclasses | Function | Blockers | Activators |
---|---|---|---|---|
Calcium-activated 6T & 1P |
|
| ||
Inwardly rectifying 2T & 1P |
|
|
|
|
|
|
|
| |
|
||||
Tandem pore domain 4T & 2P |
|
|||
Voltage-gated 6T & 1P |
|
|
|
Structure

Potassium channels have a tetrameric structure in which four identical protein subunits associate to form a fourfold symmetric (C4) complex arranged around a central ion conducting pore (i.e., a homotetramer). Alternatively four related but not identical protein subunits may associate to form heterotetrameric complexes with pseudo C4 symmetry. All potassium channel subunits have a distinctive pore-loop structure that lines the top of the pore and is responsible for potassium selective permeability.
There are over 80 mammalian genes that encode potassium channel subunits. However potassium channels found in bacteria are amongst the most studied of ion channels, in terms of their molecular structure. Using X-ray crystallography,[23][24] profound insights have been gained into how potassium ions pass through these channels and why (smaller) sodium ions do not.[25] The 2003 Nobel Prize for Chemistry was awarded to Rod MacKinnon for his pioneering work in this area.[26]
Selectivity filter

Potassium ion channels remove the hydration shell from the ion when it enters the selectivity filter. The selectivity filter is formed by five residues (TVGYG-in prokaryotic species) in the P loop from each subunit which have their electro-negative carbonyl oxygen atoms aligned towards the centre of the filter pore and form an anti-prism similar to a water solvating shell around each potassium binding site. The distance between the carbonyl oxygens and potassium ions in the binding sites of the selectivity filter is the same as between water oxygens in the first hydration shell and a potassium ion in water solution. Passage of sodium ions would be energetically unfavorable since the strong interactions between the filter and pore helix would prevent the channel from collapsing to the smaller sodium ion size. The selectivity filter opens towards the extracellular solution, exposing four carbonyl oxygens in a glycine residue (Gly79 in KcsA). The next residue towards the extracellular side of the protein is the negatively charged Asp80 (KcsA). This residue together with the five filter residues form the pore that connects the water filled cavity in the centre of the protein with the extracellular solution.[28]
The carbonyl oxygens are strongly electro-negative and cation attractive. The filter can accommodate potassium ions at 4 sites usually labelled S1 to S4 starting at the extracellular side. In addition one ion can bind in the cavity at a site called SC or one or more ions at the extracellular side at more or less well defined sites called S0 or Sext. Several different occupancies of these sites are possible. Since the X-ray structures are averages over many molecules, it is, however, not possible to deduce the actual occupancies directly from such a structure. In general, there is some disadvantage due to electrostatic repulsion to have two neighbouring sites occupied by ions. The mechanism for ion translocation in KcsA has been studied extensively by simulation techniques. A complete map of the free energies of the 24=16 states (characterised by the occupancy of the S1, S2, S3 and S4 sites) has been calculated with molecular dynamics simulations resulting in the prediction of an ion conduction mechanism in which the two doubly occupied states (S1, S3) and (S2, S4) play an essential role. The two extracellular states, Sext and S0, were found in a better resolved structure of KcsA at high potassium concentration. In free energy calculations the entire ionic pathway from the cavity, through the four filter sites out to S0 and Sext was covered in MD simulations. The amino acids sequence of the selectivity filter of potassium ion channels is conserved with the exception that an isoleucine residue in eukaryotic potassium ion channels often is substituted with a valine residue in prokaryotic channels.[28]
Central Cavity
A 10 Å wide central pore is located near the center of the transmembrane channel where the energy barrier is highest for the transversing ion due to the hydrophobity of the channel wall. The water-filled cavity and the polar C-terminus of the pore helices ease the energetic barrier for the ion. Repulsion by preceding multiple potassium ions is thought to aid the throughput of the ions. The presence of the cavity can be understood intuitively as one of the channel's mechanisms for overcoming the dielectric barrier, or repulsion by the low-dielectric membrane, by keeping the K+ ion in a watery, high-dielectric environment.
Blockers
Potassium channel blockers, such as 4-aminopyridine and 3,4-diaminopyridine, have been investigated for the treatment of conditions such as multiple sclerosis.[29]
Muscarinic potassium channel
See also G protein-coupled inwardly-rectifying potassium channel
Some types of potassium channels are activated by muscarinic receptors and these are called muscarinic potassium channels (IKACh). These channels are a heterotetramer composed of two GIRK1 and two GIRK4 subunits.[30][31] Examples are potassium channels in the heart, which, when activated by parasympathetic signals through M2 muscarinic receptors, causes an outward current of potassium which slows down the heart rate.[32][33]
See also
References
- ^ Littleton JT, Ganetzky B (2000). "Ion channels and synaptic organization: analysis of the Drosophila genome". Neuron. 26 (1): 35–43. doi:10.1016/S0896-6273(00)81135-6. PMID 10798390.
- ^ Hille, Bertil (2001). "Chapter 5: Potassium Channels and Chloride Channels". Ion channels of excitable membranes. Sunderland, Mass: Sinauer. pp. 131–168. ISBN 0-87893-321-2.
- ^ Jessell, Thomas M.; Kandel, Eric R.; Schwartz, James H. (2000). "Chapter 6: Ion Channels". Principles of Neural Science (4th ed.). New York: McGraw-Hill. pp. 105–124. ISBN 0-8385-7701-6.
{{cite book}}
: CS1 maint: multiple names: authors list (link) - ^ Rang, HP (2003). Pharmacology. Edinburgh: Churchill Livingstone. p. 60. ISBN 0-443-07145-4.
- ^ Kobayashi T, Washiyama K, Ikeda K (2006). "Inhibition of G protein-activated inwardly rectifying K+ channels by ifenprodil". Neuropsychopharmacology. 31 (3): 516–24. doi:10.1038/sj.npp.1300844. PMID 16123769.
{{cite journal}}
: CS1 maint: multiple names: authors list (link) - ^ a b c d e f Enyedi P, Czirják G (2010). "Molecular background of leak K+ currents: two-pore domain potassium channels". Physiological Reviews. 90 (2): 559–605. doi:10.1152/physrev.00029.2009. PMID 20393194.
- ^ a b c d e f Lotshaw DP (2007). "Biophysical, pharmacological, and functional characteristics of cloned and native mammalian two-pore domain K+ channels". Cell Biochemistry and Biophysics. 47 (2): 209–56. doi:10.1007/s12013-007-0007-8. PMID 17652773.
- ^ Fink M, Lesage F, Duprat F, Heurteaux C, Reyes R, Fosset M, Lazdunski M (1998). "A neuronal two P domain K+ channel stimulated by arachidonic acid and polyunsaturated fatty acids". The EMBO Journal. 17 (12): 3297–308. doi:10.1093/emboj/17.12.3297. PMC 1170668. PMID 9628867.
{{cite journal}}
: CS1 maint: multiple names: authors list (link) - ^ Goldstein SA, Bockenhauer D, O'Kelly I, Zilberberg N (2001). "Potassium leak channels and the KCNK family of two-P-domain subunits". Nature Reviews Neuroscience. 2 (3): 175–84. doi:10.1038/35058574. PMID 11256078.
{{cite journal}}
: CS1 maint: multiple names: authors list (link) - ^ Sano Y, Inamura K, Miyake A, Mochizuki S, Kitada C, Yokoi H, Nozawa K, Okada H, Matsushime H, Furuichi K (2003). "A novel two-pore domain K+ channel, TRESK, is localized in the spinal cord". The Journal of Biological Chemistry. 278 (30): 27406–12. doi:10.1074/jbc.M206810200. PMID 12754259.
{{cite journal}}
: CS1 maint: multiple names: authors list (link) CS1 maint: unflagged free DOI (link) - ^ Czirják G, Tóth ZE, Enyedi P (2004). "The two-pore domain K+ channel, TRESK, is activated by the cytoplasmic calcium signal through calcineurin". The Journal of Biological Chemistry. 279 (18): 18550–8. doi:10.1074/jbc.M312229200. PMID 14981085.
{{cite journal}}
: CS1 maint: multiple names: authors list (link) CS1 maint: unflagged free DOI (link) - ^ Kindler CH, Yost CS, Gray AT (1999). "Local anesthetic inhibition of baseline potassium channels with two pore domains in tandem". Anesthesiology. 90 (4): 1092–102. doi:10.1097/00000542-199904000-00024. PMID 10201682.
{{cite journal}}
: CS1 maint: multiple names: authors list (link) - ^ a b c Meadows HJ, Randall AD (2001). "Functional characterisation of human TASK-3, an acid-sensitive two-pore domain potassium channel". Neuropharmacology. 40 (4): 551–9. doi:10.1016/S0028-3908(00)00189-1. PMID 11249964.
- ^ Kindler CH, Paul M, Zou H, Liu C, Winegar BD, Gray AT, Yost CS (2003). "Amide local anesthetics potently inhibit the human tandem pore domain background K+ channel TASK-2 (KCNK5)". Journal of Pharmacology and Experimental Therapeutics. 306 (1): 84–92. doi:10.1124/jpet.103.049809. PMID 12660311.
{{cite journal}}
: CS1 maint: multiple names: authors list (link) - ^ Punke MA, Licher T, Pongs O, Friederich P (2003). "Inhibition of human TREK-1 channels by bupivacaine". Anesthesia & Analgesia. 96 (6): 1665–73. doi:10.1213/01.ANE.0000062524.90936.1F. PMID 12760993.
{{cite journal}}
: zero width space character in|doi=
at position 9 (help)CS1 maint: multiple names: authors list (link) - ^ Lesage F, Guillemare E, Fink M, Duprat F, Lazdunski M, Romey G, Barhanin J (1996). "TWIK-1, a ubiquitous human weakly inward rectifying K+ channel with a novel structure". The EMBO Journal. 15 (5): 1004–11. PMC 449995. PMID 8605869.
{{cite journal}}
: CS1 maint: multiple names: authors list (link) - ^ Duprat F, Lesage F, Fink M, Reyes R, Heurteaux C, Lazdunski M (1997). "TASK, a human background K+ channel to sense external pH variations near physiological pH". The EMBO Journal. 16 (17): 5464–71. doi:10.1093/emboj/16.17.5464. PMC 1170177. PMID 9312005.
{{cite journal}}
: CS1 maint: multiple names: authors list (link) - ^ Reyes R, Duprat F, Lesage F, Fink M, Salinas M, Farman N, Lazdunski M (1998). "Cloning and expression of a novel pH-sensitive two pore domain K+ channel from human kidney". The Journal of Biological Chemistry. 273 (47): 30863–9. doi:10.1074/jbc.273.47.30863. PMID 9812978.
{{cite journal}}
: CS1 maint: multiple names: authors list (link) CS1 maint: unflagged free DOI (link) - ^ Meadows HJ, Benham CD, Cairns W, Gloger I, Jennings C, Medhurst AD, Murdock P, Chapman CG (2000). "Cloning, localisation and functional expression of the human orthologue of the TREK-1 potassium channel". Pflügers Archiv : European Journal of Physiology. 439 (6): 714–22. doi:10.1007/s004240050997. PMID 10784345.
{{cite journal}}
: CS1 maint: multiple names: authors list (link) - ^ Patel AJ, Honoré E, Lesage F, Fink M, Romey G, Lazdunski M (1999). "Inhalational anesthetics activate two-pore-domain background K+ channels". Nature Neuroscience. 2 (5): 422–6. doi:10.1038/8084. PMID 10321245.
{{cite journal}}
: CS1 maint: multiple names: authors list (link) - ^ Gray AT, Zhao BB, Kindler CH, Winegar BD, Mazurek MJ, Xu J, Chavez RA, Forsayeth JR, Yost CS (2000). "Volatile anesthetics activate the human tandem pore domain baseline K+ channel KCNK5". Anesthesiology. 92 (6): 1722–30. doi:10.1097/00000542-200006000-00032. PMID 10839924.
{{cite journal}}
: CS1 maint: multiple names: authors list (link) - ^ Rogawski MA, Bazil CW (2008). "New molecular targets for antiepileptic drugs: alpha2delta, SV2A, and Kv7/KCNQ/M potassium channels". Curr Neurol Neurosci Rep. 8 (4): 345–52. doi:10.1007/s11910-008-0053-7. PMC 2587091. PMID 18590620.
{{cite journal}}
: Unknown parameter|month=
ignored (help) - ^ Doyle DA, Morais Cabral J, Pfuetzner RA, Kuo A, Gulbis JM, Cohen SL, Chait BT, MacKinnon R (1998). "The structure of the potassium channel: molecular basis of K+ conduction and selectivity". Science. 280 (5360): 69–77. doi:10.1126/science.280.5360.69. PMID 9525859.
{{cite journal}}
: CS1 maint: multiple names: authors list (link) - ^ MacKinnon R, Cohen SL, Kuo A, Lee A, Chait BT (1998). "Structural conservation in prokaryotic and eukaryotic potassium channels". Science. 280 (5360): 106–9. doi:10.1126/science.280.5360.106. PMID 9525854.
{{cite journal}}
: CS1 maint: multiple names: authors list (link) - ^ Armstrong C (1998). "The vision of the pore". Science. 280 (5360): 56–7. doi:10.1126/science.280.5360.56. PMID 9556453.
- ^ "The Nobel Prize in Chemistry 2003". The Nobel Foundation. Retrieved 2007-11-16.
- ^ Zhou Y, Morais-Cabral JH, Kaufman A, MacKinnon R (2001). "Chemistry of ion coordination and hydration revealed by a K+ channel-Fab complex at 2.0 Â resolution". Nature. 414 (6859): 43–8. doi:10.1038/35102009. PMID 11689936.
{{cite journal}}
: CS1 maint: multiple names: authors list (link) - ^ a b Hellgren M, Sandberg L, Edholm O (2006). "A comparison between two prokaryotic potassium channels (KirBac1.1 and KcsA) in a molecular dynamics (MD) simulation study". Biophys. Chem. 120 (1): 1–9. doi:10.1016/j.bpc.2005.10.002. PMID 16253415.
{{cite journal}}
: CS1 maint: multiple names: authors list (link) - ^ Judge SI, Bever CT (2006). "Potassium channel blockers in multiple sclerosis: neuronal Kv channels and effects of symptomatic treatment". Pharmacol. Ther. 111 (1): 224–59. doi:10.1016/j.pharmthera.2005.10.006. PMID 16472864.
{{cite journal}}
: Unknown parameter|month=
ignored (help) - ^ Krapivinsky G, Gordon EA, Wickman K, Velimirović B, Krapivinsky L, Clapham DE (1995). "The G-protein-gated atrial K+ channel IKACh is a heteromultimer of two inwardly rectifying K+-channel proteins". Nature. 374 (6518): 135–41. doi:10.1038/374135a0. PMID 7877685.
{{cite journal}}
: CS1 maint: multiple names: authors list (link) - ^ Corey S, Krapivinsky G, Krapivinsky L, Clapham DE (1998). "Number and stoichiometry of subunits in the native atrial G-protein-gated K+ channel, IKACh". J. Biol. Chem. 273 (9): 5271–8. doi:10.1074/jbc.273.9.5271. PMID 9478984.
{{cite journal}}
: CS1 maint: multiple names: authors list (link) CS1 maint: unflagged free DOI (link) - ^ Kunkel MT, Peralta EG (1995). "Identification of domains conferring G protein regulation on inward rectifier potassium channels". Cell. 83 (3): 443–9. doi:10.1016/0092-8674(95)90122-1. PMID 8521474.
- ^ Wickman K, Krapivinsky G, Corey S, Kennedy M, Nemec J, Medina I, Clapham DE (1999). "Structure, G protein activation, and functional relevance of the cardiac G protein-gated K+ channel, IKACh". Ann. N. Y. Acad. Sci. 868: 386–98. doi:10.1111/j.1749-6632.1999.tb11300.x. PMID 10414308.
{{cite journal}}
: CS1 maint: multiple names: authors list (link)
External links
- Potassium+Channels at the U.S. National Library of Medicine Medical Subject Headings (MeSH)
- Neuromuscular Disease Center (2008-03-04). "Potassium Channels". Washington University in St. Louis. Retrieved 2008-03-10.
{{cite web}}
: Cite has empty unknown parameter:|coauthors=
(help) - UMich Orientation of Proteins in Membranes families/superfamily-8